Abstract
Onychomycosis is difficult to treat due to long treatment durations, poor efficacy rates of treatments, high relapse rates, and safety issues when using systemic antifungal agents. Device-based treatments are targeted to specific regions of the nail, have favorable safely profiles, and do not interfere with systemic agents. They may be an effective alternative therapy for onychomycosis especially with increasing reports of squalene epoxidase gene mutations and potential resistance to terbinafine therapy. In this review, we discuss four devices used as antifungal treatments and three devices used as penetration enhancers for topical agents. Lasers, photodynamic therapy, microwaves, and non-thermal plasma have the capacity to inactivate fungal pathogens demonstrated through in vivo studies. Efficacy rates for these devices, however, remain relatively low pointing toward the need to further optimize device or usage parameters. Ultrasound, nail drilling, and iontophoresis aid in improving the permeability of topical agents through the nail and have been investigated as adjunctive therapies. Due to the paucity in clinical data, their efficacy in treating onychomycosis has not yet been established. While the results of clinical studies point toward the potential utility of devices for onychomycosis, further large-scale randomized clinical trials following regulatory guidelines are required to confirm current results.
Introduction
Onychomycosis is a hard-to-treat fungal infection of the nail unit; high complete cure and mycological cure rates remain elusive. Many oral and topical antifungal agents are approved by regulatory bodies around the world for the treatment of onychomycosis including terbinafine, itraconazole, 10% efinaconazole, 5% tavaborole, and 8% ciclopirox (Citation1,Citation2). These agents have varying rates of success in clinical studies, with limitations such as long treatment durations, drug interactions, low patient compliance, and high relapse rates (Citation3–5). The emergence of nondermatophyte mold (NDM) infections and mixed infections in patients with onychomycosis are on the rise and require updated treatment approaches (Citation6–8). Additionally, reports of dermatophyte resistance to antifungal drugs are on the rise worldwide and are expected to present clinical challenges in the future. Medical devices, in theory, can combat some of the challenges to traditional drug therapy posed by onychomycosis, and we review the utility of existing devices for toenail onychomycosis.
Device use reported in onychomycosis studies represent two basic classes: direct antifungal treatment, and topical nail penetration enhancement. Penetration enhancement is particularly useful where concern for drug delivery may exist; however, penetration enhancement itself does not provide antifungal action but only augments actions of topical antifungal medications. The mechanism of action of devices that provide direct antifungal effects vary widely and include photothermal effects, phototoxic, photodynamic activation, selective photothermolysis, and production of reactive oxygen species. Device-based antifungal treatments present many advantages for onychomycosis over standard pharmaceutical treatments. Devices have not been associated with systemic adverse effects, hepatotoxicity, and drug interactions associated with oral antifungal agents (Citation9,Citation10). In-office devices do not rely on patient compliance for efficacy (Citation3,Citation11). Unlike pharmaceuticals, the nonspecific mechanisms of action of devices can target NDMs and mixed infections in addition to dermatophyte onychomycosis, and may also remain effective for drug-resistant fungi causing onychomycosis (Citation3,Citation6,Citation8). A summary of the advantages and disadvantages of devices compared to oral and topical antifungals have been provided in .
Table 1. Advantages and disadvantages of standard oral and topical antifungal treatments versus device-based treatments.
This paper reviews the currently available clinical evidence for device efficacy in onychomycosis. Few of the devices in use have been evaluated for onychomycosis by national regulatory agencies to date, and as such, off-label use is the rule rather than the exception. The advantages of devices make such use compelling but reduces the comparability of treatment with that of pharmaceuticals; pharmaceutical approval by the FDA is based on proven antifungal activity in randomized controlled trials, while device approval is typically for the ‘temporary increase in clear nail’ in trials without requiring complete clinical cure and mycological efficacy to be established (Citation12). More treatment options for onychomycosis are needed; this review discusses the device options being evaluated for clinical use. These devices may increase the therapeutic options available for onychomycosis, but additional investigations are required to prove their competitive value versus established pharmaceutical options. Novel delivery systems not yet tested in clinical settings are beyond the scope of this review and have been discussed previously (Citation13).
Methods
A systematic search was performed following the Preferred Reporting Items for Systematic Reviews and Meta-Analyses guidelines to obtain all relevant articles discussing the results of clinical studies using devices for the treatment of onychomycosis (Citation14). The literature search was performed using PubMed, Scopus, Ovid, and Web of Science with the key words ‘onychomycosis’ and ‘device’. We aimed to identify all device-based treatments to date for onychomycosis and the highest level of evidence. The results were uploaded to Rayyan (https://www.rayyan.ai/) for deduplication and screening of titles and abstracts. Articles were excluded if they were reviews or commentaries, did not contain human data, were not treating onychomycosis, did not use devices, or if they were not available in English. The data for discussion focused preferably on higher quality reports where available. For devices with no randomized controlled trials (RCTs), all clinical studies were included. For devices with RCTs, analyses were focused primarily on studies with toenail onychomycosis, population over 18 years of age, onychomycosis confirmation using potassium hydroxide (KOH)/Periodic-Acid Schiff (PAS) and fungal culture at baseline, and defined clinical and mycological cures at endpoint. Full texts of articles included after title and abstract screening were retrieved and assessed for eligibility. The reference sections of prominent review articles were screened for eligible studies.
Results
Of the 2,079 articles obtained from the search, 68 articles were included (). Of the 68 articles, only 18 were RCTs with high quality data for comparison. A total of 4 devices for antifungal treatment (lasers, photodynamic therapy, microwaves, and non-thermal plasma) and 3 penetration-enhancing devices (iontophoresis, nail drilling, and ultrasound) were identified. An overview of the mechanism of action of these devices is elucidated in . In the following sub-sections, we review the background, mechanism of action, and clinical evidence for use of each of the identified devices.
Figure 2. Summary of device mechanisms for the treatment of onychomycosis. Starting from left: Lasers) heat the fungal infection under the nail through transmission as well as generation of reactive oxygen species causing fungal cell damage; photodynamic therapy (PDT)) involves excitation of a photosensitizer by a light source leading to the generation of reactive oxygen species; microdrilling) small holes through the nail to aid the topical antifungal agent to reach the nail bed; microwaves) increase the temperature of the fungus, denaturing its proteins, they also cause DNA damage, and trigger an immune response; ultrasound) uses acoustic streaming to push the active drug through the layers of the nail; iontophoresis) also drives drugs in a topical antifungals through the nail by first applying a charge to the drugs and then introducing an electric field across the affected nail to pull them; non-thermal plasma) in contact with the air generates both reactive oxygen and reactive nitrogen species to damage the fungus.
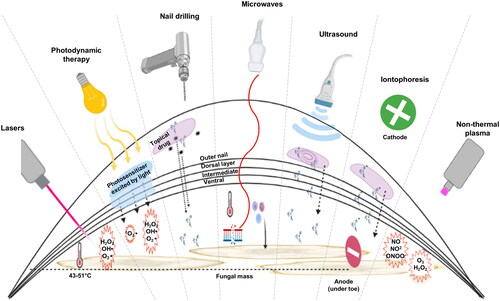
Antifungal treatment
Lasers
Background and mechanism
Lasers were first developed in the 1960s, and have since then been explored and used for several dermatological applications including the treatment of pigmented and vascular lesions, and tattoo removal (Citation15). Over the years, their utility has extended to the treatment of onychomycosis (Citation16,Citation17). Lasers, the acronym for Light Amplification by Stimulated Emission of Radiation, work by the continued release of photons from a gain medium. The gain medium, when excited using electricity or light, is the source and amplifier of the photons that form the laser beam (Citation15). Lasers are named after the gain medium used. The most commonly used lasers for onychomycosis are Neodymium-doped Yttrium Aluminum Garnet (Nd: YAG), which use a solid-state gain medium, and the Carbon Dioxide (CO2), which use a gaseous gain medium (Citation15,Citation18). Several lasers have been approved by the United States Food and Drug Administration (US FDA) and regulatory bodies of other countries such as Canada, Australia, and Japan for several cosmetic applications; some lasers, such as PinPointe™ FootLaser®, have been FDA cleared for ‘temporary increase in clear nail’ in onychomycosis patients (cosmetic approval); however, some practitioners may use them off-label to treat onychomycosis in the hopes of achieving mycologic and/or clinical cure (Citation4).
