Abstract
Purpose
This review is focused on radium and radionuclides in its decay chain in honor of Marie Curie, who discovered this element.
Materials and methods
We conglomerated current knowledge regarding radium and its history predating our present understanding of this radionuclide.
Results
An overview of the properties of radium and its dose assessment is shown followed by discussions about both the negative detrimental and positive therapeutic applications of radium with this history and its evolution reflecting current innovations in medical science.
Conclusions
We hope to remind all those who are interested in the progress of science about the vagaries of the process of scientific discovery. In addition, we raise the interesting question of whether Marie Curie’s initial success was in part possible due to her tight alignment with her husband Pierre Curie who pushed the work along.
Keywords:
1. Discoverer of radium
Many detailed and authoritative books have been written about Marie Curie whose remarkable life can be viewed from many perspectives. Polish-born and French naturalized, Curie was: (1) the first woman to earn a doctorate in France, (2) the first woman to receive a Nobel Prize (The Nobel Prize 1903, shared between Henri Becquerel, and Marie and her husband, Pierre Curie, ‘in recognition of the extraordinary services they have rendered by their joint research on the radiation phenomena discovered by Professor Henri Becquerel’ (The Nobel Prize 1903) (3) the only woman to win two Nobel Prizes in science (The Nobel Prize 2011), won on her own ‘in recognition of her services to the advancement of chemistry by the discovery of the elements radium and polonium, by the isolation of radium and the study of the nature and compounds of this remarkable element’ (The Nobel Prize Citation1911) (4) the only person (man or woman) to win two Nobel Prizes in different fields (The Nobel Prize Citation1903 for Physics and The Nobel Prize Citation1911, for Chemistry), (5) the first person to coin the term ‘radioactivity’, (6) the first to invent isotope separation techniques, (7) the person to discover the elements polonium and radium, (8) the first and only female member of the seven International Solvay Physics conferences organized from 1911 to 1933 which gathered the most renowned physicists and chemists of the day, (9) the first person to demonstrate the utility of radiation in the treatment of cancer, (10) the first person to head the Red Cross Radiology Service and to develop mobile X-ray and radiation therapy units for World War I military medical use (using her own personal supply of radium to do so), (11) the person who founded the Curie Institute in both France and Poland, (12) the person who wrote her late husband’s biography, (13) a person who served on the International Atomic Weights Committee, and (14) a person who raised a Nobel-winning daughter (Irène Joliot-Curie, Chemistry, 1935).
Her lifelong journey with radium (among other radioactive materials) began with Henri Becquerel’s discovery of natural radiation in 1896. Inspired to further investigate pitchblende – a uranium source that was found to be more radioactive than uranium itself – they eventually discovered and isolated both radium and polonium as sources of the excess radioactivity. Her 1903 PhD thesis, Recherches sur les substances radioactives (Curie Citation1903, Citation1961; Wolke Citation1988), secured her share of the Nobel Prize in the same year and struck a death blow to the concept of the atom as indivisible. If there were questions about the Nobel worthiness of a PhD candidate, they were erased in 1911 when she was the sole winner of the Nobel Prize in Chemistry for the discovery of radium and polonium and isolation of radium.
In 1898, Marie and Pierre Curie discovered radium, although the experiments were done by both examining radium chloride ore mined from the spa town of Jáchymov (which at that time was called Joachimsthal) in today’s Czech Republic (for more information, see below). While both were credited with the discovery Marie actually did the experiments that led to the finding, while Pierre added his own interpretation to the results. In 1911, Marie followed up on this finding with electrolysis of radium chloride performed in collaboration with André-Louis Debierne for the final isolation of radium itself.
During the first World War, Marie designed mobile X-ray stations for field diagnosis of injuries, donating her own effort to obtain funding and train imaging staff. Even her death from aplastic anemia was most likely due to internal and external exposures to radiation during her career and echoed her achievements in unlocking atomic mysteries and advancing physics, chemistry, and medicine. Marie was the first woman to be enshrined in the Paris Pantheon where she and her husband’s ashes were transferred to in 1995. Her life’s work had profound global impacts – for good and for ill – from her time through to the present day as highlighted in this report which was prepared for a special issue of IJRB.
2. Properties of radium
The unit of radioactivity, the ‘curie’, was named for Marie and Pierre Curie and is defined as the activity from 1 g of radium decay, about 3.7 × 1010 decays per second. Radium has 33 known isotopes ranging from 202Ra to 234Ra (), of which seven are of interest in radiation dosimetry given in . Radium is found in the form of oxides, nitrates, chlorides, sulfates and luminescing residues in industrial settings and as trace amounts in uranium ores. Mixtures of beryllium and radium as 226RaBe form neutron sources through (α, n) and (γ, n) interactions. Occupational radium originates from 224Ra, 226Ra, and 228Ra. As radium behaves similarly to calcium (Ca) and barium (Ba), 223Ra has become an emerging modality for targeted alpha therapy for bone cancer metastases.
