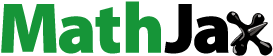
Abstract
Purpose
Well-characterized animal models that mimic the human response to potentially lethal doses of radiation are necessary in order to assess the efficacy of candidate medical countermeasures under the criteria of the U.S. Food and Drug Administration ‘Animal Rule’. Development of a model requires the determination of the radiation dose response relationship and time course of mortality and morbidity under scenarios likely to be present in the human population during mass casualty situations. These scenarios include understanding the impact of medical management on survival of the hematopoietic acute radiation syndrome (H-ARS). Little information is available to compare the impact of medical management under identical study conditions. The work presented here provides a comparison of the impact of different levels of medical management (supportive care) on the survival outcome in two large animal models: the male Gottingen minipig and the male rhesus macaque (NHP).
Materials and Methods
In the context of this comparison, limited supportive care consisted of administration of analgesics only, standard supportive care consisted of prophylactic administration of analgesics, antibiotics and fluids (minipigs) or analgesics, antibiotics, antidiarrheals, nutritional and fluid support (NHP) on a set schedule regardless of indication, and full supportive care (NHP only) consisted of analgesics, antibiotics, antidiarrheals, nutritional and fluid support, antiemetics and blood transfusions on an individual animal, trigger-to-treat regimen. Regardless of level of supportive care, minipigs were exposed to total body irradiation using a Co60 source and NHPs were exposed to total body irradiation using 6 MV photon energy.
Results
Based on estimated LD50 values, the inclusion of antimicrobial or broad-spectrum antibiotics provided a dose modifying factor (DMF) of 1.09 in the minipig, and by 1.15 in the NHP (standard supportive care to limited supportive care ratio. For the NHP, the administration of supportive care based on symptomology rather than a set schedule, and inclusion of blood transfusions yielded a DMF of 1.05 (full supportive care to standard supportive care ratio). Conversely, comparison of the estimated LD50 values between full supportive care and limited supportive care in the NHP provided a DMF of 1.21.
Conclusion
The study reported here provides a comparison of the impact of antibiotic administration on radiation-induced lethality.
Introduction
Concerns over nuclear and radiological accidents and potential acts of terrorism have driven efforts to improve methods to protect military personnel, the general population, first responders and other service groups from the health hazards associated with exposure to ionizing radiation. Much effort has focused on the development of medical countermeasures for treatment of acute radiation-induced injuries. Whole-body, or significant partial body radiation exposure targets radiosensitive cells, including circulating lymphocytes, bone marrow, and intestinal crypt cells (Brook and Ledney Citation1991; Dainiak Citation2002). Commonly, infection resulting from a breach in the intestinal mucosal wall coupled with severe neutropenia leads to a high rate of mortality. The use of broad-spectrum antibiotics combined with clinical support has been shown to increase survival of acutely irradiated dogs by 50% during the first 60 days postirradiation (Augustine et al. Citation2005). Thus, controlling any infection during the critical neutropenic phase is a major factor for successful outcome.
It has long been recognized that medical management alone can significantly improve the likelihood of surviving exposure to high doses of ionizing radiation, and the prevention of infection is a mainstay of therapy of irradiation victims (Brook et al. Citation2004). Recommendations on medical management of acute radiation syndrome include the administration of antimicrobial agents, antiemetic agents, antidiarrheal agents, fluids, electrolytes, analgesic agents and topical burn creams (Waselenko et al. Citation2004). A report by Brook et al. (Citation2005) suggests that postirradiation antibiotic therapy should include antibiotics directed against Gram-negative aerobic organisms and coverage against aerobic and facultative Gram-positive bacteria. Additionally, recommendations include initiating administration of antibiotic agents within 48 hours of exposure and continuing for a minimum of 7 days, or as long as 21–28 days until the risk of infection has declined as the bone marrow has recovered.
As part of the effort to develop and characterize appropriate animal models to facilitate the advanced development of medical countermeasures for acute radiation sickness under the U.S. Food and Drug Administration (FDA) ‘Animal Rule’, the relationship between supportive care and lethality must be established. Several laboratories investigating radiation-induced injury have developed supportive care protocols for lethally irradiated animals, ranging from provision of acidified water in mouse studies to cytokine therapy and blood transfusions in nonhuman primate studies (e.g. MacVittie et al. Citation1991; Moccia et al. Citation2010; Farese et al. Citation2012). The studies presented here provide side-by-side comparisons of the impact of various levels of supportive care on resulting lethality curves in two large animal models of total body irradiation: the male Gottingen minipig and the male rhesus macaque.