The mechanism of action of lasers in the elimination of fungal pathogens is primarily based on the photothermal effect, where the fungal cells absorb light energy from the incident laser beam and convert it into heat () (Citation17). This heat results in the denaturation of proteins and the inactivation of fungal cells (Citation19). A key factor when calibrating the laser parameters is to consider the thermal properties of the surrounding tissue, as it is equally important to ensure that the healthy human cells are not damaged (targeting of fungal cells versus anti-targeting of surrounding dermal tissue) (Citation19,Citation20). As fungal cells have a lower heat capacity compared to the surrounding human dermal cells, they heat up more quickly and are more likely to retain the heat, which is favorable for fungal inactivation while protecting the dermal cells. Several parameters of the laser system can affect the outcome of treatment; wavelength, pulse format, spatial format, and fluence. Wavelengths of 750–1300 nm enable optimal nail plate penetration. Temporal pulses promote the incremental increase in the temperature of the fungal organism leading to its death while allowing the heat to dissipate from the surrounding tissue and maintaining its temperature below the pain threshold of 45 °C (Citation17).
Figure 3. Mechanism of lasers and photodynamic therapy (PDT). (A) laser pulses have a photothermal effect on the fungal cells while sparing the surrounding dermis and tissue. (B) A topical photosensitizer is applied to a softened nail. A light source of specified wavelength excites the photosensitizer to a singlet state, to an excited singlet state, to an excited triplet state. In a type I photoreaction, the photosensitizer then interacts with biomolecules to produce unstable free radicals. Likewise, in a type II photoreaction the photosensitizer reacts with oxygen to produce singlet oxygen, which like free radicals, is cytotoxic to the fungus.
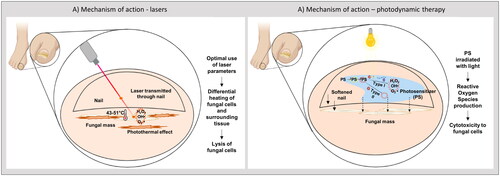
Thermal relaxation time (TRT), the time required for cells to dissipate heat, is an important parameter that determines the width of the laser pulse and time between recurring laser pulses (Citation17,Citation21). The TRT of fungal cells is longer than that of dermal cells (Citation17). For a laser to be successful, the pulse duration should not exceed the TRT of fungal cells, so that there is a temperature build-up in the target. The intervals between the pulses should be such that the dermal cells with a shorter TRT have time to cool down while the fungal organisms with a longer TRT retain some of the heat leading to incremental temperature increase over the treatment duration (Citation17). The spatial format, determined by the spot size and beam shape, and the power fluence, which is the amount of energy delivered per unit area, have an effect on the depth of penetration of the laser beam through the nail plate and its ability to target fungal cells while preventing damage to underlying tissue (Citation17). As these parameters are specific to the laser systems used and the fungal pathogens involved, their optimization is necessary to achieve a therapeutic effect and to avoid adverse reactions (Citation17).
Other phenomena such as photochemical and photomechanical effects can also play a role, but are not considered the primary mode of fungal death in Nd: YAG and CO2 lasers (Citation17). A non-thermal laser system (Lunula laserTM, Erchonia Corporation, McKinney, Texas, USA) has been FDA cleared for the clearance of toenail onychomycosis, and works on the principle of photochemical reactions (Citation22). Working at dual wavelengths of 405 nm and 635 nm, this laser does not generate heat; rather, it alters the metabolic activity of fungal and host cells leading to fungal cell death and increased immune responses by the surrounding human cells (Citation22). The mechanism of action of the laser and depth of penetration is determined by the wavelength of laser used, in addition to other laser parameters (Citation17). The device has FDA clearance; however, there are no reported randomized controlled trials on this laser system. Mycological cures and complete clinical cure rates have not been reported.
Several in vitro studies evaluated the preliminary efficacy of lasers for onychomycosis. In studies by Paasch et al. and Carney et al. Nd: YAG 1064 nm laser held at a minimum of 50 °C inhibited fungal growth; the extent of inhibition varied depending on the fungal pathogen tested (Citation23,Citation24). Despite its therapeutic potential, optimization of laser parameters is necessary to achieve a fungicidal effect.
Clinical use
A total of 53 reports on lasers, involving 2147 patients, were identified during the literature search process (). This included 22 prospective studies, 18 RCTs, 7 retrospective studies, 1 cohort study, and 5 case reports. In vivo clinical trials reported include four different lasers to date; Nd: YAG, fractional CO2, erbium-doped yttrium aluminum garnet (Er: YAG), and diode lasers. The Nd: YAG laser and the CO2 laser were most commonly reported with mixed results.
Figure 4. Summary of reports on clinical trials on devices from systematic search. From the 68 clinical studies obtained from the search, 53 reports on lasers, 9 reports on PDT, 2 reports on nail drilling, 2 reports on iontophoresis, and 2 reports on non-thermal plasma were identified. Quality of evidence in terms of studies reporting on the efficacy of monotherapy (lasers), RCTs, and complete mycological cure (negative culture and negative microscopy/histology) are depicted for (A) lasers and (B) other devices.
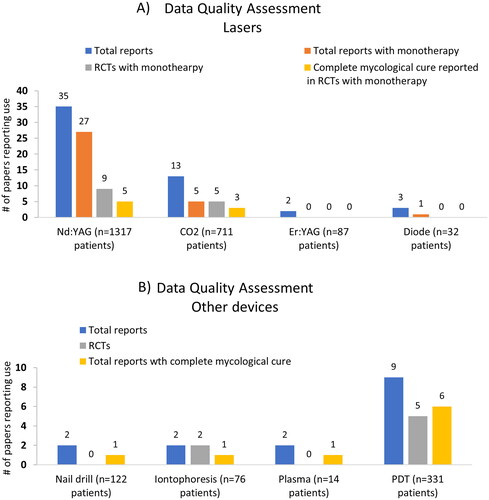
Of the 53 reports, only 4 randomized controlled trials (RCTs) using Nd: YAG 1064 nm lasers followed FDA recommendations or guidelines by other regulatory bodies for clinical trials on medical devices for onychomycosis () and reported fingernail and toenail outcomes separately (Citation3,Citation12). Laser treatment parameters varied in each of the four RCTs, with 3–4 laser sessions administered once every 2–12 weeks and clinical outcome variables were not consistent between these trials (Citation25–28). None of the patients in three of the four studies (n = 134 patients) achieved complete cure or significant improvement in the onychomycosis severity index (OSI) score, and concluded that Nd: YAG lasers alone were not effective in treating onychomycosis (Citation26–28). Of these 3 studies, only 2 studies reviewed mycological cure rates using the standard negative microscopy and negative culture definition used in pharmaceutical trials (). In a study by Bunyaratavej et al. per-protocol mycological and clinical cure were achieved by 35% (7/20) and 10% (2/20) of patients receiving Nd: YAG 1064 nm laser monotherapy, respectively.
