Abstract
Several studies have shown that pet dogs and cats, since they share the habitat with people, could be good sentinels for human exposure to environmental pollutants. However, some publications have suggested that dogs could efficiently metabolize and eliminate some persistent organic pollutants (POPs), which are accumulated by humans throughout life. For this reason, the role of domestic dogs as sentinels could not be appropriate, at least for certain contaminants. To test this hypothesis, we designed this study in which we determined plasma levels of 56 POPs (11 organochlorine pesticides [OCPs], 18 polychlorinated biphenyls [PCBs] and 27 polycyclic aromatic hydrocarbons) in the plasma of 87 dogs and 100 people from the same area (the Canary Islands, Spain). We detected most of these contaminants in the plasma of both species, although the frequencies of detection, concentrations and profiles were very different from each other, especially for ∑OCPs and ∑PCBs. In light of these results, we can conclude that, although they share the environment, sources of exposure to these pollutants and/or the metabolic capabilities do not seem to be comparable between the two species, so that the dog does not seem to be a good sentinel for human exposure to these contaminants.
1. Introduction
Since the iconic ‘canary in the cage’ began to be used to detect the presence of toxic gases in the coal mines, the potential for all kinds of animals to act as sentinels for human exposure to toxic substances has been explored in many scientific papers (Reif Citation2011). The pets, particularly the dogs and cats, are of particular interest, given that they share the habitat with humans and they respond to toxic assaults similar to their owners (Backer et al. Citation2001). Thus, many studies have been designed to reveal the shared exposure of humans and dog/cats to different environmental toxicants of anthropogenic origin, such as asbestos (Glickman et al. Citation1983; Backer et al. Citation2001), tobacco smoke (Ka et al. Citation2014), industrial pollutants (Maciejewski et al. Citation2008; Bischoff et al. Citation2010), pesticides (Knapp et al. Citation2013) or smog (Heyder & Takenaka Citation1996; Calderon-Garciduenas et al. Citation2001), among others. Most of these studies have found associations between exposure and elevation of the selected biomarkers, as well as correlations between the incidence of diseases related to exposure to such pollutants between pets and humans. It is noteworthy, however, that the majority of these studies have focused on pollutants whose primary route of exposure is by inhalation. However, it has been widely reported that the main route of exposure of many other environmental chemicals, such as persistent organic pollutants (POPs), is the ingestion of contaminated food, in which these contaminants are particularly associated with the fat fraction (El-Shahawi et al. Citation2010). This is relevant since pets, while sharing the habitat with their owners, currently can be said that mostly do not share the dietary pattern with humans, because most domestic dogs and cats are fed specially formulated feed (Rumbeiha & Morrison Citation2011).
This group of food-associated contaminants – the POPs – is constituted by a high variety of synthetic, lipophilic, persistent and cumulative substances (Safe Citation1990). Many POPs come from the production and use of organochlorine compounds, such as organochlorine pesticides (OCPs) and polychlorinated biphenyls (PCBs). Polycyclic aromatic hydrocarbons (PAHs) are ubiquitous environmental pollutants containing two or more fused benzene rings that are produced during the incomplete combustion of organic matter and during human or industrial activities (Guo et al. Citation2012). Because of their efficient metabolization, strictly speaking, PAHs cannot be considered as POPs, but due to their high prevalence in the environment and their lipophilicity, PAHs are usually considered as POPs (Lammel et al. Citation2013). Numerous studies have revealed that POPs, individually and in combination, may contribute to the development of severe health problems such as immune suppression, genotoxic effects or cancer and are of public health concern worldwide (WHO Citation2003; Kortenkamp Citation2007; Kortenkamp et al. Citation2007, Citation2011; Valeron et al. Citation2009; Lauby-Secretan et al. Citation2013; Jarvis et al. Citation2014). As far as we know, there is no research that has assessed exposure to PAHs in dogs to date, and very few scientific studies have evaluated organochlorine contamination of dogs and cats (Kunisue et al. Citation2005; Storelli et al. Citation2009; Ali et al. Citation2013; Dirtu et al. Citation2013). These studies have indicated that dogs, as occurs with other canines (Georgii et al. Citation1994; Shore et al. Citation2001), accumulated minor amounts of some of these compounds (particularly DDT and its metabolites, and the highly chlorinated biphenyls) than other mammalian species. Therefore, it has been suggested that the canines may have greater metabolic capacity and elimination of these compounds than other mammalian species (Georgii et al. Citation1994; Kunisue et al. Citation2005).
