Abstract
Exosomes carry large cargo of proteins, lipids, and nucleic acids, serving as versatile biomarkers for disease diagnosis and vehicles for drug delivery. However, up to date, no well recognized standard procedures for exosome storage were available for clinical application. This study aimed to determine the optimal storage conditions and the anticoagulants for plasma-derived exosome isolation. Fresh whole blood samples were collected from healthy participants and preserved in four different anticoagulants including sodium citrate (SC1/4), sodium citrate (SC1/9), lithium heparin (LH), or Ethylenediamine tetraacetic acid (EDTA), respectively. Exosomes were extracted from the plasma by differential ultracentrifugation and stored at three different temperatures, 4°C, −20°C or − 80°C for a duration ranging from one week to six months. All plasma samples for storage conditions comparison were pretreated with LH anticoagulant. Exosome features including morphological characteristics, pariticles size diameter, and surface protein profiles (TSG101, CD63, CD81, CD9, CALNEXIN) were assessed by transmission electron microscopy, Nanoparticle Tracking Analysis, and Western Blotting, respectively. Exosomes preserved in LH and SC1/4 group tended to remain intact microstructure with highly abundant protein biomarkers. Exosomes stored at 4°C for short time were prone to be more stable compared to thos at −80°C. Exosomes stored in plasma were superior in terms of ultrastructure, size diameter and surface protein expression to those stored in PBS. In conclusion, plasma-dervied exosome characteristics strictly depend on the anticoagulants and storage temperature and duration.
Plain Language Summary
What is the context?
Effective isolation of exosomes is a prerequisite for subsequent investigation into its involvemnt in disease development as well as potentialtherapeutic applications.
Anticoagulants, storage temperature and durations might change the microscopical structure, integrity and also the stability of plasma-derived exosomes. However, no internationally recognized standard of exosome storage procedure was available for clinical use.
What is new?
Our finding evaluated the effect of anticoagulants and storage on plasma exosome characteristics.
Exosomes isolated from plasma preserved with Li-heparin and sodium citrate (1/4) showed better physical properties and surface marker protein expression.
Isolated exosomes appeared more stable in a short time for 4°C compared to −80°C. Storage of exosomes in plasma showed better physical properties and surface marker protein expression than in PBS.
What is the impact?
Our findings inform the significance of standardizing procedure of exosome isolation and preservation.
Introduction
Exosomes are biologically active lipid-bilayer membrane vesicles with size ranging from 30 to 150 nm in diameter and ubiquitous in most of the body fluids including blood and urine.Citation1 Exosomes released from various types of alive cells could serve as transporters for a variety of cellular components including proteins, RNAs, DNAs and lipids. They shuttle between cells and play a pivotal role in intercellular communications.Citation2
Exosomes emerges as important roles in the pathophysiology of many diseases including cancer, autoimmune disorders, neurodegenerative diseases and atherosclerosis, which were reported recent decades.Citation3 Exosomes may carry biomarkers for early disease diagnosis and may function as therapeutic targets. For example, exosomes derived from stem cells have been implicated in therapeutic benefits as an alternative cellular therapy and tissue regeneration.Citation4 Attributable to the properties of the nano-size bilayer membrane vesicles of exosomes, the degradation of enveloped cargoes, good biocompatibility and high affinity with targeted cells, exosomes can function as a potential vehicle for drug delivery.Citation5
Effective isolation of exosomes is essential for minimal artifacts in exosome preparations, and a prerequisite for investigation into the role in disease development as well as therapeutic applications. Several conventional methods were developed to isolate exosomes including differential ultracentrifugation, density-gradient ultracentrifugation, size-based filtration, size-exclusion chromatography, polymer precipitation, immune affinity capture and microfluidics-based isolation techniques.Citation6–8 Among these approaches, differential ultracentrifugation is more frequently used for simple operation, high throughput, low protein contamination and limited chemical reagent residues.Citation9,Citation10 Large amounts of biomarkers were identified by the differential ultracentrifugation.Citation11
Blood, one kind of major body fluid, is a key source of extracellular vesicles (EV) with great potential in supporting clinical diagnosis.Citation12 Due to the presence of platelet-derived EVs, plasma is a physiologically rich medium for EVs.Citation13 Anticoagulants are routinely added to prevent clotting during blood collection such as calcium-chelating agents (EDTA, sodium citrate) and inhibition of thrombin enzymatic activity (Heparin).Citation13 EDTA or citrate accelerates the binding of extracellular vesicles with platelets and thus reduces the free extracellular vesicles in blood.Citation14 The quality and quantity of extracellular vesicles,Citation14–18 were also affected by pH conditions, phlebotomy technique, transportation conditions and freeze-thaw cycles. Storage temperature (4°C, −20°C, −80°C) could also change the phenotypes of exosomes.Citation14,Citation15,Citation19 However, no well recognized standards of storage procedure for exosome were available.