Table 1. Properties of radium isotopes in radiation dosimetry.
3. Dose assessment
In radiation protection from radium, most consideration is given to radon and thoron (220Rn) progeny because they comprise the main sources of exposure. Exposure to radon, whether occupational (e.g. uranium mining) or environmental (e.g. home), depends on local geology, building composition and construction and household lifestyles. According to the United Nations Scientific Committee on the Effects of Atomic Radiation (UNSCEAR), natural radiation comprises 80% of the total average annual dose of ionizing radiation to the global population, from which half (1.2 mSv) is attributable to the inhalation of radon gas (UNSCEAR Citation2010).
The most prevalent dosimetric impact from radium through the inhalation pathway comes from the radium progeny as radon (Rn). Radon gas is formed during the decay of natural uranium in the soil and rock of the earth and seeps into interior dwellings. Radiological uptake of radon is typically through the inhalation of airborne radon and its progeny, from which the predominant dose is due to its radioactive decay products (or progeny) and not the inhalation of radon gas itself. The isotopes of radon considered range from the primordial radionuclides, 238U giving rise to radon (as 222Rn) from 226Ra, 232Th giving rise to thoron from 224Ra, and 235U giving rise to actinon (219Rn) from 223Ra.
Radon, thoron and actinon gases decay into solid progeny comprised of radionuclides that emit alpha particles. These progenies deliver >95% of the dose received by the lung airway epithelia. As progeny decay, they form 1 nm clusters, termed the ‘unattached fraction’ alongside larger particles (10 nm to >1 nm), termed the ‘attached fraction’. The difference in concentration between radon gas and solid progeny is given by the ‘equilibrium factor’, its value depends on the radon progeny rather than the radon gas. Radon gas is chemically inert but can be absorbed into the pulmonary, arterial and venous blood. Measurement of the radium radionuclide, typically from gamma spectroscopy of the progeny of the 238U and 232Th series (), can be used to calculate the activity concentration and dose coefficient models to determine internalized dose. Effective dose from the inhalation of radon is attributed mostly to lung equivalent doses from the deposition in the respiratory airway of solid aerosol particles (), which is dependent on the particle size distribution. Physiologically, breathing rate is additionally a core factor in determining the intake and subsequent lung dose.
Figure 2. Different areas of lung give rise to different types of lung cancer. For example, squamous cell carcinoma (SCC) occurs mostly in large bronchi, while adenocarcinomas (ADC) affect distal lung. Radium radionuclides that emit alpha and beta particles cause ionization of biological molecules within a spherical volume that changes over time with the decay progression. The position of radium inside the lung, however, depends on the chemical form and size distribution of particulate matter that contains radium. Consequently, DNA damage resulting in mutations occurs in different areas of the lung depending on radionuclide distribution. Therefore, placement of the same radionuclide close to cells lining large vs. small and distal lung airways will result in development of different cancer types. In other words, physical objects that regulate radionuclide distribution and biological properties of the targeted cells are more important for determination of cancer type than the radionuclide itself.
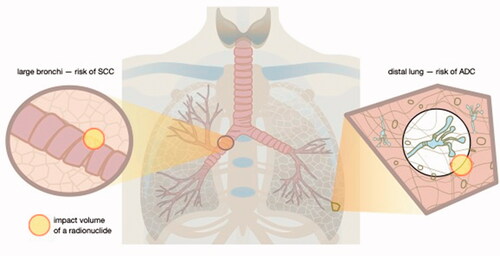
ICRP Publication 115 (ICRP Citation2010) provides updated risks of lung cancer from radon and its progeny based on estimates derived from epidemiological studies of miner cohorts exposed to low levels of radon and lung cancer risk associated with indoor radon exposure. ICRP Publication 137 (ICRP Citation2017), iterated on ICRP Publication 103 (ICRP Citation2007) detriment, ICRP Publication 115 risk coefficients, and epidemiological evidence to derive effective dose coefficients given in Table 12.6 of ICRP Publication 137 for indoor workplaces, mines and tourist caves for radon progeny (218Po/214Pb/214 Bi) and thoron progeny (212Pb/212 Bi). depicts the fractional retention from uptakes of radium progeny from 226Ra inhalation and ingestion for those concentrations that are relevant to occupational or environmental exposures (Clark Citation1997; ICRP Citation2010; Gunderman and Gonda Citation2015; Cohen and Kim Citation2017; Martinez et al. Citation2021) and 223Ra for targeted radiopharmaceutical therapy (Höllriegl et al. Citation2021).