In light of the above, the domestic dog does not seem to be a good sentinel for human exposure to PCBs and some OCPs. To test this hypothesis, and also to assess the role of the dog as monitor of human exposure to PAHs, we designed the present study. We have determined the plasmatic levels of 56 POPs (11 OCPs and metabolites, 18 PCBs, and 27 PAHs and metabolites) in dog (n = 87) and human (n = 100) plasma samples. Sampling was performed in the Canary Islands (Spain), a well-studied area regarding the levels of these pollutants (Zumbado et al. Citation2005; Luzardo et al. Citation2009, Citation2014; Henríquez-Hernández et al. Citation2011; Almeida-Gonzalez et al. Citation2012; Boada et al. Citation2012; Luzardo, Rodriguez-Hernandez, et al. Citation2013; Burillo-Putze et al. Citation2014; Rodríguez-Hernández et al. Citation2014;), and all the samples were analysed under the same instrumental conditions. The normalized results of both species were statistically analysed and compared with each other.
2. Materials and methods
2.1. Sampling and ethics statement
Dog blood samples were collected between November 2013 and March 2014 through cephalic vein puncture from 87 pet dogs (46 males and 41 females, 0.5–13 years old) visiting the Veterinary Hospital of the Veterinary Faculty of the University of Las Palmas de Gran Canaria (Canary Islands, Spain) for routine care. Only clinically normal animals (negative stool sample, negative result on a heart-worm test and no overt disease) were included in the study, after owners' consent. Human blood samples from 60 males and 40 females (19–34 years old) were collected from the Spanish Red Cross blood bank from October to December 2013. All participants were residents in Gran Canaria (Canary Island, Spain) and determined to be eligible as blood donors based on the screening by nurses before recruitment. All participants provided their written informed consent to participate in this study. The study was approved by the Research and Ethics Committee of our Institution (University of Las Palmas de Gran Canaria, Canary Islands, Spain). Samples of blood were collected in heparinized tubes, maintained at 4°C, and centrifuged at 1000 g for 15 min to separate the plasma and kept at − 20°C until chemical analysis. Although for logistical reasons we could not get blood from the owners of the dogs included in this study, it has been estimated that there are about six million domestic dogs in Spain and that more than 40% of Spanish homes have at least one dog, so we can assume that a high percentage of these blood donors share habitat with some dog.
2.2. Analytical chemical methods
We measured the serum levels of 11 OCPs: p,p′-Dichlorodiphenyltrichloroethane (p,p′ -DDT), p,p′-Dichlorodiphenyldichloroethylene (p,p′ -DDE), p,p′-Dichlorodiphenyldichloroethane (p,p′ -DDD), hexachlorobenzene (HCB), hexachlorocyclohexane (α-, β-, γ- and δ-HCH), aldrin, endrin and dieldrin. We also determined 18 PCB congeners, including marker-PCBs (M-PCBs) and dioxin-like PCBs (DL-PCBs): IUPAC numbers # 28, 52, 77, 81, 101, 105, 114, 118, 123, 126, 138, 153, 156, 157, 167, 169, 180 and 189. We also included 21 PAHs listed in the Toxics Release Inventory Program of the United States and the Environmental Protection Agency's (EPA) Priority Chemical list (EPA 2001): benzo(a)anthracene, benzo(a)phenanthrene (chrysene), benzo(a)pyrene, benzo(b)fluoranthene, benzo(j)fluoranthene, benzo(k)fluoranthene, benzo(j,k)fluorene (fluoranthene), dibenzo(a,h)anthracene, dibenzo(a,e)pyrene, dibenzo(a,h)pyrene, dibenzo(a,l)pyrene, indeno(1,2,3-cd)pyrene, 5-methylchrysene, acenaphthene, acenaphthylene, anthracene, benzo(g,h,i)perylene, fluorene, phenanthrene, pyrene and naphthalene. Finally, we also determined the levels of six common metabolites of PAHs: 1-naphthol, 2-naphthol, 2-OH-fluorene, 1-OH-phenanthrene, 7-OH-benzo(c)fluorene, and 1-OH-pyrene.
The details of sample extraction, validated chromatographic method and quality control have been previously reported (Camacho, Boada, et al. Citation2013; Camacho, Luzardo, et al. Citation2013; Luzardo, Ruiz-Suarez, et al. Citation2013; Camacho et al. Citation2014). Briefly, samples were subjected to solid-phase extraction using Chromabond® C18ec columns (Macherey-Nagel, Germany) that yielded recoveries in the range of 79%–107%. Without further purification steps, the samples were subjected to chromatographic analysis on a Trace GC Ultra coupled with a Quantum Max triple quadrupole mass spectrometer (Thermo Fisher Scientific, Palo Alto, CA, USA) for the quantification of 56 analytes in addition to internal standards and surrogates. The limit of quantification (LOQ) was set at 5 pg/mL for all the compounds. A zero value was assigned to all the compounds below the limit of detection, and those compounds below the LOQ were assigned half of the LOQ. The total cholesterol and triglyceride concentrations were used for the lipid adjustment of results of organochlorine compounds, as recommended (Bernert et al. Citation2007). Therefore, the results have been expressed in ng/g lipid weight (lw).