International Society for Extracellular Vesicles (ISEV) proposed the standard identification of isolated exosomes at three levels, Transmission Electron Microscopy (TEM) evaluation of exosome morphology, nanosight tracking analysis (NTA) measurement of exosome size and Western Blotting identification of surface protein markers of exosomes.Citation20 Previous studies indicated that anticoagulants differed in promoting the formation of artefactual microvesicles due to platelet activation. Julie Boisramé-Helms et al. reported some anticoagulants had potential efficacy for the features of exosoms.Citation21 The choice of anticoagulant is most important in exosomes isolation but it is still disputable to date. In addition, the stability of exosomes in storage is another important aspect for subsequent functional studies and potential applications. In this study, we investigated the effects of anticoagulants and storage conditions on plasma-derived exosome characteristics.
Materials and methods
Blood collection
Fresh whole blood samples were collected from healthy participants attending routine physical examinations aged between 25 and 70 years old in Shanxi Bethune Hospital, China with written informed consent from each participant. A closed blood collection system including the vacuum blood collection tubes (pH 7.1–7.35) and 22 G (07 × 25) of butterfly needle was used with no strangulation for blood collection. The blood was preserved in one of four anticoagulants, 1/4 sodium citrate (3.8% Na3 citrate), 1/9 sodium citrate (SC1/9, 25% Na3 citrate), lithium heparin (LH) or K2-EDTA. Meanwhile, LH was selected as the anticoagulant for subsequent reseaches on the storage conditions of exosomes. Immediate centrifugation at 4°C, 3000×g for 15 min was perfomed to obtain the plasma from blood, followed by differential centrifugation to isolate exosomes within four hours of collection without prior freezing procedures. To compare storage tempreture and duration, aliquots of pooled plasma were frozen at −80°C for 1 week, 1 month, 2 months, 4 months or 6 months before exosome isolation. The ethical approval was obtained from the Research Ethics Committee of Shanxi University (SXULL2021078).
Exosome isolation
Exosomes were extracted from plasma using the differential ultracentrifugation.Citation22 In brief, 4°C, 300×g for 10 min centrifugation was conducted to remove the dead cells, followed by 2000×g for 10 min to remove the cell debris, 10 000×g for 30 min twice and 20 000×g for 60 min to remove large protein aggregates, and finally 100 000×g for 5 hours with 0.22 μm pore size filtering to precipitate the exosomes. The pelleted exosomes were resuspended in 200 μl of phosphate-buffered saline (PBS), aliquoted, and then stored at 4°C, −20°C or −80°C for 1 week, 1 month, 2 months or 6 months, respectively, with the fresh sample as a control.
Transmission Electron Microscopy (TEM) observation
The aliquoted exosomes were diluted (1:10) in PBS, settled on a copper mesh for 5 min, and then washed with ddH2O for three times. The liquid residual was removed with Whatman’s filter paper. Then 3% phosphotungstic acid solution was added in drops onto the copper mesh and soaked for 5 min as negative staining. After air dry, samples were visualized under TEM (Carl Zeiss Microscopy GmbH, Germany).
Nanoparticle tracking analysis for particle size and concentrations
The aliquoted exosomes were diluted in PBS to appropriate concentrations between 1 × 10Citation7 and 1 × 10Citation9 particles/ml. Sample was injected into the sample chamber at a constant flow rate using 1 ml syringe, detected by the ZetaView® PMX 120 (Particle Metrix,Germany) and recorded by the software (ZetaView 8.04.02). Triple tests were conducted for each sample.
