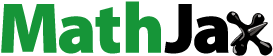
Abstract
ABSTRACT
The psychometric qualities of the proprioception and dynamic trunk control tests have rarely been studied in individuals after stroke.
Objective
To investigate the reliability and validity of the Trunk Position Sense Test (TPS) and Modified Functional Reach Test (MFRT) in persons after stroke.
Methods
Thirty-two participants were included. The TPS and MFRT were assessed by two physiotherapists during a first session. After resting, a second session was conducted. The intraclass correlation coefficient (ICC) was calculated to assess the test–retest (ICC3,k) and inter-rater reliability (ICC2,k). Pearson correlations coefficients were calculated between TPS/MFRT performances and clinical tests (trunk strength, Timed Up and Go and Balance Assessment in Sitting and Standing Positions – BASSP).
Results
The TPS inter-rater reliability was good for vertical error (ICC = 0.75 [0.50–0.88]) while it was moderate for horizontal error (ICC = 0.48 [0.10–0.75]) as well as for test–retest reliability (0.39 ≤ ICC ≤ 0.59). As for the MFRT, inter-rater (0.76 ≤ ICC ≤ 0.90) and test–retest reliability (0.71 ≤ ICC ≤ 0.91) were good to excellent for anterior, paretic et non-paretic displacements. Horizontal errors for the TPS (−0.26 ≤ r ≤ −0.36) and anterior MFRT (0.38 ≤ r ≤ 0.64) values correlated moderately with trunk strength.
Conclusion
The MFRT is a reliable test for persons after stroke with trunk control impairments. The TPS does not appear to be relevant for post-stroke individuals. This can be explained by the fact that its procedure is not easily applied for individuals after stroke – who may have significant motor and cognitive impairments.
Introduction
In order to develop an accurate diagnosis and to be able to readjust the neuro-rehabilitation treatment plan, it is crucial to use the best clinical tests. In neurology, the factors to consider when selecting outcome measure in clinical practice are mainly the type of measure, the patient and clinical factors, the feasibility and the psychometric factors (Potter, Fulk, Salem, and Sullivan, Citation2011). However, many tests are used in clinical practice without the psychometric qualities being known, especially for quantitative tests on the trunk control for individuals after stroke.
The trunk control is the ability to maintain the posture of the body, to adjust weight shifting, and to perform selective movements in the trunk to maintain the center of mass within the limits of the base of support (Jung, Kim, Chung, and Hwang, Citation2014). Hemiparesis after stroke induces limited postural control during: sitting (Morishita et al., Citation2009); standing (Duclos, Maynard, Abbas, and Mesure, Citation2015); as well as gait impairments (Hesse et al., Citation1997) in a context where the sensorimotor system is disturbed (Dos Santos, Salazar, Lazarin, and Russo, Citation2015). Proprioceptive deficits appear to negatively influence joint stability, coordination, postural control and motor learning (Afzal, Byun, Oh, and Yoon, Citation2015; Dos Santos, Souza, Desloovere, and Russo, Citation2017). Indeed, during rehabilitation, the repetition of exercises allows progressive correction of postural adjustments through trial and error, using proprioceptive feedback. Thus, inaccurate or imprecise proprioceptive information limits the development of postural adjustments which, in turn, limits motor recovery (Yousif, Cole, Rothwell, and Diedrichsen, Citation2015). A systematic review has highlighted that proprioceptive training (e.g. somato-proprioceptive stimulation, positional sense, and motion detection threshold exercises) improves patients’ motor recovery including those with neurological pathologies (Aman, Elangovan, Yeh, and Konczak, Citation2014). Thus, it is crucial to assess proprioception and postural control from the beginning of the rehabilitation in order to be able to offer adapted exercises early after the stroke.