Figure 3. The anatomical location and the total magnitude of radioactivity of radium and its various progeny is depicted following three intake scenarios: (top row) inhalation of the radioisotope 226Ra, (middle row) ingestion of the radioisotope 226Ra, and (bottom row) injection of the radioisotope 223Ra. For each scenario, we show graphically the biodistribution of total activity (parent plus ingrown daughter radionuclides) at three time points since the intake (at t = 0). The colored scale is normalized to 100% which represents a unit intake (say 1 Bq) of the radium isotope by either inhalation, ingestion, or injection. We note that with in-situ decay time and biological excretion, the displayed total activity can fall below the intake value (percentages below 100%), but in other cases, the accumulation of multiple short-lived decay products (radioactive progeny) will give us a total body activity (across all radioisotopes) that exceeds the intake value (percentages above 100%).
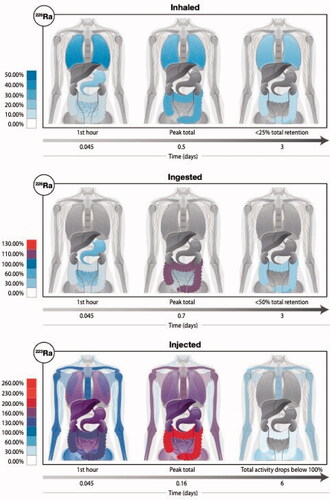
With in-situ decay time and biological excretion, the displayed total activity in can fall below the intake value (percentages below 100%), but in other cases, the accumulation of multiple short-lived decay products (radioactive progeny) will give us a total body activity (across all radioisotopes) that exceeds the intake value (percentages above 100%). As given in transfer coefficients defining the biokinetic model in ICRP Publication 137 (ICRP Citation2017), inhalation of 226Ra results in <50% initial total activity retention for radium and its progeny (). In the lungs, biological and physical decay results in retention of less than 10% of the total activity retention in the first 12 days. Meanwhile, uptake to the bone surface and marrow drops to less than 1% of the total activity retention in the first 12 days. Ingestion of 226Ra leads to accumulation primarily in the stomach (100% parent activity). The total activity peaks in the body within 17 h of ingestion with different retention in the small intestine, colon, and bone as detailed by Martinez et al. (Martinez et al. Citation2021). Injection of 223Ra results in a peak total activity exceeding the initial activity of the 223Ra parent six-fold within the first 4 h of injection, migrating initially from the blood (20%) and liver (6.4%), accumulating in the colon (221.7%) and bone (173%) due to progeny ingrowth of physically short-lived radionuclides including 219Rn (t1/2 = 3.96 s), 215Po (t1/2 = 1.78 ms), 211Pb (t1/2 = 36.1 min), 211 Bi (t1/2 = 2.14 min), 211Po (t1/2 = 0.516 s), and 207Tl (t1/2 = 4.77 min) with 223Ra pharmacokinetics described by Höllrieg et al. (Höllriegl et al. Citation2021). Total body retention drops below 10% of the initial parent activity within 30 days of injection.
4. Detrimental health effects and possible treatments
Following Marie Curie’s discovery of radium, this radioactive element was soon combined with paint to create a luminescent dye that could be used to identify items in the dark. These included airplane dials and watch dials, and the painting process required precision. Many of the individuals hired by Luminous Processes and several other companies were young women who were unaware of the risks from exposure. They would typically make the tip of the paintbrush fine by licking it thereby ingesting this radionuclide (Clark Citation1997; Gunderman and Gonda Citation2015; Cohen and Kim Citation2017; Martinez et al. Citation2021). As noted before, radium is chemically similar to calcium and targets bone; the most common isotope 226Ra releases alpha particles (and to a lesser extent gamma-rays). Once lodged in the bone it causes damage to the nearby cells.
Bone cancer (skull, jaw) often resulted from the ingested radiation (Polednak Citation1978; Adams and Brues Citation1980; Spiers et al. Citation1983; Stebbings et al. Citation1984; Carnes et al. Citation1997). Some other cancers were also shown to be increased in these women (for example multiple myeloma, which is thought to be due to the gamma-ray exposure; leukemias; breast cancer; and others). These ‘Radium Girls’ from the recently released movie of the same title were also exposed to inhalation hazards since some of the radiation became airborne in flakes or dust and resulted in lung cancer. Dose–response relationships were also examined in several studies (Rowland et al. Citation1983; Bijwaard Citation2006). Reviews have examined results from these studies (Fry Citation1998; Boice et al. Citation2019), and there is significant information about them in the literature.
