Abstract
Colonial volvocacean algae engage in two types of sexual reproduction: isogamy and anisogamy/oogamy with sperm packets. This difference is an important generic diagnosis within the Volvocaceae. Although Yamagishiella differs from the anisogamous genus Eudorina in its isogamous sexual reproduction, the vegetative morphology and asexual reproduction characteristics of the two genera are indistinguishable, especially between Eudorina unicocca G. M. Smith and Yamagishiella unicocca (Rayburn et Starr) Nozaki. We re-examined morphological characteristics of E. unicocca and related species, using multiple strains of E. unicocca and Y. unicocca and molecular phylogenetic analyses. Strains from two Japanese lakes, which produced aplanospores and were solely asexual, could be assigned to either E. unicocca or Y. unicocca, based on traditional morphological diagnoses. However, a new morphological diagnosis (the difference in the distribution and number of contractile vacuoles on the cell surface) and molecular phylogenetic analyses demonstrated that all were E. unicocca. Furthermore, E. unicocca can be divided into two species on the basis of the presence or absence of individual cellular sheaths in the colonial gelatinous matrix, which are observable with methylene blue staining. These two species, E. peripheralis (Goldstein) T. K. Yamada stat. nov. (= E. unicocca var. peripherialis Goldstein) and E. unicocca (including the Japanese aplanosporic strains), formed two robust monophyletic groups, based on chloroplast gene sequences for the large RuBisCO subunit and internal transcribed spacer regions of nuclear ribosomal DNA.
Introduction
Eudorina Ehrenberg is a cosmopolitan volvocacean genus, comprising about eight species (Goldstein, Citation1964; Nozaki & Krientz, Citation2001). The genus is distinguished from Pandorina Bory de St. Vincent, Yamagishiella Nozaki and Pleodorina Shaw by the presence of cellular envelopes in the gelatinous (extracellular) matrix, anisogamous sexual reproduction with sperm packets and the absence of obligately somatic cells (Nozaki et al., Citation1989; Nozaki & Kuroiwa, Citation1992; Nozaki & Ito, Citation1994). However, in recent molecular phylogenetic analyses of multiple chloroplast genes (Nozaki et al., Citation2000; Nozaki, Citation2003), Eudorina forms a non-monophyletic, basal lineage within the clade containing anisogamous/oogamous members of the colonial Volvocales (Volvox, excluding Volvox sect. Volvox; Pleodorina; and Eudorina).
Yamagishiella Nozaki in Nozaki & Kuroiwa (Citation1992) is a monotypic genus containing Y. unicocca (Rayburn et Starr) Nozaki, which was originally described as Pandorina unicocca Rayburn et Starr (1974). This genus is distinguished from Pandorina by its cellular envelopes and 32-celled colonies (Nozaki & Kuroiwa, Citation1992). Although Yamagishiella differs from Eudorina in its isogamous sexual reproduction, the vegetative morphology and asexual reproduction characteristics of the two genera are indistinguishable (Nozaki & Kuroiwa, Citation1992; Nozaki & Ito, Citation1994). For example, like Y. unicocca (Rayburn & Starr, Citation1974), Eudorina unicocca G. M. Smith is recognized by the presence of a single, basal pyrenoid in the chloroplast of vegetative cells (Smith, Citation1930; Goldstein, Citation1964). Thus, E. unicocca cannot be distinguished from Y. unicocca without molecular markers when the mode of sexual reproduction is unknown (Nozaki et al., Citation1998).
Goldstein (Citation1964) established a species-level taxonomic system for the genus Eudorina, based on comparative morphological observations of cultured material. However, he did not observe sexual reproduction in several strains of E. unicocca that produced parthenospores or aplanospores (Goldstein, Citation1964). These ‘aplanosporic’ strains are impossible to re-identify, as they were not maintained in culture collections (Starr & Zeikus, Citation1993; Kasai et al., Citation2004). Therefore, new strains of the aplanosporic algae and new generic diagnoses of Eudorina and Yamagishiella are needed to establish a clear morphological distinction between the two genera and a straightforward identification system. We re-examined the morphological characteristics of E. unicocca and related species by examining many strains that could be identified as E. unicocca or Y. unicocca by their vegetative morphology. Several strains originating from Japanese lakes produced aplanospores and were solely asexual, but a new morphological diagnosis and molecular phylogenetic analyses demonstrated their identity as E. unicocca, morphologically distinguishable from E. peripheralis (Goldstein) T. K. Yamada stat. nov. (= E. unicocca var. peripheralis Goldstein).