Proprioceptive disorders in individuals after stroke have several causes: disruption in normal muscle tone and muscle weakness seems to induce a decreased sensitivity of sensors (Yang and Kim, Citation2015); the central nervous system processes the information in an abnormal way following the hemispheric lesion (Son et al., Citation2013); and cognitive deficits (Learman et al., Citation2016). Proprioception includes statesthesia (i.e. joint position sense) and kinesthesia (i.e. sensation of joint movement) (Han et al., Citation2016). After stroke, previous studies highlighted increased positional errors of the limbs in both sides (i.e. contralateral and ipsilateral to the damaged hemisphere) compared to age-matched controls (Lin, Hsu, and Wang, Citation2012; Niessen et al., Citation2008; Son et al., Citation2013; Yalcin et al., Citation2012). In contrast, kinesthesia (i.e. motion detection threshold) is difficult to assess because it requires complex mechanical tools (Niessen et al., Citation2009). While a systematic review has shown the importance of trunk control deficits after stroke (Van Criekinge et al., Citation2019), only four studies have tested trunk position sense in individuals after stroke (Jung, Kim, Chung, and Hwang, Citation2014; Learman et al., Citation2016; Oh and Choi, Citation2017; Ryerson et al., Citation2008). Compared to healthy subjects, individuals with hemiparesis have increased trunk positional errors in the acute (Learman et al., Citation2016) and chronic phases (Ryerson et al., Citation2008). These deficits may correlate with increased postural control disorders and risk of falls (Ryerson et al., Citation2008). The trunk position sense capacities seem to improve after motor imagery training (Oh and Choi, Citation2017) or exercises with body transfers in sitting position (Jung, Kim, Chung, and Hwang, Citation2014). Learman et al. (Citation2016), using an electromagnetic tracking tool, showed a moderate to good intra-day reliability of the trunk position sense in individuals after stroke in acute phase. However, inter-rater reliability was not tested and this tool is not widely available in clinical practice. Previously, for healthy subjects and individuals with low back pain, a simple clinical tests of the trunk position sense had shown good reliability (Enoch et al., Citation2011; Petersen et al., Citation2008). It would therefore be interesting to test the reliability of a simple clinical test of the trunk position sense in stroke context.
Assessing seated postural control is crucial after stroke because this deficit negatively influences: standing balance (van Nes Ij, Nienhuis, Latour, and Geurts, Citation2008); walking (Van Criekinge et al., Citation2019); upper limbs motor function (Lee, Shin, and Song, Citation2016); and functional recovery (Duarte et al., Citation2002). Deficits in trunk control may be associated with trunk muscle weakness (Karthikbabu and Verheyden, Citation2021) and proprioceptive impairments (Ryerson et al., Citation2008). Studies of test reliability in patients after stroke are more numerous for standing than sitting balance position with a predominance of qualitative tests and scales (Bruyneel, Citation2017). In sitting position, a previous study of the Modified Functional Reach Test (MFRT) showed moderate-to-excellent intra-session reliability in ten individuals after stroke (Katz-Leurer et al., Citation2009) without testing test-retest and inter-rater reliability. The MFRT assesses dynamic sitting balance by dissociating the paretic and non-paretic sides displacements, and has the advantage of being simple and quick to perform in clinical practice. Our objective was to assess the reliability (i.e. test-retest and inter-rater) and the validity of a trunk position sense (TPS) and MFRT tests for individuals with post-stroke hemiparesis in subacute phase. Our hypothesis was that both tests have intra-class correlation coefficient (ICC) greater than 0.75 which makes them suitable for use in clinical practice (Portney and Watkins, Citation2009). Nevertheless, the MFRT, which seems to be less sensitive to cognitive deficits, should be more reliable than the TPS. For construct validity the TPS and MFRT tests should be related to trunk strength and Timed Up and Go tests (TUG) that assess balance and functional mobility (Jung, Kim, Chung, and Hwang, Citation2014).
Methods
Participants
The target population was individuals after stroke with hemiparesis aged 50 to 75. This age category represents the typology of patients that is present in neuro-rehabilitation services. The upper limit of 75 years of age was applied to limit bias related to the age of the patients (i.e. cognitive and motor) while preserving the possibility of including enough participants. To be included, individuals had to have had a single stroke episode in the 3 months prior to their recruitment (i.e. subacute phase) and to be medically stable. The choice of the subacute phase is justified by the potential for motor recovery, which is the highest in this phase and which requires an accurate assessment of trunk capacity disorders (Bernhardt et al., Citation2017). In order to ensure that the test instructions were well-understood, individuals had to have a Mini-Mental State Examination (MMSE) score higher than 22 (Learman et al., Citation2016). In addition, they had to be able to sit for 30 seconds independently in order to perform the TPS and MFRT tests (Jung, Kim, Chung, and Hwang, Citation2014). Individuals were excluded if they had other pathologies affecting balance, spinal pathologies or trunk pain, medical complications, and if they had major impairments in understanding the tests.
All individuals were recruited in the neuro-rehabilitation department of the Geneva University Hospitals. Potentially eligible patients were informed orally, and after a reflection period of at least 24 hours, they were given a written and oral explanation before signing the consent form. To encourage free consent, a person independent from the department carried out this step. This study was approved by the cantonal research ethics committees (CCER Geneva – 2018-02026). The study was registered (ClinicalTrials.gov Identifier: NCT04639453).