The numerous studies performed on the radium dial workers generated a rich source of information that continues to inform an understanding of radiation carcinogenesis in the world today. Studies of this exposed population enhanced a broad scientific understanding of the health effects of internally deposited radionuclides, basic modeling of internal emitters and understanding of molecular mechanisms of cancers induced by internal emitters.
Because of the large amount of radioactivity, many of the bodies of these radium workers remain buried at a site at the Argonne National Laboratory.
There is no specific decorporation treatment currently available to promote the targeted removal of radium from contaminated patients. However, administration of calcium is recommended and may be beneficial (Hickman Citation2010), as both calcium and radium are alkaline earth metals and a mass effect could help calcium interfere with the absorption and deposition of radium. Large doses of calcium can be provided as common, over-the-counter oral calcium supplements, or through the repeated intravenous administration of the calcium salt of gluconic acid, calcium gluconate, a medication frequently used in the management of hypocalcemia (Fong and Khan Citation2012), cardiac arrest (Kette et al. Citation2013), and cardiotoxicity due to hyperkalemia or hypermagnesemia (Vanden Hoek et al. Citation2010). An additional potential treatment option is the use of sodium alginate, a kelp derivative known to bind another alkaline earth metal (Gao et al. Citation2020), strontium, in the gastrointestinal tract, thereby preventing radium absorption.
Recent studies have begun to re-assess the work done with radium dial painters, and a new review by Martinez et al. (Martinez et al. Citation2021) examines the history and the updates that are ongoing with the epidemiological cohort. This work is being done as part of the Million Person Study of American workers and veterans; updates have included new dosimetric analyses as well as modeling. In late 2021, the National Council on Radiation Protection and Measurements (NCRP) formally established Scientific Committee 6-13 to explore a systematic reassessment of the radium intakes of the Dial Painters and their resulting organ doses over time.
5. Therapeutic health effects
5.1. Radon spa – is every radon dose dangerous?
The idea to use radon as a therapeutic agent and the rise of radon spas came to prominence during the period of heavy uranium mining that took place in the cold war but can be traced back much further than that, perhaps even to the Roman empire (Trott Citation2005). The first major mines were in former Czechoslovakia and East Germany in central Europe, isolating more than 300,000 tons of uranium ore between the end of WWII until recently (Miloš Citation2017). Marie Curie’s initial source of pitchblende and radium are famously linked to that area along the Czech/German border. As Mathew Vickery (BBC) noted, there was a dark side to uranium mining during that time which was its connection to forced labor of political prisoners. With time, the uranium mining stopped, but the spas are still in use. The ‘Radium Palace’ in Jáchymov (Czech Republic) that first opened in 1912 still runs to this day. Similar radon spas or radium-rich springs still exist in Austria, Germany, Poland, Romania, Bulgaria, Russia, Italy, France, Serbia, Greece, Chile and Japan (Deetjen et al. Citation2005; Zdrojewicz and Strzelczyk Citation2006).
The dose of radon in the air in the European mines can range from about 37 Bq/L up to 167 Bq/L, which is about 1000 times the US EPA’s recommendations for maximum home exposure (Erickson Citation2006). Bad Gastein Spa in Austria reports <1000 Bq/L for their radon water, while Jáchymov’s radioactive water baths measure around 2500 Bq/L, which in turn is a magnitude below that on the German side in Bad Brambach (Erickson Citation2006; Miloš Citation2017). In the US, whether out of caution or because of competing interests, radon treatment has never been approved by the American Medical Association and is not covered by insurance companies. Instead, patients have to rely on their own good judgment and initiative, when visiting places such as the old uranium mines around Boulder and Basin in Montana (Erickson Citation2006). There, the general suggestion is to follow a 10-day treatment course and a total of 32 h spent in the mine with no oversight, no personnel, patient records or any inspection by governmental health agencies. Similarly, patients who travel to Safaga in Egypt and bury their rheumatic joints in radioactive sands during ‘vacations with benefit’ or climatotherapy are left to their own devices (Nuzzo Citation2007). With regard to the ‘radon at homes’ even, a few of the recent studies found that the relative number of lung cancers decreases with increasing radon concentrations in the air for radon concentrations up to 200–300 Bq/m3 (Thompson et al. Citation2008; Dobrzynski et al. Citation2018; Pylak et al. Citation2021).