Materials and methods
Cultures
We isolated aplanosporic strains from water samples collected from Lake Tsukui (TKI-C-1, -2 and -3), Tsukui-machi, Sagamihara, Kanagawa Prefecture, Japan, in July 2005 (water temperature 24.7°C; pH>8.4) and Lake Sagami (990601-IE-3, -4, -5 and -6), Fujino-machi, Tsukui-gun, Kanagawa Prefecture, Japan, in June 1999 (water temperature 20.0°C; pH 8.3). Three Y. unicocca strains (Hasu-1, -2 and -4) were isolated from soil samples collected from a lotus paddy field in Noumi-cho, Etajima, Hiroshima Prefecture, Japan, in August 2004. Using the pipette-washing method (Pringsheim, Citation1946), clonal cultures were established directly from the water sample or from Petri dishes (90×20mm) in which a small amount (∼0.5g) of dried soil had been wetted with distilled water. Four strains of E. unicocca (NIES-724, -725, -726 and UTEX 1221; Goldstein Citation1964), E. elegans NIES-717, E. cylindrica Korshikov NIES-722, E. illinoisensis (Kofoid) Pascher NIES-723, and five strains of Y. unicocca (NIES-578, -666, -762, -870 and -872) were obtained from culture collections (National Institute for Environmental Studies, Japan, NIES, or the Culture Collection of Algae at the University of Texas at Austin, UTEX) (Kasai et al., Citation2004; Starr & Zeikus, Citation1993; ). The cultures were grown in screw-cap tubes (18×150mm) containing about 11ml AF-6 medium (Kato, Citation1982), modified by the elimination of CaCO3 and addition of 400mgl−1 MES (Kasai et al., Citation2004). The cultures were cultivated at about 20–25°C, on a 10:14 h light–dark cycle, under cool-white fluorescent lamps at 150–200µmolm−2s−1 intensity.
Table 1. List of rbcL gene and ITS sequences used in this study.
Light microscopy
To observe vegetative morphology and asexual reproduction, about 0.5ml of each actively growing culture was inoculated into fresh medium every 3 to 12 days. To induce sexual reproduction, 11ml of actively growing culture were concentrated to 0.3–0.5ml by centrifugation. The concentrated cultures of two complementary mating types (e.g., Y. unicocca Hasu-1 and -4, ) were mixed and added to 1.5ml of nitrogen-deficient AFM medium (Nakazawa et al., Citation2001) in watch glasses (60 mm diameter) supported on glass depressions in Petri dishes. To minimize evaporation, about 0.5ml of distilled water was added to the bottom of the Petri dishes. The Petri dishes were cultured under the usual conditions (14:10-h light–dark cycle; 20–25°C). For aplanospore formation, a concentrated culture (0.3–0.5ml) of a single strain was mixed with 1.5–2.0ml AFM medium and cultured as described above. In order to examine individual cellular sheaths of the gelatinous matrix of the vegetative colonies, about 10µl of the cultured material were mixed with 2–5µl 0.002% (w/v in distilled water) methylene blue (1B-429 Methylene Blue med., Puriss., WALDECK GmbH & Co Division Chroma, Münster, Germany). Light microscopy was carried out using an OLYMPUS BX60 microscope (KS OLYMPUS, Tokyo, Japan), equipped with Nomarski interference optics.