2.3. Statistical analysis
We used PASW Statistics v 19.0 (SPSS Inc., Chicago, IL, USA) to manage the database of the study and to perform statistical analyses. Normality was examined using the Kolmogorov–Smirnov test. The POPs distributions lacked normality and homoscedasticity; therefore, we used non-parametric tests (the Mann–Whitney and Kruskal–Wallis tests). We used the chi-square test to examine the relationships between the categorical variables. The results were reported as medians and ranges (or interquartile ranges). Probability levels of less than 0.05 (two-tailed) were considered statistically significant.
3. Results and discussion
3.1. Concentrations of PAHs in dog and human plasma
All PAHs included in this study were detected in this series of samples (humans and dogs), with the exception of benzo(a)pyrene, which was not detected in none of the dog plasma samples. The compounds most frequently detected in both species were phenanthrene, fluorene and fluoranthene, and 2-naphthol, which were present in nearly 100% of the samples. The frequencies of detection of the rest of compounds of this chemical group were highly variable and different between the two species, as can be seen in .
Table 1. Individual PAHs, PAH metabolites, ∑PAH7 and ∑PAH21 concentrations (ng/g lw) in dog (n = 87) and human (n = 100) plasma samples from the Canary Islands, Spain.
We also show in the fat-normalized median values, ranges and means plus standard deviations (SDs) of the 21 PAH and 6 PAH metabolites in dog and human plasma, respectively. The median values of ∑PAH21 were 747.6 and 1404.1 ng/g lw (0.87 and 1.71 ng/g lw), for dogs and humans, respectively. When we considered only those PAHs that are classified as probable human carcinogens by the US EPA (PAH7, benzo(a)anthracene, chrysene, benzo(a)pyrene, benzo(b)fluoranthene, benzo(k)fluoranthene, dibenzo(a,h)anthracene, and indeno(1,2,3-c,d)pyrene) the median (and mean ± SD) values were 0 (6.8 ± 17.2) ng/g lw in dog plasma and 10.4 (21.9 ± 43.5) ng/g lw in human plasma. We consider that it is also interesting to highlight the results of the sum of PAH metabolites in plasma samples, whose median values were 144.8 and 93.7 ng/g lw in dogs and humans, respectively. As far as we know, there is no study that has reported plasma levels of PAHs in dogs, and we can only compare the results with other studies in humans. Thus, the levels found in human plasma are comparable to those found in cord blood in Hong Kong in 2005 (1158 ng/g lw) (Tsang et al. Citation2011) and in China in 2011 (1370 ng/g lw) (Yu et al. Citation2011), but much lower than those found in maternal and cord blood in Texas, USA (5.5 and 12.8 ng/g ww) (Sexton et al. Citation2011). In any case, it should be noted that these comparisons are not totally reliable since in these papers a different number of compounds were included, with 15 analytes in the works of Hong Kong and China and 50 individual PAHs in the work of Texas. However, if we compare only the values of the most toxic PAHs (PAH7) with those reported in the literature, we found that levels of this study were much lower than those described in China (Yu et al. Citation2011; Guo et al. Citation2012; Song et al. Citation2013) and Hong Kong (Tsang et al. Citation2011).
However, our main objective in this study was the comparison of the levels and detection frequencies of pollutants between the two species, and in this sense, we have found many significant differences. So, we found remarkable differences for both PAH21 () and for PAH7 (, inset) levels, which were detected at much higher levels in humans than in dogs (p < .0001). These results suggest that exposure of both species to this contaminant group could be different. However, it also seems quite remarkable that for metabolites of PAHs, the relationship was the opposite, plasma levels of these chemicals being significantly higher in dogs than in humans (), which could also indicate that dogs possess superior ability to metabolize these compounds than humans. Obviously, to confirm this point additional research is needed, but our results allow us to hypothesize that the lower levels of PAHs detected in the plasma of dogs could be due to a higher rate of biotransformation and elimination thereof.
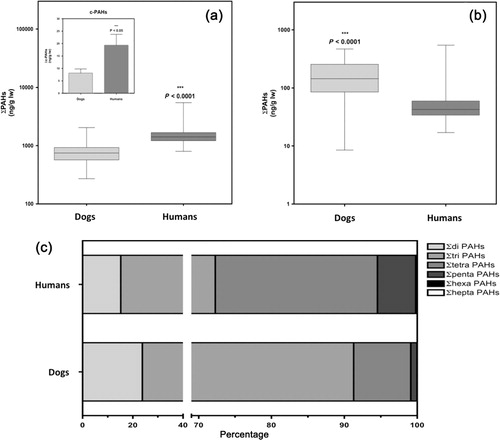
Furthermore, as shown in , the profiles of PAH contamination were not similar between dogs and humans, with a clear predominance of the four ring compounds in human and three ring compounds in dogs. In fact, as seen in , it is noteworthy that some compounds such as pyrene, which were detected in almost 100% of the human samples, were barely detectable in 17% of samples from dogs, and yet, others such as acenaphthylene or 1-naphthol were much more frequently detected in dog plasma than in human plasma. Thus, in the light of the above discussion, we can conclude that the dog does not seem to be a good sentinel for human exposure to PAHs.