Radon therapy was historically tried for a variety of ailments, including painful joints, skin problems, respiratory illness and allergies. In the present day, radon therapy is primarily used to treat painful degenerative or inflammatory joint diseases, particularly rheumatoid arthritis and ankylosing spondylitis (Bechterew’s disease) – conditions that tend to have strong inflammatory and often autoimmune components. Exposure takes place within warm radon water baths, steam baths or by inhalation of radon gas/steam and in some rarer cases through ingestions of radon-infused water. The route of uptake is therefore generally transcutaneous or through pulmonary or intestinal mucosal resorption. The elevated temperature of water and steam tends to aid this process by increasing blood circulation. A typical treatment of 9–10 independent 20 min baths in 600–1600 Bq/L radon water over 3 weeks might result in an estimated epidermal skin dose of about 2 mGy, and as an alpha emitter with only a weak gamma component, the amount reaching the circulation and inner organs is likely in the order of a few µGy or 0.2–0.5 mSv (Deetjen et al. Citation2005). The low fluence of radon emission suggests that resultant cellular ionization events can be dense but very localized, although they may be potentially amplified through bystander effects. This is where exploration of the mechanism of action becomes interesting ().
Figure 4. Mechanisms of action. The current school of thought suggests that there is enough alpha particle damage to cause apoptosis in a few keratinocytes or other epithelial cells, which in turn get phagocytosed by Langerhans cells (LC) or similar antigen presenting cells that migrate to the lymph nodes where they can modulate the immune balance systemically away from the pro-inflammatory Th1 bias (Deetjen et al. Citation2005). Much of this seems to rely on anti-inflammatory cytokines such as TGF-β and IL-10 either directly from dying cells or following uptake by neighboring phagocytes with the assumption that these cytokines are potent inhibitors of inflammatory processes such as leukocyte infiltration and macrophage/neutrophil function. This concept is largely an extrapolation from studies on ultraviolet (UV) radiation or low dose ionizing radiation. Nonetheless, there is growing evidence from clinical and pre-clinical studies that would support this therapeutic radon concept of rebalancing inflammatory/immune networks.
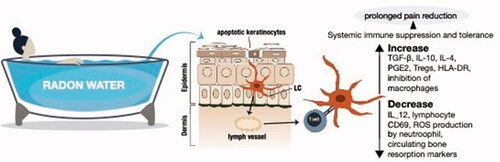
The clinical experience with radon therapy, including in more recent prospective, randomized, double-blind and placebo-controlled settings has been one of fairly consistent anti-inflammatory and prolonged analgesic effects (Franke et al. Citation2000; Deetjen et al. Citation2005; Rühle et al. Citation2017). Compared to other treatments, these benefits are slow to develop and tend not to reach a level of significance until months later but can be long-lasting (Falkenbach et al. Citation2005; Rühle et al. Citation2019). Even though the reports of reduction in painkiller usage by many radon-treated patients appear at first sight to be fairly trivial, they should not be dismissed too easily as side effects of prolonged drug treatment and can be especially severe in the elderly. The fact that treatment-induced pain reduction can coincide with a rise in circulating TGF-β and/or a fall in TNF-β adds some weight to the general concept as does the drop in activation markers on lymphocytes and reduced reactive oxygen species (ROS) output by phagocytes (Reinisch Citation1999; Shehata et al. Citation2004; Rühle et al. Citation2017; Kullmann et al. Citation2019). Those familiar with the topic will recognize parallels to the use of low-dose radiotherapy for the treatment of chronic benign conditions of different disease etiology, using low linear energy transfer (LET) and slightly higher radiation dose levels than radon therapy.
5.2. Cancer treatment and radiopharmaceutical therapy
Radium was in use for the treatment of different types of topical cancers (uterine, cervical, rectal, etc.) from the early 1900s and ‘radium institutes’ opened not only in France and Poland where Marie Curie was engaged as one of the contributors but also in other parts of the world (Lenz Citation1973). A PubMed search in August 2021 on radium and cancer brings over 11,000 references.
Over the past two decades, the radiological and chemical properties of radium isotopes have re-gained prominence in medical physics and radiation oncology through the development of 223RaCl2 as a radiopharmaceutical for alpha-therapy (Marques et al. Citation2018). Alpha therapy (referred to as α-emitter radiopharmaceutical therapy) holds great potential as a cancer treatment: It promises the delivery of a highly cytotoxic dose to cancer cells while lessening damage to surrounding tissue, due to the short-range and high linear energy transfer (LET) of alpha particles (Parker et al. Citation2018). In addition, alpha therapy could help circumvent adaptive resistance and cell cycle progression, which are hurdles associated with conventional radiation therapy. Ra-223, commercialized as Xofigo is the first alpha emitter approved by both the U.S. Food and Drug Administration (FDA) and the European Medicines Agency (EMA) to treat bone metastatic tumors () that originated from castration-resistant prostate cancer (Kluetz et al. Citation2014; Gourd Citation2018). The relatively short half-life of 223Ra (11.4 days, vide supra) is ideal for a radiotherapeutic treatment, with up to 27.9 MeV released from the four alpha and two beta particles sequentially emitted throughout its decay pathway down to 207Pb. New therapies with Ra are being explored in the cancer treatment literature almost daily. Further studies are needed to investigate the beneficial or adverse biological effects of radon overall.