Molecular phylogenetic analyses
The protocol of Fawley & Fawley (Citation2004) was used to prepare the total DNA of seven strains (), with some modifications (see Nakada & Nozaki, Citation2007). The method for sequencing the chloroplast gene (rbcL) for the large subunit of RuBisCO of two strains of Y. unicocca (Hasu-1 and NIES-870), E. unicocca UTEX 1221 and two aplanosporic strains (TKI-C-2 and 990601-IE-5) was essentially identical to that described previously (Nozaki et al., Citation1995, 1997, 2000, 2002). The region sequenced corresponded to rbcL positions 31–1159 in Chlorella vulgaris Beijerinck (Yoshinaga et al., Citation1988; Wakasuagi et al., Citation1997). For phylogenetic analyses, identical sequences were treated as a single operational taxonomic unit (OTU). The coding regions (1,129bp) of the sequences were aligned by Clustal X (Thompson et al., Citation1997), including 14 other Eudorina strains, six other Yamagishiella strains, 17 Volvox strains, five Pleodorina strains, one Platydorina strain and two Pandorina strains (). From this alignment, a distance matrix was calculated by applying the two-parameter method (Kimura, Citation1980) in Clustal X. A phylogenetic tree was constructed using the neighbor-joining (NJ) algorithm (Saitou & Nei, Citation1987) with Clustal X, and the robustness of the resulting lineages was tested using bootstrap analysis (Felsenstein, Citation1985) with 1,000 replications. Based on the alignment data, a maximum parsimony (MP) analysis (including bootstrap analysis based on 1,000 replications of the general heuristic search using the tree-bisection-reconnection branch-swapping algorithm) was performed using PAUP* 4.0b10 (Swofford, Citation2003). In addition, the internal transcribed spacer (ITS) regions of nuclear ribosomal DNA from three strains of E. unicocca (NIES-724, NIES-726 and UTEX 1221) and two aplanosporic strains (TKI-C-2 and 990601-IE-5) were sequenced, as described by Sakayama et al. (Citation2004), except for the primers used for polymerase chain reaction (PCR) and sequencing. The primers for the ITS regions were those of Coleman et al. (Citation1994), with newly designed PCR primers (ITS-F1: 5′ GTGCTGCCGTACAGCCCTCAGTCG 3′; ITS F2: 5′ AACTAACCAACCAACTCCAAACCA 3′; ITS R3: 5′ GCCTCGAGCGCAATTTGCGT TCAA3′; and ITS R4: 5′ AGTGAGTATTAACCCGACGCTGAG 3′). The coding regions were aligned with those of E. cylindrica (NIES-722) and Pleodorina thompsonii Ott et al. (UTEX 2804), using Clustal X, taking account of their secondary structure (Mai & Coleman, Citation1997; Coleman, Citation2002). The sequence alignment is available upon request from the corresponding author (H.N.). After removing gaps in the ingroup OTUs, the data matrix (548bp) was subjected to the same phylogenetic analyses as for rbcL, described above. Based on recent phylogenetic results for the colonial Volvocales (Nozaki et al., Citation2000, 2006; Nozaki, Citation2003), two strains of Pandorina were designated as the outgroup for the rbcL phylogeny, and E. cylindrica and P. thompsonii as the outgroups for the ITS phylogeny.
Results and discussion
Morphology of aplanosporic strains from Japan
The vegetative colonies of strains isolated from Japanese lakes () were ovoid or ellipsoidal in shape, up to 160µm long, containing 16 or 32 cells of almost identical size embedded in the periphery of the gelatinous matrix (). Cells were broadly ovoid or subspherical, measuring up to 25µm in diameter (). Each cell had two equal flagella, a massive cup-shaped chloroplast, a stigma and a nucleus. In addition to the two apical contractile vacuoles, several (up to nine) contractile vacuoles were distributed over the entire surface of the protoplast (). The size of the stigmata gradually diminished from the anterior to posterior pole of the colony. The chloroplast had a single large pyrenoid at the bottom (). No additional pyrenoids developed around the large pyrenoid, even in mature cells (). When stained with methylene blue, the gelatinous matrix of the colony revealed individual cellular sheaths that were rectangular in surface view ().
Figs. 1–7 Light microscopy of an aplanosporic strain of Eudorina unicocca originating from Lake Tsukui, Kanagawa, Japan (TKI-C-2). . Surface view of 32-celled vegetative colony. Note a single, basal pyrenoid (P) in the chloroplast of each cell. . Optical section of 32-celled vegetative colony. Arrowheads indicate colonial envelope surrounding whole colony. . Surface view of vegetative cell, showing contractile vacuoles (arrowheads) distributed in the protoplast surface. . Optical section of vegetative cell, showing a single large pyrenoid (P) in the chloroplast, and contractile vacuoles (arrowheads) distributed in the protoplast surface. . Daughter colony formation in asexual reproduction. Note each cell is enclosed by a transparent vesicle or cellular envelope (arrows) within the parental gelatinous matrix (arrowheads). . Vegetative colony stained with methylene blue. Individual cellular sheaths of the colonial gelatinous matrix (asterisks) are apparent. . Mature aplanospores 27 days after transferring into nitrogen-deficient medium.