Digestion of proteins in exosomes and western blot (WB)
Exosomes were dissolved in the protein lysis buffer (Solarbio, China) and heated at 95°C for 10 min. Bicinchoninic Acid Assay (BCA) Kit (Solarbio, China) was used to determine the concentration of total proteins of exosomes. Amounts of 10 μg total protein were loaded into 10% sodium dodecyl sulfate polyacrylamide gel (SDS-PAGE),transferred to PVDF membrane (Millipore, America) and blocked with 5%BSA. The membrane was then incubated with primary antibodie, anti-CD9, anti-CD63, anti-CD81, anti-TSG101 or anti-Calnexin (Abcam, Cambridge, UK) overnight at 4°C and secondary compatible antibody anti-rabbit IgG (ABclonal, China) at room temperature for 1 hour. After washing, protein bands were visualized using Chemiluminescence (Engreen, China) and the Gel documentation system (Syngene, UK).
Statistical analysis
The data were plotted and analyzed using GraphPad Prism 5.0 Software (San Diego, CA). All experiments were performed in triplicate and data are presented as mean ± standard deviation (SD). Student’s t test was used to compare the means between two groups while one-way ANOVA test was used for the means among multiple groups.
Results
To explore the optimal anticoagulant and storage conditions to isolate exosomes, we evaluated the structural integrity, stability and protein contents of exosomes that were either isolated from fresh plasma pretreated with anticoagulants, or from -80°C frozen plasma and then stored at varied tempretures and durations or from fresh plasma with direct cryopreservation at varied tempretures and durations. The schematic flowchart for isolation of exosomes by differential ultracentrifugation was illustrated in .
Figure 1. Differential ultracentrifugation schemes for isolating exosomes from fresh or frozen plasma with series of measurement by TEM, NTA and WB. *T0 samples were measured for exosomal characteristics immediately after isolation without storage.
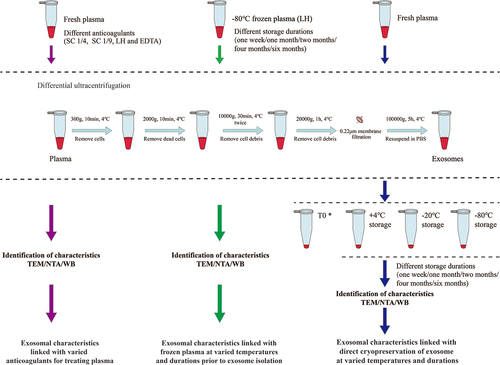
Effect of anticoagulant on microscopic characterization of exosomes by TEM
We found the majority of isolated vesicles appeared to be round to oval-like or cup-shaped with bilayer outer membrane, low electron density with varied sizes . Most vesicles were dispersed felling into the size range of exosomes at 30-150nm but some aggregated. Vesicle particles of EDTA group and the SC1/9 group were uneven in size distribution with some larger particles than 150nm. The particle sizes of EDTA group vesicles were concentrated between 30-150 nm, with obvious vesicle aggregation. Most of vesicle of SC1/9 group was close to 150 nm. The size distribution of vesicles in LH group and SC1/4 group was more uniform with the vesicle diameter of the LH group about 50-100 nm and the SC1/4 group about 100 nm ().
Figure 2. Characteristics of plasma-derived exosomes reserved with varied anticoagulants. (a) Morphological features was observed by TEM. (b) The concentration of exosome particles was analyzed by NTA. (c) Size distribution of exosomes was detected by NTA. (d) Particle peak diameter of exosomes was determined by NTA. (e) Protein content of Exosome positve markers (TSG101, CD63 and CD9) and negative marker (Calnexin) were measured by WB. One-way ANOVA test was used for the means comparison among multiple groups followed by LSD for comparison between two groups. Data were expressed as mean ± SD (n = 3 per group). *p <0 .05, **p <0 .01.
