Abstract
Connexins are a family of gap junction forming proteins widely expressed by mammalian cells. They assemble into hexameric hemichannels, which can either function independently or dock with opposing hemichannels on apposite cells, forming a gap junction. Pannexins are structurally related to the connexins but extensive glycosylation of these channels prevents docking to form gap junctions and they function as membrane channels. Platelets express pannexin-1 and several connexin family members (Cx37, Cx40 and Cx62). These channels are permeable to molecules up to 1,000 Daltons in molecular mass and functional studies demonstrate their role in non-vesicular ATP release. Channel activation is regulated by (patho)physiological stimuli, such as mechanical stimulation, making them attractive potential drug targets for the management of arterial thrombosis. This review explores the structure and function of platelet pannexin-1 and connexins, the mechanisms by which they are gated and their therapeutic potential.
Introduction
Platelets circulate in a complex environment, interacting with other blood cells and the vessel wall. Vascular injury or rupture of atherosclerotic plaques expose thrombogenic molecules (e.g., vWF and collagen) that initiate platelet recruitment, activation and thrombus formation, resulting in hemostasis or thrombosis, respectively. The platelet response to injury is rapid and proportionate, orchestrated by complex interplay of activatory and inhibitory signals [Citation1]. Conversely, arterial thrombosis arises from pathological platelet activation and leads to myocardial infarction and stroke. The discovery of platelet connexin (Cx) gap junction proteins and a structurally related pannexin-1 (Panx1) channel on platelets may provide previously unrecognized mechanisms for communication between platelets to regulate the thrombotic response. This review explores recent developments in the structure and function of platelet connexins and Panx1, the potential roles that these proteins may play in platelet-related diseases and their prospects as antiplatelet targets.
Structure and Function of Connexins and Pannexins
Connexins have been studied widely and members of this family of 21 proteins [Citation2] play critical roles in regulating signal transduction and propagating calcium waves in a range of tissues. Connexin gap junctions ensure coordinated responses by neighboring cells (reviewed in [Citation3]) since connexin mediated intercellular signaling is limited only by the rate of diffusion of signal molecules conveyed by these channels. Connexins form a broad family of proteins and are denoted by their molecular mass (i.e., Cx32 displays an apparent molecular mass of 32 kDa when analyzed by SDS-PAGE). Connexin monomers assemble into hexameric hemichannels and are trafficked to the plasma membrane where docking of two hemichannels on apposite cells forms a gap junction, allowing intercellular flow of molecules up to 1000 Daltons in size. Connexin hemichannels may be formed by a single member of the connexin family (homomeric) or from a mixture of family members (heteromeric) [Citation4]. Docking of homomeric or heteromeric hemichannels, gives rise to assembly of homotypic and heterotypic gap junctions, respectively (Summarized in ). Intermixing of connexins in this way has been shown to influence the nature of intercellular signal transduction; for example, Cx26:Cx37 heterotypic gap junctions have enhanced permeability to cGMP over cAMP, compared with Cx37 homotypic junctions [Citation4]. The functional significance of potential connexin intermixing in platelets is not understood, although it is interesting to note the substantial and similar impact of the inhibition or deletion of each of Cx37, 40 and 62, which may suggest that platelet connexins may collaborate in these cells [Citation5–7]. Connexins also function as hemichannels at the plasma membrane, providing a route for release of cytosolic signaling molecules, such as ATP [Citation8]. Hemichannel and gap junction modalities may provide distinct roles for connexins in freely circulating platelets and within thrombi, respectively.
Figure 1. Expression and assembly of platelet connexins and pannexin-1. (a) Platelets express Cx37, Cx40 and Cx62 and pannexin-1 (Panx1). Connexins form nonselective hexameric hemichannels and pannexin-1 forms heptameric anion-selective channels that are detected at the plasma membrane. (b) Docking of two apposite hemichannels forms a gap junction that facilitates intercellular communication. (c) Gap junctions form between two hemichannels by either homotypic (e.g., Cx37:Cx37) or heterotypic (e.g., Cx37:Cx40) assembly, which may influence gap junction permeability. The molecular identity of platelet gap junctions and whether they are homo/heterotypic has not been studied. Panx1 cannot form gap junctions due to glycosylation on its second extracellular loop. Created in BioRender
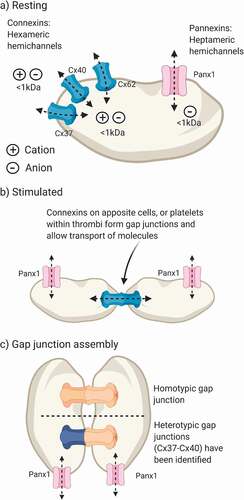
Pannexins were first reported in 2000 by Panchina and colleagues, during a genetic screen for invertebrate gap junction homologues [Citation9]. This family of three proteins displays distinct expression patterns whereby Panx1 is ubiquitously expressed, pannexin-2 is restricted to the brain and central nervous system and pannexin-3 is predominantly found in bone and skin cells [Citation10]. Early studies of Panx1 suggested that these proteins assemble into hexameric channels before becoming glycosylated and trafficked to the plasma membrane [Citation11]. However, this classical view of hexameric channels has been challenged by two independent cryo-EM studies of the Panx1 structure, which report that seven Panx1 monomers assemble to form heptameric channels [Citation12,Citation13]. These channels diverge further from connexins as glycosylation of the second extracellular loop at N254 prevents docking of apposite Panx1 channels [Citation14]. This was tested by two-electrode voltage clamp measurements of Xenopus laevis oocytes expressing Panx1. When the glycosidase PNGaseF was applied to the bath solution, N-linked glycans on Panx1 were broken down and a junctional conductance could be recorded [Citation11]. Under physiological conditions Panx1 does not form gap junctions[Citation15], rather, these proteins form anion-selective ion channels at the plasma membrane () [Citation16].
