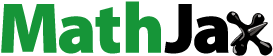
Abstract
Purpose
The hemorrhagic syndrome is a major cause of morbidity and mortality associated with the acute radiation syndrome (ARS). We previously characterized the dose–response relationship for total body irradiation (TBI)-induced ARS in the New Zealand White (NZW) rabbit. Thrombocytopenia, hemorrhage, and anemia were strongly associated with morbidity/mortality during the first three weeks post-TBI. The objective of the current study was to further characterize the natural history of thrombocytopenia, hemostatic dysfunction and hemorrhage in the rabbit model at a TBI dose range to induce ARS.
Methods
Fifty male NZW rabbits were randomized to receive 7.0 or 7.5 Gy of 6 MV-derived TBI. Sham-irradiated controls (n = 6) were included as a comparator. Animals were treated with minimal supportive care including pain medication, antibiotics, antipyretics for temperature >104.8 °F, and fluids for signs of dehydration. Animals were culled at pre-determined timepoints post-TBI, or for signs of imminent mortality based on pre-defined euthanasia criteria. Hematology parameters, serum chemistry, viscoelasticity of whole blood, coagulation tests, and coagulation factor activities were measured. A gross exam of vital organs was performed at necropsy.
Results
Findings in this study include severe neutropenia during the first week post-TBI followed by thrombocytopenia and severe acute anemia with petechial hemorrhages of the skin and hemorrhage of the vital organs during the second to third weeks post-TBI. Abnormalities in whole blood viscoelastometry were observed concurrent with thrombocytopenia and hemorrhage. Antithrombin activity was significantly elevated in animals after exposure to 7.5 Gy, but not 7.0 Gy TBI.
Conclusions
The hemorrhagic syndrome in the rabbit model of TBI recapitulates the pathogenesis described in humans following accidental or deliberate exposures. The rabbit may present an alternative to the rodent model as a small animal species for characterization of the full spectrum of multiorgan injury following TBI and early testing of promising medical countermeasures.
Introduction
The hemorrhagic syndrome is a predominant feature of acute radiation sickness (ARS) and is a major cause of death in acutely exposed individuals (Liebow et al. Citation1949). In humans with ARS, clotting defects characterized by prolonged bleeding and clotting times as well as capillary fragility are routinely observed. Autopsy records from ARS victims describe hemorrhage in the heart, lungs, skeletal musculature, bladder, and intestines (Liebow et al. Citation1949). It has long been speculated that hemorrhage subsequent to total body irradiation (TBI) was the result of impaired clotting ability due to severe thrombocytopenia (platelet count <10,000 cells/μL) in victims with ARS (Cronkite Citation1950; Cronkite et al. Citation1950, Citation1952; Jackson et al. Citation1952). However, elegant studies by Allen and Jacobsen in the late 1940s found a substance with heparin-like properties in the blood of irradiated dogs prior to the onset of thrombocytopenia that caused prolonged clotting time of blood samples taken from healthy, non-irradiated animals (Allen and Jacobson Citation1947). The effect was reversed by anti-heparin substances (e.g. toluidine blue), both in vitro and in vivo, even in the presence of platelet counts <50,000 cells/μL (Allen and Jacobson Citation1947). The ability of anti-heparin substances to control bleeding, with no effect on thrombocytopenia, suggested the deficiencies in hemostasis had origins that were independent of platelet loss alone.
The observations by Allen and Jacobsen were further supported by studies conducted in goats and swine exposed to radiation as a result of an experimental aerial and underwater atomic bomb detonations at Bikini Atoll in the 1940s (Cronkite Citation1950). In goats, coagulation abnormalities were first observed approximately 9 to 16 days after exposure. Again, treatment with anti-heparin substances in vitro reversed the observed clotting abnormalities and restored pro-thrombin times to the normal range (Cronkite Citation1950). In swine, the increased whole blood clotting time occurred between day 6 and 8 post-exposure, which was accompanied by an increase in prothrombin time (Cronkite Citation1950).
While the hemorrhagic syndrome, characterized by prolonged clotting times, visible petechiae and ecchymosis of the skin, and uncontrollable bleeding within the vital organs, is a well-reported phenomenon, the natural history of hemostatic dysfunction has been ill-defined. We previously developed a rabbit model of thrombocytopenia and coagulopathy across the dose-range to induce myelosuppression associated with ARS to elucidate the role of the hemorrhagic syndrome in multiorgan dysfunction/failure after TBI (Jackson et al. Citation2019). In those studies, the pathogenesis of ARS followed a time course consistent with that observed in the canine, sheep, swine, non-human primate and human with similar clinical manifestations – leukopenia, thrombocytopenia, anemia, and, in some cases, severe and fatal hemorrhage (Allen and Jacobson Citation1947; Liebow and Warren Citation1947; Liebow et al. Citation1947, Citation1949; Cronkite Citation1950; Cronkite et al. Citation1950, Citation1952, Citation1995; Stohlman et al. Citation1957; Moroni et al. Citation2011). The objective of the current study was to examine the natural history of the hemorrhagic syndrome in the context of thrombocytopenia and hemostasis dysfunction in the New Zealand White rabbit model of TBI-induced ARS.