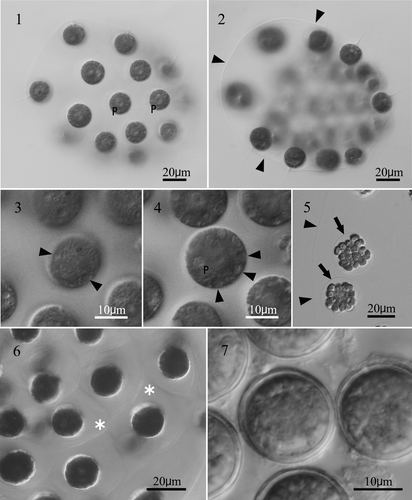
Asexual reproduction was accomplished by autocolony formation. Each cell divided four or five times successively to form a 16- or 32-celled plate, which inverted to form a compact spheroidal daughter colony within a transparent vesicle (i.e., cellular envelope; Nozaki & Kuroiwa Citation1992) in the parental gelatinous matrix ().
Sexual reproduction did not occur, even when all possible combinations of the seven strains were mixed in nitrogen-deficient AFM medium. In each strain, the cells lost their flagella and secreted a heavy cell wall within the colonial envelope 48–72 hours after transference to AFM medium. The aplanospores were spherical, subsequently turned reddish-brown and measured 17–25µm in diameter (). Germination of the aplanospores was not observed.
The presence of a 32-cell, ovoid-to-ellipsoidal, colony without somatic cells, cellular envelopes and a single large pyrenoid in the chloroplast (, ) identifies this alga as E. unicocca or Y. unicocca (Goldstein, Citation1964, Nozaki et al., Citation1998). Because sexual reproduction did not occur, based on morphology alone, we could not determine whether the alga belonged to Eudorina or Yamagishiella. However, the number and distribution of contractile vacuoles in the vegetative cells of the aplanosporic alga appeared to differ from that in Yamagishiella. According to Rayburn & Starr (Citation1974) and Nozaki et al. (Citation1998), the latter only has two contractile vacuoles near the base of the flagella. In contrast, Goldstein (Citation1964) described “two apical and generally several randomly-distributed contractile vacuoles” for Eudorina. However, distinguishing Yamagishiella vegetative cells by the presence of the two contractile vacuoles (Rayburn & Starr, Citation1974; Nozaki et al., Citation1998) appears unreliable, as Smith (Citation1950) reported two contractile vacuoles near the base of the flagella for Eudorina. The individual cellular sheaths in the colonial gelatinous matrix, revealed by methylene blue staining (, ), have not been reported for either E. unicocca or Y. unicocca (Smith, Citation1930; Goldstein, Citation1964; Rayburn & Starr, Citation1974; Nozaki et al., Citation1998).
Molecular phylogenetic analyses of rbcL and nuclear rDNA ITS sequences
Based on phylogenetic analyses of rbcL sequences, two aplanosporic strains (TKI-C-2 and 990601-IE-5) and four E. unicocca strains (NIES-724, -725, -726 and UTEX 1221) formed a robust monophyletic group (with 100% bootstrap values for both NJ and MP analyses) that was firmly positioned within the anisogamous/oogamous members of the Volvocaceae (Volvox, excluding Volvox sect. Volvox; Pleodorina; and Eudorina), but distantly separated from the clade comprising eight Yamagishiella strains (). Furthermore, the latter group was subdivided into two well-resolved clades, with 87–92% and 92–100% bootstrap values for the NJ and MP analyses, respectively. One clade comprised two aplanosporic strains and NIES-724, and the other contained NIES-725, NIES-726 and UTEX 1221.
Fig. 9. Neighbor-joining (NJ) tree based on rbcL genes from 17 strains of Eudorina species, 17 strains of Volvox, five strains of Pleodorina, one strain of Platydorina, eight strains of Yamagishiella, and two strains of Pandorina (). Branch lengths are propotional to Kimura (Citation1980) distances, which are indicated by the scale bar besides the tree. Numbers above or below the branches represent 50% or more bootstrap values based on 1,000 replications of the NJ or maximum parsimony analyses, respectively.