Expression and Localization of Platelet Pannexin-1 and Connexins
Platelet proteomic and transcriptomic analyses report expression of Panx1 and significant levels of Cx32, Cx37, Cx40 and Cx62 [Citation17–20]. Panx1 is the sole pannexin expressed by platelets and qPCR analysis of platelet mRNA demonstrated that Panx1 may be expressed at similar levels to the ATP-gated ion channel P2X1 [Citation21]. Protein expression, biotinylation and biochemical studies suggest that platelet Panx1 is glycosylated and the majority of these channels are expressed at the plasma membrane, where they become activated downstream of agonist-evoked platelet activation [Citation21]. Molica and colleagues reported a functional defect in subjects that have a single nucleotide polymorphism of C400A, leading to reduced collagen-evoked platelet aggregation responses [Citation22].
Of the 16 connexins screened from blood cells and megakaryocytes, notable levels of Cx37, Cx40 and Cx62 mRNA were detected from cultured megakaryocytes [Citation18]. Expression of Cx37 and Cx40 has been confirmed by Western blotting, immunocytochemistry and flow cytometry [Citation5,Citation6,Citation18]. More recently, Cx62 was identified in human platelets, where it was shown to reside intracellularly before translocating to the plasma membrane upon stimulation by the thromboxane A2 mimetic U46619 [Citation7]. People carrying a single nucleotide polymorphism P319S in the Cx37 gene display modestly enhanced platelet activation, suggesting a role for Cx37 in regulating platelet activation [Citation5]. To date, platelet connexins have been shown to function both as hemichannels and gap junctions [Citation5–7,Citation18]. Of the platelet connexins, evidence suggests that whilst Cx37 and Cx40 cannot form heteromeric structures, they are able to assemble in a heterotypic manner between apposite cells [Citation23]. Clot retraction is a coordinated process whereby microtubules contract to facilitate wound healing [Citation24]. Interestingly, selective peptide inhibitors of either Cx37, Cx40 or Cx62 inhibit clot retraction, suggesting that there may be a role for gap junctions in the coordination of this process [Citation6,Citation7,Citation18]. Connexin intermixing has not been studied in platelets but this could serve to regulate platelet interactions within a thrombus or with other cell types.
Functional roles for Panx1 and platelet connexins have been demonstrated in vitro and in vivo. Conflicting reports indicate that global knockout of Cx37 reduces bleeding time [Citation5], whilst administration of a Cx37 blocking peptide decreases thrombus formation in vivo [Citation18]. The underlying differences between these reports remain unclear and further studies with platelet-specific deletion of Cx37 are required. Furthermore, Cx40−/- mice have increased tail bleeding times, suggesting a regulatory role for this connexin during platelet activation [Citation6]. Cx62 has been shown to contribute to thrombus formation in vivo as thrombi were significantly smaller in mice treated with a selective inhibitory peptide [Citation7]. A common platelet phenotype was observed with each connexin knockout mouse whereby fibrinogen binding, P-selectin exposure and ATP release were all reduced [Citation6,Citation7]. In vivo studies demonstrate that Panx1 contributes to both hemostasis and thrombosis with significantly elevated tail bleeding times and reduced mortality in a thromboembolic model in Panx1−/- mice [Citation25]. Extensive evidence therefore supports the notion that connexins, specifically Cx375[Citation18], 406 and 627, and Panx121 [Citation25,Citation26], perform important regulatory roles in platelet function in hemostasis and thrombosis.
What Messages are Conveyed by Connexins and Pannexins
Connexins and Panx1 contribute to platelet function in vivo and may represent potential antithrombotic targets. These proteins form large pores but the precise nature of the signals conveyed by platelet connexins and/or Panx1 remains unclear. Functional studies have tended to focus on the loss or transfer of cytosolic calcein, a 622 Dalton anionic fluorescent dye, in flow cytometry and FRAP (fluorescence recovery after photobleaching) assays [Citation7,Citation21]. These channels are also known to represent pathways for non-vesicular release of cytosolic ATP [Citation27,Citation28]. ATP release through Panx1 and connexin hemichannels has been demonstrated using ATP-dependent luciferin-luciferase assays in platelet suspensions [Citation6,Citation7,Citation18,Citation21]. To date, transfer of other molecules, such as ADP, cyclic nucleotides, ions (e.g., Ca2+Citation2, Cl−, Zn2+) and inositol-1,4,5-trisphosphate (IP3), has not been demonstrated in platelets or megakaryocytes. It is tempting to speculate that transfer of IP3 via gap junctions within a thrombus, akin to that within cardiac muscle[Citation29], may serve to coordinate and propagate platelet calcium waves within a growing thrombus.