Table 2. Summary of randomized controlled trials (RCTs) on lasers for the treatment of toenail onychomycosis.
Two other RCTs were not included in as outcomes for fingernails and toenails were not reported separately (Citation29,Citation30). Nd: YAG and fractional CO2 lasers in combination with topical agents were equally efficacious as laser monotherapies, and were more effective than topical monotherapies. The treatment related adverse effects of laser therapy reported in a majority of the trials were mild-to-moderate, and primarily include pain. One study, however, reported moderate-to-severe pain, burning sensation, and nail -fold swelling (Citation30). Other RCTs (n = 12) were excluded as they did not use a laser monotherapy arm to demonstrate laser contribution to clinical outcomes, or did not report clinical and mycological outcomes following recommended guidelines.
The reported laser data is of poor quality, and even within RCTs the treatment parameters and clinical outcomes measured varied widely. The limited number of trials utilizing standard mycology outcomes () such as negative culture and negative microscopy/histology diminishes the comparability of the studies to established pharmaceutical treatment and could reduce promotion of lasers among physicians (Citation30–32). Larger, better-controlled RCTs are required to provide convincing evidence of laser efficacy (Citation24–26).
Photodynamic therapy
Background and mechanism
Photodynamic therapy (PDT) aims to induce the apoptosis of fungal cells by the localized generation of reactive oxygen species (ROS) (Citation33). This is achieved by selective uptake of a photosensitizing agent by fungal cells, which is then activated by an appropriate light source (Citation33). Photosensitizers (PSs), one of the three main factors required for PDT, may belong to the porphyrin, chlorophyll, or dye families. Porphyrin photosensitizers such as methyl aminolevulinate (MAL) and 5-aminolevulinic acid (ALA) are the most commonly used agents in dermatological PDT applications and have regulatory clearance for actinic keratosis and several types of cancers (Citation19,Citation34). Other PSs such as methylene blue (MB), Rose Bengal, and hypericin have also been investigated and show therapeutic efficacy against fungal pathogens, in vitro and in vivo (Citation34). None of the existing PSs have been cleared for use in onychomycosis to date (Citation33).
A light source specific to the PS, and oxygen in the surrounding area for ROS generation, are the two other factors essential for PDT. Intense pulsed light, lasers, light-emitting diodes, and lamps may be used as the light source depending on their wavelength; an optimum source of light provides a wavelength that penetrate the nail or other tissue while also activating the PS (Citation33).
For onychomycosis, a solution containing PS is applied to the nail. The PS is ideally preferentially absorbed by the fungal cells and not the surrounding tissue. The PS may be in the form of a prodrug which is converted to the active agent upon illumination, as is the case with MAL. When the nail is illuminated with a light source, the PS is sensitized and utilizes the energy and the oxygen in the surroundings to produce ROS through one of two pathways (). In the type I reaction, electron or proton transfer occurs between the PS and components of the fungal cell to produce radical species that can react with oxygen to produce ROS, causing cellular damage (Citation35). In the type II reaction, energy transfer occurs from the PS to the oxygen molecules in the surrounding resulting in singlet oxygen production (Citation35,Citation36). This is a highly reactive form and is cytotoxic to the cells. The oxidative stress on the fungal pathogen leads to cellular damage and apoptosis, resulting in its destruction and elimination by the immune system (Citation19,Citation33,Citation34,Citation37). One of the primary advantages of PDT is the reduced cross-reactivity with other drugs, enabling its use in populations with comorbidities who are undergoing other treatments. Being a noninvasive and targeted approach, selective areas of the nail can be treated (Citation33).
Clinical use
Multiple clinical studies including RCTs (n = 5) and non-randomized open label clinical trials (n = 4) have been published on PDT to date (Citation34). MB, MAL, and ALA are the most commonly used PSs for onychomycosis (Citation33,Citation34). Of the 9 reports identified from our systematic search, 5 studies were RCTs and 6 studies reported complete mycological cure rates in the study population () (Citation38–42). Among the 5 RCTs, three reports of 2% aqueous solution of MB provided outcomes specifically for toenails in 140 patients and are summarized in . As with lasers, treatment regimens varied with PDT: 8-12 sessions at 1-2-week intervals were administered. Urea 40% ointment was used as a pretreatment in two studies to increase the permeability of the nail and the penetration of MB through the nail plate [Citation39,Citation43], but PDT without urea pretreatment was not used as comparator in either trial, and so may bias the outcomes versus PDT monotherapy. Urea/PDT demonstrated higher mycological (70% [7/10] vs. 40% [4/10]) and clinical (60% [6/10] vs. 30% [3/10]) cure rates at week 40 from baseline (per protocol analysis) than fractional Er:YAG laser/PDT, although the differences were not statistically significant and complete cure rates were not reported (Citation38).
Table 3. Summary of randomized controlled trials (RCTs) on photodynamic therapy (PDT) for the treatment of toenail onychomycosis.
Similarly, in another study, urea/PDT had higher mycological (70% [14/20] vs. 30% [6/20]) and clinical (80% [16/10] vs. 25% [5/20]) cure rates at week 28 compared to intense pulsed light (Citation42).
Comparing MB PDT to oral fluconazole in a study by Souza et al., a significantly higher complete cure rate was achieved with MB PDT(50% [20/40] vs. 25% [10/40]) (Citation39).
There is a paucity of reports on PDT therapy for onychomycosis, and given the lack of standardized methods and outcome reporting, including the inconsistent definitions of mycological and clinical cure, clinical improvement outcomes cannot be compared between the existing reports (Citation3,Citation34). PDT showed no or mild adverse effects in the summarized trials. Mycology outcomes in the few RCTs suggest PDT may provide effective antifungal effect, but larger, better controlled trials are required to confirm this effect.
Microwaves
Background and mechanism
The use of microwaves to treat onychomycosis has been considered a possibility for several years (Citation43–45). Microwaves are non-ionizing electromagnetic waves with lengths between 1 and 1000 mm, and frequencies between 0.3 and 300 GHz (Citation44). The microwave oven was patented by Percy Spencer in 1945, following his recognition of magnetron emitting heat-generating microwaves during experimentation with radar (Citation46). Although the first models were the size of a refrigerator, the next few decades saw innovation to bring the microwave down to the size it is today (Citation46).
In addition to the role they play in heating food for the preference of the palate, microwaves have both thermal and non-thermal germicidal activities (Citation44,Citation47). Through its thermal mechanism as demonstrated in vitro, microwaves denature proteins for the direct killing of pathogens (Citation48). Non-thermal effects include the induction of DNA damage, as well as the deactivation of genes involved in oxidation regulation, leading to bacterial deactivation (Citation47). Other research suggests that microwaves upregulate both an immune response and apoptosis via hyperthermic stimulation (Citation44). The Swift® microwave device (Emblation Ltd, UK) is proposed to upregulate immune factors such as IL-6, IL-8, IL-12, IL-18, INF-γ, TNF-α, and heat shock proteins; in addition to downregulating PI3K/PIK3R2 signaling (Citation49). The role of any of these mechanisms in onychomycosis remains to be determined.
