Abstract
Skeletal muscle hypertrophy is an exercise-induced adaptation, particularly in resistance training (RT) programs that use large volumes and low loads. However, evidence regarding the role of rest intervals on metabolic stress and muscular adaptations is inconclusive. Thus, we aimed to investigate the effects of a strenuous RT model (jump-training) on skeletal muscle adaptations and metabolic stress, considering the scarce information about RT models for rats. We hypothesized that jump-training induces metabolic stress and influences negatively the growth of soleus (SOL) and extensor digitorum longus (EDL) muscles of rats. Male Wistar rats (aged 60 days) were randomly assigned to non-trained or trained groups (n = 8/group). Trained rats performed jump-training during 5 days a week for 1, 3, or 5 weeks with 30 s of inter-set rest intervals. Forty-eight hours after the experimental period, rats were euthanized and blood samples immediately drawn to measure creatine kinase activity, lactate and corticosterone concentrations. Muscle weight-to-body weight ratio (MW/BW), cross-sectional area (CSA) and myosin heavy chain (MHC) isoform expression were determined. Higher lactate levels occurred after 20 min of training in weeks 1 and 3. Corticosterone levels were higher after 5 weeks of training. Jump-training had negative effects on hypertrophy of types-I and II muscle fibers after 5 weeks of training, as evidenced by decreased CSA and reduced muscle weight. Our results demonstrated that pronounced metabolic stress and impairment of muscle growth might take place when variables of exercise training are not appropriately manipulated.
Lay summary
Resistance training (RT) has been used to increase muscle mass. In this regard, training variables (intensity, volume, and frequency) must be strictly controlled in order to evoke substantial muscular fitness. This study shows that rats submitted to 5 weeks of intensive resistance jump-training – high intensity, large volume, and short rest intervals – present high levels of blood corticosterone associated with negative effects on hypertrophy of types-I and II muscle fibers.
Introduction
Resistance training (RT) is a modality of exercise training indicated to achieve specific training outcomes, including skeletal muscle hypertrophy and strength (Kwon, Jang, Cho, Jang, & Lee, Citation2018). Training variables (intensity, volume, and frequency) must be strictly controlled in order to evoke substantial muscular fitness (Burd et al., Citation2010a; Ogasawara, Arihara, Takegaki, Nakazato, & Ishii, Citation2017). Training volume consists of the total number of repetitions performed during a training session, multiplied by the load used, and reflects the duration for which muscles are being stressed (Lorenz, Reiman, & Walker, Citation2010). Interaction of these variables results in training overload (Bågenhammar & Hansson, Citation2007). Accordingly, combination of large volumes and low loads have been shown to induce greater muscle hypertrophy (Burd et al., Citation2010b; Ikezoe, Kobayashi, Nakamura, & Ichihashi, Citation2017). However, evidence regarding the role of rest intervals on metabolic stress and muscular adaptations in response to RT are inconclusive (Fink, Schoenfeld, Kikuchi, & Nakazato, Citation2017; Grgic, Lazinica, Mikulic, Krieger, & Schoenfeld, Citation2017). Thus, whether the combination of high loads, large volume, elevated frequency, and short rest intervals evoke maladaptation in skeletal muscle and intense metabolic stress is not fully elucidated.
Although RT has been consistently used to investigate the molecular mechanisms of exercise training in rodents (Cholewa et al., Citation2014; Hornberger & Farrar, Citation2004), metabolic stress might affect the training-induced muscle adaptations. Indeed, exercise training protocols might lead to disruption of glucocorticoid release in rats, including corticosterone (Tharp, Citation1975). Indeed, corticosterone has been used as a key biomarker of stress in rodents (Contarteze, Manchado, Gobatto, & De Mello, Citation2008; Gong et al., Citation2015) and high levels of glucocorticoids are associated with skeletal muscle catabolism (Hasselgren, Citation1999). Moreover, short rest intervals have been shown to play a crucial role in skeletal muscle growth (De Souza et al., Citation2011; McKendry et al., Citation2016), resulting in increased muscle fatigue and diminished recovery phase (Ratamess et al., Citation2007). One of the responses that takes place during a rest period is the removal of lactate accumulated in the muscles (Ratamess et al., Citation2007), which might represent a metabolic parameter of energy sources restoration (ATP re-synthesis), affecting post-training recovery (Brooks, Citation1986; Menzies et al., Citation2010).
A progressive resistive exercise has been used for studies in rats, where jumps are performed in a cylinder containing water (jump-training) (Das Neves et al., Citation2013; De Souza et al., Citation2011), simulating squat training. In this regard, we have previously used genomic DNA extraction and protein content to show that jump-training did not elicit soleus (SOL) muscle hypertrophy in rats (Cunha, Tanno, Marcondes, Perez, & Selistre-Araújo, Citation2006). Considering the limited information regarding the characterization of RT models for rats, we sought to investigate the effects of a jump-training protocol on skeletal muscle adaptations and metabolic stress. We hypothesized that jump-training would affect muscle growth of active hindlimb skeletal muscles (SOL and extensor digitorum longus [EDL]), myosin heavy chain (MHC) isoform expression, and circulating corticosterone concentration.