Materials and methods
Animals
Minipigs
Male Gottingen minipigs, approximately 4–6 months of age, were obtained from Marshall BioResources (North Rose, NY); animals weighed approximately 8 to 13 kg at the time of irradiation. Animals were pair housed when possible in galvanized steel modular floor pens measuring 1.5 m3. Pens were attached in units, allowing animals to see and touch neighboring animals. Autoclaved aspen wood shavings were provided for bedding and were changed at least daily. Individual water lines with lixit valves were secured to pen walls. A variety of veterinarian approved toys were provided for enrichment. Animals were fed Lab Diet K599 Certified Minipig Grower/Maintenance diet (PMI Nutrition International, LLC, Brentwood, MO) twice daily (BID) in a ration according to age. Certified fruit crunchies (Bio-Serv, Frenchtown, NJ), locally procured apples, and miniature marshmallows were used for positive reinforcement. The light cycle was 12-h light and 12-h dark and temperature and humidity were as recommended for minipigs provided bedding: 18–22 °C and 35–65%, respectively. Animals were allowed to acclimate to the facility for at least one week prior to vascular access port (VAP) implantation; clicker training was initiated during acclimation and continued post VAP implantation surgery until study start. Temperature and identification transponders were implanted subcutaneously (BMDS IPTT-300, Seaford, DE) at VAP implant. Animal protocols were approved by the Institutional Animal Care and Use Committee (IACUC) at the Pacific Northwest National Laboratory. The facility was accredited by the Association for Assessment and Accreditation of Laboratory Animal Care International (AAALAC), registered with the USDA, and held an Office of Laboratory Animal Welfare (OLAW) assurance.
Non-human primates
Three separate studies were conducted with a total of 110 male rhesus macaque (Macaca mulatta) of Chinese origin; body weights ranged from 3.2 to 8.7 kg at the time of irradiation. A separate cohort of rhesus (approximately 4–11 kg) served as non-terminal blood donors for the full supportive care study. An automatic lighting system provided a 12-h light, 12-h dark cycle and temperature and humidity were maintained at 18–29 °C and 35–70%, respectively. Animals were housed in individual stainless-steel cages and were provided commercial, certified primate biscuits twice daily in a ration appropriate for the species. Biscuits were supplemented with fresh produce (i.e. bananas, apples, cucumbers, grapes, pears) and primate treats. Water was provided ad libitum. Animals were allowed to acclimate to the facility for a minimum of 3 weeks prior to irradiation. All animal protocols were approved by the IACUC at SNBL USA, Ltd. The facility is accredited by AAALAC, is registered with the USDA, and holds an OLAW assurance.
Vascular access port implantation
Minipigs
Vascular access port (VAP) implant procedures were adapted from a technique described by Moroni et al. (Citation2011c). Briefly, minipigs were anesthetized with ketamine (33 mg kg−1 subcutaneous (SC)) and acepromazine (1.1 mg kg−1 SC), then intubated and maintained on isoflurane (1.5-3%) anesthesia. Venous access was established with a 22-gauge intravenous catheter placed in the lateral saphenous or auricular vein. Pre-operatively, all minipigs received a single dose of ampicillin (6 mg kg−1 intravenous (IV)) and buprenorphine (0.05 mg kg−1 intramuscular (IM)). Intra-operatively, all minipigs received lactated ringers solution at a rate of approximately 5–10 ml kg−1 h−1 through the intravenous catheter. Strict aseptic technique was utilized throughout the surgical procedure.
Titanium Soloport MID Vascular Access Ports with a 5 or 7 French polyurethane catheter with rounded tip (Instech Laboratories, Plymouth Meeting PA) were utilized for all implants. A 5-cm curvilinear incision was made in the right dorsolateral neck just cranial to the scapula. A pocket just larger than the port was created between the skin and underlying musculature using blunt dissection. A 3–4 cm incision was made over the right jugular groove and the right external jugular vein was isolated and secured with elastic vessel loops. The catheter and port were flushed with heparinized saline (100 U mL−1). A small incision was made into the external jugular vein and the catheter was inserted into the vessel and advanced approximately 8 cm. One anchoring bead was placed inside the vessel and the catheter secured within the vessel using multiple ligatures of 3-0 polypropylene suture (Securos, Fiskdale, MD). Patency of the catheter was confirmed by unrestricted aspiration of a blood sample. Once the catheter was secured within the vessel, a curved carmalt was utilized to tunnel the catheter from the insertion site to the port site, where the catheter was connected to the port and the port was sutured to the underlying musculature using 3-0 polypropylene suture. Both incision sites were infiltrated with bupivacaine (2 mg kg−1 SC) to provide additional analgesia. The pocket and jugular insertion sites were then closed in two and three layers, respectively, with 3-0 polydioxanone suture (Vedco, Saint Joseph MO). Tissue glue (Vetbond®, 3 M Animal Care Products, St. Paul MN) was applied to both incisions. The patency of the system was confirmed at the conclusion of the procedure.