Following exposure at 832 MHz, microwaves either partially or completely inhibited the in vitro growth of Aspergillus niger, Trichophyton mentagrophytes, and Penicillium spp (Citation50). Using a different radiation source (2450 MHz), a 30-s microwave session at 240 W resulted in the complete growth inhibition of T. rubrum (Citation51). In vitro growth inhibition of T. interdigitale occurred after microwave irradiation at 480 W for 50 s; Microsporum canis was inhibited after microwave irradiation at 240 W for 70 s (Citation51). When high-energy microwaves are used on yeast over a long period of time, it is believed that their thermal effect is the main fungicidal mechanism (Citation50,Citation52). This is evidenced by the finding of reduced fungicidal effects that required longer radiation periods in dry environments, where microwaves have less water to heat (Citation50,Citation52).
Clinical use
There are no microwave devices cleared specifically for onychomycosis, and no RCTs with microwaves for onychomycosis to date. Reports for microwaves in onychomycosis utilized systems cleared for general dermatology and podiatry applications at an 8 GHz fixed frequency with variable power of 1–10 W (Swift® microwave system), or at a 5.8 GHz fixed frequency with variable treatment intervals and energy levels (miraDry®, Miramar Labs Inc., Santa Clara USA) (Citation44). The Swift® device has been reported to successfully treat onychomycosis in a 78-year-old woman after three passes at 7–9 W for 3 s each with the treatment being repeated six times over several months (Citation44). Representative patient photographs demonstrating clinical improvements are shown in . On 6 January 2023, another pilot study using the Swift® microwave device to treat toenail onychomycosis began recruiting; the results are not expected until December 2024 (NCT05674747). Utility of microwave therapy for onychomycosis cannot be determined until more clinical data becomes available.
Figure 5. Photographs showing microwave treatment of toenail. Toenails of two patients treated with 4 and 5 sessions. (A) The toenail of a patient after the first microwave treatment, after 6 weeks, having received a total of 4 microwave treatments, and after 2 years follow-up. (B) The toenail of another patient after the first microwave treatment, after 1.5 years, having received 5 microwave treatments, and after 3 years at follow-up (Citation44).
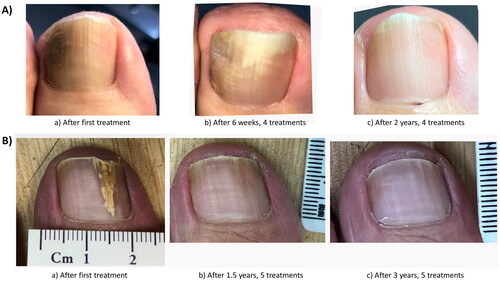
Non-thermal plasma
Background and mechanism
Non-thermal plasma (NTP) is electrically energized neutral gas or air molecules, which can be generated through passage across an electric field, among other methods (Citation53,Citation54). NTP is used in multiple industries in addition to medicine, including the food, aerospace, chemical synthesis, and power generation industries (Citation55–59). In both food processing and medicine, NTP can be used to modify proteins, biomolecule oxidation, enzyme and prodrug activation, for pesticide dissipation, and as an antimicrobial treatment (Citation60–63). NTP kills fungus in five ways: (1) electron and ion bombardment, (2) radiation, (3) heat, (4) reactive oxygen species such as hydrogen peroxide and ozone, and (5) reactive nitrogen species such as nitrogen dioxide, nitric oxide, nitrous acid, and peroxynitrite anion (Citation64,Citation65). Reactive oxygen species, and to a lesser extent reactive nitrogen species can destroy cell walls and are thought to be the main mechanism by which NTP inactivates fungus (Citation64,Citation66).
In vitro, a 5-, 8- and 10-min daily NTP session administered for 8 days inhibited the growth of dermatophytes (T. rubrum and M. canis); the degree of inhibition appears to be proportional with the duration of exposure (Citation67).
Clinical use
Only one pilot clinical study on NTP use in onychomycosis has been conducted (Citation68). Of the 13 patients, 12 had T. rubrum and 1 had Candida albicans (Citation68). Three distinct protocols were tested: (1) three 10-min plasma treatments per week for one week with follow-up treatments at months 1, 3, and 6; (2) three 10-min treatments per week for one week, with monthly follow-up treatments at months 1, 2, 3, 4, 5, and 6; (3) three 20-min treatments per week for one week, with monthly follow-up treatments at months 1, 2, 3, 4, 5, and 6 (Citation68). The clinical cure rates at the 6-month follow-up after the start of treatment were measured by nail plate planimetry and defined as a 3–5 mm increase of clear nail or a clinically normal nail (Citation68). For protocol 1, clinical cure rates were 75% (3/4 patients), for protocol 2 − 33.3% (2/6 patients), and for the third protocol 66.7% (2/3 patients) (Citation68). Mycological cure rates, measured by negative fungal culture and KOH 6 months after the start of treatment were 25% (1/4) for the first treatment protocol, 16.7% (1/6) for the second protocol, and 0% for the third NTP protocol (Citation68). Only one adverse event was observed in protocol group 1, where a patient’s nail was singed due to an electrode in disrepair (Citation68). An additional case report indicated no adverse effects for a diabetic patient with dermatophyte onychomycosis treated with plasma therapy for 3 weeks (Citation69). Increased treatment frequency appears to provide less efficacy, but there are too few patients treated to draw any significant conclusions. More investigation and standardization are needed before NTP can be endorsed as an effective onychomycosis therapy.
Penetration enhancement
Iontophoresis
Background and mechanism
Iontophoresis is a technique in which a mild electric current assists drugs in their passage through a barrier like skin (Citation70). First proposed in the mid-eighteenth century, iontophoretic drug delivery has never been widely adopted for any purpose, but has proved its worth in select cases of transdermal delivery (Citation71).
As with ultrasound, iontophoresis does not directly affect onychomycosis, but instead increases nail permeability to topical medications. Three mechanisms may play a role in iontophoresis-enhanced transport of drugs. The first is electropermeabilization, which produces field-induced changes to a membrane resulting in increased membrane permeability (Citation72,Citation73). The second mechanism is electroosmosis, where the application of an electric current causes a solvent to flow across a charged biomembrane (Citation74). The third is electrophoresis or electromigration in which a charged particle is pulled toward an oppositely charged electrode (Citation75,Citation76). Iontophoresis therefore works best on ionized drugs, as without a charge they would not move toward an oppositely charged electrode or have an affinity for the charged membrane (here, the nail plate).
To date, the transungual delivery of several drugs including terbinafine, ciclopirox, mannitol, and salicylic acid can be enhanced by iontophoresis (Citation70,Citation77–79). A study examined the application of ciclopirox nail lacquer, in conjunction with iontophoretic (9 V) or passive diffusion, on human cadavers (Citation70). Ciclopirox was delivered across the nail plate at a significantly higher rate in the iontophoresis group after both 9 and 24 h of treatment (Citation70). Furthermore, nail clippings subjected to iontophoresis with topically applied terbinafine increased terbinafine concentration by 16-fold compared to control (Citation80).
Clinical use
Several iontophoretic products are marketed for transdermal drug delivery, but none are currently purchasable for transungual, or inter-nail plate, drug delivery (Citation70). In our systematic search, two RCTs investigating the efficacy of iontophoresis for onychomycosis were identified ().