Materials and methods
Animals and total body irradiation
Fifty-six adult, uncastrated male New Zealand White Rabbits (Charles River Laboratories, Wilmington, DE), weighing 2.46 to 3.47 kg on the day of irradiation, were randomized by the study biostatistician to one of three radiation arms [0 Gy (n = 6), 7.0 Gy (n = 19), 7.5 Gy, (n = 31)]. These doses were selected as they were estimated to result in 30–70% lethality by day 45 (e.g. LD30/45 and LD70/45) (Jackson et al. Citation2019). Prior to irradiation, animals were further randomized to pre-determined timepoints for scheduled euthanasia on days 5 (n = 3), 7 (n = 4), 10 (n = 3–5), 14 (n = 3–5), 20 (n = 3–5), or 45 (n = 3–9), relative to the day of irradiation (day 0), to avoid bias due to attrition over the time course to disease-progression. Three sham-irradiated animals were scheduled for euthanasia on study day 15 and three on study day 45 as an age- and sex-matched comparator arm.
Animals were anesthetized with ketamine (30–45 mg/kg) and xylazine (4.0–9.0 mg/kg) and placed in a 38 × 38 cm2 Vac-Loc™ positioning cushion with negligible attenuation to prevent movement out of the field during TBI. A 5 mm bolus was placed underneath the animal and a 8.5 mm beam spoiler placed above the animal to improve the dose homogeneity throughout the body of the rabbit (Poirier et al. Citation2019).
Anesthetized animals were exposed to a TBI dose of 7.0 or 7.5 Gy of 6-MV photons (0.83 ± 0.1 Gy/min) set to mid-depth of the animal abdomen as previously described (Jackson et al. Citation2019; Poirier et al. Citation2019). In-vivo dosimetry was carried out using Landauer® nanoDots™ (Landauer®, Glenwood Illinois) dosimeters, which are optically stimulated luminescent detectors (OSLDs). OSLDs were placed in pairs on the surface of both sides of the animal nears to the animal’s maximal width. Each OSLD pair was measured post-TBI. Average measurements for individual animals were within 5.5% of the prescribed dose with an OSLD measurement statistical uncertainty of ∼5%. Sham-irradiated controls were treated in the same way, except that the radiation source was not turned on.
Animals were followed for up to 45-days post-TBI. This included twice-daily cage-side and thrice weekly clinical exams (at a minimum) to evaluate changes in behavior, skin, hydration status, respiratory and heart rates, peripheral capillary oxygen saturation, rectal body temperature (RBT), and body weight. Animals were provided supportive care including pain medication [e.g. Buprenorphine, 0.01–0.05 mg/kg; twice per day (BID) on days 0–45, subcutaneous injection (SC)] and antibiotics [e.g. Enrofloxacin, 2.5–5mg/kg, once per day (QD) on days 1–45, SC]. Antipyretics (e.g. liquid acetaminophen, 160 mg/5 mL) and fluids were administered for RBT 104.8 °F. Animals meeting pre-defined criteria for major morbidity/imminent mortality were humanely euthanized prior to the pre-defined endpoint as previously described (Jackson et al. Citation2019). Briefly, animals were euthanized due to hemorrhage and/or severe acute anemia (e.g. red blood cell (RBC) count
3.0 × 106 cells/μL and/or hematocrit
15%) and/or fever >104.8 °F that was not resolved with antipyretics. Gross examination of vital organs was performed at necropsy, and tissue was collected, weighed, and processed for future microscopic evaluation by a board-certified veterinary pathologist.
Phlebotomy
Peripheral blood samples were collected prior to and post-TBI from the marginal ear vein per the schedule in to monitor changes in hematological parameters, biochemical markers, and whole blood thromboelastometry. Blood was also sampled at the time of unscheduled or scheduled euthanasia by cardiac puncture for measurement of routine laboratory-based coagulation tests and coagulation factors.
Table 1. Study schedule.