Raters
To participate in the study, raters had to be a physiotherapist with more than 5 years of experience in neurology. All raters were recruited from the neuro-rehabilitation department of the Geneva University Hospitals.
Procedure
First, a physiotherapist (rater 1) tested each volunteer’s clinical status using : the Balance Assessment in Sitting and Standing Positions test (BASSP) (Huang et al., Citation2016); the maximal isometric force value of the trunk (MiFV) (Karthikbabu and Chakrapani, Citation2017); and the TUG test (Chan, Si Tou, Tse, and Ng, Citation2017).
After this first step, two physiotherapists (rater 1 and rater 2) tested the 32 individuals with stroke in one session in a random order to investigate inter-rater reliability. After resting for 2–4 hours, a second session was conducted by rater 1 to assess test–retest reliability. Raters were blinded to the data collected by the other rater.
Clinical tests
The BASSP test contains four tests: static sitting balance, static standing balance, dynamic sitting balance and dynamic standing balance (Huang et al., Citation2016). The first static assessment consisted in testing the postural reactions of individuals during an external push in four directions (i.e. front, back, left, and right). The static items were rated on a 5-point scale (0 = subject needed an external support; to 4 = subject was stable without aid). For dynamic assessment, three objects were placed on the floor (i.e. front/left, front, and front/right) and the subject had to pick them up. The dynamic items were rated on a 4-point scale (0 = no possibility to pick up the objects; to 3 = objects picked up without external aid). The total scores ranged from 0 to 14. The reliability of the BASSP test is excellent for individuals after a stroke (Huang et al., Citation2016).
Trunk strength was tested in isometric condition with the hand-held dynamometer (MicroFET® 2, Biometrics). This tool, used for the trunk MiFV assessment, is reliable for individuals after stroke (Karthikbabu and Chakrapani, Citation2017). The patient was in a stable sitting position with feet support. The dynamometer was placed on the lateral part of the trunk: the sub-axillary zone to test the paretic side and then the non-paretic side; on the sternum (flexion); and on the T4 vertebra (extension). The individual pushed against the dynamometer for 5 seconds and the MiFV was recorded (N). The rater stabilized his upper limb in the axis of the dynamometer, against the wall. Two trials were performed with a 30 seconds rest between each trial.
The TUG test was used for assessing functional mobility and dynamic postural control (Chan, Si Tou, Tse, and Ng, Citation2017). A cone was placed in front of a chair, 3 m away. The TUG test measures the time (s) taken for an individual to rise from the chair, walk 3 m, half turn around the cone, walk back to the chair, and sit back down. This test showed a good reliability in individuals with hemiparesis after stroke (Chan, Si Tou, Tse, and Ng, Citation2017).
Trunk Position Sense Test (TPS)
The TPS test was performed in sitting position to assess how accurately the subject could re-position his trunk to the initial trunk position, after the trunk had been actively displaced. Test procedure was based on a TPS test reliability study conducted in individuals with low back pain (Enoch et al., Citation2011) and on studies testing the TPS in post-stroke context (Jung, Kim, Chung, and Hwang, Citation2014; Learman et al., Citation2016; Ryerson et al., Citation2008). Participants were in a stable sitting position on a stool with their feet on the floor. The patients’ eyes were closed to avoid visual feedback. The rater guided them into the target trunk position (i.e. 30° flexion checked with the inclinometer). Behind patients, an electric table was positioned to adjust the laser pointer on the T12 vertebra (i.e. anatomical landmark marked with a cross). Individuals were instructed to remember this target position, and then to rotate twice for both the paretic and non-paretic side and then return to the target position. The divergence between laser projection and center of the cross was measured vertically and horizontally. Patients did one training test followed by three successive real trials.
Modified Functional Reach Test (MFRT)
The MFRT was performed following the procedure described in the study by Katz-Leurer et al. (Citation2009). The participant was in a sitting position on a stool, feet flat on the floor, hips and knees at 90° flexion with the non-paretic side of the wall. The non-paretic shoulder was in 90° flexion, elbow extended and fist closed (). The position of the metacarpophalangeal joint was marked on the wall (marker 1). The participant bent forward as far as possible with the arm horizontal while maintaining balance. The metacarpophalangeal joint marker was then noted in this new position (marker 2). The distance between the two landmarks was then recorded.
Then, participants were seated with their back to the wall with upper limbs relaxed. The location of the acromion was noted (marker 1) before individuals leaned as far as possible toward the non-paretic or paretic side by tilting the trunk without destabilizing the pelvis. In this final position, the position of the acromion was noted (marker 2) and the horizontal distance from marker 1 was measured. Individuals performed two training trials before taking three successive measurements in each direction (i.e. anterior, paretic, and non-paretic).