3.2. Concentrations of OCPs in dog and human plasma
In this study we included only a selection of 11 OCPs, among the most frequently detected in environmental samples, and as it was expected, all of them were detected in plasma samples of both dog and humans. However, for many of them, we found significant differences in both the frequency of detection and, above all, in concentrations. In and in , we summarize the results of this group of contaminants. It is noteworthy that the levels of the sum of OCPs was an order of magnitude higher in humans than in dogs (), although the differences were not homogeneous among the different pollutants in this group.
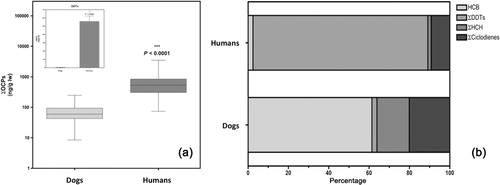
Table 2. OCP concentrations (ng/g lw) in dog (n = 87) and human (n = 100) plasma samples from the Canary Islands, Spain.
Thus, the OCP that was detected more frequently and at higher concentrations in canine plasma was HCB, as was detected in 96.5%. This detection rate was similar to that found in human plasma (98%), but the levels were higher in the latter (median 0.2 vs. 10.1 ng/g lw, p < .01). It is also noteworthy that the concentrations of this pollutant in dogs accounted for over 60% of all pollutants in this group (), whereas in humans represented only 2.5% of ∑OCPs. There are very few references that have reported the levels of this pollutant in dogs, and, as far as we know, none in plasma. However, the results reported here are comparable in concentration to those described in the livers from Russian wolves, although the detection rate was considerably lower in those animals (3.5% vs. 96.5%, respectively, in wolves and dogs) (Shore et al. Citation2001). Our results, however, were much higher than those described in gonadal tissues of domestic dogs in Japan in 2005 (Kunisue et al. Citation2005), although this comparison may not be appropriate, since it has been reported that HCB in dogs is distributed effectively firstly to the lungs, and later to other visceral organs (mainly liver, kidneys and spleen), and afterwards, much more slowly, to fatty tissues and other target tissues, where this pollutant reaches low concentrations (Sundlof et al. Citation1982). On the other hand, the plasma levels of HCB in humans of this study were fully comparable with those described recently in other European countries (Koppen et al. Citation2009; Mari et al. Citation2013).
With regard to plasma concentrations of OCPs in dogs, the most important group was that of cyclodienes, mainly dieldrin (median 8.2 ng/g lw), representing 20.1% of total OCPs detected in this species (). However, both the frequency and the plasma levels of this pollutant were significantly lower than those detected in human plasma (91%, 34.1 ng/g lw, p < .0001). The other two cyclodienes included in this study, aldrin and endrin, were also detected in both types of samples, albeit at very low concentrations, without significant differences, neither in their concentrations nor in their detection frequencies between the two species. The values and the frequency of detection of dieldrin described for dogs of this study are very similar to those described in livers of Russian wolves in 2001 (67.2%, 13.1 ng/g lw) (Shore et al. Citation2001). We do not have further information of other works that have been published the levels of these cyclodienes in dogs or other canines. With respect to the values detected in humans in this study, we found that the profile was somewhat different to that previously described in a population sample taken in this same region in 1998, as now we have found higher levels of dieldrin and lower levels of aldrin and endrin in people from the Canary Islands (Luzardo et al. Citation2006).
The second set of most relevant pollutants in terms of concentrations in canine plasma was formed by the isomers of HCH, which represented 16% of total OCPs in this species (), whereas in humans only represents 1.7%. The four isomers were detected in both species, but at very low concentrations (the median value was 0 ng/g lw in all cases). The mean values of this group of pollutants in dogs were higher than those reported in Pakistan in 2013 (Ali et al. Citation2013), but the median values were much lower, indicating that there was a high dispersion of the concentrations. However, it should be noted that the sample sizes of both studies were very different, being much higher in this study (n = 87 vs. 16), so probably the median value of this study more accurately reflects the actual level of contamination in this species. Still, because the detection frequencies of the beta and gamma isomers were much higher in the samples of human plasma, we found that the values were significantly higher in humans than in dogs (p < .0001 for both chemicals).
Finally, we have to remark that the most striking difference between the two species is the relative to the group of DDTs, as can be seen in the inset of . The concentrations of these contaminants in humans, in particular p,p′ -DDE, were very high (median 352.8 ng/g lw) as has been described in numerous publications for this and other Western populations (Zumbado et al. Citation2005). Indeed, in human plasma samples, the p,p′ -DDE was detected in 100% of the samples, and its parent compound (p,p′ -DDT) in 52% samples. In fact, the concentration of these compounds represented 86.7% of ∑OCPs in human samples. Reversely, in samples of canine plasma the situation was radically different, as the median value was 0 ng/g lw, and detection frequency did not exceed 8% for any of these analytes. This had already been described in dogs of Pakistan and Japan (Kunisue et al. Citation2005; Ali et al. Citation2013), and also in Russian wolves (Shore et al. Citation2001), so it has been suggested that canines, unlike many other mammalian species, do not accumulate these pollutants throughout their life, probably because they are equipped with efficient mechanisms of biotransformation and elimination (Kunisue et al. Citation2005).