Complete blood count with five-part manual differential
EDTA-stabilized blood samples (e.g. 0.5 mL sample volume) were maintained at room temperature and analyzed for complete blood count by automated analysis using a Beckman Coulter Ac·T diff™ (Beckman Coulter, Inc., Miami, FL). A manual five-part differential was performed and peripheral blood films evaluated for cellular abnormalities.
Clinical chemistries to evaluate organ function
Whole blood (1–3.5 mL) was collected and placed into lithium heparin tubes for serum chemistry analysis. Samples were analyzed using a Diasys respons®910VET automated clinically chemistry analyzer (DiaSys Diagnostic Systems GmbH, Holzheim, Germany). Tests included a lipid panel (e.g. cholesterol, triglycerides), renal function tests (e.g. blood urea nitrogen, calcium, chloride, creatinine, glucose, phosphorus, potassium, sodium), liver function tests [e.g. total protein, albumin, total bilirubin, alanine transaminase (ALT), aspartate transaminase (AST), alkaline phosphatase (ALP), gamma-glutamyl transpeptidase (GGTP), globulin, albumin/globulin ratio], and magnesium, chloride, amylase, and creatinine phosphokinase (CPK).
Thromboelastometry
Whole blood was processed for thromboelastometry as previously described (Jackson et al. Citation2019). Briefly, blood samples were maintained at room temperature and run within two hours of collection. Prior to analysis, blood samples were warmed to 37° C for 10 minutes and mixed thoroughly. A 300 μL aliquot of citrated whole blood was tested on a Rotational Thromboelastometry whole blood coagulation analyzer (ROTEM®delta Thromboleastometry, TEM Systems, Inc. Research Triangle Park, NC) in NATEM (e.g. non-activated) test mode. A correction formula for the adjustment of the citrate anticoagulant volume was used to compensate for a lower than normal hematocrit values and a calculated volume of citrate was added to the collection tube. In NATEM test mode, the citrated blood sample was re-calcified with the ROTEM system reagent star-TEM. Forty (40) microliters of star-TEM reagent was added by automated electronic pipette to a ROTEM disposable test cup followed by 300 μl of the citrated whole blood. The program requires the test solution to be drawn into the automated electronic pipette tip for mixing. The test solution (sample plus star-TEM) was then placed on the measuring head for a 60-minute analysis. The proper functioning of the ROTEM was confirmed weekly with quality control standards (TEM Systems, Inc. Research Triangle Park, NC).
Coagulation factor tests
Citrate-stabilized blood samples were collected by mixing nine parts of fresh blood with one part of 3.2% (0.11 mol/L) sodium citrate anticoagulant and gently mixing by inversion three to four times. Samples were frozen at −70 °C or below until analysis. Tests for activated partial thromboplastin time, prothrombin, fibrinogen, antithrombin, individual coagulation factors (FII, FV, FVII, FX, FVIII, FIX, FXI, FXII) were performed on a BCS XP System (Siemens HealthCare, Newark, DE), a fully automated hemostasis analyzer. Lyophilized preparation of pooled normal plasma stabilized with HEPES buffer solution (12 g/L) was provided by Siemens HealthCare (Newark, DE) for quality control (normal and pathological) tests.
Statistical analysis
Data are summarized using descriptive statistics (e.g. mean, standard error mean) at each time point by radiation dose. Group means were analyzed by one-way analysis of variance (ANOVA) with Tukey’s multiple comparisons test to determine statistically significant differences between time points. All statistical analysis was performed at alpha = 0.05.
Results
Mortality
Five of 19 animals exposed to 7.0 Gy TBI and 10 of 31 animals exposed to 7.5 Gy were euthanized due to criteria or expired prior to the pre-determined study endpoint. One animal was found expired, and blood samples were not collected. At the time of unscheduled euthanasia, 12 out of 14 animals presented with anemia (hemoglobin <10.0 g/dL), which was severe in 10 out of the 14 (hemoglobin < 5 g/dL, hematocrit < 15% or RBC < 3.0 × 106 cells/μL). Nine of the 10 animals presenting with severe, acute anemia were also thrombocytopenic with an average platelet count of 5000 cells/μL. One animal presented with a low RBC count (2.85 × 106 cells/μL) and very low iron (29.9 μg/dL), but without severe thrombocytopenia (PLT = 57,000/μL) at euthanasia. All animals were neutropenic at the time of euthanasia due to criteria, except for one animal euthanized on day 1 post-TBI due to seizures and high fever (e.g. RBT 107–108 °F). At that time, all hematological parameters were normal, except for lymphocytes, which were depleted (0.230 × 103 cells/μL). All animals were lymphopenic at the time of euthanasia due to criteria.