In a study examining terbinafine iontophoresis-assisted delivery through the nail plate, 38 patients with KOH and culture confirmed distal and lateral subungual dermatophyte onychomycosis were treated with 1% terbinafine HCl either with (20 patients) or without (18 patients) a 1.5 V iontophoretic patch (Citation78). The treatment regimen was 5 overnight applications per week, for four weeks (Citation78). Twelve weeks after the start of treatment (8 weeks after the end of active treatment), the percentage of patients with direct microscopy-detected fungal elements in a nail specimen (KOH positive) were 53% in the non-iontophoretic group, and 16% in the group receiving a patch (Citation78). Another study of iontophoresis-assisted terbinafine application examined 40 subjects with KOH confirmed mild-moderate onychomycosis of the big toe (Citation81). While both groups received 1% terbinafine HCl, the experimental group was treated with 30 min of 3–4 mA/min iontophoresis 3 times per week, for 4 weeks (Citation81). KOH microscopy was done immediately post-treatment with 16 of the 20 KOH positive patients (80%) treated with iontophoresis and terbinafine now being recorded negative (Citation81). In the group that did not receive iontophoresis treatment with terbinafine, only 3 of 20 KOH positive subjects were negative after treatment (Citation81). The pain level and OSI scores significantly decreased between pretreatment and post-treatment (4 weeks difference) in the group receiving iontophoresis with terbinafine (Citation81). Both iontophoresis regimens appear promising, but more rigorous RCTs are needed, with broader mycology investigation.
Nail drilling
Background and mechanisms
The nail plate is a keratinized, multilayered, and highly cross-linked structure with pores and channels (). Among the three layers of the nail plate (dorsal, intermediate, and ventral layers), the top two layers are thicker than the ventral layer and consist of approximately 30% and 50% vs 20% of the nail plate [Citation77]. Fungal pathogens causing onychomycosis are commonly found in the ventral layer and in the subungual space between the nail plate and nail bed (Citation82). Given this anatomy of the nail plate, the primary challenge faced by topical antifungal agents is their penetration through the dorsal and intermediate layers to reach the fungal cells in the ventral layer and the subungual space (Citation76,Citation82).
Figure 6. Photographs showing nail drilling of a toenail. (A) the toenail Shortly after nail drilling and application of topical antifungal. (B) Healthy nail after 6 months, with clear nail observed.
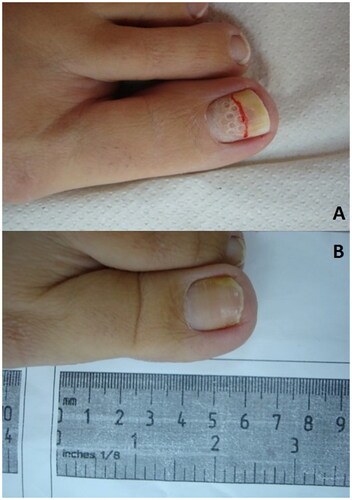
In addition to being multilayered, the cross-linked keratin network has small pores and channels that are difficult to penetrate. Lipophilic drugs are impeded by this keratin network due to their binding affinity to keratin (Citation76). Nail drilling, also known as mechanical debridement or microporation, aids in the penetration of topical antifungal agents by removing sections of the thick dorsal and intermediate layers. This process using nail drills involves the creation of channels in the nail plate that act as reservoirs for topical antifungals, thereby facilitating access to the fungal cells. Nail drilling is used as an adjunctive treatment with topical therapies and cannot lead to the inactivation or removal of fungal cells as a monotherapy (Citation76,Citation82).
Preliminary in vitro testing by Díaz et al. using the Clearanail® device investigated the penetration of ciclopirox through the nail plate ex vivo and found that the penetration of ciclopirox increased significantly (3–4 times higher) after 42 days (Citation82).
Clinical use
No RCTs for nail drilling have been reported to date. In an open trial by Shemer at al., nail drilling plus topical terbinafine significantly improved the degree of nail clearance and mycological cure rates at week 28 compared to topical terbinafine alone (Citation83). An example of clear nail obtained after topical treatment assisted by nail drilling is presented in . Use of nail drilling to augment topical antifungal penetration after detailed foot care intervention has also been reported in a small number of patients, with some favorable outcomes noted (Citation84). Wider trials are needed to establish utility conclusively.
Ultrasound
Background and mechanism
The concept of sound navigation and ranging (SONAR), using the expulsion of a pulse of sound and the detection of its echoes, is the basis of medical ultrasound technology (Citation85). The earliest applications of this technology in biological tissue were during and after World War II, where it was used to visualize the thickness of the intestinal wall in humans for the detection of gastric cancer, and to detect gallstones in dogs (Citation85). In the modern clinic, ultrasound is still mainly purposed for imaging although focused ultrasound can also be used to increase the permeability of tissues (Citation86–88).
Ultrasound does not directly produce antifungal activity; instead, the actions of ultrasound increase nail permeability to other external treatment applications. There are two main mechanisms by which ultrasound can increase tissue permeability: cavitation, in which micrometer-sized pores are introduced to a barrier by microbubbles; and acoustic streaming where local fluid currents move in response to ultrasound waves as bulk streaming (same direction as waves) and micro-streaming (movement not in direction with waves) (Citation89–91). In nails, the mechanism by which ultrasound increases permeability is incompletely understood; however, due to the observation that increased compound delivery occurs at higher ultrasound frequencies, Kline-Schoder et al. suggested both types of acoustic streaming: micro-streaming and bulk streaming (Citation90,Citation91). Higher frequencies are associated with higher acoustic streaming velocity, which could be aiding the topical drugs in their journey through the nail (Citation92).
In a 2011 study, an ultrasound transducer device applied for 30 s increased the permeability of a canine nail to blue dye (Citation93). A similar system, tested on porcine nails, also increased nail permeability in both cell diffusion and luminosity testing, which assessed the ability of ciclopirox to penetrate the nail plate, and dye to enter the nail, respectively (Citation45,Citation91,Citation94). Ciclopirox permeated the nail optimally at ultrasound frequencies of 800–1000 kHz; however, this frequency generated considerable levels of heat (Citation91,Citation94). In a follow-up paper by Klein-Schroder et al. ultrasound applied to porcine and human nails in a continuous mode at full strength for 1 min was more effective than use in 50% pulsing mode for 3 and 5 min (Citation90).
Clinical use
There is no reported clinical use of ultrasound for onychomycosis to date, and this device cannot be considered as a useful device for penetration enhancement at this time.
Conclusions
This review illustrates that no devices to date have established reliable efficacy for onychomycosis for toe nails. There are few clinical reports available for devices with the exception of lasers, and even within lasers the data quality is not sufficient to conclusively promote its use for onychomycosis. Device testing should be attentive to providing the best possible data to potential users that can clearly establish its advantages in onychomycosis.
In theory, device-based antifungal therapies for treating onychomycosis are appealing alternatives to oral and topical antifungal agents due to the low likelihood of systemic side-effects, such as potential liver toxicity and drug interactions. Their mechanisms of action are not reliant on the molecular pathways associated with drug resistance in onychomycosis, and they offer a targeted approach to treatment due to control over the application area and intensity. Current clinical data does not move the utility of devices beyond theory.
In the era of developing antifungal resistance, device research needs to provide data more directly comparable to that of pharmaceutical options in order to be widely adopted by clinicians for onychomycosis.
Author contributions
Conceptualization, A.K.G.; Investigation, S.P.R. and S.H-N.; Methodology; S.P.R., S.H-N. and T.W.; Project Administration, A.K.G. and E.A.C.; Supervision, A.K.G. and E.A.C.; Validation; A.K.G., S.P.R., S.H-N., T.W. and E.A.C.; Visualization, S.P.R. and S.H-N.; Writing – Original Draft Preparation: S.P.R., S.H-N. and E.A.C.; Writing – Review and Editing; A.K.G., S.P.R., S.H-N., T.W. and E.A.C.
Acknowledgements
Some of the figures were created using BioRender.