All the evidences set out above, in which the values of most of the contaminants in this group were much higher in humans than in dogs, especially so different pollution profiles between the two species, indicate that the dog is not a good indicator of human exposure to OCPs.
3.3. Concentrations of PCBs in dog and human plasma
With respect to contaminants in this group, we also found highly significant differences for both the group (∑PCBs) and for each one of the individual congeners between both species. With respect to the summed values, the medians were almost 20 times higher in humans than in dogs (276.3 vs. 16.7 ng/g lw) ( and ). The levels reported in this work in humans are comparable to those of any other Western populations (Koppen et al. Citation2009; Mari et al. Citation2013), and the profile of contamination was very similar in both species, as shown in , with a predominance the M-PCBs, which accounted for about 75% of total plasma PCB concentrations in both canine and human plasma. Indeed seven congeners of this group were detected in both the samples of both species. However, the significant difference levels remained for all of the congeners, whose concentrations were much higher in human plasma, where they also reach percentages of detection very close to 100% (except PCB 118) ().
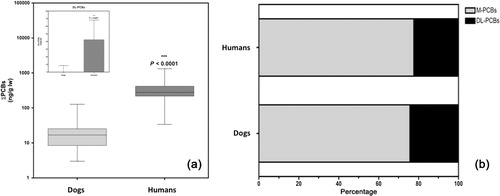
Table 3. PCB concentrations (ng/g lw) in dog (n = 87) and human (n = 100) plasma samples from the Canary Islands, Spain.
Regarding the DL-PCBs, all congeners (except the PCB 126) were detected in human plasma, whereas in the canine plasma congeners #77, 81, 105, 114, 123 and 126 were not detected in any sample, and the median values for the remaining congeners were 0 ng/g lw in this species. In fact, as shown in the insert of , the differences between species became more evident in this subgroup of PCBs.
As it was described for the previously discussed groups of pollutants that were included in this study, it is possible that the source of exposure to contaminants (in this case PCBs) is different between the two species, given that these are food-associated pollutants and the eating pattern is generally also different between humans and dogs. In any case, it has also been suggested that the canines could have the ability to efficiently metabolize PCBs, or at least some congeners (Kunisue et al. Citation2005), since the few studies that have investigated the levels of these pollutants in dogs have also reported a very low level thereof as compared to those commonly found in mammalian species (Shore et al. Citation2001; Kunisue et al. Citation2005; Ali et al. Citation2013).
So, as we have concluded for the other groups of contaminants, in the light of the above discussion, it does not appear that the dogs can be used as indicators or sentinels for human exposure to PCBs.
4. Conclusions
In this paper, we compared the plasma levels of a broad panel of contaminants commonly associated with food (PAHs, PCBs and OCPs) in two species that closely share the habitat, such as dogs and humans. The main purpose of this study was to test whether domestic dogs are equally exposed than humans to these pollutants, and thus whether they could serve as sentinels for human exposure to them. In accordance with the results found in this study, the levels of most pollutants investigated were much higher in humans than in dogs, and the profiles of contamination were completely different, indicating that the sources of exposure between the two species are probably different. Furthermore, different evidences such as significantly higher levels of PAH metabolites in dogs, or a null rate of bioaccumulation of contaminants so common in humans such as DDTs and PCBs, suggest that the dog could be provided with different enzymatic capabilities to the human, which would allow them to effectively remove these pollutants. Therefore, we can conclude that, although the domestic dog has been shown as an excellent indicator of human exposure to contaminants by inhalation, for the pollutants studied in this work this species does not behave as a good indicator of human exposure. It will be extremely interesting to carry out further research to elucidate the differential biotransformation routes between canines and humans that may explain these results.
Acknowledgements
The authors thank Mrs María de los Reyes Suárez Hanna for her technical assistance. The authors also thank the veterinarians Dr Elena Carretón and Dr Alberto Montoya for providing dog blood samples.