Statistical analysis
Sample size calculation
Sample size calculation used the method of Walter, Eliasziw, and Donner (Citation1998) based on an: acceptable ICC = 0.75 (i.e. threshold for use in clinical practice) (Portney and Watkins, Citation2009); expected ICC = 0.85 based on ICCs obtained in healthy subjects for the TPS (Enoch et al., Citation2011); and a power at 80% and a significance of p = .05. A sample size of 32 participants was necessary.
Data processing
For MiFV of the trunk, data processing consisted of normalizing the data with the subject’s weight according to the following formula:
The values of the MFRT were normalized with the participant’s height:
The different trials were averaged for each test (MFRTnormalized and TPS), for each session (session 1 and session 2) and for each rater (rater 1 and rater 2).
Descriptive statistics
We used descriptive statistics to analyze the demographic variables and the performance of each test. Descriptive statistics consisted of calculating means and standard deviations for quantitative variables, and frequencies for qualitative variables (e.g. gender and stroke side).
Reliability
Inter-rater reliability was assessed by the agreement of data between rater 1 and rater 2 tested by the ICC(2,k) model (Koo and Li, Citation2016). To assess the test–retest reliability (i.e. agreement of data between session 1 and 2), the ICC(3,k) model was applied. An ICC value: below 0.40 was considered poor; between 0.40 and 0.59 moderate; between 0.60 and 0.74 good; and from 0.75 excellent (Cicchetti, Citation1994). To assess the errors in terms of units of measurement, if the ICC was greater than or equal to 0.60, the standard error of measurement (SEM – unit of measurement) was calculated according to this formula (Weir, Citation2005): . SD is the standard deviation of the values obtained for all participants for both raters or both sessions. The minimal detectable change (MDC95%) was calculated as follows:
.
Absolute reliability was investigated using Bland Altman analysis to determine between-session agreement. The 95% limits of agreement (LOA95%) represent two standard deviations (SD) above and below the mean difference (bias) between sessions.
Construct validity
To assess validity, Pearson correlation coefficients (r) were calculated between target tests (MFRTnormalized; TPS) and with clinical tests: MiFVnormalized, BASS and TUG. Results were considered statistically significant when the p value was less than 0.05. A correlation between two tests on the same capacities should fall within the range of 0.4–0.8 (Streiner and Norman, Citation2008). A lower correlation suggests either that the reliability of one of the tests is low, or that they are measuring different phenomena. A correlation higher than 0.8 suggests that both tests might be interchangeable to assess patients in clinical practice. Statistics were performed using SPSS software (SPSS Inc., Chicago, IL, USA).
Results
Thirty-two individuals with hemiparesis after stroke were included in this study (9 women and 23 men, age: 60.93 ± 9.51 years, height: 1.73 ± 0.09 m, weight: 75.17 ± 13.87 kg, body mass index: 24.98 ± 3.28 kg/m2). The mean duration since the stroke event (12 hemorrhagic and 20 ischemic) was 55.64 ± 26.56 days. A majority of hemiparesis cases concerned the left side (22 vs. 10 for the right side). The mean MMSE score was 25.62 ± 2.67/30 points. The results of BASSP, MiFVnormalized, and TUG are described in . Three physiotherapists carried out the tests (age between 30 and 45 years, 2 women and 1 man, > 5 years’ experience in neuro-rehabilitation).
Table 1. Clinical characteristics of included participants (mean ± standard deviation).
Feasibility of the tests
All 32 participants successfully completed both TPS and MFRT tests without adverse events.
Reliability for the TPS
The inter-rater reliability was excellent for vertical error measurement (ICC2,k = 0.75[0.50–0.88], SEM = 0.40 cm and MDC = 1.12 cm) ().
Table 2. Inter and intra-rater reliability for the trunk position sense test.
Bland Altman analysis highlighted a bias between the two raters of −0.28 cm with a LOA95% at 1.44 cm ().
Figure 2. Bland and Altman plot for inter-rater reliability of the trunk position sense test (vertical error). Bias = −0.28 cm, CI95% = 1.44 cm.

When the horizontal error measurement was collected, inter-rater reliability was moderate (ICC2,k = 0.48[0.10–0.75]). Concordance between session 1 and 2 for rater 1 (test–retest reliability) showed moderate reliability for horizontal error (ICC3,k = 0.59[0.12–0.79]) and poor reliability for vertical error measurement (ICC3,k = 0.39[0.22–0.70]) ().