Disclosure statement
No potential conflict of interest was reported by the author(s).
Data availability statement
Data sharing not applicable – no new data generated.
Additional information
Funding
References
- Lipner SR. Pharmacotherapy for onychomycosis: new and emerging treatments. Expert Opin Pharmacother. 2019;20(6):1–13. doi: 10.1080/14656566.2019.1571039.
- Gupta AK, Stec N. Recent advances in therapies for onychomycosis and its management. F1000Res. 2019;8:968. doi: 10.12688/f1000research.18646.1.
- Gupta AK, Hall DC, Simkovich AJ. How effective are devices in the management of onychomycosis?: a systematic review. J Am Podiatr Med Assoc. 2023;113(1):21–240.
- Gupta AK, Stec N, Summerbell RC, et al. Onychomycosis: a review. J Eur Acad Dermatol Venereol. 2020;34(9):1972–1990. doi: 10.1111/jdv.16394.
- Falotico JM, Lipner SR. Updated perspectives on the diagnosis and management of onychomycosis. Clin Cosmet Investig Dermatol. 2022;15:1933–1957. doi: 10.2147/CCID.S362635.
- Gupta AK, Mays RR, Versteeg SG, et al. Global perspectives for the management of onychomycosis. Int J Dermatol. 2019;58(10):1118–1129. doi: 10.1111/ijd.14346.
- Gupta AK, Gupta G, Jain HC, et al. The prevalence of unsuspected onychomycosis and its causative organisms in a multicentre Canadian sample of 30 000 patients visiting physicians’ offices. J Eur Acad Dermatol Venereol. 2016;30(9):1567–1572. doi: 10.1111/jdv.13677.
- Gupta AK, Summerbell RC, Venkataraman M, et al. Nondermatophyte mould onychomycosis. J Eur Acad Dermatol Venereol. 2021;35(8):1628–1641. doi: 10.1111/jdv.17240.
- Gupta AK, Versteeg SG, Shear NH. Confirmatory testing prior to initiating onychomycosis therapy is cost-effective. J Cutan Med Surg. 2018;22(2):129–141. doi: 10.1177/1203475417733461.
- Del Rosso JQ. The role of topical antifungal therapy for onychomycosis and the emergence of newer agents. J Clin Aesthet Dermatol. 2014;7:10.
- Ebert A, Monod M, Salamin K, et al. Alarming India-wide phenomenon of antifungal resistance in dermatophytes: a multicentre study. Mycoses. 2020;63(7):717–728. doi: 10.1111/myc.13091.
- US Food and Drug Administration. Medical devices and clinical trial design for the treatment or improvement in the appearance of fungally-infected nails guidance for industry and Food and Drug Administration staff preface public comment [Internet]. 2016. Available from: https://www.fda.gov/regulatory-information/search-fda-guidance-documents/medical-devices-and-clinical-trial-design-treatment-or-improvement-appearance-fungally-infected.
- Abd-Elsalam WH, Abouelatta SM. Contemporary techniques and potential transungual drug delivery nanosystems for the treatment of onychomycosis. AAPS PharmSciTech. 2023;24(6):150. doi: 10.1208/s12249-023-02603-x.
- Page MJ, McKenzie JE, Bossuyt PM, et al. The PRISMA 2020 statement: an updated guideline for reporting systematic reviews. BMJ. 2021;372:n71. doi: 10.1136/bmj.n71.
- Gianfaldoni S, Tchernev G, Wollina U, et al. An overview of laser in dermatology: the past, the present and … the future (?). Open Access Maced J Med Sci. 2017;5(4):526–530. doi: 10.3889/oamjms.2017.130.
- Ma W, Si C, Kasyanju Carrero LM, et al. Laser treatment for onychomycosis: a systematic review and meta-analysis. Medicine. 2019;98(48):e17948. doi: 10.1097/MD.0000000000017948.
- Gupta AK, Simpson FC, Heller DF. The future of lasers in onychomycosis. J Dermatolog Treat. 2016;27(2):167–172. doi: 10.3109/09546634.2015.1066479.
- Gupta AK, Simpson FC. Laser therapy for onychomycosis. J Cutan Med Surg. 2013;17(5):301–307. doi: 10.2310/7750.2012.12060.
- Bhatta AK, Keyal U, Wang X, et al. A review of the mechanism of action of lasers and photodynamic therapy for onychomycosis. Lasers Med Sci. 2017;32(2):469–474. doi: 10.1007/s10103-016-2110-9.
- Farkas JP, Hoopman JE, Kenkel JM. Five parameters you must understand to master control of your laser/light-based devices. Aesthet Surg J. 2013;33(7):1059–1064. doi: 10.1177/1090820X13501174.
- Hruza GJ, Dover JS. Laser skin resurfacing. Arch Dermatol. 1996;132(4):451–455. doi: 10.1001/archderm.1996.03890280115016.
- Zang K, Sullivan R, Shanks S. A retrospective study of non-thermal laser therapy for the treatment of toenail onychomycosis. J Clin Aesthet Dermatol. 2017;10:24–30.
- Paasch U, Mock A, Grunewald S, et al. Antifungal efficacy of lasers against dermatophytes and yeasts in vitro. Int J Hyperthermia. 2013;29(6):544–550. doi: 10.3109/02656736.2013.823672.
- Carney C, Cantrell W, Warner J, et al. Treatment of onychomycosis using a submillisecond 1064-nm neodymium:yttrium-aluminum-garnet laser. J Am Acad Dermatol. 2013;69(4):578–582. doi: 10.1016/j.jaad.2013.04.054.
- Bunyaratavej S, Wanitphakdeedecha R, Ungaksornpairote C, et al. Randomized controlled trial comparing long-pulsed 1064-Nm neodymium: yttrium-aluminum-garnet laser alone, topical amorolfine nail lacquer alone, and a combination for nondermatophyte onychomycosis treatment. J Cosmet Dermatol. 2020;19(9):2333–2338. doi: 10.1111/jocd.13291.
- Sabbah L, Gagnon C, Bernier FE, et al. A randomized, double-blind, controlled trial evaluating the efficacy of Nd: YAG 1064 nm short-pulse laser compared with placebo in the treatment of toenail onychomycosis. J Cutan Med Surg. 2019;23(5):507–512. doi: 10.1177/1203475419861071.
- Karsai S, Jäger M, Oesterhelt A, et al. Treating onychomycosis with the short-pulsed 1064-nm-Nd: YAG laser: results of a prospective randomized controlled trial. J Eur Acad Dermatol Venereol. 2017;31(1):175–180. doi: 10.1111/jdv.13798.
- Nijenhuis-Rosien L, Kleefstra N, van Dijk PR, et al. Laser therapy for onychomycosis in patients with diabetes at risk for foot ulcers: a randomized, quadruple-blind, sham-controlled trial (LASER-1). J Eur Acad Dermatol Venereol. 2019;33(11):2143–2150. doi: 10.1111/jdv.15601.
- Kim TI, Shin MK, Jeong KH, et al. A randomised comparative study of 1064 nm neodymium-doped yttrium aluminium garnet (Nd: YAG) laser and topical antifungal treatment of onychomycosis. Mycoses. 2016;59(12):803–810. doi: 10.1111/myc.12534.
- Bashir S, Hassan I, Mubashir S. Carbon dioxide laser plus topical 5% luliconazole: a better combination therapeutic modality for onychomycosis. J Cutan Aesthet Surg. 2021;14:318–322.