Regulation of Pannexin and Connexin Channels
Connexin and pannexin channels and junctions must be appropriately regulated to prevent leakage of cytosolic content (e.g., ATP) and influx of extracellular ions (e.g., Ca2+), which would disrupt various aspects of cellular homeostasis. To this end, multiple regulatory mechanisms have evolved to control gating of pannexin and connexin channels and ultimately gap junction formation. Such mechanisms include post-translational modifications (PTMs), mechanical stimulation, ischemia, channel gating by extracellular ions and enzymatic cleavage [Citation27,Citation30–35], and are explored herein.
Phosphorylation ()
Regulation of platelet connexins and Panx1 by PTMs presents a mechanism for the efficient switching on/off of these channels in response to environmental cues. In the case of Panx1, several putative phosphorylation sites have been reported for Src Family Kinases (SFKs), protein Kinase A (PKA) and Protein Kinase C (PKC) [Citation31,Citation36,Citation37]. Collagen-evoked platelet activation leads to Panx1 phosphorylation at Y308, which is inhibited by preincubation with the SFK inhibitor PP2 [Citation25]. The functional relevance of predicted PKA and PKC sites on Panx1 are not yet clear. Regulation of platelet Cx37 and Cx40 by phosphorylation is unknown, although UniProt annotations suggest that these channels have several predicted serine, threonine and tyrosine phosphorylation sites. Related Cx43 channels are broadly expressed and deletion of this gene is embryonic lethal [Citation35]. A detailed review of Cx43 phosphorylation [Citation38] highlights 19 conserved kinase recognition motifs in the C terminus, and discusses how changes in Cx43 phosphorylation lead to alteration of hemichannel and gap junction permeability and electrophysiological properties. Cx37 has been shown to be negatively regulated by PKC in rat insulinoma cells, where PKC inhibition increased the open state probability [Citation30]. A potential link between Cx62 and PKA was recently reported; platelets incubated with a selective Cx62 inhibitory peptide had increased levels of PKA-mediated VASP phosphorylation, which occurs in a cAMP-independent manner [Citation7]. It is currently unclear whether this implicates PKA in the phosphorylation and regulation of Cx62 function, or that Cx62 is able to induce inhibitory signaling pathways that involve PKA. Further studies are required to fully understand the functional significance of phosphorylation-mediated regulation of platelet connexins and Panx1.
Figure 2. Proposed mechanisms for regulation of platelet connexins and pannexin-1. (a) Phosphorylation- Pannexin-1 (Panx1) is phosphorylated by Src Family Kinases (SFKs) at Y308, leading to channel opening. Platelet connexins have predicted phosphorylation protein kinase C (PKC) sites and Cx62 may interact with protein kinase A (PKA). (b) Gating by Ca+- Connexin hemichannels are open in the absence of extracellular Ca2+ ([Ca2+o) and close when [Ca2+]o rises to 2–4 mM. Panx1 channels open in response to a rise of intracellular Ca2+ ([Ca2+]i) that leads to ATP release and stimulation of P2X1 on platelets. (c) Enzymatic cleavage- The intracellular C-terminus of Panx1 contains a zymogen site that is cleaved by caspase-3, removing an auto inhibitory domain, facilitating channel activation and ATP release. (d) Mechanical stimulation- Mechanical deformation and elevated shear stress have been shown to stimulate Panx1 channels. Studies of cardiac muscle report increased expression of Cx43 following sustained exposure to shear stress. (e) Protein trafficking- Connexins are expressed at the plasma membrane for relatively short periods (half-life (T1/2) = 1.5–3 hours) and are trafficked and recycling in response to cellular activation. Panx1 is stably expressed at the plasma membrane with a T1/2 of approximately 24 hours. Created in BioRender
![Figure 2. Proposed mechanisms for regulation of platelet connexins and pannexin-1. (a) Phosphorylation- Pannexin-1 (Panx1) is phosphorylated by Src Family Kinases (SFKs) at Y308, leading to channel opening. Platelet connexins have predicted phosphorylation protein kinase C (PKC) sites and Cx62 may interact with protein kinase A (PKA). (b) Gating by Ca+- Connexin hemichannels are open in the absence of extracellular Ca2+ ([Ca2+o) and close when [Ca2+]o rises to 2–4 mM. Panx1 channels open in response to a rise of intracellular Ca2+ ([Ca2+]i) that leads to ATP release and stimulation of P2X1 on platelets. (c) Enzymatic cleavage- The intracellular C-terminus of Panx1 contains a zymogen site that is cleaved by caspase-3, removing an auto inhibitory domain, facilitating channel activation and ATP release. (d) Mechanical stimulation- Mechanical deformation and elevated shear stress have been shown to stimulate Panx1 channels. Studies of cardiac muscle report increased expression of Cx43 following sustained exposure to shear stress. (e) Protein trafficking- Connexins are expressed at the plasma membrane for relatively short periods (half-life (T1/2) = 1.5–3 hours) and are trafficked and recycling in response to cellular activation. Panx1 is stably expressed at the plasma membrane with a T1/2 of approximately 24 hours. Created in BioRender](/cms/asset/c31bd054-aa40-441a-bcfc-681e4ef0fa47/iplt_a_1902971_f0002_oc.jpg)
Gating by Ca2+ ()
Gating of connexin hemichannels by extracellular ([Ca2+]0) was demonstrated by atomic force microscopy studies in HeLa cells expressing recombinant Cx40. In this study hemichannels were open in Ca+ free conditions but closed when [Ca2+]0 was elevated to 3.6 mM [Citation39]. Molecular dynamic simulations based on Cx26 suggest that a [Ca+]0 sensing motif within the hemichannel pore is responsible for channel closure and this motif is conserved between connexin family members [Citation32]. Whilst, these studies suggest that physiological levels of [Ca2+] markedly reduce the open probability of connexin hemichannels, it is not clear whether this phenomenon can be overcome by other regulatory mechanisms, such as phosphorylation or mechanical stimulation. Given that connexins contribute to platelet activation in flow cytometric studies with physiological levels of [Ca2+]0 [Citation5–7,Citation18], where hemichannels are predicted to be closed, it is possible that this inhibition could be overcome by direct stimulation of the connexin by phosphorylation or mechanical forces. Panx1 channels do not appear to be directly affected by fluctuations of [Ca2+]o [Citation40], rather elevation of intracellular Ca+ ([Ca2+]o) leads to the activation of Panx1, via an unknown mechanism [Citation41]. Activation of platelet Panx1 was proposed to be mediated by collagen-evoked [Ca2+]o+ calcium signaling, leading to ATP release and amplification of the aggregation response [Citation21]. Further studies are required to unravel the mechanisms that govern Ca+-mediated gating of platelet Pannexin and Connexin channels.