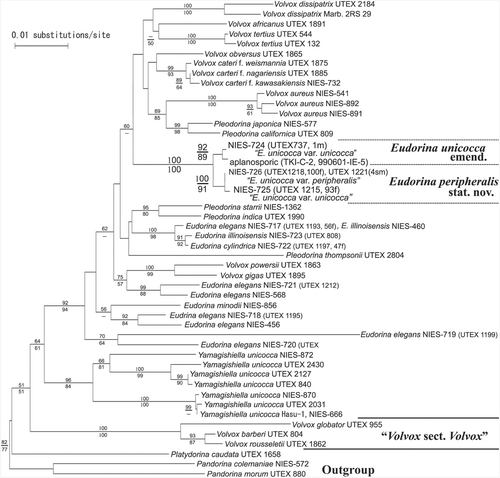
Phylogenetic analyses of the ITS sequences showed two aplanosporic strains (TKI-C-2, 990601-IE-5) and four E. unicocca strains (NIES-724, -725, -726 and UTEX 1221) forming two robust monophyletic groups (), identical to the clades resolved in the rbcL gene phylogeny ().
Fig. 10. Neighbor-joining (NJ) tree based on ITS sequences from 6 strains of Eudorina species and ones strain of Pleodorina (). Branch lengths are proportional to Kimura (Citation1980) distances, which are indicated by the scale bar besides the tree. Numbers above or below the branches represent 50% or more bootstrap values based on 1,000 replications of the NJ or maximum parsimony analyses, respectively.
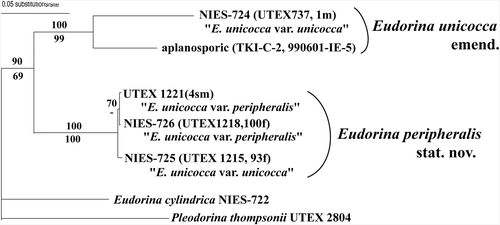
Thus, our rbcL phylogeny places the aplanosporic strains in Eudorina, based on their phylogenetic position within the anisogamous/oogamous Volvocaceae members (). Furthermore, these strains can be classified as E. unicocca, on the basis of the single basal chloroplast pyrenoid in mature vegetative cells, the ovoid or ellipsoidal colony form (; Goldstein Citation1964) and the sister phylogenetic relationship to NIES-724 that identified (Goldstein, Citation1964) as E. unicocca var. unicocca (, ; ). As no additional small pyrenoids developed around the basal pyrenoid in this aplanosporic alga (), we identified it as E. unicocca var. unicocca, according to Goldstein's taxonomic system (1964).
Morphological differences in vegetative colonies of Eudorina and Yamagishiella
To elucidate the taxonomic significance of differences in the number and distribution of contractile vacuoles in vegetative cell protoplasts, we performed comparative morphological observations of four strains of E. unicocca (NIES-724, -725, -726 and UTEX 1221), E. elegans (NIES-717), E. cylindrica (NIES-722), E. illinoisensis (NIES-723) and five strains of Y. unicocca (NIES-578, -666, -762, -870 and -872). All Eudorina strains had several contractile vacuoles distributed over the entire surface of vegetative colony protoplasts (), whereas Yamagishiella strains only had two contractile vacuoles near the base of the flagella ().
Figs 11–18. Vegetative cells of Eudorina and Yamagoshiella showing two types of distribution of contractile vacuoles in the protoplasts. . Eudorina. Note each cell has several contractile vacuoles (arrowheads) distributed in the protoplast surface. , give surface views of protoplasts and , provide optical sections. , . E. unicocca NIES-724 (UTEX 737, 1m). . E. peripheralis NIES-725 (UTEX 1215, 93f). . E. illinoisensis NIES-723. . Yamagishiella unicocca. Note only two anterior contractile vacuoles (arrowheads) in the protoplast surface. , , are surface views of anterior regions of protoplasts and an optical section of the protoplast. No contractile vacuoles are visible in the periphery. , . Hasu-2. . NIES-762. . NIES-870.
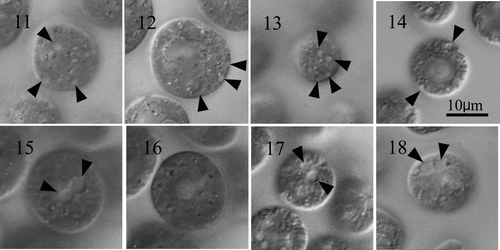
Several or many contractile vacuoles throughout the surface of vegetative cell protoplasts have been observed in E. illinoisensis (NIES-459, NIES-460) (Nozaki, Citation1986), E. minodii (Chodat) Nozaki et Krienitz (NIES-856) (Nozaki & Krienitz, Citation2001) and E. elegans strains (NIES-456, -457 and -458) (Nozaki, Citation1984, 1985). Thus, based on the number and the distribution of contractile vacuoles, Eudorina and Yamagishiella can be clearly distinguished, indicating that the phylogenetic difference between these two genera (Nozaki et al., Citation1998; Nozaki, Citation2003; ) reflects not only modes of sexual reproduction (Nozaki & Kuroiwa, Citation1992) but also basic vegetative morphology.