Post-operatively, all minipigs received buprenorphine (0.05 mg kg−1 SC) and carprofen (2–3 mg kg−1 orally (PO)) BID for three days, and sulfamethoxazole-trimethoprim (60 mg PO) once per day (SID) for five days. All minipigs were allowed to recover for a minimum of three weeks post implantation prior to irradiation. VAPs were flushed at least once per week utilizing strict aseptic technique with approximately 6–8 mL of sterile 0.9% sodium chloride, and locked with approximately 1 mL of sterile heparinized saline (100 U ml−1).
Supportive medical management
Minipig standard supportive care
Minipig standard supportive care was defined as antibiotics and fluids administered in a daily routine to all animals regardless of indication. All animals received prophylactic antibiotic treatment and fluid support within 72 h of irradiation and administration continued through post-irradiation Day 30. Based on current recommendations for the management of post-irradiation infection, two antibiotics were administered – a broad-spectrum antimicrobial agent (Amoxicillin suspension 10 mg kg−1 PO, BID) and an aminoglycoside antibiotic (soluble gentamicin 2 mg kg−1 PO, SID) to cover Gram-negative infections. Fluids were provided during this same time interval to help alleviate any potential dehydration: approximately 240 mL of water was added to regular food rations both morning and afternoon and the food allowed to soften for approximately 10 min. The softened food was readily consumed by the animals. Oral carprofen (2–3 mg kg−1) was administered BID beginning at post irradiation Day 1 and continued through Day 30.
Minipig minimal support care
Minipig limited supportive care was defined as the administration of analgesic support only. Oral carprofen (2–3 mg kg−1) was administered BID beginning at postirradiation Day 1 and continued through Day 30.
NHP full supportive care
The NHP full supportive care regimen has been described previously (Thrall et al. Citation2015) and included analgesics, nutritional and fluid supplementation, antiulceratives support, antidiarrheal support, antipyretic support, whole blood transfusions, and antiemetic support administered on an individual animal basis based on symptomology.
NHP standard supportive care
Tramadol (1–4 mg kg−1 PO) was administered BID beginning post irradiation day 5 and continued to day 30. Animals refusing oral administration of tramadol for two consecutive treatments received buprenorphine (0.01 mg kg−1) by IM injection BID for the duration of the treatment period. Animals demonstrating pain or distress not relieved by tramadol or buprenorphine at the 0.01 mg kg−1 dose level received buprenorphine at a dose of 0.02 mg kg−1 IM BID.
Nutritional support for all animals included fresh produce daily (excluding citrus). Softened primate diet (e.g. Softies, other BioServ certified food supplements), approximately 50 g were provided SID on Days 5 to 30 in addition to regular biscuits. Gatorade ice cubes (2) prepared diluted 1:1 with tap water, were provided to all animals BID on Days 5 to 30.
All animals were provided oral antidiarrheal (Loperamide HCl) in the form of a tablet (approximately ½ of a 2 mg tablet) beginning on Day 3 and continued through Day 30.
All animals were provided oral antibiotic (enrofloxacin, 5 mg kg−1) SID postirradiation Days 5 through 30. Animals that refused oral administration for two consecutive treatments were treated by IM injection (5 mg kg−1) for the duration of the treatment period.
NHP minimal supportive care
Oral tramadol (1–4 mg kg−1 PO) or buprenorphine (IM, 0.01 mg kg−1) was administered BID beginning post irradiation day 2 and continued through day 30. Animals refusing oral administration of tramadol for two consecutive treatments received buprenorphine (0.01 mg kg−1) by IM injection BID for the duration of the treatment period. Animals demonstrating pain or distress not relieved by tramadol or buprenorphine at the 0.01 mg kg−1 dose level received buprenorphine at a dose of 0.02 mg kg−1 IM BID.