Enzymatic Cleavage ()
Structural studies of Panx1 indicate that the cytoplasmic C-terminus becomes inserted into the pore region, serving as an autoinhibitory mechanism. Furthermore, this region has a zymogen site that is cleaved by caspase 3. Cleavage of the C-terminus leads to a terminal open state that is thought to release ATP as an apoptotic “find-me” signal [Citation33,Citation42]. These findings suggest that Panx1 may exist in multiple open states to respond to different stimuli. Indeed, direct stimulation of Panx1 by extracellular K+ led to channel activation with conductance of 500ps, whereas voltage-dependent activation of the channel led to a lower 50ps conductance that was not associated with ATP release [Citation43]. Such regulation may enable Panx1 to release different cargos in response to different stimuli.
Mechanical Stimulation ()
Platelets and megakaryocytes express stretch-activated Piezo 1 [Citation44], TRPM7 [Citation45,Citation46] and TRPV4 [Citation47] cation channels. This could ultimately prove to be an important mechanism for shear-dependent platelet activation in the context of stenosed vessels with pathological shear rates. Intercellular transmission of Ca[Citation2]+ signals has been reported during in vitro adhesion assays and displays an ADP-dependent component [Citation48]. The pathway for this ADP release is unclear and may be mediated by dense granule release, Panx1 channels or connexin hemichannels. Notably, erythrocyte Panx1 channels were shown to release ATP in response to mechanical deformation [Citation27]. Furthermore, sustained exposure to shear stress increased endothelial Cx40 expression [Citation49]. Platelet adhesion to collagen under arteriolar shear rates (1000s−1) has been shown to be sensitive to connexin and Panx1 inhibitors [Citation6,Citation18,Citation21].
Protein Trafficking ()
Protein trafficking represents an alternative mechanism for the regulation of pannexins and connexins. The half-life of connexin hemichannels at the plasma membrane is estimated to be between 1.5 and 5 h [Citation50]. By comparison, heterologously expressed rat Panx1 channels in human embryonic kidney cells are stably expressed at the plasma membrane, with 45% of channels remaining after 24 h [Citation11]. D-STORM imaging of platelet Cx62 demonstrated that these channels are increasingly associated with the plasma membrane following stimulation [Citation7]. Further studies are required to evaluate the role of trafficking in the dynamic regulation of connexins in response to (patho)physiological stimuli.
Therapeutic Potential
Connexins and Panx1 have been studied using the inhibitors carbenoxolone, probenecid, and spironolactone, which are also FDA-approved drugs for the management of tracheal ulcers, gouty arthritis and hypertension, respectively [Citation18,Citation21,Citation27,Citation51]. This raises the question as to whether these drugs could be repurposed to manage arterial thrombosis. One potential limitation to such an approach would be off-target effects upon other connexin family members or through inhibition of Panx1 on other cell types. For example, inhibitors of Cx40 in the circulation would not only target platelet function but also endothelial and cardiac cells that also express Cx40. It is worth noting, however, that Cx37−/- mice do not have a cardiovascular phenotype and Cx40−/- mice have reduced cardiac conduction velocity, leading to increased incidence of arrhythmias, suggesting that there may be some functional redundancy between family members [Citation52–54]. Furthermore, it is worth considering that these drugs target other receptors, introducing the potential for undesired effects. However, these drugs have been in clinical use for several decades and therefore have established safety profiles. Interestingly, orally administered probenecid achieves plasma concentrations of ≈78 µM, which is sufficient to achieve inhibition of platelet Panx1 channels [Citation21]. Probenecid has also been shown to enhance the effects of indomethacin and heparin, without increasing the plasma half-life of these drugs [Citation55,Citation56]. Pharmaco-epidemiological approaches could be employed to assess the relationship between administration of known gap junction or pannexin blockers on the incidence of cardiovascular diseases. However, this would require careful consideration of confounding factors as people diagnosed with gouty arthritis or hypertension may be predisposed to thrombotic diseases.