Reclassification of Eudorina unicocca based on morphological and molecular data
To elucidate the taxonomic significance of the individual cellular sheaths in the colonial gelatinous matrix observed in the aplanosporic strains of E. unicocca (, ), we examined the gelatinous matrices using methylene blue on four other strains of E. unicocca (NIES-724, -725, -726 and UTEX 1221) that formed a monophyletic group with the aplanosporic strains in our rbcL phylogenetic analyses (). The stain revealed individual cellular sheaths in the colonial gelatinous matrix of NIES-724 (), as well as our aplanosporic strains (, ). However, NIES-725, NIES-726 and UTEX 1221 did not have this structure (). Based on our phylogenetic analyses, the six strains of E. unicocca form two clades, one containing aplanosporic strains from two Japanese lakes and NIES-724, and the other comprising NIES-725, NIES-726 and UTEX 1221 (, ). Thus, the presence or absence of individual cellular sheaths in the colonial gelatinous matrix distinguishes these groups within E. unicocca. Goldstein (Citation1964) reported almost complete sexual isolation between heterothallic strains belonging to these two groups. In Pleodorina, the presence or absence of similar individual cellular sheaths is a taxonomic criterion for distinguishing species (Nozaki et al. Citation1989, 2006). Thus, the two groups within E. unicocca could be classified as two species. However, both lineages contain strains (Japanese aplanosporic strains, NIES-724 and NIES-725) that contain only a single, basal pyrenoid in mature vegetative cell chloroplasts (i.e., E. unicocca var. unicocca, Goldstein, Citation1964) (), as in the original description (Smith, Citation1930). Therefore, either could be designated as E. unicocca. Here, we designate the monophyletic group comprising strains of E. unicocca var. unicocca sensu Goldstein (Citation1964) as E. unicocca, and designate the other group, including E. unicocca var. peripheralis (NIES-726 and UTEX 1221; ), as E. peripheralis (, ). Therefore, E. unicocca, as emended here, is characterized by having a single, basal pyrenoid in mature vegetative cell chloroplasts, ovoid to ellipsoidal colonies and individual cellular sheaths in the gelatinous matrix of the colony. Eudorina peripheralis differs from E. unicocca in lacking individual sheaths. According to Goldstein (Citation1964), E. unicocca var. peripheralis is characterized by having small pyrenoids around the large basal pyrenoid in mature vegetative cells. However, E. peripheralis includes NIES-725 (, ), which Goldstein (Citation1964) identified as E. unicocca var. unicocca. Therefore, the presence or absence of small pyrenoids in mature cells represents intraspecific variability within E. peripheralis.
Figs. 19–22. Vegetative colonies of Eudorina unicocca and E. peripheralis stained with methylene blue. . E. unicocca NIES-724 (UTEX 737,1m), showing individual cellular sheaths (asterisk). . E. peripheralis NIES-725 (UTEX 1215, 93f). . E. peripheralis NIES-726 (UTEX 1218, 100f). . E. peripheralis UTEX 1221 (4sm). Scale bars: 10µm.
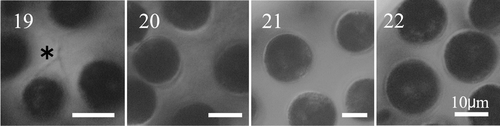
Taxonomic treatment
Eudorina peripheralis (Goldstein) T. K. Yamada stat. nov. (, )
BASIONYM: Eudorina unicocca G. M. Smith var. peripheralis Goldstein, Citation1964, J. Protozool. 11: 324, .
Acknowledgements
We wish to thank Suzue Arii (The Kanagawa Prefectural Water Works, Tanigahara, Japan) for her kind help collecting the water samples in Lake Tsukui. This work was supported by Grant-in-Aid for Creative Scientific Research (No. 16GS0304 to HN) and by Grant-in-Aid for Scientific Research (No. 17370087 to HN) from the Ministry of Education, Culture, Sports, Science and Technology, Japan.