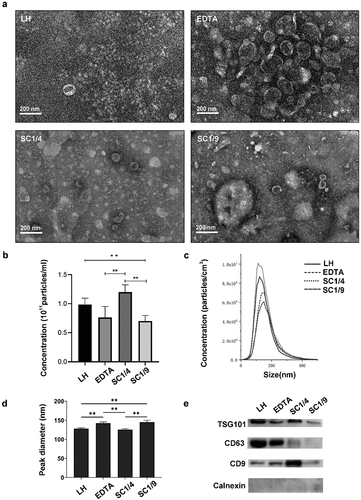
Effect of anticoagulant on concentrations, size distribution and stability of exosome samples
Particle of exosomes extracted from plasma pretreated with varied anticoagulants was concentrated in a size range of 30-150 nm, with EDTA and SC1/9 groups at the oversize range (, ). Significant differences among four groups in particle size and concentration were observed. The vesicle concentrations in a descending order obtained by four methods were SC1/4 > LH > EDTA and SC1/9 (). There is no significant difference of concentration between EDTA and SC1/9 groups ().
Table 1. Particles concentrations and peak diameter of exosomes derived from plasma pretreated with anticoagulants
Effect of anticoagulant on quantitative and qualitative analysis of proteins in exosomes
The isolated exosomes had detectable protein levels for TSG101, CD63 and CD9 by WB analysis. The signal of Calnexin, serving as a negative control, was very weak, suggesting minimal contamination from non-exosomal sources. Comparing different anticoagulant groups, LH or SC1/4 samples showed smiliar amount of TSG101 protein, higher than that in EDTA. Regarding CD63 protein, it was more easily enriched in exsomes from LH, follwed by EDTA, then SC1/4 and SC1/9. CD9 signal in SC1/4 was significantly higher than that in LH or EDTA. The amount of TSG101, CD63 and CD9 were lowest in SC1/9 ().
Effect of storage temperatures and durations on exosome ultrastructure
The exosome ultrastructure was observed by TEM to evaluate the structural integrity under different storage conditions. As shown in , exosomes after direct cryopreservation or from cryopreservation of plasma showed round to overall shaped vesicles with varied sizes, each bilayer’s outer membranes with light staining and low electron density substance with deep staining. The membrane was white, less clear but transparent, while the interior content was dark. These vesicles were mostly distributed between 30–100 nm in size and were either dispersed or aggregated.
Figure 3. Transmission electron microscopic examination of exosomes isolated from plasma at different storage conditions. (a) Fresh exosomes without storage. (b–d) One-week stored exosomes with direct cryopreservationat 4°C, −20°C and −80°C, respectively. (e) One-week stored exosomes isolated from −80°C frozen plasma. (f–h) One-month stored exosomes with direct cryopreservation at 4°C, −20°C and −80°C, respectively. (i) One-month stored exosomes isolated from frozen −80°C plasma. (j–l) Two-month stored exosomes with direct cryopreservation at 4°C, −20°C and −80°C, respectively. (m) Two-month stored exosomes isolated from −80°C plasma. (n–p) Six-month stored exosomes with direct cryopreservation at 4°C, −20°C and −80°C, respectively. (q) Six-month stored exosomes isolated from −80°C frozen plasma. Direct mag: 40 000 ×, HV = 200.0 kV.
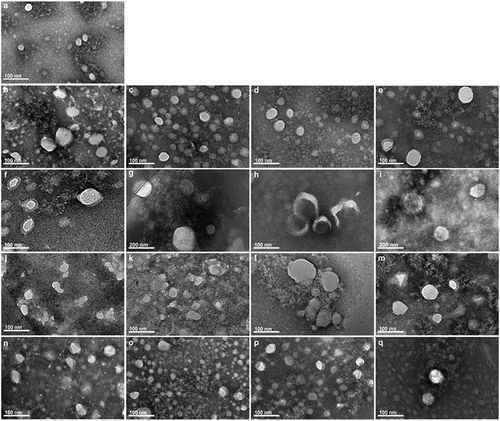
Fresh exosomes without storage looked round and plump (). In the case of storage duration of one week, exosomes stored at 4°C were larger and plumper compared to other storage temperatures (). This indicatd thawing for one week may be a risk factor for the structural integrity of exosomes. In the case of long-term storage, 1 month, 2 months or 6 months, exosomes stored at − 80°C tended to be more stable and much plumper rounded with less or no morphological wrinkles and deformationsthan those stored at − 80°C or 4°C (). Vesicles stored at 4°C or −20°C for extended durations showed a large amount of amorphous material, deformation and shrink. These findings suggested that plasma samples should be stored at −80°C to maintain better exosome ultrastructure. Nevertheless, the shorter the storage duration, the better the exosome structural integrity. The exosomes stored less than a month were larger and plumper. Macroscopically, exosomes stored at 4°C for four months underwent a metamorphosis with a black flocculent solution (Supplementary Figure S1). Storage at 4°C for less than a week appeared to be acceptable.