An alternative approach would be to develop small molecule inhibitors that target specific connexin or pannexin isoforms. Isotype specific inhibitory peptides that target the extracellular loops of connexins [Citation7,Citation57] and Panx1[Citation58] have been employed successfully to study the function of these proteins in vitro and in vivo. These target sequences could therefore be used to inform the development of small molecule inhibitors to target these proteins on platelets and manage thrombosis.
Ex vivo analyses demonstrated that mouse platelets treated with probenecid, reduced murine platelet aggregation [Citation25,Citation51]. In vitro thrombus formation assays also demonstrate reduced thrombus formation in the presence of carbenoxolone or probenecid [Citation18,Citation21]. Furthermore, potential roles for platelet gap junctions and pannexin channels in ischemic stroke are not resolved, although a middle cerebral artery occlusion stroke model demonstrated that female Panx1-deficient mice exhibited reduced neurological impairment at 48 h compared with controls [Citation59]. These data suggest that there may be therapeutic potential in repurposing drugs that are well-tolerated, for the cost-effective management of thrombosis and ischemic stroke.
Future Directions and Conclusions
To date, studies have primarily focussed on the role of platelet connexins and pannexins, however, these proteins are also expressed within the megakaryocyte. Within the bone marrow, Cx43 is the predominant connexin isoform and is expressed by megakaryocytes [Citation60]. Indeed, megakaryocytes have been shown to reduce deposition of type I collagen by osteoclasts, through Cx43-mediated interactions [Citation61]. The direct roles of connexins and pannexins during megakaryocyte development and thrombopoiesis have not been evaluated, but data suggest that platelet counts are normal in both Cx37 and panneixn-1 knockout mice [Citation5,Citation25].
Despite their structural and functional similarities, there is relatively little data available on the interactions between the pannexins and connexins. Noveielli-Kuntz and colleagues evaluated the cardiovascular phenotype of Cx40−/-, Panx1−/- and Cx40−/-Panx1−/- mice [Citation62]. Cx40-deficient mice have impaired vasodilation of aortic segments, leading to hypertension, a phenotype not observed in Panx1−/- mice. This phenotype was explored further with the double knockout and Panx1 depletion could not rescue the vascular phenotype of Cx40−/- [Citation62]. However, the impact of this model upon platelet function and thrombus formation was not assessed. In vivo thrombosis studies with Cx40−/- [Citation6] and Panx1−/- [Citation25] mice both report reduced thrombus formation, indicating therapeutic potential in targeting these channels.
Platelets express Panx1 and multiple members of the connexin gap junction family. These channels are activated following platelet stimulation, contributing to ATP release, Ca[Citation2]+ signaling and platelet activation dynamics. Our understanding of the role for platelet gap junctions during thrombus formation is currently limited. Gap junctions may represent, however, a route for rapid transmission of signals between the core and shell of a growing thrombus. Finally, these channels have been shown to be susceptible to drugs that are in clinical use, which could potentially be repurposed to manage arterial thrombosis.
Authorship Contributions
All authors contributed equally to the manuscript.
Acknowledgements
The authors were supported by a British Heart Foundation Project grant (PG/17/76/33082) and a PhD studentship (FS/17/31/32848).
Disclosure statement
The authors have no conflicts of interest to declare.
Additional information
Funding
References
- Bye AP, Unsworth AJ, Gibbins JM Platelet signaling: a complex interplay between inhibitory and activatory networks. Journal of Thrombosis and Haemostasis vol. 14 918–930 (2016). 5 https://doi.org/10.1111/jth.13302
- Söhl G Gap junctions and the connexin protein family. Cardiovasc Res vol. 62 228–232 (2004). 2 https://doi.org/10.1016/j.cardiores.2003.11.013
- Kumar NM, Gilula NB The gap junction communication channel. Cell vol. 84 381–388 (1996). 3 https://doi.org/10.1016/S0092-8674(00)81282-9
- Bevans CG, Kordel M, Rhee SK, Harris AL Isoform composition of connexin channels determines selectivity among second messengers and uncharged molecules. Journal of Biological Chemistry 273, 2808–2816 (1998). 5 https://doi.org/10.1074/jbc.273.5.2808
- Angelillo-Scherrer A Fontana P, Burnier L, Roth I, Sugamele R, Brisset A, Morel S, Nolli S, Sutter E, Chassot A, Capron C et al., Connexin 37 limits thrombus propensity by downregulating platelet reactivity. Circulation 124, 930–939 (2011). 8 https://doi.org/10.1161/CIRCULATIONAHA.110.015479
- Vaiyapuri S, Moraes LA, Sage T, Ali MS, Lewis KR, Mahaut-Smith MP, Oviedo-Orta E, Simon AM, Gibbins JM. Connexin40 regulates platelet function. Nat Commun. 4, (2013). 4 https://doi.org/10.1038/ncomms3564
- Sahli K, Flora GD, Sasikumar P, Maghrabi AH, Holbrook LM, AlOuda SK, Elgheznawy A, Sage T, Stainer AR, Adiyaman R et al. Structural, Functional and Mechanistic Insights Uncover the Fundamental Role of Orphan Connexin62 in Platelets. Blood (2020) doi:https://doi.org/10.1182/blood.2019004575.