Clinical signs and symptoms of ARS after TBI in the New Zealand white rabbit
The prodromal phase of ARS was notably absent amongst all animals. Rabbits are incapable of vomiting due their gastrointestinal physiology, and therefore, this was not an observed feature. None of the animals on study presented with diarrhea; however, stool production was observed to be consistently decreased amongst the majority of animals on days 2 to 5. Decreased stool production had an earlier onset (day 2 vs. 3) and longer duration (2 vs. 5 days) following exposure to 7.5 Gy as compared to 7.0 Gy. In two animals irradiated at a dose of 7.0 Gy TBI, decreased stool production was observed on days 7 and/or 8; however, this did not appear to be predictive of outcomes.
Fur loss consistent with epilation/alopecia was observed starting as early as day 7 (). Visible petechiae on the skin were observed starting approximately seven days post-TBI and progressed to ecchymosis during the second week (). The presence of petechiae and ecchymosis coincided with severe thrombocytopenia during the same time-period and generally resolved by day 20 as platelet counts returned to normal. Petechiae and ecchymosis were not observed in animals culled on days 5 or 7 for scheduled euthanasia. Eleven animals developed fevers (RBT >104.8 °F). Fevers predominantly developed between days 12 and 20 post-TBI, although one animal had a high fever (e.g. 107.0−108.0 °F) on day 1. One animal presented with hypothermia (99.4 °F) on days 9–10. Fevers were treated with antipyretics (e.g. liquid acetominophen) and returned to normal between days 20–23 in three of the 11 animals. Five animals were treated with 17.5 to 25 mL of liquid acetaminophen, 160 mg per 5 mL. Animals scheduled for euthanasia on the same day due to criteria or pre-determined endpoint for scheduled euthanasia were not treated with antipyretics.
Table 2. Clinical data at the time of unscheduled euthanasia (7.0 Gy Arm).
Table 3. Clinical data at the time of unscheduled euthanasia (7.5 Gy Arm).
Animals began to develop severe hemorrhage (e.g. diffuse bleeding in internal organs with rapid loss in hematocrit) during the second week-post exposure at 7.0 and to a greater extent at 7.5 Gy, which resolved as platelet counts recovered (). Red blood cell loss and development of severe acute anemia during this time was attributed to extensive and uncontrollable bleeding, particularly at 7.5 Gy, based on observations of petechiae and ecchymosis, and internal hemorrhage at necropsy. There was no evidence of hemolytic anemia based on evaluation of peripheral blood smears and serum bilirubin, although this cannot be entirely ruled out (data not shown). By day 20, platelet counts had returned to normal and petechiae and ecchymosis had disappeared. Animals surviving through the second and third-weeks post-TBI remained alive throughout the rest of the follow-up period until study termination on day 45 post-exposure.
Longitudinal changes in hematology parameters after TBI in the New Zealand white rabbit
In animals exposed to 7.0–7.5 Gy TBI, lymphocyte depletion occurred as early as day 1 post-exposure (). A transient increase in the absolute neutrophil count (ANC) was observed on the first day post-exposure followed by a steep decline in ANC that reached an initial nadir on day 3 (). A transient recovery occurred between days 5 and 7, after which the ANC steadily fell until reaching a nadir approximately 14 days post-exposure. Platelet counts reached a nadir approximately 10-day post-exposure followed by recovery during the second- to third-week post-exposure among animals surviving through the thrombocytopenia phase (). Red blood cell counts and hematocrit rapidly declined during the second week post-exposure (). Neutrophil counts remained below baseline levels in three animals surviving to day 45 (e.g. one at 7.0 Gy and two at 7.5 Gy), although ANC remained >500 cells/μL (). Platelet counts frequently fell below 5000 cells/μL during the second week post-exposure (n = 13/19, 68%). Platelet and RBC count fully recovered by the third week and remained within the normal range through day 45 in those animals that were followed until endpoint for study termination. Five of 19 animals exposed to 7.0 Gy TBI were euthanized prior to the study endpoint due to criteria. In those animals, the RBC fell to 1.48, 2.02, 2.40, 3.19 and 3.90 × 106 cells/μL. Ten of 31 animals exposed to 7.5 Gy TBI were euthanized due to criteria or expired prior to the study endpoint. Of those animals, ten presented with RBC < 3.0 × 106 cells/μL.
Figure 1. Cellular kinetics of the hematopoietic-subsyndrome of acute radiation syndrome following a single dose of 7.0 or 7.5 Gy of 6-MV derived total body irradiation. Hematology parameters for all animals are represented on each graph. Data are censored due to culling of animals at scheduled timepoints, or for criteria.