Nutritional support for all animals included providing fresh produce daily (excluding citrus) throughout the study. Softened primate diet (e.g. Softies, other BioServ certified food supplements), approximately 50 g were provided SID on Days 5 to 30 in addition to regular biscuits. Gatorade ice cubes (2) prepared diluted 1:1 with tap water, were provided to all animals BID on Days 5 to 30.
Irradiation
Minipigs
Radiation exposure and dosimetry conditions followed recommendations set forth in Report 30 of the International Commission on Radiation Units and Measurements (Citation1979). In brief, anesthetized animals were irradiated using a nominal 247,900 GBq 60Co source. The source was moved into place using a pneumatic system to a position 170-cm above the floor within a collimator that provided an approximate 30-degree solid angle beam. Prior to irradiating animals, the correlations between internal center-line dose measurements using LiF:Mg:Ti ‘chipstrate’ dosimeters in a euthanized animals and external exposure using a Capintec ionization chamber were established. Two separate dosimetric methods were used so results could be compared and uncertainties in the accuracy of the measured doses known to a high degree. Chipstrate dosimeters were calibrated in a National Institute of Standards and Technology (NIST)-traceable 60Co beam using tissue-equivalent plastic for buildup to accommodate a reference to absorbed dose to tissue.
Exposure conditions were established to provide a known absorbed dose-rate at the geometric center of a euthanized animal representative of the study population. Calibrated dosimeters were placed at 14 locations at different depths along the length of the animal. The average width of each minipig determined at multiple locations and length were measured immediately prior to irradiation and area calculated. Using this minipig area correlation with average depth, as opposed to using correlation with pig weight, was determined to result in better dose reproducibility. For irradiation, animals were anesthetized with ketamine (33 mg kg−1) and acepromazine (1.1 mg kg−1) by IM injection and restrained in a hammock-style sling with legs positioned under the animal and placed on a remotely operated rotating platform turntable. Whole body gamma-irradiation doses ranged from 1.5 to 2.5 Gy at a dose rate of 0.8 Gy min−1.
Non-human Primates. Radiation exposure and dosimetry conditions were established by a Certified Medical Physicist using a 6 MV linear accelerator and were consistent across all three studies. In brief, food was removed from the animals overnight prior to the day of exposure. For irradiation, animals were administered an antiemetic (1.0 mg mL−1 ondansetron, Hospira, Inc., Lake Forest IL) by intramuscular (IM) injection, sedated with ketamine (Ketaset®, Fort Dodge IA)/xylazine (AnaSed®, Lloyd Laboratories, Shenandoah IA; 0.10-0.15 mL kg−1 of a solution containing 95 mg mL−1 ketamine and 4.8 mg mL−1 xylazine) and transferred to the linear accelerator. Sedated animals were positioned and restrained in a patented procedure restraint cage (RYO-V, SNBL Japan) in an upright position and placed at 230 cm source-to-axis distance from the beam with an acrylic plate between the animal and the beam entry to serve as a beam spoiler. Irradiation parameters were established to expose animals to TBI at the prescribed dose using the linear accelerator to the midline at the xiphoid process. Animals were exposed bilaterally in an anterior-posterior (AP) and posterior-anterior (PA) technique by the 6 MV linear accelerator photon beam with approximately 50% contribution from both the AP and PA beams. A nominal dose rate of 0.8 Gy min−1 was delivered using the formula:
where MU is the machine monitor unit setting; dose is the desired delivered dose (in cGy), TMR is the tissue maximum ratio, based on the effective field size and calculation depth; Output at 230 cm is the radiation output (cGy) measured using the virtual water phantom at 230 cm with the procedure cage and the acrylic plate; and Normalization Factor is the linear accelerator output measured on the day of irradiation. The day of irradiation was designated as Day 0. Animals were observed via in-room cameras throughout the entire procedure. In vivo dosimetry (IVD, Sun Nuclear) was used for real time assessment of the delivered dose.
In-life procedures
Minipigs
Blood was collected from the implanted VAP or via the cephalic or cranial epigastric veins for assessment of hematological (daily) and clinical chemistry (weekly) parameters. For blood collection using peripheral veins, animals were sedated using midazolam (0.1–0.5 mg kg−1) by SC or IM injection. A pilot study was conducted to evaluate any variations in measured hematological values when collected serially from the VAP or peripheral veins, with and without midazolam sedation. Although matched collections showed expected fluctuations, no consistent differences or trends were observed between intra-animal matched samples (data not shown). Animals were observed twice daily and cageside clinical observations recorded. Body weights were obtained at least three times per week, and temperature was measured daily via the implanted transponder.