- Aukstikalnyte AE, Cibien E, De Demo P, et al. Combination of a triple wavelength (650 nm, 810 nm, and 915 nm) class IV laser system and local mechanical abrasion in the treatment of chronic toenail onychomycosis: an uncontrolled prospective pilot study. Int J Dermatol. 2022;61(3):367–371. doi: 10.1111/ijd.15869.
- El-Tatawy RA, El-Naby NMA, El-Hawary EE, et al. A comparative clinical and mycological study of Nd-YAG laser versus topical terbinafine in the treatment of onychomycosis. J Dermatolog Treat. 2015;26(5):461–464. doi: 10.3109/09546634.2014.998607.
- Bhatta AK, Keyal U, Wang XL. Photodynamic therapy for onychomycosis: a systematic review. Photodiagnosis Photodyn Ther. 2016;15:228–235. doi: 10.1016/j.pdpdt.2016.07.010.
- Shen JJ, Jemec GBE, Arendrup MC, et al. Photodynamic therapy treatment of superficial fungal infections: a systematic review. Photodiagnosis Photodyn Ther. 2020;31:101774. doi: 10.1016/j.pdpdt.2020.101774.
- Sai DL, Lee J, Nguyen DL, et al. Tailoring photosensitive ROS for advanced photodynamic therapy. Exp Mol Med. 2021;53(4):495–504. doi: 10.1038/s12276-021-00599-7.
- Dai T, Fuchs BB, Coleman JJ, et al. Concepts and principles of photodynamic therapy as an alternative antifungal discovery platform. Front Microbiol. 2012;3:120. doi: 10.3389/fmicb.2012.00120.
- Boltes Cecatto R, Siqueira de Magalhães L, Fernanda Setúbal Destro Rodrigues M, et al. Methylene blue mediated antimicrobial photodynamic therapy in clinical human studies: the state of the art. Photodiagnosis Photodyn Ther. 2020;31:101828. doi: 10.1016/j.pdpdt.2020.101828.
- Alberdi E, Gómez C. Urea versus fractional Er: YAG laser pretreatment of methylene blue photodynamic therapy in the treatment of moderate toenail onychomycosis: short- and medium-term effects. Arch Dermatol Res. 2023;315(4):787–794. doi: 10.1007/s00403-022-02448-7.
- Figueiredo Souza LW, Souza SVT, Botelho ACC. Randomized controlled trial comparing photodynamic therapy based on methylene blue dye and fluconazole for toenail onychomycosis. Dermatol Ther. 2014;27(1):43–47. doi: 10.1111/dth.12042.
- Gilaberte Y, Robres MP, Frías MP, et al. Methyl aminolevulinate photodynamic therapy for onychomycosis: a multicentre, randomized, controlled clinical trial. J Eur Acad Dermatol Venereol. 2017;31(2):347–354. doi: 10.1111/jdv.13842.
- Sobhy N, Talla Eweed H, Omar SS. Fractional CO2 laser - assisted methylene blue photodynamic therapy is a potential alternative therapy for onychomycosis in the era of antifungal resistance. Photodiagnosis Photodyn Ther. 2022;40:103149. doi: 10.1016/j.pdpdt.2022.103149.
- Alberdi E, Gómez C. Efficiency of methylene blue-mediated photodynamic therapy vs intense pulsed light in the treatment of onychomycosis in the toenails. Photodermatol Photoimmunol Photomed. 2019;35(2):69–77. doi: 10.1111/phpp.12420.
- Gupta AK, Venkataraman M, Renaud HJ, et al. A paradigm shift in the treatment and management of onychomycosis. Skin Appendage Disord. 2021;7(5):351–358. doi: 10.1159/000516112.
- Gupta AK, Venkataraman M, Joshi LT, et al. Potential use of microwave technology in dermatology. J Dermatolog Treat. 2022;33(7):2899–2910. doi: 10.1080/09546634.2022.2089333.
- Gupta AK, Hall DC, Simkovich AJ. How effective are devices in the management of onychomycosis? A systematic review. J Am Podiatr Med Assoc. 2023;113(1)doi: 10.7547/21-240.
- Strauss R. Microwave oven [Internet]. Smithsonian Snapshot; 2013 [cited 2023 May 22]. Available from: https://www.si.edu/newsdesk/snapshot/microwave-oven.
- Shaw P, Kumar N, Mumtaz S, et al. Evaluation of non-thermal effect of microwave radiation and its mode of action in bacterial cell inactivation. Sci Rep. 2021;11(1):14003. doi: 10.1038/s41598-021-93274-w.
- Deng X, Huang H, Huang S, et al. Insight into the incredible effects of microwave heating: driving changes in the structure, properties and functions of macromolecular nutrients in novel food. Front Nutr. 2022;9:941527. doi: 10.3389/fnut.2022.941527.
- Beale G, McErlean E, Kidd M, et al. Treatment of fungal infections 2022 emblation patent [Internet]. USA; 2022. p. 1–12. Available from: https://patentimages.storage.googleapis.com/66/8c/25/f44def8c5e006d/US20220219007A1.pdf.
- Ahmed LT, Majeed AD, Salhi A. The effect of mobile waves on the growth of pathogenic fungi. IntJCurrMicrobiolAppSci. 2015;4:838–842.
- Budihardja D, Mayser P. The effect of microwave irradiation on the vitality of various dermatophytes. Mycoses. 2014;57(4):209–213. doi: 10.1111/myc.12144.
- A. Al-Mayah A, T. Ali E. Mobile microwave effect on bacterial antibiotic sensitivity. Bas.J.Vet.Res. 2010;9(2):89–102. doi: 10.33762/bvetr.2010.55052.
- López M, Calvo T, Prieto M, et al. A review on non-thermal atmospheric plasma for food preservation: mode of action, determinants of effectiveness, and applications. Front Microbiol. 2019;10:622. doi: 10.3389/fmicb.2019.00622.
- Conrads H, Schmidt M. Plasma generation and plasma sources. Plasma Sources Sci. Technol. 2000;9(4):441–454. doi: 10.1088/0963-0252/9/4/301.
- Molokov S, Moreau R, Moffatt HK. Magnetohydrodynamics: historical evolution and trends. In: Molokov S, Moreau R, Moffatt HK, editors. Dordecht: Springer; 2007.
- Neyts EC, Bogaerts A. Understanding plasma catalysis through modelling and simulation – a review. J Phys D Appl Phys. 2014;47(22):224010.
- Sherman A. Magnetohydrodynamic channel flows with nonequilibrium ionization. Physics of Fluids. 1966;9(9):1782–1787. doi: 10.1063/1.1761933.
- Kar R, Chand N, Bute A, et al. Cold plasma: clean technology to destroy pathogenic micro-organisms. Trans Indian Natl Acad Eng. 2020;5(2):327–331. doi: 10.1007/s41403-020-00133-7.
- Laroussi M. Sterilization of contaminated matter with an atmospheric pressure plasma. IEEE Trans Plasma Sci. 1996;24(3):1188–1191. doi: 10.1109/27.533129.
- Wende K, Nasri Z, Striesow J, et al. Is biomolecule oxidation by plasma-derived reactive species restricted to the Gas-Liquid interphase? 2022 IEEE International Conference on Plasma Science (ICOPS); 2022. p. 1–2.
- Ahmadi M, Potlitz F, Link A, et al. Flucytosine-based prodrug activation by cold physical plasma. Arch Pharm. 2022;355(9):e2200061. doi: 10.1002/ardp.202200061.
- Nasri Z, Memari S, Wenske S, et al. Singlet-Oxygen-Induced phospholipase A2 inhibition: a major role for interfacial tryptophan dioxidation. Chemistry. 2021;27(59):14702–14710. doi: 10.1002/chem.202102306.