Figure 2. Whole blood viscoelastic measurements following 7.0 or 7.5 Gy TBI in the New Zealand White rabbit. Top Panel. Representative TEMograms (ROTEM, TEM International, Munich, Germany) depict clotting kinetics at baseline and at the time of scheduled, or unscheduled euthanasia, across the time course to disease-progression. Bottom Panel. Rotational thromboelastometry (ROTEM, TEM International, Munich, Germany) was run on whole blood samples collected via cardiac puncture at the time of unscheduled euthanasia or scheduled euthanasia at pre-determined time points. Clotting time, clot formation time, alpha-angle, and the maximum clot firmness are shown. Sham-irradiated animals euthanized on day 15 or 45 were included as controls. The line represents the median value for the cohort. In instances where clots were not formed due to the blood being incoagulable, an arbitrary time of 2500 seconds was assigned.
Table 4. Clinical data at the time of scheduled euthanasia (7.0 Gy Arm).
Table 5. Clinical data at the time of scheduled euthanasia (7.5 Gy Arm).
The hemorrhagic syndrome and clotting abnormalities in the New Zealand white rabbit at the dose-range to induce the H-ARS
Petechiae appeared during the first week post-exposure and progressed to ecchymosis, coinciding with the temporal onset and duration of thrombocytopenia. During this time, the blood was noticeably thinned with a water-like appearance. Severe clotting abnormalities, defined by increased clotting time and clot formation time and decreased maximum clot firmness, appeared during the first or second-week post-exposure (), corresponding to the period of thrombocytopenia following exposure to 7.0 or 7.5 Gy TBI. Clotting kinetics returned to normal alongside platelet recovery and the disappearance of petechiae and ecchymosis. The onset of clotting dysfunction appeared earlier among animals exposed to 7.5 Gy TBI as compared to 7.0 Gy TBI, which is reflected in the more rapid and steeper decline in platelet counts over the same period. In some animals, the blood was incoagulable and clot formation time was not reported due to lack of clot formation.
Figure 3. Longitudinal changes in whole blood clotting kinetics across the time-course to disease progression following exposure to 7.0 or 7.5 Gy TBI in the New Zealand White rabbit model. Rotational thromboelastometry (ROTEM, TEM International, Munich, Germany) was run on whole blood samples collected from the marginal ear vein at scheduled timepoints post-exposure to 7.0 or 7.5 Gy TBI. Clotting time, clot formation time, alpha-angle, and the maximum clot firmness are shown. In instances where clots were not formed, an arbitrary time of 2500 seconds was assigned. In some cases, the maximum clot firmness and alpha-angle did not report due to lack of clot formation. Data are censored due to attrition over time.

Animals euthanized due to criteria generally presented with hemorrhage of the mediastinal lymph nodes, stomach, pancreas, kidneys, small and/or large intestine, and bladder, which ranged in severity across animals. Hemorrhage of the heart and lungs was compounded by cardiac puncture performed at the time of euthanasia to collect blood. Distention of the small and large intestines consistent with paralytic ileus was common. The medulla of the kidneys was often pale.One animal euthanized on the first day post-exposure due to seizures and elevated RBT (107 °F) presented with hemorrhage of the brain, pale and mottled liver, and pale cortex and congested medulla of the kidneys consistent with acute kidney injury. Gross morphological changes in the liver and kidneys were accompanied by elevated blood urea nitrogen (50.42-51.45 mg/dL), creatine protein (1.85 mg/dL), aspartate aminotransferase (259 U/L). The animal had normal neutrophil, red blood cell, and platelet counts.
Time-dependent changes in routine laboratory coagulation tests and individual tissue factors in the New Zealand white rabbit across the dose-range to induce the H-ARS
Prothrombin time (PT) appeared to be shortened in response to radiation (). Shortened activated partial thromboplastin times (aPTT) were observed during the first- to second-week post-exposure, but this did not achieve statistical significance (one-way ANOVA with Tukey’s multiple comparisons test). The shortened prothrombin time was observed at a higher rate among animals in the 7.0 Gy arm (11/19; 57.9%) than the 7.5 Gy arm (n = 9/31; 29%). A shortened thrombin time was observed during the second week post-exposure (e.g. 11−13.8 seconds and 13.4−13.5 seconds, unscheduled vs. scheduled) vs. 14.1−17.8 seconds. However, there was a marked difference in thrombin time between animals euthanized due to study endpoint (scheduled) vs. criteria (unscheduled) in the 7.5 Gy arm. Antithrombin was elevated in animals between days 5 and 20 regardless of TBI dose, with the most noticeable elevation occurring during the second-week post-exposure after which values returned to normal. One animal presented with antithrombin deficiency (37.2% of normal activity) on day 7 post-exposure to 7.0 Gy ().