- Lohman AW, Isakson BE Differentiating connexin hemichannels and pannexin channels in cellular ATP release. in FEBS Lett vol. 588 1379–1388 ( Elsevier, 2014). 8 https://doi.org/10.1016/j.febslet.2014.02.004
- Panchina Y, Kelmanson I, Matz M, Lukyanov K, Usman N, Lukyanov S. A ubiquitous family of putative gap junction molecules [2]. Current Biology vol. 10 (2000). 13 R473–R474 https://doi.org/10.1016/S0960-9822(00)00576-5
- Bruzzone R, Hormuzdi SG, Barbe MT, Herb A, Monyer H Pannexins, a family of gap junction proteins expressed in brain. Proc. Natl. Acad. Sci. U. S. A 100, 13644–13649 (2003). 23 https://doi.org/10.1073/pnas.2233464100
- Boassa D, Qiu F, Dahl G, Sosinsky G Trafficking dynamics of glycosylated pannexin1 proteins. Cell Commun. Adhes 15, 119–132 (2008). 1–2 https://doi.org/10.1080/15419060802013885
- Qu R, Dong L, Zhang J, Yu X, Wang L, Zhu S. Cryo-EM structure of human heptameric Pannexin 1 channel. Cell Res vol. 30 446–448 (2020). 5 https://doi.org/10.1038/s41422-020-0298-5
- Michalski K, Syrjanen JL, Henze E, Kumpf J, Furukawa H, Kawate T. The Cryo-EM structure of pannexin 1 reveals unique motifs for ion selection and inhibition. Elife 9, (2020). https://doi.org/10.7554/eLife.54670
- Yonashiro R, Sugiura A, Miyachi M, Fukuda T, Matsushita N, Inatome R, Ogata Y, Suzuki T, Dohmae N, Yanagi S et al. Mitochondrial Ubiquitin Ligase MITOL Ubiquitinates Mutant SOD1 and Attenuates Mutant SOD1-induced Reactive Oxygen Species Generation . Molecular Biology of the Cell 20, 4524–4530 (2009). 21 https://doi.org/10.1091/mbc.e09-02-0112
- Sosinsky GE, Boassa D, Dermietzel R, Duffy HS, Laird DW, MacVicar B, Naus CC, Penuela S, Scemes E, Spray DC et al. Pannexin channels are not gap junction hemichannels. Channels vol. 5 3 (2011). 193–197 https://doi.org/10.4161/chan.5.3.15765
- Ma W, Compan V, Zheng W, Martin E, North RA, Verkhratsky A, Surprenant A. Pannexin 1 forms an anion-selective channel . Pflügers Archiv – European Journal of Physiology 463, 585–592 (2012). 4 https://doi.org/10.1007/s00424-012-1077-z
- Wright JR, Amisten S, Goodall AH, Mahaut-Smith MP Transcriptomic analysis of the ion channelome of human platelets and megakaryocytic cell lines. Thrombosis and Haemostasis 116, 272–284 (2016). 8 https://doi.org/10.1160/TH15-11-0891
- Vaiyapuri S, Jones CI, Sasikumar P, Moraes LA, Munger SJ, Wright JR, Ali MS, Sage T, Kaiser WJ, Tucker KL et al. Gap junctions and connexin hemichannels underpin hemostasis and thrombosis. Circulation 125, 2479–2491 (2012). 20 https://doi.org/10.1161/CIRCULATIONAHA.112.101246
- Burkhart JM, Vaudel M, Gambaryan S, Radau S, Walter U, Martens L, Geiger J, Sickmann A,Zahedi RP. The first comprehensive and quantitative analysis of human platelet protein composition allows the comparative analysis of structural and functional pathways. Blood 120, (2012). 15 e73–e82 https://doi.org/10.1182/blood-2012-04-416594
- Lewandrowski U, Wortelkamp S, Lohrig K, Zahedi RP, Wolters DA, Walter U, Sickmann A. Platelet membrane proteomics: a novel repository for functional research. Blood 114, (2009). 1 e10–e19 https://doi.org/10.1182/blood-2009-02-203828
- Taylor KA, Wright JR, Vial C, Evans RJ, Mahaut-Smith MP Amplification of human platelet activation by surface pannexin-1 channels. Journal of Thrombosis and Haemostasis 12, 987–998 (2014). 6 https://doi.org/10.1111/jth.12566
- Molica F, Morel S, Meens M, Denis J-F, Bradfield P, Penuela S, Zufferey A, Monyer H, Imhof B, Chanson M et al. Functional role of a polymorphism in the Pannexin1 gene in collageninduced platelet aggregation . Thrombosis and Haemostasis 114, 325–336 (2015). 8 https://doi.org/10.1160/TH14-11-0981
- Koval M, Molina SA, Burt JM Mix and match: investigating heteromeric and heterotypic gap junction channels in model systems and native tissues. in FEBS Lett vol. 588 1193–1204 ( Elsevier, 2014). 8 https://doi.org/10.1016/j.febslet.2014.02.025
- Chao FC, Shepro D, Tullis JL, Belamarich FA, Curby WA. Similarities between platelet contraction and cellular motility during mitosis: role of platelet microtubules in clot retraction. J Cell Sci 20, 569–88 (1976).