Reliability for the MFRTnormalized
All MFRTnormalized directions highlighted ICC values above 0.75, except for test–retest reliability in non-paretic side displacement (). Inter-rater reliability was excellent for movements in the anterior, paretic and non-paretic directions (0.76 ≤ ICC2,k ≤ 0.90). SEM values ranged from 1.25% to 1.57%. For anterior direction, the mean differences between raters were low (bias = 0.84%) and the LOA95% = 5.79% ().
Figure 3. Bland and Altman plot for inter-rater reliability of the modified functional reach test (anterior movement). Bias = 0.84%, CI95% = 4.19%.

Table 3. Intra and inter-rater reliability for the modified functional reach test (normalized values).
Test–retest reliability for non-paretic movements was lower (ICC3,k = 0.71 [0.41–0.86]) than paretic (ICC3,k = 0.84 [0.68–0.92]) and anterior measures (ICC3,k = 0.91 [0.78–0.96]) (). SEM values ranged from 1.14% to 1.51%. The mean difference between sessions was 1.37% for anterior movements (LOA95% = 5.33%) and 0.47% for paretic side (LOA95% = 4.19%) ().
Construct validity
describes the correlations between MFRTnormalized and TPS values and the following clinical tests: BASSP, MiFVnormalized and TUG. A non-significant correlation was observed with the BASSP. The horizontal error of the TPS was significantly correlated with the non-paretic and posterior MiFVnormalized (r = −0.36, p = .042), and with TUG performance (r = 0.50, p = .004). For MFRTnormalized values, only the forward measures were significantly related to the MiFVnormalized values (0.38 ≤ r ≤ 0.64, p < .030).
Table 4. Pearson correlation coefficients between tests and p value.
Discussion
This study investigated the reliability (test-retest and inter-rater) and the validity of the TPS and MFRTnormalized for individuals with post-stroke hemiparesis. The results partially confirmed our hypotheses. For the TPS, only the inter-rater reliability of the vertical positional error was higher than ICC = 0.75, while reliability was good to excellent for all values of MFRTnormalized. Test performances were partially associated with MiFVnormalized of the trunk and TUG. These contrasting results highlighted the importance of assessing the psychometric qualities of the tests for individuals with stroke before using them in research and clinical practice.
Trunk Position Sense Test (TPS)
The results of the TPS highlighted low inter-rater and test–retest reliability as well as high SEM (MDC). For this simple clinical test with a laser tool, test–retest reliability was lower than the results of Learman et al. (Citation2016) who used an electromagnetic device. Since the positional error is usually less than 4 cm with very small deviations, the tool’s accuracy could influence quality of measurements. Nevertheless, the reliability of the TPS test with simple clinical measurements appears to be excellent in healthy subjects and individuals with low back pain (Enoch et al., Citation2011; Petersen et al., Citation2008). Factors that may influence the quality of the test are mainly the tool used, but also the method. Previously, Goble (Citation2010) observed in healthy subjects divergent results of the joint positional sense test depending on the testing method. Positional errors are less important when the ipsilateral limb is repositioned than when the instruction was given with the contralateral limb. For the TPS, only the ipsilateral repositioning method is applicable. The positional error is therefore influenced by proprioception, the disruption in muscle tone, muscle weakness (Yang and Kim, Citation2015), but also by concentration and memory (Goble, Citation2010). Thus, in individuals after stroke, cognitive disorders can have an impact on the quality of the patient’s performance during the test, which would explain the lower reliability observed in comparison with subjects without cognitive disorders (Enoch et al., Citation2011). When performing the TPS, it is therefore essential to assess patients’ cognitive status to ensure that it is trunk proprioception that is being measured and not memory capacity.
Inter-rater reliability was greater than 0.75 for vertical error. Horizontal error was also recorded, whereas this is not usually the case in other trunk position sense tests (Jung, Kim, Chung, and Hwang, Citation2014; Learman et al., Citation2016; Oh and Choi, Citation2017). A previous study in individuals after stroke in chronic phase suggested distinguishing betweenvertical and horizontal errors (Ryerson et al., Citation2008). The authors observed that horizontal error correlates with the Berg Balance Scale and the Postural Assessment Scale for Stroke, which was not the case for vertical error. Our results seem to confirm that only horizontal error may correlate with MiFVnormalized. However, the reliability of the TPS seems too low to be conclusive and to use this parameter with individuals after stroke.