Figure 4. Changes in markers of hemostasis following 7.0 or 7.5 Gy TBI in the rabbit. Data are grouped based on animals euthanized due to study endpoint on day 5 or day 7 (week 1), day -10 or day 14 (week 2), day 20 or 45 (after week 2), or at the time of unscheduled euthanasia due to criteria. These time points coincide with the progression of myelosuppression progressing to bone marrow failure or spontaneous recovery. Data are presented as mean ± SEM. *p <.05, **p < .01, ***p < .001, ****p < .0001 vs. sham-control.

Deficiency in Factor VIII activity was observed during the second week post-exposure in the 7.0 Gy TBI arm (). An increase in Factors VII and IX activity was observed among animals exposed to 7.5 Gy TBI. In these animals, elevated levels of Factor X activity, antithrombin activity and fibrinogen (e.g. common pathway) as well as shortened prothrombin time were also noted post-exposure (). Fibrinogen levels were within the normal range after week two consistent with the resolution of thrombocytopenia and disappearance of petechiae/ecchymosis in surviving animals (). Changes in tissue factors were noticeable during the first- and second-week post-exposure after which the majority of values returned to normal (). Factor X was markedly elevated during the second week post-exposure and considerably so amongst animals euthanized due to criteria (). The most inter-animal variability occurred in fibrinogen levels. Plasma fibrinogen levels were markedly elevated between days 5 and 14 post-exposure among animals exposed to 7.0 (n = 4/19; 21%) and 7.5 Gy (n = 10/31, 32%) TBI.
Figure 5. Individual coagulation factor tests measured at the time of scheduled euthanasia following 7.0 or 7.5 Gy TBI in the rabbit. Data are grouped based on animals euthanized due to study endpoint on day 5 or day 7 (week 1), day -10 or day 14 (week 2), day 20 or 45 (after week 2), or at the time of unscheduled euthanasia due to criteria. Data are presented as mean ± SEM. *p < .05, **p < .01, ***p < .001, ****p < .0001 vs. sham-control.

Discussion
The hemorrhagic syndrome is a leading cause of major morbidity and mortality in radiation accident victims presenting with ARS. While the majority of preclinical studies in animal models have focused on the cellular changes following radiation, few have focused on defining the natural history of the hemorrhagic syndrome. Descriptions of the hemorrhagic syndrome and contribution to mortality are primarily derived from the immediate survivors of the atomic bomb detonation at Hiroshima and Nagasaki (Liebow et al. Citation1947, Citation1949) and animal models in the 1940s-50s (Allen and Jacobson Citation1947; Cronkite Citation1950).
The current study was designed to elucidate the time-dependent changes in coagulopathy within the context of multiorgan injury associated with ARS. Animals were exposed to 7.0 or 7.5 Gy TBI and treated with minimal population-based supportive care measures including antibiotics, pain medication, and anti-pyretics. Animals were randomized to pre-determined timepoints for euthanasia prior to TBI. Time points were selected based on prior data and designed to coincide with the onset of thrombocytopenia (5–7 days), nadir (10–14 days), and recovery (20+ days) post-exposure. Blood was sampled post-exposure to monitor changes in hematology, serum chemistry, and viscoelasticity. Animals meeting the criteria for major morbidity/imminent mortality were euthanized prior to the pre-determined study endpoint. At the time of euthanasia, blood was sampled, and a gross exam was performed. Age- and sex-matched sham-irradiated animals were included as a comparator arm and euthanized at either study day 15 (n = 3) or study day 45 (n = 3).
The severity of coagulopathy and hemorrhage in animals was greater at 7.5 Gy than 7.0 Gy. This is consistent with previous data (Jackson et al. Citation2019), where coagulopathy and severe hemorrhage were observed in the context of multiorgan injury following exposure to TBI doses estimated to result in lethality for 50% or more of the population within the first three weeks post-exposure. At TBI doses expected to result in 30% or less lethality by day 45 (e.g. LD30/45), the dominant features leading to morbidity appeared to be pancytopenia and infection.
In the 7.5 Gy TBI arm, the first day of neutropenia (e.g. ANC < 500 cells/μL) occurred on study day 3–5 in the majority of animals. The exceptions were one animal who expired on day 1 due to high-grade fever and seizures, and a second who developed neutropenia starting on day 1. The onset of severe neutropenia (ANC < 100 cells/μL) occurred between days 10–12, although not all animals progressed to severe neutropenia. One animal had a precipitous decline in ANC with an onset of severe neutropenia on day 7 and was euthanized on day 12 with fever, thrombocytopenia, and severe acute anemia.