Non-human primates
Regardless of the supportive care regimen, cageside clinical observations were performed twice daily beginning on post-irradiation day 1. Animals were graded on the following: level of activity (normal, decreased, non-responsive), posture (normal, hunched, recumbent), stool consistency (normal, soft, loose and/or liquid, hard, or none present), appearance of blood in stool (blood observed, none present), emesis (none, present, profuse), blood in cage (none, spotting, pooling), respiration (normal, increased, labored), and alopecia (normal, slight, moderate, extreme, complete) and convulsions (observed, none present). Body weights, rectal temperatures, hematology and serum chemistry analyses were conducted as necessary to evaluate animal condition.
Euthanasia criteria
Minipigs
Clearly defined moribund criteria were established and consistently followed for euthanasia prior to study end. Criteria included recumbency, non-responsiveness to stimuli, dyspnea or labored breathing, and weight loss >20% from baseline (day of irradiation). In general, animals meeting euthanasia criteria displayed a collection of signs. At the completion of the study, or for moribund condition, animals were anesthetized with ketamine (33 mg kg−1) and acepromazine (1.1 mg kg−1) by SC injection and euthanized by IV administration of a pentobarbital overdose.
Non-human primates
Strictly defined euthanasia criteria were defined by SOP to avoid decision bias. Absolute euthanasia criteria included any single one of the following observations: indication of unrelieved pain or distress following administration of two consecutive increased doses of buprenorphine (0.02 mg kg−1 IM BID); inactivity (e.g. recumbent in the cage for at least 15 minutes or non-responsive to touch); respiratory distress (e.g. open mouth breathing, cyanotic appearance, labored breathing); uncontrolled hemorrhage; severe dehydration as evidenced by a skin tent time >3 s, sunken eyes, rapid and weak pulse, cold extremities, and/or comatose and non-responsive to treatment (full supportive care regimen). Non-absolute euthanasia criteria included any combination of two or more of the following observations: loss of body weight ≥ 25% of baseline (most recent pre-irradiation body weight) for two consecutive days; observations of severe injury or condition (e.g. non-healing wound); hyperthermia (rectal temperature ≥41 °C); hypothermia (rectal temperature ≤35 °C); complete anorexia for 48 hrs. Moribund animals and surviving animals at study end were sedated with ketamine/xylazine and euthanized by IV administration of a pentobarbital overdose.
Statistical methods
Average values for hematological parameters were calculated for each exposure group for each sampling time point; average and standard deviations are reported when at least 3 points are available. Ranges or single values are reported for sampling time points with less than 3 data points. Statistically significant differences between group means at individual time points were determined by one-way ANOVA (p < .05) and Tukey’s HSD Post-Hoc test as appropriate. Lethality was estimated based on the method of Miller and Tainter (Citation1944) using a probit transformation of percent mortality.
Results
All minipigs recovered well from VAP implantation surgery and all incision sites healed without complication. In total, 83% of the animals had fully functional VAPs for the entire study duration; devices that failed did so at various time points throughout the study. At necropsy, it was determined that all device failures involved five French catheters that became kinked which resulted in the inability to withdraw a blood sample. Gross evaluation of the implantation sites for all animals revealed dense tissue encapsulation of the port without evidence of infection or inflammation at either site.
Gottingen minipigs were irradiated using a Co60 source. Passive dosimetry and ionization chamber measurements showed a dose rate ranging from 76 to 82 cGy min−1. The uncertainty associated with the delivered free-field dose rate is 1.5% at the 95% confidence level, and the resulting uncertainty in the calculated bi-directional dose to tissue was estimated to be 2.4% at the 95% confidence level. The NHPs were irradiated with the 6 MV photon beam using the linear accelerator. Linac output was measured each day of irradiation using a calibrated ionization chamber in solid water and output was used to prescribe dose. The percent variation in the midline tissue dose (both AP and PA) from the target dose ranged from −3.8 to +2.6% (full supportive care), −4.9 to −1.8% (standard supportive care) and 0.0 to +2.7% (minimal supportive care). Passive dosimeter and ionization chamber measurements conducted using a phantom showed an estimated absorbed dose rate of 78 to 82 cGy min−1 at the midline of the animal.