The effect of storage temperatures and durations on exosome concentration and size
To determine the effect of storage temperature on exosome size, whole exosomes were isolated from the plasma, diluted in PBS and subjected to NTA analysis (, ). Samples prepared for NTA were placed on ice before analysis and applied to the sample chamber of NTA. The size distribution of the particles and medium concentration were shown in , felling into the diameter range for exosomes. For the one week group, the sample with the highest exosome concentration was from the exosomes stored at 4°C. For the one-month group, the highest exosome concentration and stability were from samples stored at −80°C. However, as for the 4-month-group and 6-month-group, exosomes from plasma stored at −80°C were prone to be more intact in microscopical structure and stable in size distribution. For long-term storage, 4°C and −20°C led to the most fragile exosomes ().
Figure 4. NTA analysis for particle size and concentrations under different storage temperatures and durations. (a – d) Concentrations and sizes distribution of the plasma exosomes after storage for one week, one month, four months and six months, respectively. The y-axis represents concentrations of particles per ml and the x-axis shows their sizes. (e) Concentration of particles in exosomes under different storage temperatures and durations by NTA. (f, g) Particle peak diameter of exosomes under different storage temperatures and durations by NTA. Data were analyzed by one-way ANOVA test for multiple groups comparison followed by LSD for further intergroup. Data were expressed as mean ± SD (n = 3 per group). T0 stands for exosomes from fresh plasma.
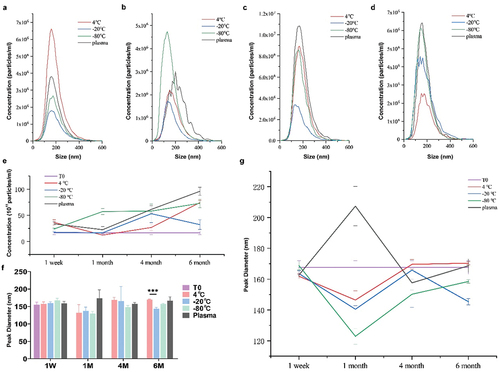
Table 2. Concentrations and diameter of plasma-derived exosomes.
The asymmetric size of freshly prepared exosomes ranged from 50 nm to 170 nm with an average size of 154.67 nm (). As expected, the peak diameter of exosomes stored at 4°C for one week was most approximate to the freshly prepared exosomes. No statistical differences of peak diameter were observed between the studied storage conditions (p > 0.05). While the peak diameter of exosomes stored at 4°C and −20°C fluctuated with time, exosomes from plasma stored at −80°C were relatively stable as shown in . Both short-term storage and long-term storage at 4°C did not have a significant impact on size distribution ().
The effect of storage temperatures and durations on exosome protein
Protein biomarkers in isolated exosomes were evaluated by WB analysis. In all most samples, TSG101, CD63 and CD81 as positve biomarkers of exosomes were found highly expressed, while the signal of Calnexin, as the negative control, was weak. Regardless of storage temperatures, the protein markers amount from exosomes stored for one week showed similar pattern, albeit the contents of exosomes stored at 4°C were abundant. When exosomes were cryopreserved for one month and two months, the lower the temperature, the higher the protein amount of exosomes. The contents of exosomes, and levels of TSG101 decreased sharply after two months of storage at 4°C and the difference becomes significant after four or six months of storage. Among them, direct freezing of plasma at −80°C was most effective in maintaining the protein content. After exosomes stored for four and six months, protien biomarkers stored at 4°C failed to display in WB analysis (). .Exosomes from −80°C frozen plasma was superior to those from other conditions in term of protein biomarkers contents.