Modified Functional Reach Test (MFRTnormalized)
Test-retest and inter-rater reliability were sufficient to use MFRTnormalized in all three directions of trunk movement for individuals after stroke in sub-acute phase, except for non-paretic side displacement for test–retest reliability. For the same population, Katz-Leurer et al. (Citation2009) observed higher reliability than our results. They tested the concordance of the results between two successive trials during the same session, whereas we tested two sessions separated by a rest of 2 h to 4 h. This time interval between the two sessions was intended to avoid a memorization bias for the rater and to verify the stability of the test when it is performed twice by the same person on the same participants. For test–retest reliability, the appropriate rest period seems to be 1 day maximum in the subacute stroke phase (Gray, Ivanova, and Garland, Citation2014) and seven days in chronic phase (Gasq et al., Citation2014). However, when tests are performed on the same day, it is not possible to rule out that daily activities in the rehabilitation center and the tests of the study (duration 1h30) impact the test’s quality in comparison with immediate reevaluation.
When assessing test–retest reliability, displacement measurement on the paretic side was more reliable than on the non-paretic side, which corroborates the results of Katz-Leurer et al. (Citation2009) on MFRT and the reliability results obtained during the evaluation of the joint positional sense of the hand (Rinderknecht et al., Citation2018). One possible hypothesis may be that data on the non-paretic side are more homogeneous than on the paretic side, which would weaken the ICC. Nevertheless, in our study, results between two sides were very close and the reliability remains sufficient for both sides.
The MDC values highlight that a change between two measurements greater than 5% is required to be sure that the change in performance is related to a change in the patient.
The results of the construct validity suggest that there is a moderate relationship between the ability to move the trunk forward and MiFVnormalized. These results seem to confirm the link between trunk muscle strength and trunk control abilities after stroke (Karthikbabu and Verheyden, Citation2021); and the influence of abdominal strength training on the increase in forward movement during the functional reach test (Lee et al., Citation2020).
Limitations
For the TPS and MFRTnormalized to be relevant for individuals after stroke, certain abilities are required: autonomous sitting during 30 seconds and MMSE > 22 (Katz-Leurer et al., Citation2009). Therefore, the results are not transposable to individuals with stroke in case of severe deficits. All subjects completed the entire test session. Nevertheless, despite strict eligibility criteria, some participants had difficulties in performing the tests, mainly due to fatigue or difficulty in understanding the instructions. This affected four of the 32 participants included. In case of failure of doubt about the quality of the test, the trial was repeated. For the TPS, the values were better in the second session. Given that the test conditions were the same, it is likely that a learning effect influenced performance despite the first trial not being taken into consideration for the results. Raters were blinded to the other rater’s measurements, but not to their own measurements. This methodological choice was made in order to respect the usual conditions of testing in clinical practice. To limit the risk of bias, the raters had homogeneous characteristics and were specialists in neuro-rehabilitation.
Conclusion
Reliability and validity of trunk tests is variable, depending on the evaluated test and the target population. In individuals after stroke, the reliability of the TPS seems too low to be conclusive and to use this parameter with individuals after stroke in clinical practice and research. In contrast, the MFRTnormalized demonstrated a good to excellent test-retest and inter-rater reliability. Construct validity analysis revealed a moderate correlation of MFRT anterior displacement with the trunk muscle strength. This test may be used as a screening test for sitting postural control taken into account the limitations with the validity.
Disclosure statement
No potential conflict of interest was reported by the authors.
Additional information
Funding
References
- Afzal MR, Byun HY, Oh M, Yoon J 2015 Effects of kinesthetic haptic feedback on standing stability of young healthy subjects and stroke patients. Journal of NeuroEngineering and Rehabilitation 12: 27. 10.1186/s12984-015-0020-x.
- Aman JE, Elangovan N, Yeh IL, Konczak J 2014 The effectiveness of proprioceptive training for improving motor function: A systematic review. Frontiers in Human Neuroscience 8: 1075. 10.3389/fnhum.2014.01075.
- Bernhardt J, Hayward KS, Kwakkel G, Ward NS, Wolf SL, Borschmann K, Krakauer JW, Boyd LA, Carmichael ST, Corbett D, et al. 2017 Agreed definitions and a shared vision for new standards in stroke recovery research: The stroke recovery and rehabilitation roundtable taskforce. Neurorehabilitation and Neural Repair 31: 793–799. 10.1177/1545968317732668.
- Bruyneel AV 2017 Tests cliniques d’évaluation de l’équilibre assis et des tâches de transfert pour les patients atteints d’une hémiparésie secondaire à un accident vasculaire cérébral: Revue de littérature [Clinical tests for seated balance and transfer task assessment in patients with hemiparesis post-stroke: Literature review. Kinésithérapie, La Revue 17: 14–23.