References
- Coleman , AW . 2002 . Comparison of Eudorina/Pleodorina ITS sequences of isolates from nature with those from experimental hybrids . Am. J. Bot. , 89 : 1523 – 1530 .
- Coleman , AW , Suarez , A and Goff , LJ . 1994 . Molecular delineation of species and syngens in volvocalean green algae (Chlorophyta) . J. Phycol. , 30 : 80 – 90 .
- Fawley , MW and Fawley , KP . 2004 . A simple and rapid technique for the isolation of DNA from microalgae . J. Phycol. , 40 : 223 – 225 .
- Felsenstein , J . 1985 . Confidence limits on phylogenies: an approach using the bootstrap . Evolution , 39 : 783 – 791 .
- Goldstein , M . 1964 . Speciation and mating behavior in Eudorina . J. Protozool. , 11 : 317 – 334 .
- Kasai , F , Kawachi , M , Erata , M and Watanabe , MM . 2004 . NIES-Collection. List of Strains. Microalgae and Protozoa. , 7th ed. , Tsukuba, , Japan : National Institute for Environmental Studies .
- Kato , S . 1982 . Laboratory culture and morphology of Colacium vesiculosum Ehrb. (Euglenophyceae). . Jpn J. Phycol. , 30 : 63 – 67 . (In Japanese with English abstract.)
- Kimura , M . 1980 . A simple method for estimating evolutionary rates of base substitutions through comparative studies of nucleotide sequences . J. Mol. Evol. , 16 : 111 – 120 .
- Mai , JC and Coleman , AW . 1997 . The internal transcribed spacer 2 exhibits a common secondary structure in green algae and flowering plants . J. Mol. Evol. , 44 : 258 – 271 .
- Nakada , T and Nozaki , H . 2007 . Re-evaluation of three Chlorogonium (Volvocales, Chlorophyceae) species based on 18S ribosomal RNA gene phylogeny . Eur. J. Phycol. , 42 : 177 – 182 .
- Nakazawa , A , Krienitz , L and Nozaki , H . 2001 . Taxonomy of the unicellular green algal genus Vitreochlamys (Volvocales), based on comparative morphology of cultured material . Eur. J. Phycol. , 36 : 113 – 128 .
- Nozaki , H . 1984 . “ Eudorina elegans Ehrenberg var. elegans ” . In Photomicrographs of the Fresh-water Algae , Edited by: Yamgishi , T and Akiyama , M . Vol. 1 , 51 Tokyo : Uchida Rokakuho .
- Nozaki , H . 1985 . “ Eudorina elegans Ehrenberg var. synoica Goldstein ” . In Photomicrographs of the Fresh-water Algae , Edited by: Yamgishi , T and Akiyama , M . Vol. 3 , 44 Tokyo : Uchida Rokakuho .
- Nozaki , H . 1986 . Notes on microalgae in Japan (10). Eudorina illinoisensis (Chlorophyta, Volvocales) . Jpn J. Phycol. , 34 : 144
- Nozaki , H . 2003 . Origin and evolution of the genera Pleodorina and Volvox (Volvocales) . Biologia , 58 : 425 – 431 .
- Nozaki , H and Ito , M . 1994 . Phylogenetic relationships within the colonial Volvocales (Chlorophyta) inferred from cladistic analysis based on morphological data . J. Phycol. , 30 : 353 – 365 .
- Nozaki , H and Krienitz , L . 2001 . Morphology and phylogeny of Eudorina minodii (Chodat) Nozaki et Krienitz, comb. nov. (Volvocales, Chlorophyta) from Germany . Eur. J. Phycol. , 36 : 23 – 28 .
- Nozaki , H and Kuroiwa , T . 1992 . Ultrastructure of the extracellular matrix and taxonomy of Eudorina, Pleodorina, and Yamagishiella gen. nov. (Volvocaceae, Chlorophyta) . Phycologia , 31 : 529 – 541 .
- Nozaki , H , Kuroiwa , H , Mita , T and Kuroiwa , T . 1989 . Pleodorina japonica sp. nov. (Volvocales, Chlorophyta) with bacteria-like endosymbionts . Phycologia , 28 : 252 – 267 .