Figure 5. Western blot analysis for protein biomarkers of exosomes stored under different temperatures and durations. Exosome surface protens included (TSG101, CD63, CD81) as positive biomarkers and Calnexin as negative markers. T0 stands for exosomes from fresh plasma samples. −80°a stands for exosomes with direct cryopreservation at −80°C; −80°b stands for exosomes isolated from frozen plasma but with no further frozen storage.

Discussion
Standardized procedure of exosome isolation is essential for clinical application and diagnosis. Exosomes have immense promising potentials in various medical fields, yet their clinical applications are largely limited.Citation23 To date, there is still a lack of standardized specifications for the isolation and storage of exosomes. Based on the ideal clinical plasma pre-treatment, storage conditions should have a minimal negative effect on vesicle integrity, contents and functions. To standardize the procedure for exosome preservation, we performed a comparative evaluation of exosomes storage conditions. In our study exosomes prepared by differential ultracentrifugation met the international definitions of exosome by size and morphology.Citation24 We found that micro-structures by TEM and immune-biomarkers including three positive biomarkers by WB of exosomes in Li-heparin and SC1/4 group remained intact with high abundance. Storage temperatures affected the stability of exosomes and the content of protein.
Another finding of our study is that storage conditions also affect exosomes protein content and morphological characteristics. Several studies indicated that storage conditions significantly alter both the physical and functional properties of extracellular vesicles.Citation25 A study by Maroto et al. indicated that compared with fresh samples, exosomes stored at 4°C and −80°C increased size in diameter, resulting in structural changes of exosomes.Citation15 Our results showed that exosomes stored at 4°C or −20°C for short-term preservation did not significantly change compared with exosomes stored at −80°C, and −80°C would be preferable for long-term preservation of exosomes. This trend is similar to the findings of Wu et al.Citation17 In our study, protein analysis of plasma-derived exosomes showed that storage at 4°C induces biomarker changes reflected by alteration of CD81, TSG101and CD63. Exosomes stored at 4°Chad little effect on their concentration and protein levels of exosomes for short intervals. However, for long-term storage, metamorphism of exosomes were observed. The essence of this metamorphosis phenomenon might be due to exosome membrane rupture or membrane degradation and subsequent release of the contents when exosomes are stored at 4°C for a long time. Thereafter, the remnants of the membrane components might undergo fusion and aggregation, which could explain the presence of brownish, flocculent aggregates seen in the samples. The contents of exosomes, and levels of TSG101 decreased sharply after two months of storage at 4°C and the difference becomes significant after four months of storage. The exosomes stored at −20°C and −80°C also showed varying degrees of degradation. The exosomes degradation peaked after six months. An increasing concentration of exosome particles under low temperatures found in the NTA results reflected the possible disaggregation of large exosomes lump or possible disintegration of large exosomes.Citation17 The effect of plasma cryopreservation at different time points was better than that of direct cryopreservation of exosomes. This was mainly reflected in the specific marker protein.
In the study, we used a combination of methods including ultrastructure, nanoparticle tracking analysis and positive protein marker analysis to evaluate the quality and quantity of preserved exosome preparations. From the combined analysis, we found that plasma anticoagulants of sodium citrate (SC1/4) and Li-heparin given better outcomes. Considering the storage temperatures, there are limitations in the current study. Firstly, pooled plasma samples with cohorts of healthy participants were used in our study to evaluate the associaton of storge conditions with exosomal characteristics, which led to selection bias due to the inability to stratify the impact of individual sociodemographical characteristics on the association. Secondly, in the 0.22μm filtration process, the different resistence from exosomal particles with varied size through filtration membrane may affect the final protein concentration and protein content to some extent.
In conclusion, our study confirmed the importance of anticoagulants and storage temperatures and durations for structure integrity and protein stability of exosomes and found that the SC1/4 and LH as anticoagulants may provide better protection for plasma-derived exosomes from degradation. Exosomes stored at 4°C is acceptable for short-term storage but −80°C frozen plasma with subsequent isolation is recommended for long-term storage. These findings will greatly inform the standardized protocols for isolation and storage of exosomes in the future.