- Ahmadi M, Nasri Z, Von Woedtke T, et al. D -Glucose oxidation by cold atmospheric plasma-induced reactive species. ACS Omega. 2022;7(36):31983–31998. doi: 10.1021/acsomega.2c02965.
- Hoppanová L, Kryštofová S. Nonthermal plasma effects on fungi: applications, fungal responses, and future perspectives. Int J Mol Sci. 2022;23(19):11592. doi: 10.3390/ijms231911592.
- Ehlbeck J, Schnabel U, Polak M, et al. Low temperature atmospheric pressure plasma sources for microbial decontamination. J Phys D Appl Phys. 2011;44(1):013002. doi: 10.1088/0022-3727/44/1/013002.
- Misra NN, Yadav B, Roopesh MS, et al. Cold plasma for effective fungal and mycotoxin control in foods: mechanisms, inactivation effects, and applications. Compr Rev Food Sci Food Saf. 2019;18(1):106–120. doi: 10.1111/1541-4337.12398.
- Heinlin J, Maisch T, Zimmermann JL, et al. Contact-free inactivation of Trichophyton rubrum and microsporum canis by cold atmospheric plasma treatment. Future Microbiol. 2013;8(9):1097–1106. doi: 10.2217/fmb.13.86.
- Lipner SR, Friedman G, Scher RK. Pilot study to evaluate a plasma device for the treatment of onychomycosis. Clin Exp Dermatol. 2017;42(3):295–298. doi: 10.1111/ced.12973.
- Terabe Y, Kaneko N, Ando H. Treating hard-to-heal skin and nail onychomycosis of diabetic foot with plasma therapy. Dermatol Ther. 2021;34:e15127.
- Hao J, Smith KA, Li SK. Iontophoretically enhanced ciclopirox delivery into and across human nail plate. J Pharm Sci. 2009;98(10):3608–3616. doi: 10.1002/jps.21664.
- Helmstadtler A. The history of electrically-assisted transdermal drug delivery (iontophoresis). Pharmazie. 2001;56:583–587.
- Glaser RW, Leikin SL, Chernomordik LV, et al. Reversible electrical breakdown of lipid bilayers: formation and evolution of pores. Biochim Biophys Acta. 1988;940(2):275–287. doi: 10.1016/0005-2736(88)90202-7.
- Chizmadzhev YA, Indenbom AV, Kuzmin PI, et al. Electrical properties of skin at moderate voltages: contribution of appendageal macropores. Biophys J. 1998;74(2 Pt 1):843–856. doi: 10.1016/S0006-3495(98)74008-1.
- Pikal MJ. The role of electroosmotic flow in transdermal iontophoresis. Adv Drug Deliv Rev. 2001;46(1–3):281–305. doi: 10.1016/s0169-409x(00)00138-1.
- Kasting GB. Theoretical models for iontophoretic delivery. Adv Drug Deliv Rev. 1992;9(2):177–199.
- Gupta AK, Polla Ravi S, Choi SY, et al. Strategies for the enhancement of nail plate permeation of drugs to treat onychomycosis. J Eur Acad Dermatol Venereol. 2023;37(2):243–255. doi: 10.1111/jdv.18638.
- Murthy SN, Wiskirchen DE, Bowers CP. Iontophoretic drug delivery across human nail. J Pharm Sci. 2007;96(2):305–311. doi: 10.1002/jps.20757.
- Amichai B, Nitzan B, Mosckovitz R, et al. Iontophoretic delivery of terbinafine in onychomycosis: a preliminary study. Br J Dermatol. 2010;162(1):46–50. doi: 10.1111/j.1365-2133.2009.09414.x.
- Dutet J, Delgado-Charro B. Electroosmotic transport of mannitol across human nail during constant current iontophoresis. J Pharm Pharmacol. 2010;62(6):721–729. doi: 10.1211/jpp.62.06.0008.
- Nair AB, Vaka SRK, Sammeta SM, et al. Trans-ungual iontophoretic delivery of terbinafine. J Pharm Sci. 2009;98(5):1788–1796. doi: 10.1002/jps.21555.
- Samhan AF, Abdelhalim NM. Terbinafine hydrochloride 1% iontophoresis for the treatment of toenail onychomycosis: a randomized placebo controlled study. J Nov Physiother. 2015;05(02):1–6. doi: 10.4172/2165-7025.1000252.
- Díaz DC, Iglesias MEL, Vallejo Rb de B, et al. Transungual delivery of ciclopirox is increased 3–4-fold by mechanical fenestration of human nail plate in an in vitro model. Pharmaceutics. 2019;11:29.
- Shemer A, Gupta A, Amichai B, et al. An open comparative study of nail drilling as adjunctive treatment for toenail onychomycosis. J Dermatolog Treat. 2016;27(5):480–483. doi: 10.3109/09546634.2016.1151856.
- Sumikawa M, Egawa T, Honda I, et al. Effects of foot care intervention including nail drilling combined with topical antifungal application in diabetic patients with onychomycosis. J Dermatol. 2007;34(7):456–464. doi: 10.1111/j.1346-8138.2007.00310.x.
- Newman PG, Rozycki GS. The history of ultrasound. Surg Clin North Am. 1998;78(2):179–195. doi: 10.1016/s0039-6109(05)70308-x.
- Sun Y, Xiong X, Pandya D, et al. Enhancing tissue permeability with MRI guided preclinical focused ultrasound system in rabbit muscle: from normal tissue to VX2 tumor. J Control Release. 2017;256:1–8. doi: 10.1016/j.jconrel.2017.04.017.
- Thakkar D, Gupta R, Monson K, et al. Effect of ultrasound on the permeability of vascular wall to nano-emulsion droplets. Ultrasound Med Biol. 2013;39(10):1804–1811. doi: 10.1016/j.ultrasmedbio.2013.04.008.
- Mcmahon D, Bendayan R, Hynynen K. Acute effects of focused ultrasound-induced increases in blood-brain barrier permeability on rat microvascular transcriptome. Sci Rep. 2017;7(1):45657. doi: 10.1038/srep45657.
- Duan L, Yang L, Jin J, et al. Micro/nano-bubble-assisted ultrasound to enhance the EPR effect and potential theranostic applications. Theranostics. 2020;10(2):462–483. doi: 10.7150/thno.37593.
- Kline-Schoder A, Le Z, Liam Sweeney D, et al. Optimization of Ultrasound-Mediated drug delivery for treatment of onychomycosis. J Am Podiatr Med Assoc. 2019. doi: 10.7547/18-084.
- Kline-Schoder A, Le Z, Zderic V. Ultrasound-enhanced drug delivery for treatment of onychomycosis. J Ultrasound Med. 2018;37(7):1743–1752. doi: 10.1002/jum.14526.
- Loh B-G, Hyun S, Ro PI, et al. Acoustic streaming induced by ultrasonic flexural vibrations and associated enhancement of convective heat transfer. J Acoust Soc Am. 2002;111(2):875–883. doi: 10.1121/1.1433811.
- Abadi D, Zderic V. Ultrasound-Mediated nail drug delivery system. J Ultrasound Med. 2011;30(12):1723–1730. doi: 10.7863/jum.2011.30.12.1723.
- Kline-Schoder A, Le Z, Zderic V. Ultrasound-Enhanced ciclopirox delivery for treatment of onychomycosis. Annu Int Conf IEEE Eng Med Biol Soc. 2018;2018:5717–5720. doi: 10.1109/EMBC.2018.8513552.