An overview of the different regimens of supportive care is provided in . Specific supportive care treatments provided to NHPs under full supportive care have been described previously (Thrall et al. Citation2015). Regardless of provision for supportive care, all irradiated animals displayed characteristic hematological responses, including neutropenia, thrombocytopenia, and anemia, as illustrated in for minipig and NHP, respectively. Although time to nadir differs slightly between species, post irradiation changes in hematological components were similar to that described previously for large animals (Moroni et al. Citation2011a; Farese et al. Citation2012).
Figure 1. Average hematology values over time from post-irradiation day 0 to 45 for male Gottingen minipigs administered standard supportive care and exposed to total body irradiation by Co60 at doses of 1.78, 1.96, 2.15 and 2.30 Gy; (a) red blood cells; (b) platelets; (c) absolute neutrophil count; and d) absolute lymphocyte count.
Figure 2. Average hematology values over time from post-irradiation day 0 to 60 for male rhesus macaques administered full supportive care and exposed to total body irradiation by 6 MV photon linear accelerator at doses of 6.25, 6.75, 7.25, 7.75, 8.25 and 8.75 Gy; (a) red blood cells; (b) platelets; (c) absolute neutrophil count; and (d) absolute lymphocyte count.
Table 1. Comparison of supportive care regimens (full, standard, limited) for minipigs and NHPs. Specific details on supportive care measures is provided in the Materials and Methods section.
Clearly defined moribund criteria were established and consistently followed for euthanasia prior to study end for both NHP and minipig studies. Regardless of irradiation dose level or supportive care, all minipigs presented a steady weight gain for the duration of the study. Anorexia, when present, occurred 24 to 36 hr prior to meeting other absolute indications for moribund criteria regardless of whether animals were provided moistened food. Conversely, body weight in the irradiated NHP decreased beginning by approximately postirradiation Day 4, regardless of level supportive care provided (data not shown).
The primary endpoint for evaluating the impact of supportive care on the dose response relationship is survival. In general, higher doses of irradiation resulted in greater body weight decrease over the course of the study. Typically, animals succumb to the hematopoietic component of acute radiation sickness between post irradiation days 12 and 25, with few deaths occurring after Day 30 (Moroni et al. Citation2011b; Farese et al. Citation2012). With few exceptions, this was similarly observed for both species of animals evaluated in this study, regardless of level of supportive care. As shown in , survival for all animals decreased with increase in irradiation dose, regardless of the level supportive care.
Table 2. Study enrollment and survival outcome for each species and by level of supportive care.
For the minipig, administration of antibiotics and fluids (standard supportive care) resulted in a non-linear increase in survival (; ). At the high end of the lethality profile, overall survival was 25% for the supportive care group of animals exposed to 2.3 Gy versus 13% survival for animals receiving no supportive care and exposed at the same dose (). Differences in survival due to provision of supportive care were more pronounced at the LD50 exposure level and below. The calculated LD50 values for total body irradiated Gottingen minipigs were determined to be 2.14 with inclusion of standard supportive care and 1.96 Gy for limited supportive care. In total, the dose response relationship for animals provided antibiotics and fluids (standard supportive care) shifted to the right compared to that for limited supportive care (). In either case, the slope of the dose–response relationship is steep, with a dose differential between 10% and 90% mortality less than 1 Gy.
Figure 3. Estimated percent lethality versus radiation dose based on a probit transformation of percent mortality based on the method of Miller and Tainter (Citation1944) for (a) Gottingen minipigs receiving standard supportive care (dotted line) versus limited supportive care (dashed line) following total body exposures to gamma irradiation; and (b) for NHPs receiving full supportive care (solid line), standard supportive care (dotted line) or limited supportive care (dashed line) following total body exposures to 6 MV photon irradiation.

For the NHP, administration of supportive care similarly impacted survival, with the greatest impact associated with the inclusion of antibiotics (limited supportive care versus standard supportive care; ). For example, no animals survived exposure to 6.9 Gy in the limited supportive care group, compared to 70% survival at the same exposure level when administered standard supportive care (). A comparison of the dose response relationships for all three levels of supportive care is provided in . The slope of the dose–response relationship under limited supportive care is steep, with a dose differential between 10% and 90% mortality approximately 1.2 Gy.