- Chan PP, Si Tou JI, Tse MM, Ng SS 2017 Reliability and validity of the Timed Up and Go Test with a motor task in people with chronic stroke. Archives of Physical Medicine and Rehabilitation 98: 2213–2220. 10.1016/j.apmr.2017.03.008.
- Cicchetti DV 1994 Guidelines, criteria, and rules of thumb for evaluating normed and standardized assessment instruments in psychology. Psychological Assessment 6: 284–290. 10.1037/1040-3590.6.4.284.
- Dos Santos GL, Salazar LF, Lazarin AC, Russo TL 2015 Joint position sense is bilaterally reduced for shoulder abduction and flexion in chronic hemiparetic individuals. Topics in Stroke Rehabilitation 22: 271–280.
- Dos Santos GL, Souza MB, Desloovere K, Russo TL 2017 Elastic tape improved shoulder joint position sense in chronic hemiparetic subjects: A randomized sham-controlled crossover study. PloS One 12: e0170368. 10.1371/journal.pone.0170368.
- Duarte E, Marco E, Muniesa JM, Belmonte R, Diaz P, Tejero M, Escalada F 2002 Trunk control test as a functional predictor in stroke patients. Journal of Rehabilitation Medicine 34: 267–272. 10.1080/165019702760390356.
- Duclos NC, Maynard L, Abbas D, Mesure S 2015 Hemispheric specificity for proprioception: Postural control of standing following right or left hemisphere damage during ankle tendon vibration. Brain Research 1625: 159–170. 10.1016/j.brainres.2015.08.043.
- Enoch F, Kjaer P, Elkjaer A, Remvig L, Juul-Kristensen B 2011 Inter-examiner reproducibility of tests for lumbar motor control. BMC Musculoskeletal Disorders 12: 114. 10.1186/1471-2474-12-114.
- Gasq D, Labrunée M, Amarantini D, Dupui P, Montoya R, Marque P 2014 Between-day reliability of centre of pressure measures for balance assessment in hemiplegic stroke patients. Journal of Neuroengineering and Rehabilitation 11: 39. 10.1186/1743-0003-11-39.
- Goble DJ 2010 Proprioceptive acuity assessment via joint position matching: From basic science to general practice. Physical Therapy 90: 1176–1184. 10.2522/ptj.20090399.
- Gray VL, Ivanova TD, Garland S 2014 Reliability of center of pressure measures within and between sessions in individuals post-stroke and healthy controls. Gait and Posture 40: 198–203. 10.1016/j.gaitpost.2014.03.191.
- Han J, Waddington G, Adams R, Anson J, Liu Y 2016 Assessing proprioception: A critical review of methods. Journal of Sport and Health Science 5: 80–90. 10.1016/j.jshs.2014.10.004.
- Hesse S, Reiter F, Jahnke M, Dawson M, Sarkodie-Gyan T, Mauritz KH 1997 Asymmetry of gait initiation in hemiparetic stroke subjects. Archives of Physical Medicine and Rehabilitation 78: 719–724. 10.1016/S0003-9993(97)90079-4.
- Huang CY, Song CY, Chen KL, Chen Y, Lu W, Hsueh I, Hsieh C 2016 Validation and establishment of an interval-level measure of the balance assessment in sitting and standing positions in patients with stroke. Archives of Physical Medicine and Rehabilitation 97: 938–946. 10.1016/j.apmr.2016.01.014.
- Jung K, Kim Y, Chung Y, Hwang S 2014 Weight-shift training improves trunk control, proprio-ception, and balance in patients with chronic hemiparetic stroke. Tohoku Journal of Experimental Medicine 232: 195–199. 10.1620/tjem.232.195.
- Karthikbabu S, Chakrapani M 2017 Hand-held dynamometer is a reliable tool to measure trunk muscle strength in chronic stroke. Journal of Clinical and Diagnostic Research 11: YC09–YC12.
- Karthikbabu S, Verheyden G 2021 Relationship between trunk control, core muscle strength and balance confidence in community-dwelling patients with chronic stroke. Topics in Stroke Rehabilitation 28: 88–95.
- Katz-Leurer M, Fisher I, Neeb M, Schwartz I, Carmeli E 2009 Reliability and validity of the modified functional reach test at the sub-acute stage post-stroke. Disability and Rehabilitation 31: 243–248.
- Koo TK, Li MY 2016 A Guideline of selecting and reporting intraclass correlation coefficients for reliability research. Journal of Chiropractic Medicine 15: 155–163.
- Learman KE, Benedict JA, Ellis AR, Neal AR, Wright JA, Landgraff NC 2016 An exploration of trunk reposition error in subjects with acute stroke: An observational design. Topics in Stroke Rehabilitation 23: 200–207.