6. Conclusion
As a discoverer of radium, Marie Curie can be at least partially credited for all the radium-associated topics discussed in this review. We can also expand this statement and, in this special issue of the IJRB say that these discoveries should be attributed to the joint work done by the women in science. The discoveries of Marie Curie have changed the world – from the therapy of diseases to the imaging of diseases to mechanistic understandings of our environment. Her example and prominence have inspired young people, male and female alike, influencing generations to study physics, chemistry, biology, and more.
Despite the importance of Marie Curie in this, we also must consider the question of the role of women in science differently. For example, we could ask ourselves – would Marie accomplish all she did if she was not working with a male colleague (her husband) who had much fewer barriers on his path compared to a woman working on her own? Is it not logical to assume that there were and perhaps still are other women with knowledge and capacity whose path to scientific discoveries was blocked by obstacles arising from everyday prejudice and whose potential discoveries the world will never see? This special issue is prepared to honor women scientists who were fortunate enough to contribute to science; we should try to leverage a fuller understanding of the challenges of research to decrease some of the barriers still faced by female scientists.
Acknowledgments
We thank Susan Brand and Caitlin Youngquist for creating new artwork for this manuscript, which was supported by the University of California Contractor Supporting Research Program at Lawrence Berkeley National Laboratory under U.S. Department of Energy Contract No. DE-AC02-05CH11231.
Disclosure statement
No potential conflict of interest was reported by the author(s).
Additional information
Funding
Notes on contributors
Rebecca Abergel
Rebecca Abergel is an Associate Professor of Nuclear Engineering at the University of California, Berkeley, and a Faculty Scientist at the Lawrence Berkeley National Laboratory, where she leads the Heavy Element Chemistry group.
John Aris
John Aris, PhD, is an Associate Professor in the Department of Anatomy and Cell Biology at the University of Florida, Gainesville, Florida, USA.
Wesley E. Bolch
Wesley E. Bolch, PhD, is a Distinguished Professor in the J. Crayton Pruitt Family Department of Biomedical Engineering at the University of Florida, Gainesville, Florida USA, and Director of ALRADS – Advanced Laboratory for Radiation Dosimetry Studies.
Shaheen A. Dewji
Shaheen A. Dewji, PhD, is an Assistant Professor in the Nuclear and Radiological Engineering and Medical Physics Programs at the George W. Woodruff School of Mechanical Engineering, Georgia Institute of Technology, Atlanta, GA, USA.
Ashley Golden
Ashley Golden, PhD, is the Director of ORISE Health Studies and Senior Biostatistician in Epidemiology and Exposure Science at Oak Ridge Associated Universities, Oak Ridge Tennessee.
David A. Hooper
David A. Hooper, PhD, is a Senior R&D Staff Member and the Interim Group Leader for the Detonation Forensics and Response group at Oak Ridge National Laboratory, Oak Ridge, Tennessee.
Dmitri Margot
Dmitri Margot is a PhD student in the Nuclear and Radiological Engineering and Medical Physics Programs at the George W. Woodruff School of Mechanical Engineering, Georgia Institute of Technology, Atlanta, GA, USA.
Carly G. Menker
Carly G. Menker is a Researcher in the Department of Radiation Biology, at Northwestern University Lurie Comprehensive Cancer Center, Feinberg School of Medicine, Chicago, IL.
Tatjana Paunesku
Tatjana Paunesku, PhD, is a Research Associate Professor in the Department of Radiation Biology, at Northwestern University Lurie Comprehensive Cancer Center, Feinberg School of Medicine, Chicago, IL.
Dörthe Schaue
Dörthe Schaue, PhD, is an Associate Professor in the Department of Radiation Oncology, David Geffen School of Medicine at the University of California at Los Angeles (UCLA), Los Angeles, California, USA.
Gayle E. Woloschak
Gayle E. Woloschak, PhD, is Professor of Radiation Oncology at Northwestern University Lurie Comprehensive Cancer Center, Feinberg School of Medicine, Chicago, IL.
References
- Adams E, Brues AM. 1980. Breast cancer in female radium dial workers first employed before 1930. J Occup Med. 22(29):583–587.
- Bijwaard H. 2006. Mechanistic models of bone cancer induction by radium and plutonium in animals compared to humans. Radiat Prot Dosimetry. 122(1–4):340–344.
- Boice J, Held KD, Shore RE. 2019. Radiation epidemiology and health effects following low-level radiation exposure. J Radiol Prot. 39(4):S14–S27.
- Carnes B, Groer PG, Kotek TJ. 1997. Radium dial workers: issues concerning dose response and modeling. Radiat Res. 147(6):707–114.