- Nozaki , H , Ito , M. Sano , R , Uchida , H , Watanabe , MM and Kuroiwa , T . 1995 . Phylogenetic relationships within the colonial Volvocales (Chlorophyta) inferred from rbcL gene sequence data . J. Phycol. , 31 : 970 – 979 .
- Nozaki , H , Ito , M , Uchida , H , Watanabe , MM and Kuroiwa , T . 1997 . Phylogenetic analysis of Eudorina species (Volvocaceae, Chlorophyta) based on rbcL gene sequences . J. Phycol. , 33 : 859 – 863 .
- Nozaki , H , Song , L , Liu , Y , Hiroki , M and Watanabe , MM . 1998 . Taxonomic re-examination of a Chinese strain labeled ‘Eudorina sp.’ (Volvocaceae, Chlorophyta) based on morphological and DNA sequence data . Phycol. Res. , 46 ( Suppl. ) : 63 – 70 .
- Nozaki , H , Misawa , K , Kajita , T , Kato , M , Nohara , S and Watanabe , MM . 2000 . Origin and evolution of the colonial Volvocales (Chlorophyceae) as inferred from multiple, chloroplast gene sequences . Mol. Phylogenet. Evol. , 17 : 256 – 268 .
- Nozaki , H , Takahara , M , Nakazawa , A , Kita , Y , Yamada , T , Takano , H , Kawano , S and Kato , M . 2002 . Evolution of rbcL group IA introns and intron open reading frames within the colonial Volvocales (Chlorophyceae) . Mol. Phyloget. Evol. , 23 : 326 – 338 .
- Nozaki , H , Ott , FD and Coleman , AW . 2006 . Morphology, molecular phylogeny and taxonomy of two new species of Pleodorina (Volvocaceae, Chlorophyceae) . J. Phycol. , 42 : 1072 – 1080 .
- Pringsheim , EG . 1946 . Pure Cultures of Algae , Cambridge, , UK : Cambridge University Press .
- Rayburn , WR and Starr , RC . 1974 . Morphology and nutrition of Pandorina unicocca sp. nov . J. Phycol. , 10 : 42 – 49 .
- Saitou , N and Nei , M . 1987 . The neighbor-joining method: a new method for reconstructing phylogenetic trees . Mol. Biol. Evol. , 4 : 406 – 425 .
- Sakayama , H , Hara , Y , Arai , S. Sato , H and Nozaki , H . 2004 . Phylogenetic analyses of Nitella subgenus Tieffallenia (Charales, Charophyceae) using nuclear ribosomal DNA internal transcribed spacer sequences . Phycologia , 43 : 672 – 678 .
- Smith , GM . 1930 . Notes on the Volvocales I–IV . Bull Torrey Bot. Club , 57 : 359 – 370 . pls 17–18
- Smith , GM . 1950 . The Fresh-water Algae of United States , 2nd ed. , New York, , USA : McGraw-Hill .
- Starr , RC and Zeikus , JA . 1993 . UTEX: The culture collection of algae at the University of Texas at Austin . J. Phycol. , 29 ( Suppl. ) : 1 – 106 .
- Swofford , DL . 2003 . PAUP*. Phylogenetic Analysis Using Parsimony (*And Other Methods). Version 4.0b10. , Sunderland, , USA : Sinauer Associates .
- Thompson , JD , Gibson , TJ , Plewniak , F , Jeanmougin , F and Higgins , DG . 1997 . The Clustal X windows interface: flexible strategies for multiple sequence alignment aided by quality analysis tools . Nucl. Acids Res. , 25 : 4876 – 4882 .
- Wakasugi , T , Nagai , T , Kapoor , M , Sugita , M , Ito , M , Ito , S , Tsudzuki , J , Nakashima , K , Tsudzuki , T , Suzuki , Y , Hamada , A , Ohta , T , Inamura , A , Yoshinaga , K and Sugiura , M . 1997 . Complete nucleotide sequence of the chloroplast genome from the green alga Chlorella vulgaris: the existence of genes possibly involved in chloroplast division . Proc. Natl Acad. Sci. USA , 94 : 5967 – 5972 .
- Yoshinaga , K , Ohta , T , Suzuki , Y and Sugiura , M . 1988 . Chlorella chloroplast DNA sequence containing a gene for the large subunit of ribulose-1, 5-bisphosphate carboxylase/oxygenase and a part of a possible gene for the β′ subunit of RNA polymerase . Plant Mol. Biol. , 10 : 245 – 250 .