Author contributions
Caiting Yang and Jie Han contributed equally to this manuscript. Li D, Caiting Yang conceived and designed the experiments. Caiting Yang, Jie Han, Hai Liu, Yuyu He, Zhenhua Zhang, Lizhong Zhang, Huiping Duan and Xiaochun Liu collected the experimental specimens and clinical data. Caiting Yang, Jie Han, Hai Liu and Jing He performed the experiments. Caiting Yang, Jie Han, Hai Liu and Li Dong performed the data analysis. Caiting Yang, Jie Han, Farooq Waqas and Li Dong participated in manuscript writing. All people participated in the results interpretation and the final manuscript.
Availability of data and materials
All relevant data are available within the manuscript and Supporting Informations.
Ethical approval
Research involving human subjects complied with all relevant national regulations, institutional policies and is in accordance with the tenets of the Helsinki Declaration (revised in 2013). The written informed consent was obtained from all participants involved in this study and ethical approval was obtained from the Research Ethics Committee of Shanxi University (SXULL2021078).
Informed consent
Informed consent was obtained from all individuals authored in this study.
Acknowledgments
We thank all the participants in this study for their important support. We are very grateful to Jialiang Zhou and Daijun Li for their help in blood collection.
Disclosure statement
No potential conflict of interest was reported by the author(s).
References
- Thery C, Zitvogel L, Amigorena S. Exosomes: composition, biogenesis and function. Nat Rev Immunol. 2002;2(8):569–9. doi:10.1038/nri855.
- van Niel G, D’Angelo G, Raposo G. Shedding light on the cell biology of extracellular vesicles. Nat Rev Mol Cell Biol. 2018;19(4):213–28. doi:10.1038/nrm.2017.125.
- Kalluri R, LeBleu VS. The biology, function, and biomedical applications of exosomes. Science. 2020;367(6478):6478. doi:10.1126/science.aau6977.
- Phinney DG, Pittenger MF. Concise review: MSC-Derived exosomes for cell-free therapy. Stem Cells (Dayton, Ohio). 2017;35(4):851–8. doi:10.1002/stem.2575.
- Liang Y, Duan L, Lu J, Xia J. Engineering exosomes for targeted drug delivery. Theranostics. 2021;11(7):3183–95. doi:10.7150/thno.52570.
- Li P, Kaslan M, Lee SH, Yao J, Gao Z. Progress in Exosome Isolation Techniques. Theranostics. 2017;7(3):789–804. doi:10.7150/thno.18133.
- Zhang Y, Bi J, Huang J, Tang Y, Du S, Li P. Exosome: a review of its classification, isolation techniques, storage, diagnostic and targeted therapy applications. IJN. 2020;15:6917–34. doi:10.2147/IJN.S264498.
- Zhu L, Qu XH, Sun YL, Qian YM, Zhao XH, et al. Novel method for extracting exosomes of hepatocellular carcinoma cells. World J Gastroenterol. 2014;20(21):6651–7. doi:10.3748/wjg.v20.i21.6651.
- Xie XD, Nie HF, Zhou Y, Lian S, Mei H, Lu Y, Dong H, Li F, Li T, Li B. et al. Eliminating blood oncogenic exosomes into the small intestine with aptamer-functionalized nanoparticles. Nat Commun. 2019;10(1):10. doi:10.1038/s41467-019-13316-w.
- Kumar A, Deep G. Exosomes in hypoxia-induced remodeling of the tumor microenvironment. Cancer Lett. 2020;488:1–8. doi:10.1016/j.canlet.2020.05.018.
- Cao F, Gao Y, Chu Q, Wu Q, Zhao L, Lan T, Zhao L. Proteomics comparison of exosomes from serum and plasma between ultracentrifugation and polymer-based precipitation kit methods. Electrophoresis. 2019;40(23–24):3092–8. doi:10.1002/elps.201900295.
- Zhou B, Xu K, Zheng X, Chen T, Wang J, Song Y, Shao Y, Zheng S. Application of exosomes as liquid biopsy in clinical diagnosis. Signal Transduct Target Ther. 2020;5(1):144. doi:10.1038/s41392-020-00258-9.