- Clark C. 1997. Radium girls, women and industrial health reform: 1910-1935. Chapel Hill (NC): Univ of North Carolina Press.
- Cohen DE, Kim RH. 2017. The legacy of the radium girls. JAMA Dermatol. 153(8):801.
- Curie M. 1903. Recherches sur les substances radioactives [PhD Thesis]. Paris (France): Faculty of Sciences.
- Curie M. 1961. [Radioactive substances] [PhD thesis]. Paris (France): Faculty of Sciences. French.
- Deetjen P, Falkenbach A, Harder D, Jöckel H, Kaul A, Philipsborn H. 2005. Radon als Heilmittel. Therapeutische Wirksamkeit, biologischer Wirkungsmechanismus und vergleichende Risikobewertung. Hamburg (Germany): Verlag.
- Dobrzynski L, Fornalski KW, Reszczynska J. 2018. Meta-analysis of thirty-two case-control and two ecological radon studies of lung cancer. J Radiat Res. 59(2):149–163.
- Erickson BE. 2006. The therapeutic use of radon: a biomedical treatment in Europe; an “alternative” remedy in the United States. Dose-Response. 5(1):48–62.
- Falkenbach A, Kovacs J, Franke A, Jörgens K, Ammer K. 2005. Radon therapy for the treatment of rheumatic diseases-review and meta-analysis of controlled clinical trials. Rheumatol Int. 25(3):205–210.
- Fong J, Khan A. 2012. Hypocalcemia: updates in diagnosis and management for primary care. Can Fam Physician. 58(2):158–162.
- Franke A, Reiner L, Pratzel HG, Franke T, Resch KL. 2000. Long-term efficacy of radon spa therapy in rheumatoid arthritis-a randomized, sham-controlled study and follow-up. Rheumatology. 39(8):894–902.
- Fry SA. 1998. Studies of U.S. radium dial workers: an epidemiological classic. Radiat Res. 150(155):S121–S159.
- Gao X, Guo C, Hao J, Zhao Z, Long H, Li M. 2020. Adsorption of heavy metal ions by sodium alginate based adsorbent-a review and new perspectives. Int J Biol Macromol. 164:4423–4434.
- Gourd E. 2018. EMA guidance on radium-223 dichloride in prostate cancer. Lancet Oncol. 19(4):e190.
- Gunderman RB, Gonda AS. 2015. Radium girls. Oak Brook (IL): Radiological Society of North America.
- Hickman DP. 2010. Management of persons contaminated with radionuclides. Vol. 1. Oxford (UK): Oxford University Press.
- Höllriegl V, Petoussi-Henss N, Hürkamp K, Ramos JCO, Li WB. 2021. Radiopharmacokinetic modelling and radiation dose assessment of 223Ra used for treatment of metastatic castration-resistant prostate cancer. EJNMMI Phys. 8(1):1–18.
- ICRP. 2007. ICRP publication 103: recommendations of the international commission on radiological protection. Ann ICRP. 37(2–4):1–332.
- ICRP. 2010. ICRP publication 115: lung cancer risk from radon and progeny and statement on radon. Ann ICRP. 40(1):1–64.
- ICRP. 2017. ICRP publication 137: occupational intakes of radionuclides: part 3. Ann ICRP. 46(3–4):1–486.
- Kette F, Ghuman J, Parr M. 2013. Calcium administration during cardiac arrest: a systematic review. Eur J Emerg Med. 20(2):72–78.
- Kluetz PG, Pierce W, Maher VE, Zhang H, Tang S, Song P, Liu Q, Haber MT, Leutzinger EE, Al-Hakim A, et al. 2014. Radium Ra 223 dichloride injection: US Food and Drug Administration drug approval summary. Clin Cancer Res. 20(1):9–14.
- Kullmann M, Rühle PF, Harrer A, Donaubauer A, Becker I, Sieber R, Klein G, Fournier C, Fietkau R, Gaipl US, et al. 2019. Temporarily increased TGFβ following radon spa correlates with reduced pain while serum IL-18 is a general predictive marker for pain sensitivity. Radiat Environ Biophys. 58(1):129–135.
- Lenz M. 1973. The early workers in clinical radiotherapy of cancer at the Radium Institute of the Curie Foundation, Paris, France. Cancer Sep. 32(3):519–523.
- Marques I, Neves A, Abrantes A, Pires A, Tavares-da-Silva E, Figueiredo A, Botelho M. 2018. Targeted alpha therapy using radium-223: from physics to biological effects. Cancer Treat Rev. 68:47–54.