Discussion
There is much interest in the development of standardized animal models consistent with the criteria established by the U.S. Food and Drug Administration (FDA) “Animal Rule” specifically focused on development of countermeasures to treat potentially lethal irradiation exposures. Frequently, the level of supportive care included in animal model development varies considerably from limited (analgesics only) to essentially mirroring the clinical intensive care unit, with individualized medicine and blood transfusions based on response of each animal. The objective of the work presented here was to provide a complete side-by-side dose range comparison of total body irradiation in two large animal models, the Göttingen minipig and the NHP and the resultant shift in lethality due to supportive care regimens.
Historical research conducted over 50 years ago established that infection and hemorrhage are two critical determinants in whether radiation exposures in the hematopoietic range have lethal consequences (Miller et al. Citation1952; Sorensen et al. Citation1960; Byron et al. Citation1964). Studies conducted in non-human primates, canines and rodents have established that even modest medical management can significantly enhance survival of lethally irradiated animals (Farese et al. Citation2012). To date, however, no systematic comparison of the impact of provision of supportive care on has been reported. Given the increasing use of experimental animal models for utility in development of radiation medical countermeasures, it is vital that characterization of the model include evaluation of the relationship between supportive care and lethality.
For the study reported here, the initial selection of irradiation doses for comparison of lethality with and without supportive care with the Gottingen minipig was based on a prior publication by Moroni et al. (Citation2011b) indicating an estimated LD50 of 1.73 Gy using total body irradiation, bilateral 60Co exposures at a dose rate of 60 cGy min−1 with no provision for supportive care. The dose response relationship profile established under similar exposure conditions and under limited supportive care measures was used to estimate a somewhat higher LD50 value of 1.96 Gy. Provision of standard supportive care shifted the lethality to the right, and a LD50 value of 2.15 Gy was estimated. Thus, in the minipig, provision of antibiotics and fluids administered on a set regimen regardless of symptom or indication provided a DMF of 1.09 (LD50 under standard supportive care to LD50 under limited supportive care ratio).
The estimated LD50 value (7.43 Gy) for the NHP exposed to total body irradiation and provided full supportive care based on individual animal indication and symptom was determined to be statistically identical to that reported previously (Farese et al. Citation2012; Thrall et al. Citation2015). In comparison, administration of supportive care under a set schedule regardless of indication and exclusion of blood transfusions shifted the dose response relationship to the left with an estimated LD50 of 7.05 Gy. The difference in these two scenarios of supportive care resulted in a DMF of 1.05 (LD50 under full supportive care to LD50 under standard supportive care ratio). A LD50 value of 6.14 Gy was estimated based on the dose response relationship established under the administration of limited supportive care. A comparison of the estimated LD50 values provided a DMF of 1.15 for the standard supportive care to limited supportive care ratio, and 1.21 for the full supportive care to limited supportive care ratio. It bears noting that the NHP lethality profiles are based on radiation doses that do not span the entire range resulting in LD0 to LD100, which may impact the slope of the curve. In spite of this, the side-by-side comparison provides value in directing future research efforts.
For both the minipig and the NHP, the shift in lethality in animals provided supportive care reported here is consistent with previous data from large research models, including canine and non-human primate. For example, in the canine, the estimated LD50 with clinical support (antibiotics, intravenous fluids and fresh platelets) was DMF of 1.3.
In summary, the work described here establishes the dose lethality relationship for whole body 60Co irradiation for the Göttingen minipig with and without provision of minimal supportive care. The administration of supportive care results in increased survival (DMF 1.1) and increased time to lethality relative to animals not receiving supportive care. Together, the studies reported here and work by others indicates the promising utility of the minipig as an alternative large animal model for radiation countermeasure development, consistent with the FDA “Animal Rule”.
Acknowledgements
The authors wish to acknowledge and thank the dedicated animal care staff for their skilled handling of the animals.
Declaration of interest
The authors declare no conflicts of interest.
Additional information
Funding
Notes on contributors
Karla D. Thrall
Karla D. Thrall, PhD, DABT, is the Senor Director of Radiation Biology at Altasciences Preclinical Seattle, Everett WA.
Saikanth Mahendra
Saikanth Mahendra, MS, DABR, is a board-certified Medical Physicist at Northwest Medical Physics Center, Lynnwood, WA.
Jamie Lovaglio
Jamie Lovaglio, DVM, DACLAM, is a board-certified Clinical Veterinarian at Rocky Mountain Laboratories, Hamilton, MT.