- Lee MM, Shin DC, Song C 2016 Canoe game-based virtual reality training to improve trunk postural stability, balance, and upper limb motor function in subacute stroke patients: A randomized controlled pilot study. Journal of Physical Therapy Science 28: 2019–2024.
- Lee J, Jeon J, Lee D, Hong J, Yu J, Kim J 2020 Effect of trunk stabilization exercise on abdominal muscle thickness, balance and gait abilities of patients with hemiplegic stroke: A randomized controlled trial. NeuroRehabilitation 47: 435–442.
- Lin SI, Hsu LJ, Wang HC 2012 Effects of ankle proprioceptive interference on locomotion after stroke. Archives of Physical Medicine and Rehabilitation 93: 1027–1033.
- Morishita M, Amimoto K, Matsuda T, Arai Y, Yamada R, Baba T 2009 Analysis of dynamic sitting balance on the Independence of gait in hemiparetic patients. Gait and Posture 29: 530–534.
- Niessen MH, Veeger D, Koppe PA, Konijnenbelt MH, van Dieën J, Janssen TW 2008 Proprioception of the shoulder after stroke. Archives of Physical Medicine and Rehabilitation 89: 333–338.
- Niessen MH, Veeger DH, Meskers CG, Koppe PA, Konijnenbelt MH, Janssen TW 2009 Relationship among shoulder proprioception, kinematics, and pain after stroke. Archives of Physical Medicine and Rehabilitation 90: 1557–1564.
- Oh DS, Choi JD 2017 The effect of motor imagery training for trunk movements on trunk muscle control and proprioception in stroke patients. Journal of Physical Therapy Science 29: 1224–1228.
- Petersen CM, Zimmermann CL, Cope S, Bulow ME, Ewers-Panveno E 2008 A new measurement method for spine reposition sense. Journal of Neuroengineering and Rehabilitation 5: 9.
- Portney LG, and Watkins MP 2009 Foundations of clinical research: Applications to practice 3rd. Upper Saddle River, NJ: Pearson/Prentice Hall.
- Potter K, Fulk GD, Salem Y, Sullivan J 2011 Outcome measures in neurological physical therapy practice: Part I. Making Sound Decisions. Journal of Neurologic Physical Therapy 35: 57–64.
- Rinderknecht MD, Lambercy O, Raible V, Büsching I, Sehle A, Liepert J, Gassert R 2018 Reliability, validity, and clinical feasibility of a rapid and objective assessment of post-stroke deficits in hand proprioception. Journal of Neuroengineering and Rehabilitation 15: 47.
- Ryerson S, Byl NN, Brown D, Wong R, Hidler JM 2008 Altered trunk position sense and its relation to balance functions in people post-stroke. Journal of Neurologic Physical Therapy 32: 14–20.
- Son SM, Kwon YH, Lee N, Nam S, Kim K 2013 Deficits of movement accuracy and proprioceptive sense in the ipsi-lesional upper limb of patients with hemiparetic stroke. Journal of Physical Therapy Science 25: 567–569.
- Streiner DL, Norman GR 2008 Health measurement scales a practical guide to their development and use. Oxford: Oxford University Press.
- Van Criekinge T, Truijen S, Schröder J, Maebe Z, Blanckaert K, van der WC, Vink M, Saeys W 2019 The effectiveness of trunk training on trunk control, sitting and standing balance and mobility post-stroke: A systematic review and meta-analysis. Clinical Rehabilitation 33: 992–1002.
- van Nes Ij, Nienhuis B, Latour H, Geurts AC 2008 Posturographic assessment of sitting balance recovery in the subacute phase of stroke. Gait and Posture 28: 507–512.
- Walter SD, Eliasziw M, Donner A 1998 Sample size and optimal designs for reliability studies. Statistics in Medicine 17: 101–110.
- Weir JP 2005 Quantifying test-retest reliability using the intraclass correlation coefficient and the SEM. Journal of Strength and Conditioning Research 19: 231–240.
- Yalcin E, Akyuz M, Onder B, Kurtaran A, Buyukvural S, Ozbudak Demir S 2012 Position sense of the hemiparetic and non-hemiparetic ankle after stroke: Is the non-hemiparetic ankle also affected? European Neurology 68: 294–299.
- Yang JM, Kim SY 2015 Correlation of knee proprioception with muscle strength and spasticity in stroke patients. Journal of Physical Therapy Science 27: 2705–2708.
- Yousif N, Cole J, Rothwell J, Diedrichsen J 2015 Proprioception in motor learning: Lessons from a deafferented subject. Experimental Brain Research 233: 2449–2459.