References
- Ali N, Malik RN, Mehdi T, Eqani SA, Javeed A, Neels H, Covaci A. 2013. Organohalogenated contaminants (OHCs) in the serum and hair of pet cats and dogs: biosentinels of indoor pollution. Sci Total Environ. 449:29–36. 10.1016/j.scitotenv.2013.01.037
- Almeida-Gonzalez M, Luzardo OP, Zumbado M, Rodriguez-Hernandez A, Ruiz-Suarez N, Sangil M, Camacho M, Henriquez-Hernandez LA, Boada LD. 2012. Levels of organochlorine contaminants in organic and conventional cheeses and their impact on the health of consumers: an independent study in the Canary Islands (Spain). Food Chem Toxicol. 50:4325–4332. 10.1016/j.fct.2012.08.058
- Backer LC, Grindem CB, Corbett WT, Cullins L, Hunter JL. 2001. Pet dogs as sentinels for environmental contamination. Sci Total Environ. 274:161–169. 10.1016/S0048-9697(01)00740-9
- Bernert JT, Turner WE, Patterson DG, Jr, Needham LL. 2007. Calculation of serum “total lipid” concentrations for the adjustment of persistent organohalogen toxicant measurements in human samples. Chemosphere. 68:824–831. 10.1016/j.chemosphere.2007.02.043
- Bischoff K, Priest H, Mount-Long A. 2010. Animals as sentinels for human lead exposure: a case report. J Med Toxicol. 6:185–189. 10.1007/s13181-010-0014-9
- Boada LD, Zumbado M, Henriquez-Hernandez LA, Almeida-Gonzalez M, Alvarez-Leon EE, Serra-Majem L, Luzardo OP. 2012. Complex organochlorine pesticide mixtures as determinant factor for breast cancer risk: a population-based case-control study in the Canary Islands (Spain). Environ Health. 11:28.
- Burillo-Putze G, Luzardo OP, Garcia CP, Zumbado M, Yanes C, Del Mar Trujillo-Martin M, Boada Fernandez Del Campo C, Boada LD. 2014. [Exposure to persistent and non-persistent pesticides in a non-occupationally exposed population in Tenerife Island (Spain).]. Gaceta Sanitaria (Gac Sanit)/SESPAS. 28:301–304.
- Calderon-Garciduenas L, Mora-Tiscareno A, Fordham LA, Chung CJ, Garcia R, Osnaya N, Hernandez J, Acuna H, Gambling TM, Villarreal-Calderon A, et al. 2001. Canines as sentinel species for assessing chronic exposures to air pollutants: part 1. Respiratory pathology. Toxicol Sci. 61:342–355.
- Camacho M, Boada LD, Oros J, Lopez P, Zumbado M, Almeida-Gonzalez M, Luzardo OP. 2013. Comparative study of organohalogen contamination between two populations of Eastern Atlantic loggerhead sea turtles (Caretta caretta). Bull Environ Contam Toxicol. 91:678–683.
- Camacho M, Boada LD, Oros J, Lopez P, Zumbado M, Almeida-Gonzalez M, Luzardo OP. 2014. Monitoring organic and inorganic pollutants in juvenile live sea turtles: results from a study of Chelonia mydas and Eretmochelys imbricata in Cape Verde. Sci Total Environ. 481C:303–310. 10.1016/j.scitotenv.2014.02.051
- Camacho M, Luzardo OP, Boada LD, Lopez Jurado LF, Medina M, Zumbado M, Oros J. 2013. Potential adverse health effects of persistent organic pollutants on sea turtles: evidences from a cross-sectional study on Cape Verde loggerhead sea turtles. Science Total Environ. 458–460C:283–289.
- Dirtu AC, Niessen SJ, Jorens PG, Covaci A. 2013. Organohalogenated contaminants in domestic cats' plasma in relation to spontaneous acromegaly and type 2 diabetes mellitus: a clue for endocrine disruption in humans? Environ Int. 57–58:60–67.
- El-Shahawi MS, Hamza A, Bashammakh AS, Al-Saggaf WT. 2010. An overview on the accumulation, distribution, transformations, toxicity and analytical methods for the monitoring of persistent organic pollutants. Talanta. 80:1587–1597. 10.1016/j.talanta.2009.09.055
- EPA. 2001. United States Environmental Protection Agency, Office of Environmental Information, Emergency planning and community right-to-know act - Section 313: Guidance for reporting toxic chemicals: polycyclic aromatic compounds category. (EPA.260-B-01-03). Environmental Protection Agency.
- Georgii S, Bachour G, Failing K, Eskens U, Elmadfa I, Brunn H. 1994. Polychlorinated biphenyl congeners in foxes in Germany from 1983 to 1991. Arch Environ Contam Toxicol. 26:1–6.
- Glickman LT, Domanski LM, Maguire TG, Dubielzig RR, Churg A. 1983. Mesothelioma in pet dogs associated with exposure of their owners to asbestos. Environ Res. 32:305–313. 10.1016/0013-9351(83)90114-7
- Guo Y, Huo X, Wu K, Liu J, Zhang Y, Xu X. 2012. Carcinogenic polycyclic aromatic hydrocarbons in umbilical cord blood of human neonates from Guiyu, China. Sci Total Environ. 427–428:35–40.
- Henríquez-Hernández LA, Luzardo PL, Almeida-González M, Álvarez-León EE, Serra-Majem L, Zumbado M, Boada LD. 2011. Background levels of polychlorinated biphenyls in the population of the Canary Islands (Spain). Environ Res. 111:10–16.