- Molica F Meens MJ, Pelli G, Hautefort A, Emre Y, Imhof BA, Fontana P, Scemes E, Morel S, Kwak BR. Selective inhibition of Panx1 channels decreases hemostasis and thrombosis in vivo. Thrombosis Research 14, 56–62 (2016). https://doi.org/10.1016/j.thromres.2019.09.028
- Stierlin FB, Molica F, Reny J-L, Kwak BR, Fontana P Pannexin1 single nucleotide polymorphism and platelet reactivity in a Cohort of cardiovascular patients. Cell Communication & Adhesion 23, 11–15 (2017). 1 https://doi.org/10.1080/15419061.2017.1282469
- Locovei S, Bao L, Dahl DG Pannexin 1 in erythrocytes: function without a gap . Proceedings of the National Academy of Sciences 103, 7655–7659 (2006). 20 https://doi.org/10.1073/pnas.0601037103
- Goodenough DA, Paul DL Beyond the gap: functions of unpaired connexon channels. Nat Rev Mol Cell Biol vol. 4 285–294 (2003). 4 https://doi.org/10.1038/nrm1072
- Van Veen TAB Cardiac gap junction channels: modulation of expression and channel properties. Cardiovasc Res vol. 51 217–229 (2001). 2 https://doi.org/10.1016/S0008-6363(01)00324-8
- Jacobsen NL, Pontifex TK, Li H, Solan JL, Lampe PD, Sorgen PL, Burt JM. Regulation of Cx37 channel and growth-suppressive properties by phosphorylation. Journal of Cell Science 130, 3308–3321 (2017). 19 https://doi.org/10.1242/jcs.202572
- Weilinger NL, Tang PL, Thompson RJ Anoxia-induced NMDA receptor activation opens Pannexin channels via Src family kinases. Journal of Neuroscience 32, 12579–12588 (2012). 36 https://doi.org/10.1523/JNEUROSCI.1267-12.2012
- Lopez W, Ramachandran J, Alsamarah A, Luo Y, Harris AL, Contreras JE. Mechanism of gating by calcium in connexin hemichannels . Proceedings of the National Academy of Sciences 113, E7986–E7995 (2016). 49 https://doi.org/10.1073/pnas.1609378113
- Sandilos JK, Chiu Y-H, Chekeni FB, Armstrong AJ, Walk SF, Ravichandran KS, Bayliss DA. Pannexin 1, an ATP release channel, is activated by caspase cleavage of its pore-associated C-terminal autoinhibitory region. Journal of Biological Chemistry 287, 11303–11311 (2012). 14 https://doi.org/10.1074/jbc.M111.323378
- Solan JL, Lampe PD Specific Cx43 phosphorylation events regulate gap junction turnover in vivo. in FEBS Lett vol. 588 1423–1429 ( Elsevier, 2014). 8 https://doi.org/10.1016/j.febslet.2014.01.049
- Reaume AG, De Sousa P, Kulkarni S, Langille B, Zhu D, Davies T, Juneja S, Kidder G, Rossant J. Cardiac malformation in neonatal mice lacking connexin43 43. Science (80-.). 267, 1831–1834 (1995). 5205 https://doi.org/10.1126/science.7892609
- Barbe MT, Monyer H, Bruzzone R Cell-cell communication beyond connexins: the pannexin channels. Physiology vol. 21 103–114 (2006). 2 https://doi.org/10.1152/physiol.00048.2005
- DeLalio LJ, Billaud M, Ruddiman CA, Johnstone SR, Butcher JT, Wolpe AG, Jin X, Keller TCS, Keller AS, Rivière T et al. Constitutive SRC-mediated phosphorylation of pannexin 1 at tyrosine 198 occurs at the plasma membrane . Journal of Biological Chemistry 294, 6940–6956 (2019). 17 https://doi.org/10.1074/jbc.RA118.006982
- Solan JL, Lampe PD Connexin phosphorylation as a regulatory event linked to gap junction channel assembly. Biochimica Et Biophysica Acta - Biomembranes vol. 1711 154–163 (2005). 2 https://doi.org/10.1016/j.bbamem.2004.09.013
- Allen MJ, Gemel J, Beyer EC, Lal R Atomic force microscopy of Connexin40 gap junction hemichannels reveals calcium-dependent three-dimensional molecular topography and open-closed conformations of both the extracellular and cytoplasmic faces. Journal of Biological Chemistry 286, 22139–22146 (2011). 25 https://doi.org/10.1074/jbc.M111.240002
- Ma W, Hui H, Pelegrin P, Surprenant A Pharmacological characterization of pannexin-1 currents expressed in mammalian cells. Journal of Pharmacology and Experimental Therapeutics 328, 409–418 (2009). 2 https://doi.org/10.1124/jpet.108.146365
- Locovei S, Wang J, Dahl G Activation of pannexin 1 channels by ATP through P2Y receptors and by cytoplasmic calcium. FEBS Lett. 580, 239–244 (2006). 1 https://doi.org/10.1016/j.febslet.2005.12.004
- Chekeni FB, Elliott MR, Sandilos JK, Walk SF, Kinchen JM, Lazarowski ER, Armstrong AJ, Penuela S, Laird DW, Salvesen GS et al. Pannexin 1 channels mediate ‘find-me’ signal release and membrane permeability during apoptosis. Nature 467, 863–867 (2010). 7317 https://doi.org/10.1038/nature09413