At the time of unscheduled euthanasia due to criteria, animals in the 7.5 Gy arm had significant elevation in plasma fibrinogen and antithrombin as well as factors VII, X, and IX. There did not appear to be a correlation between coagulation or tissue factor levels and visual signs and symptoms of ARS at the time of morbidity (e.g. fever, petechiae/ecchymosis, thrombocytopenia, anemia). All animal euthanasia for cause (e.g. major morbidity/imminent mortality) occurred during weeks one and two post-exposure. Differences in the rate of decline were not determined.
Taken together, the data suggest moribund animals enter a prothrombotic phase that fluctuations between hyper- and hypocoagulable states following TBI. The increase in tissue factors suggestion outcomes are not directly related to consumption coagulopathy.
The signs and symptoms of the hemorrhagic syndrome described in the rabbit model are consistent with those observed in the canine (Cronkite et al. Citation1950), swine (Moroni et al. Citation2011, Citation2013, Citation2014), goat (Cronkite Citation1950), and humans (Liebow et al. Citation1947, Citation1949). Across species, the onset of the hemorrhagic syndrome occurs approximately two to three weeks post-exposure to TBI with recovery by the fourth week. The exception appears to be the rodent model of TBI, where hemorrhage (e.g. profuse, uncontrollable bleeding) is not a dominant feature. In the murine model, while gross hemorrhage of vital organs may be observed at necropsy, the severity and extent are not as significant as that seen in other species and does not appear to play a predominant role in outcomes after TBI.
Under reporting of the hemorrhagic syndrome in the non-human primate in the literature has led to some misperception that uncontrollable bleeding is not a feature of ARS. More importantly, the majority of published data in the non-human primate model includes transfusions with whole blood and/or packed red blood cells based on individual triggers to treat. However, petechiae, ecchymosis, and profuse bleeding in the vital organs of the NHP following TBI with or without blood transfusions are routinely observed. Therefore, further studies are warranted to better understand the role of hemostasis dysfunction and hemorrhage in relation to morbidity/mortality in the non-human primate model of TBI-induced ARS to enable more accurate comparisons across species.
In conclusion, the current study was designed to describe the clinical pathology associated with the hemorrhagic syndrome and major morbidity/mortality. The pathogenesis of the hemorrhagic syndrome is likely complex and may involve vascular injury, sepsis, and/or thrombocytopenia. Future studies will be designed to probe the mechanisms underlying hemostatic dysfunction and hemorrhage to better elucidate the clinical picture of multiorgan injury associated with TBI-induced ARS.
Disclosure statement
The authors declare no conflicts of interest related to the data generated within this study.
Additional information
Funding
Notes on contributors
Isabel L. Jackson
Isabel L. Jackson is an Associate Professor, Radiation Biologist, and Deputy Director of the Division of Translational Radiation Sciences, Department of Radiation Oncology, at the University of Maryland School of Medicine. She is the Director of the division’s Medical Countermeasures program.
Ganga Gurung
Ganga Gurung was a Research Associate within the Division of Translational Radiation Sciences, Department of Radiation Oncology, at the University of Maryland School of Medicine. She is now practicing medicine in the United Kingdom.
Emmanuel Ayompe
Emmanuel Ayompe was a Research Assistant within the Division of Translational Radiation Sciences, Department of Radiation Oncology, at the University of Maryland School of Medicine.
Elena-Rose Fown
Elena Rose-Fown is a Project Coordinator within the Division of Translational Radiation Sciences, Department of Radiation Oncology, at the University of Maryland School of Medicine.
Sarah Triesler
Sarah Triesler is a Veterinary Research Specialist within the Division of Translational Radiation Sciences, Department of Radiation Oncology, at the University of Maryland School of Medicine.
Buddha Mali
Buddha Mali is a Lead Veterinary Research Specialist within the Division of Translational Radiation Sciences, Department of Radiation Oncology, at the University of Maryland School of Medicine.
Andrea Casildo
Andrea Casildo was a Veterinary Research Specialist within the Division of Translational Radiation Sciences, Department of Radiation Oncology, at the University of Maryland School of Medicine.
Allison Gibbs
Allison Gibbs is a Research Laboratory Manager within the Division of Translational Radiation Sciences, Department of Radiation Oncology, at the University of Maryland School of Medicine.