M. Keven Jackson
M. Keven Jackson, DVM, DACVP, is a board-certified research pathologist at Altasciences Preclinical Seattle, Everett WA.
References
- Augustine AD, Gondré-Lewis T, McBride W, Miller L, Pellmar TC, Rockwell S. 2005. Animal models for radiation injury, protection and therapy. Radiat Res. 164(1):100–109.
- Brook I, Elliott TB, Ledney GD, Shoemaker MO, Knudson GB. 2004. Management of postirradiation infection: Lessons learned from animal models. Mil Med. 169(3):194–197.
- Brook I, Elliott TB, Shoemaker MO, Ledney GD. 2005. Antimicrobials in the management of post-irradiation infection. Nato Rtg-099. 20:1–7.
- Brook I, Ledney GD. 1991. Ofloxacin and penicillin G combination therapy in prevention of bacterial translocation and animal mortality after irradiation. Antimicrob Agents Chemother. 35(8):1685–1687.
- Byron JW, Haigh MV, Lajtha LG. 1964. Effect of an antibiotic regime on monkeys exposed to total-body irradiation. Nature. 202(4936):977–979.
- Dainiak N. 2002. Hematological consequences of exposure to ionizing radiation. Exp Hematol. 30(6):513–528.
- Farese AM, Cohen MV, Katz BP, Smith CP, Jackson W, III, Cohen DM, MacVittie TJ. 2012. A nonhuman primate model of the hematopoietic acute radiation syndrome plus medical management. Health Phys. 103(4):367–382.
- International Commission on Radiological Units and Measurements: Quantitative Concepts and Dosimetry in Radiobiology 1979. ICRU Report 30.
- MacVittie TJ, Monroy R, Vigneulle RM, Zeman GH, Jackson WE. 1991. The relative biological effectiveness of mixed fission-neutron-ɣ radiation on the hematopoietic syndrome in the canine: Effect of therapy on survival. Radiat Res. 128(1 Suppl):S29–S36.
- Miller CP, Hammond CW, Thompkins MJ, Shorter G. 1952. The treatment of post irradiation infection with antibiotics. An experimental study in mice. J Lab Clin Med. 39:462–479.
- Miller LC, Tainter ML. 1944. Estimation of LD50 and its error by means of log-probit graph paper. Proc Soc Exp. Bio Med. 57(2):261–264.
- Moccia KD, Olsen CH, Mitchell JM, Landauer MR. 2010. Evaluation of hydration and nutritional gels as supportive care after total-body irradiation in mice (Mus musculus). J Am Assoc Lab Animal Sci. 49:323–328.
- Moroni M, Collbaugh TV, Mitchell JM, Lombardini E, Moccia KD, Shelton LJ, Nagy V, Whitnall MH. 2011c. Vascular access port implantation and serial blood sampling in the Göttingen minipig (sus scrofa domestica) model of acute radiation injury. J Am Assoc Lab. Animal Sci. 50:65–72.
- Moroni M, Coolbaugh TV, Lombardini E, Mitchell JM, Moccia KD, Shelton LJ, Nagy V, Whitnall MH. 2011a. Hematopoietic radiation syndrome in the Göttingen minipig. Radiat Res. 176(1):89–101.
- Moroni M, Lombardini E, Salber R, Kazemzedeh M, Nagy V, Olsen C, Whitnall MH. 2011b. Hematological changes as prognostic indicators of survival: Similarities between Gottingen minipigs, humans, and other large animal models. PLoS One. 6(9):e25210
- Sorensen DK, Bond VP, Cronkite EP, Perman V. 1960. An effective therapeutic regimen for the hemopoietic phase of the acute radiation syndrome in dogs. Radiat Res. 13(5):669–685.
- Thrall KD, Love R, Oʼdonnell KC, Farese AM, Manning R, MacVittie TJ. 2015. An interlaboratory validation of the radiation dose response relationship (DRR) for H-ARS in the rhesus macaque. Health Phys. 109(5):502–510.
- Waselenko JK, MacVittie TJ, Blakely WF, Pesik N, Wiley AL, Dickerson WE, Tsu H, Confer DL, Coleman CN, Seed T, Strategic National Stockpile Radiation Working Group, et al. 2004. Medical management of the acute radiation syndrome: Recommendations of the Strategic National Stockpile Radiation Working Group. Ann Intern Med. 140(12):1037–1051.