- Heyder J, Takenaka S. 1996. Long-term canine exposure studies with ambient air pollutants. Eur Respir J. 9:571–584. 10.1183/09031936.96.09030571
- Jarvis IW, Dreij K, Mattsson A, Jernstrom B, Stenius U. 2014. Interactions between polycyclic aromatic hydrocarbons in complex mixtures and implications for cancer risk assessment. Toxicology. 321C:27–39. 10.1016/j.tox.2014.03.012
- Ka D, Marignac G, Desquilbet L, Freyburger L, Hubert B, Garelik D, Perrot S. 2014. Association between passive smoking and atopic dermatitis in dogs. Food Chem Toxicol. 66:329–333. 10.1016/j.fct.2014.01.015
- Knapp DW, Peer WA, Conteh A, Diggs AR, Cooper BR, Glickman NW, Bonney PL, Stewart JC, Glickman LT, Murphy AS. 2013. Detection of herbicides in the urine of pet dogs following home lawn chemical application. Sci Total Environ. 456–457:34–41.
- Koppen G, Den Hond E, Nelen V, Van De Mieroop E, Bruckers L, Bilau M, Keune H, Van Larebeke N, Covaci A, Van De Weghe H, et al. 2009. Organochlorine and heavy metals in newborns: results from the Flemish Environment and Health Survey (FLEHS 2002–2006). Environ Int. 35:1015–1022. 10.1016/j.envint.2009.05.002
- Kortenkamp A. 2007. Ten years of mixing cocktails: a review of combination effects of endocrine-disrupting chemicals. Environ Health Perspect. 115 (Suppl 1):98–105. 10.1289/ehp.9357
- Kortenkamp A, Faust M, Scholze M, Backhaus T. 2007. Low-level exposure to multiple chemicals: reason for human health concerns? Environ Health Perspect. 115 (Suppl 1):106–114.
- Kortenkamp A, Martin O, Faust M, Evans R, McKinlay R, Orton F, Rosivatz E. 2011. State of the art assessment of endocrine disrupters, final report; EU; [cited 2014 June 24]. Available from: http://ec.europa.eu/environment/chemicals/endocrine/pdf/sota_edc_final_report.pdf.
- Kunisue T, Nakanishi S, Watanabe M, Abe T, Nakatsu S, Kawauchi S, Sano A, Horii A, Kano Y, Tanabe S. 2005. Contamination status and accumulation features of persistent organochlorines in pet dogs and cats from Japan. Environ Pollut. 136:465–476. 10.1016/j.envpol.2004.12.039
- Lammel G, Heil A, Stemmler I, Dvorska A, Klanova J. 2013. On the contribution of biomass burning to POPs (PAHs and PCDDs) in air in Africa. Environ Sci Technol. 47: 11616–11624. 10.1021/es401499q
- Lauby-Secretan B, Loomis D, Grosse Y, El Ghissassi F, Bouvard V, Benbrahim-Tallaa L, Guha N, Baan R, Mattock H, Straif K. 2013. Carcinogenicity of polychlorinated biphenyls and polybrominated biphenyls. Lancet Oncol. 14:287–288. 10.1016/S1470-2045(13)70104-9
- Luzardo OP, Goethals M, Zumbado M, Alvarez-Leon EE, Cabrera F, Serra-Majem L, Boada LD. 2006. Increasing serum levels of non-DDT-derivative organochlorine pesticides in the younger population of the Canary Islands (Spain). Sci Total Environ. 367:129–138. 10.1016/j.scitotenv.2006.01.027
- Luzardo OP, Mahtani V, Troyano JM, Alvarez de la Rosa M, Padilla-Perez AI, Zumbado M, Almeida M, Burillo-Putze G, Boada C, Boada LD. 2009. Determinants of organochlorine levels detectable in the amniotic fluid of women from Tenerife Island (Canary Islands, Spain). Environ Res. 109:607–613. 10.1016/j.envres.2009.03.008
- Luzardo OP, Rodriguez-Hernandez A, Quesada-Tacoronte Y, Ruiz-Suarez N, Almeida-Gonzalez M, Henriquez-Hernandez LA, Zumbado M, Boada LD. 2013. Influence of the method of production of eggs on the daily intake of polycyclic aromatic hydrocarbons and organochlorine contaminants: an independent study in the Canary Islands (Spain). Food Chem Toxicol. 60:455–462. 10.1016/j.fct.2013.08.003
- Luzardo OP, Ruiz-Suarez N, Almeida-Gonzalez M, Henriquez-Hernandez LA, Zumbado M, Boada LD. 2013. Multi-residue method for the determination of 57 persistent organic pollutants in human milk and colostrum using a QuEChERS-based extraction procedure. Anal Bioanal Chem. 405:9523–9536.