Yannick Poirier
Yannick Poirier is an Assistant Professor and board-certified clinical medical physicist in the Department of Radiation Oncology, Division of Medical Physics and Division of Translational Radiation Sciences at the University of Maryland School of Medicine. He oversees the dosimetry and physics of all radiation biological studies of the Division of Translational Radiation Sciences.
Eric P. Cohen
Eric P. Cohen is a Professor of Medicine within the University of Medicine School of Medicine.
Diana Newman
Diana Newman is a Program Director within the Division of Translational Radiation Sciences, Department of Radiation Oncology, University of Medicine School of Medicine.
Zeljko Vujaskovic
Zeljko Vujaskovic is a Professor of Radiation Oncology and Director of the Division of Translational Radiation Sciences, Department of Radiation Oncology, University of Medicine School of Medicine.
References
- Allen JG, Jacobson LO. 1947. Hyperheparinemia: Cause of the hemorrhagic syndrome associated with total body exposure to ionizing radiation. Science. 105(2728):388–389.
- Cronkite EP. 1950. The hemorrhagic syndrome of acute ionizing radiation illness produced in goats and swine by exposure to the atomic bomb at Bikini, 1946. Blood. 5(1):32–45.
- Cronkite EP, Bond VP, Conard RA. 1995. Medical effects of exposure of human beings to fallout radiation from a thermonuclear explosion. Stem Cells. 13(Suppl 1):49–57.
- Cronkite EP, Halpern B, Jackson DP, Le RG. 1950. A study of the hemorrhagic state in dogs after a lethal dose of two million volt X-rays. J Lab Clin Med. 36(5):814.
- Cronkite EP, Jacobs GJ, Brecher G, Dillard G. 1952. The hemorrhagic phase of the acute radiation syndrome due to exposure of the whole body to penetrating ionizing radiation. Am J Roentgenol Radium Ther Nucl Med. 67(5):796–804.
- Jackson DP, Cronkite EP, Jacobs GJ, Behrens CF. 1952. Prothrombin utilization in radiation injury. Am J Physiol. 169(1):208–217.
- Jackson IL, Gurung G, Poirier Y, Gopalakrishnan M, Cohen EP, Shea Donohue T, Cohen MJ, Newman D, Vujaskovic Z. 2019. A New Zealand White rabbit model of thrombocytopenia and coagulopathy following total body irradiation across the dose range to induce the hematopoietic-subsyndrome of acute radiation syndrome. Rev Int J Radiat Biol. 1–36. DOI:https://doi.org/10.1080/09553002.2019.1668981
- Liebow AA, Warren S. 1947. Injuries produced by the atomic bomb: observations on human beings at Hiroshima and Nagasaki. Am J Pathol. 23(5):888–891.
- Liebow AA, Warren S. 1947. Early effects of radiation. Am J Pathol. 23(5):888.
- Liebow AA, Warren S, De CE. 1949. Pathology of atomic bomb casualties. Am J Pathol. 25(5):853–1027.
- Moroni M, Coolbaugh TV, Lombardini E, Mitchell JM, Moccia KD, Shelton LJ, Nagy V, Whitnall MH. 2011. Hematopoietic radiation syndrome in the Gottingen minipig. Radiat Res. 176(1):89–101.
- Moroni M, Elliott TB, Deutz NE, Olsen CH, Owens R, Christensen C, Lombardini ED, Whitnall MH. 2014. Accelerated hematopoietic syndrome after radiation doses bridging hematopoietic (H-ARS) and gastrointestinal (GI-ARS) acute radiation syndrome: early hematological changes and systemic inflammatory response syndrome in minipig. Int J Radiat Biol. 90(5):363–372.
- Moroni M, Ngudiankama BF, Christensen C, Olsen CH, Owens R, Lombardini ED, Holt RK, Whitnall MH. 2013. The Gottingen minipig is a model of the hematopoietic acute radiation syndrome: G-colony stimulating factor stimulates hematopoiesis and enhances survival from lethal total-body γ-irradiation. Int J Radiat Oncol Biol Phys. 86(5):986–992.
- Poirier Y, Prado C, Prado K, Draeger E, Jackson IL, Vujaskovic Z. 2019. Use of CT simulation and 3-D radiation therapy treatment planning system to develop and validate a total-body irradiation technique for the New Zealand White rabbit Manuscript under review at International Journal Radiation Biology.(BARDA RadNuc Special Issue).
- Stohlman F, Jr., Brecher G, Schneiderman M, Cronkite EP. 1957. The hemolytic effect of ionizing radiations and its relationship to the hemorrhagic phase of radiation injury. Blood. 12(12):1061–1085.