- Wang J, Ambrosi C, Qiu F, Jackson DG, Sosinsky G, Dahl G. The membrane protein Pannexin1 forms two open-channel conformations depending on the mode of activation . Science Signaling. 7, (2014). 335 ra69–ra69 https://doi.org/10.1126/scisignal.2005431
- Ilkan Z, Wright JR, Goodall AH, Gibbins JM, Jones CI, Mahaut-Smith MP. Evidence for shear-mediated Ca2+ entry through mechanosensitive cation channels in human platelets and a megakaryocytic cell line . Journal of Biological Chemistry 292, 9204–9217 (2017). 22 https://doi.org/10.1074/jbc.M116.766196
- Carter RN, Tolhurst G, Walmsley G, Vizuete-Forster M, Miller N, Mahaut-Smith MP. Molecular and electrophysiological characterization of transient receptor potential ion channels in the primary murine megakaryocyte. The Journal of Physiology 576, 151–162 (2006). 1 https://doi.org/10.1113/jphysiol.2006.113886
- Stritt S, Nurden P, Favier R, Favier M, Ferioli S, Gotru SK, Van Eeuwijk JMM, Schulze H, Nurden AT, Lambert MP et al. Defects in TRPM7 channel function deregulate thrombopoiesis through altered cellular Mg2+ homeostasis and cytoskeletal architecture . Nature Communications. 7, (2016). 1 https://doi.org/10.1038/ncomms11097
- Abbonante V, Di Buduo CA, Gruppi C, De Maria C, Spedden E, De Acutis A, Staii C, Raspanti M, Vozzi G, Kaplan DL et al., A new path to platelet production through matrix sensing. Haematologica 102, 1150–1160 (2017). 7 https://doi.org/10.3324/haematol.2016.161562
- Nesbitt WS, Giuliano S, Kulkarni S, Dopheide SM, Harper IS, Jackson SP.. Intercellular calcium communication regulates platelet aggregation and thrombus growth. J. Cell Biol 160, 1151–1161 (2003). 7 https://doi.org/10.1083/jcb.200207119
- Vorderwülbecke BJ, Maroski J, Fiedorowicz K, Da Silva-Azevedo L, Marki A, Pries AR, Zakrzewicz A. Regulation of endothelial connexin40 expression by shear stress. Am J Physiol - Hear Circ Physiol. 302, (2012).
- Laird DW Life cycle of connexins in health and disease. Biochemical Journal vol. 394 527–543 (2006).
- Silverman W, Locovei S, Dahl G Probenecid, a gout remedy, inhibits pannexin 1 channels. Am J Physiol - Cell Physiol. 295, (2008). 3 C761–C767 https://doi.org/10.1152/ajpcell.00227.2008
- Simon AM, Goodenough DA, Li E, Paul DL Female infertility in mice lacking connexin 37. Nature 385, 525–529 (1997). 6616 https://doi.org/10.1038/385525a0
- Simon AM, Goodenough DA, Paul DL Mice lacking connexin40 have cardiac conduction abnormalities characteristic of atrioventricular block and bundle branch block. Curr. Biol 8, 295–298 (1998). 5 https://doi.org/10.1016/S0960-9822(98)70113-7
- Kirchhoff S, Nelles E, Hagendorff A, Krϋger O, Traub O, Willecke K. Reduced cardiac conduction velocity and predisposition to arrhythmias in connexin40-deficient mice. Curr. Biol 8, 299–302 (1998). 5 https://doi.org/10.1016/S0960-9822(98)70114-9
- Sanchez G Enhancement of Heparin Effect by Probenecid. N Engl J Med 292, 48 (1975).
- Cunningham RF, Israili ZH, Dayton PG Clinical Pharmacokinetics of Probenecid. Clin. Pharmacokinet 6, 135–151 (1981). 2 https://doi.org/10.2165/00003088-198106020-00004
- Martin PEM, Wall C, Griffith TM Effects of connexin-mimetic peptides on gap junction functionality and connexin expression in cultured vascular cells. Br. J. Pharmacol 144, 617–627 (2005). 5 https://doi.org/10.1038/sj.bjp.0706102
- Pelegrin P, Surprenant A Pannexin-1 mediates large pore formation and interleukin-1β release by the ATP-gated P2X7 receptor. Embo J. 25, 5071–5082 (2006). 21 https://doi.org/10.1038/sj.emboj.7601378
- Bargiotas P, Krenz A, Monyer H, Schwaninger M Functional outcome of pannexin-deficient mice after cerebral ischemia. Channels 6, 453–456 (2012). 6 https://doi.org/10.4161/chan.22315
- Krenacs T, Rosendaal M Connexin43 gap junctions in normal, regenerating, and cultured mouse bone marrow and in human leukemias: their possible involvement in blood formation. Am. J. Pathol 152, 993–1004 (1998). 4
- Ciovacco WA, Goldberg CG, Taylor AF, Lemieux JM, Horowitz MC, Donahue HJ, Kacena MA.. The role of gap junctions in megakaryocyte-mediated osteoblast proliferation and differentiation. Bone 44, 80–86 (2009). 1 https://doi.org/10.1016/j.bone.2008.08.117
- Novielli-Kuntz NM, Jelen M, Barr K, DeLalio LJ, Feng Q, Isakson BE, Gros R, Laird DW. Ablation of both Cx40 and Panx1 results in similar cardiovascular phenotypes exhibited in Cx40 knockout mice. Biosci Rep. 39, (2019). 2 https://doi.org/10.1042/BSR20182350