- Witwer KW, Buzás EI, Bemis LT, Bora A, Lässer C, Lötvall J, Nolte‐‘t Hoen EN, Piper MG, Sivaraman S, Skog J. et al. Standardization of sample collection, isolation and analysis methods in extracellular vesicle research. J Extracell Vesicles. 2013;2(1):2. doi:10.3402/jev.v2i0.20360.
- Baek R, Sondergaard EK, Varming K, Jørgensen MM. The impact of various preanalytical treatments on the phenotype of small extracellular vesicles in blood analyzed by protein microarray. J Immunol Methods. 2016;438:11–20. doi:10.1016/j.jim.2016.08.007.
- Maroto R, Zhao Y, Jamaluddin M, Popov VL, Wang H, Kalubowilage M, Zhang Y, Luisi J, Sun H, Culbertson CT. et al. Effects of storage temperature on airway exosome integrity for diagnostic and functional analyses. J Extracell Vesicles. 2017;6(1):1359478. doi:10.1080/20013078.2017.1359478.
- Jamaly S, Ramberg C, Olsen R, Latysheva N, Webster P, Sovershaev T, Brækkan SK, Hansen J-B. Impact of preanalytical conditions on plasma concentration and size distribution of extracellular vesicles using nanoparticle tracking analysis. Sci Rep. 2018;8(1):17216. doi:10.1038/s41598-018-35401-8.
- Wu JY, Li YJ, Hu XB, Huang S, Xiang D-X. Preservation of small extracellular vesicles for functional analysis and therapeutic applications: a comparative evaluation of storage conditions. Drug Deliv. 2021;28(1):162–70. doi:10.1080/10717544.2020.1869866.
- Cheng Y, Zeng Q, Han Q, Xia W. Effect of pH, temperature and freezing-thawing on quantity changes and cellular uptake of exosomes. Protein Cell. 2019;10(4):295–9. doi:10.1007/s13238-018-0529-4.
- Wisgrill L, Lamm C, Hartmann J, Preißing F, Dragosits K, Bee A, Hell L, Thaler J, Ay C, Pabinger I. et al. Peripheral blood microvesicles secretion is influenced by storage time, temperature, and anticoagulants. Cytometry Pt A. 2016;89(7):663–72. doi:10.1002/cyto.a.22892.
- Théry C, Witwer KW, Aikawa E, Alcaraz MJ, Anderson JD, Andriantsitohaina R, Antoniou A, Arab T, Archer F, Atkin‐Smith GK. et al. Minimal information for studies of extracellular vesicles 2018 (MISEV2018): a position statement of the International society for extracellular vesicles and update of the MISEV2014 guidelines. J Extracell Vesicles. 2018;7(1). doi:10.1080/20013078.2018.1535750.
- Boisramé-Helms J, Delabranche X, Degirmenci SE, Zobairi F, Berger A, Meyer G, Burban M, Mostefai HA, Levy B, Toti, F. et al. Pharmacological modulation of procoagulant microparticles improves haemodynamic dysfunction during septic shock in rats. Thromb Haemost. 2014;111(1):154–164. doi:10.1160/TH13-04-0313.
- Kimiz-Gebologlu I, Oncel SS. Exosomes: large-scale production, isolation, drug loading efficiency, and biodistribution and uptake. J Control Release. 2022;347:533–43. doi:10.1016/j.jconrel.2022.05.027.
- Gurunathan S, Kang MH, Jeyaraj M, Qasim M, Kim J-H. Review of the isolation, characterization, biological function, and multifarious therapeutic approaches of exosomes. Cells. 2019;8(4):307. doi:10.3390/cells8040307.
- Lotvall J, Hill AF, Hochberg F, Buzás EI, Di Vizio D, Gardiner C, Gho YS, Kurochkin IV, Mathivanan S, Quesenberry P. et al. Minimal experimental requirements for definition of extracellular vesicles and their functions: a position statement from the International society for extracellular vesicles. J Extracell Vesicles. 2014;3(1):26913. doi:10.3402/jev.v3.26913.
- Lorincz AM, Timar CI, Marosvari KA, Veres DS, Otrokocsi L, Kittel Á, Ligeti E. Effect of storage on physical and functional properties of extracellular vesicles derived from neutrophilic granulocytes. J Extracell Vesicles. 2014;3(1):25465. doi:10.3402/jev.v3.25465.