- Martinez NE, Jokisch DW, Dauer LT, Eckerman KF, Goans RE, Brockman JD, Tolmachev SY, Avtandilashvili M, Mumma MT, Boice JD, et al. 2021. Radium dial workers: back to the future. Int J Radiat Biol. :1–19. doi:https://doi.org/10.1080/09553002.2021.1917785.
- Miloš R. 2017. History of uranium mining in Central Europe. Uranium - safety, resources, separation and thermodynamic calculation. London (UK): IntechOpen.
- Nuzzo R. 2007. Vacations with benefits. Los Angeles Times. May 28, 2007 https://www.latimes.com/archives/la-xpm-2007-may-28-he-climate28-story.html
- Parker C, Lewington V, Shore N, Kratochwil C, Levy M, Lindén O, Noordzij W, Park J, Saad F. 2018. Targeted alpha therapy, an emerging class of cancer agents: a review. JAMA Oncol. 4(12):1765–1772.
- Polednak AP. 1978. Bone cancer among female radium dial workers. Latency periods and incidence rates by time after exposure: brief communication. J Natl Cancer Inst. 60(1):77–82.
- Pylak M, Fornalski KW, Reszczyńska J, Kukulski P, Waligórski MPR, Dobrzyński L. 2021. Analysis of indoor radon data using bayesian, random binning, and maximum entropy methods. Dose Response. 19(2):15593258211009337.
- Reinisch N. 1999. Reduktion der Sauerstoffradikalfreisetzung aus Neutrophilen bei Paienten mit ankylosierender Spondylitis durch aine kombinierte Radon- und Hyperthermietherapie. Radon und Gesundheit. Frankfurt (Germany): Verlag.
- Rowland R, Stehney AF, Lucas HF. 1983. Dose-response relationships for radium-induced bone sarcomas. Health Phys. 44(41):15–31.
- Rühle PF, Klein G, Rung T, Tiep Phan H, Fournier C, Fietkau R, Gaipl US, Frey B. 2019. Impact of radon and combinatory radon/carbon dioxide spa on pain and hypertension: results from the explorative RAD-ON01 study. Mod Rheumatol. 29(1):165–172.
- Rühle PF, Wunderlich R, Deloch L, Fournier C, Maier A, Klein G, Fietkau R, Gaipl US, Frey B. 2017. Modulation of the peripheral immune system after low-dose radon spa therapy: detailed longitudinal immune monitoring of patients within the RAD-ON01 study. Autoimmunity. 50(2):133–140.
- Shehata M, Schwarzmeier JD, Hilgarth M, Hubmann R, Duechler M, Gisslinger H. 2004. TGF-beta1 induces bone marrow reticulin fibrosis in hairy cell leukemia. J Clin Invest. 113(5):676–185.
- Spiers F, Lucas HF, Rundo J, Anast GA. 1983. Leukaemia incidence in the U.S. dial workers. Health Phys. 44(41):65–72.
- Stebbings J, Lucas HF, Stehney AF. 1984. Mortality from cancers of major sites in female radium dial workers. Am J Ind Med. 5(6):435–459.
- The Nobel Prize in Physics. 1903. NobelPrize.org. Nobel Prize Outreach AB 2021; [accessed 2021 Dec 30]. https://www.nobelprize.org/prizes/physics/1903/summary/
- The Nobel Prize in Chemistry. 1911. NobelPrize.org. Nobel Prize Outreach AB 2021; [accessed 2021 Dec 30]. https://www.nobelprize.org/prizes/chemistry/1911/summary/
- Thompson RE, Nelson DF, Popkin JH, Popkin Z. 2008. Case-control study of lung cancer risk from residential radon exposure in Worcester County, Massachusetts. Health Phys. 94(3):228–241.
- Trott K-R. 2005. Radon als Heilmittel. Therapeutische Wirksamkeit, biologischer Wirkungsmechanismus und vergleichende Risikobewertung (Radon therapy. Therapeutic effects, biological mechanism, and relative risk assessment). Radiat Environ Biophys. 44(2):157–158.
- UNSCEAR. 2010. UNSCEAR 2008 report: sources and effects of ionizing radiation. New York (NY): United Nations.
- Vanden Hoek TL, Morrison LJ, Shuster M, Donnino M, Sinz E, Lavonas EJ, Jeejeebhoy FM, Gabrielli A. 2010. Part 12: cardiac arrest in special situations: 2010 American Heart Association guidelines for cardiopulmonary resuscitation and emergency cardiovascular care. Circulation. 122(18):S829–S861.
- Wolke RL. 1988. Marie Curie’s doctoral thesis: prelude to a nobel prize. J Chem Educ. 65(7):561.
- Zdrojewicz Z, Strzelczyk JJ. 2006. Radon treatment controversy. Dose Response. 4(2):106–118.