- Luzardo OP, Ruiz-Suarez N, Henriquez-Hernandez LA, Valeron PF, Camacho M, Zumbado M, Boada LD. 2014. Assessment of the exposure to organochlorine pesticides, PCBs and PAHs in six species of predatory birds of the Canary Islands, Spain. Sci Total Environ. 472:146–153. 10.1016/j.scitotenv.2013.11.021
- Maciejewski R, Glickman N, Moore G, Zheng C, Tyner B, Cleveland W, Ebert D, Glickman L. 2008. Companion animals as sentinels for community exposure to industrial chemicals: the Fairburn, GA, propyl mercaptan case study. Public Health Rep. 123:333–342.
- Mari M, Nadal M, Schuhmacher M, Domingo JL. 2013. Body burden monitoring of dioxins and other organic substances in workers at a hazardous waste incinerator. Int J Hygiene Environ Health. 216:728–734. 10.1016/j.ijheh.2013.01.003
- Reif JS. 2011. Animal sentinels for environmental and public health. Public Health Rep. 126 (Suppl 1):50–57.
- Rodríguez-Hernández A, Camacho M, Boada LD, Ruiz-Suarez N, Almeida Gonzalez M, Henríquez-Hernández LA, Zumbado M, Luzardo OP. 2014. Daily intake of anthropogenic pollutants through yogurt consumption in the Spanish population. J Appl Anim Res. doi:10.1080/09712119.2014.978777
- Rumbeiha W, Morrison J. 2011. A review of class I and class II pet food recalls involving chemical contaminants from 1996 to 2008. J Med Toxicol. 7:60–66. 10.1007/s13181-010-0123-5
- Safe S. 1990. Polychlorinated biphenyls (PCBs), dibenzo-p-dioxins (PCDDs), dibenzofurans (PCDFs), and related compounds: environmental and mechanistic considerations which support the development of toxic equivalency factors (TEFs). Crit Rev Toxicol. 21:51–88. 10.3109/10408449009089873
- Sexton K, Salinas JJ, McDonald TJ, Gowen RM, Miller RP, McCormick JB, Fisher-Hoch SP. 2011. Polycyclic aromatic hydrocarbons in maternal and umbilical cord blood from pregnant Hispanic women living in Brownsville, Texas. Int J Environ Res Public Health. 8:3365–3379. 10.3390/ijerph8083365
- Shore RF, Casulli A, Bologov V, Wienburg CL, Afsar A, Toyne P, Dell'Omo G. 2001. Organochlorine pesticide, polychlorinated biphenyl and heavy metal concentrations in wolves (Canis lupus L. 1758) from north-west Russia. Sci Total Environ. 280:45–54.
- Song XF, Chen ZY, Zang ZJ, Zhang YN, Zeng F, Peng YP, Yang C. 2013. Investigation of polycyclic aromatic hydrocarbon level in blood and semen quality for residents in Pearl River Delta Region in China. Environ Int. 60:97–105. 10.1016/j.envint.2013.08.003
- Storelli MM, Storelli A, Barone G, Franchini D. 2009. Accumulation of polychlorinated biphenyls and organochlorine pesticide in pet cats and dogs: assessment of toxicological status. Sci Total Environ. 408:64–68. 10.1016/j.scitotenv.2009.09.018
- Sundlof SF, Hansen LG, Koritz GD, Sundlof SM. 1982. The pharmacokinetics of hexachlorobenzene in male beagles. Distribution, excretion, and pharmacokinetic model. Drug Metab Dispos. 10:371–381.
- Tsang HL, Wu S, Leung CK, Tao S, Wong MH. 2011. Body burden of POPs of Hong Kong residents, based on human milk, maternal and cord serum. Environ Int. 37:142–151. 10.1016/j.envint.2010.08.010
- Valeron PF, Pestano JJ, Luzardo OP, Zumbado ML, Almeida M, Boada LD. 2009. Differential effects exerted on human mammary epithelial cells by environmentally relevant organochlorine pesticides either individually or in combination. Chem Biol Interact. 180:485–491. 10.1016/j.cbi.2009.04.010
- WHO. 2003. Health risks of persistent organic pollutants from long-range transboundary air pollution; [cited 2014 June 20]. Available from: http://www.euro.who.int/__data/assets/pdf_file/0009/78660/e78963.pdf.
- Yu Y, Wang X, Wang B, Tao S, Liu W, Wang X, Cao J, Li B, Lu X, Wong MH. 2011. Polycyclic aromatic hydrocarbon residues in human milk, placenta, and umbilical cord blood in Beijing, China. Environ Sci Technol. 45:10235–10242. 10.1021/es202827g
- Zumbado M, Goethals M, Alvarez-Leon EE, Luzardo OP, Cabrera F, Serra-Majem L, Dominguez-Boada L. 2005. Inadvertent exposure to organochlorine pesticides DDT and derivatives in people from the Canary Islands (Spain). Sci Total Environ. 339:49–62. 10.1016/j.scitotenv.2004.07.022