Methods
Animals
Male Wistar rats (aged 60 days, weighing 250–300 g), and specific pathogen free (SPF), were housed in collective cages (four rats per cage), in a temperature-controlled room (22 ± 2 °C) with a 12:12-h light-dark cycle (with lights on from 07.00 to 19.00 h). The multidisciplinary Center of Research Biology (CEMIB) of UNICAMP supplied the rats. They received rodent chow and filtered drinking water ad libitum. All procedures were performed in compliance with The Guide for the Care and Use of Laboratory Animals published by the US National Institute of Health (NIH Publication No. 85-23, revised 1996), and the Institutional Committee for Ethics in Animal Research from the University of Campinas approved all experiments (Protocol Number 944-1). The rats were randomly assigned to non-trained or trained groups.
Experimental design
Procedures
The jump exercise protocol is illustrated in . Jump exercise has been used as a model of high intensity RT in our previous studies (Cunha et al., Citation2005a; Sousa et al., Citation2016). Briefly, 1 week was used as a period of adaptation of rats to the water and training apparatus. The adaptation was set in order to attenuate the water-induced stress without promoting physiological alterations in relation to the physical training (Contarteze et al., Citation2008). Trained rats performed four sets of 10 jumps in a polyvinyl chloride (PVC) cylinder tank measuring 50 cm in height, 25 cm in diameter, containing water (30 ± 2 °C) to a depth of 38 cm. Jump-training was performed 5 days a week for 1, 3, or 5 weeks with 30 s of inter-set rest intervals. In weeks 1 and 2, trained rats carried a load (fishing sinkers tied to the chest) that matched 50% of their body weight. In the following weeks 3 and 4, the load was adjusted to 60% of body weight. In week 5, the load was increased to 70% of body weight (). The weights were attached to the chest of the rats not only to avoid flotation but also to make them exercise against a load. The investigator allowed one repetition of the exercise when rats reached the top of the cylinder and breathed. The jump-exercise protocol occurred between 11:00 and 13:00 h to avoid the diurnal corticosterone peak, as observed in rodents after 20:00 h (Gong et al., Citation2015). Rats performed each session of jump-training over 15 s. Non-trained rats were placed into the cylinder containing water for 1 min, with no loads. Eight rats (n = 8/group) were used for each experimental week, totaling 48 rats per group throughout the experimental period. This approach was used both to reduce the stressor effect of water immersion and to ensure that the observed effects were in response to exercise training per se. Rats were weighed twice a week. To assess time-course effects, weeks 1, 3, or 5 were used in this study.
Table 1. Jump-training protocol.
Blood sampling, tissue collection, and analytical methods
Forty-eight hours after the experimental period, trained and non-trained rats were euthanized by decapitation with no previous anesthesia in order to avoid anesthetic-induced high corticosterone plasma levels (Vahl et al., Citation2005). Blood samples were immediately collected from the trunk into heparin-coated tubes. The blood was centrifuged (2000×g, 15 min, 4 °C), and plasma was isolated and stored at −20 °C. Plasma corticosterone was assayed using a Corticosterone Enzyme Immunoassay Kit (Assay Designs®, Ann Arbor, MI), with sensitivity of 0.027 ng/mL and the intra- and inter-assay coefficients of variation being 7.7 and 9.7%, respectively. Creatine kinase activity was measured spectrophotometrically at 37 °C by using a commercially available kit (CK-NAC UV unitest y AA Wiener lab, Rosario, Argentina) according to the manufacturer’s instructions. Replicates of samples were obtained in this condition (Level: 144.5 U/L; SD ± 3.36 U/L; C.V 2.33%). Assay sensitivity was 8 U/L and the intra- and inter-assay coefficients of variation were 2.33 and 2.12%, respectively.
Time-course of blood lactate in trained rats
In the last day of training from each week (week 1, 3, or 5), blood samples of trained rats were collected before the onset of training (rest), immediately after the last session of jumping and 20, 40, and 60 min post-training. Blood samples (25 µL) were collected from a cut at the tail tip and deposited in separate microcentrifuge tubes (1.5 mL) containing 50 µL of sodium fluoride (1%). Each rat’s tail was carefully dried with a paper towel for appropriate blood collection. Blood lactate was determined using a lactate analyzer (YSI model 1500 SPORT) (Simoes et al., Citation2003), coefficient of variation C.V < 5% (Halle, Citation2001). Assay sensitivity was 0.55 mmol/L and the intra- and inter-assay coefficients of variation were 0.79 and 0.72%, respectively.
Skeletal muscle tissue preparation
Right SOL and EDL muscles were quickly dissected from the rats, rinsed in ice-cold saline (0.9%), dried and weighed. A distal portion of the middle, belly was isolated from each muscle and frozen in liquid nitrogen. Samples were stored at −80 °C for morphology and cross-sectional area (CSA) analyses. Muscle weight was normalized relative to final body weight, matched by experimental week rats. Muscle weight-to-body weight ratio was expressed as muscle weight (mg)/body weight (g).
Morphological analysis
For the morphological study of SOL and EDL muscles, samples were cut (10-μm-thick cross-sections) using a cryostat (JUNG CM1800, Leica, Germany) at −20 °C and submitted to hematoxylin and eosin (H&E) staining. Images of four different regions were obtained using a light microscope. Images of CSA of 100 randomly chosen fibers were measured using a microscope attached to a computerized imaging analysis system (QWin Plus, Leica, Germany – 40X objective). CSA of ∼400 fibers of SOL and EDL muscles from each animal were used (n = 8/group, ∼3200 fibers per group).
Electrophoretic separation of myosin heavy chain (MHC) proteins
MHC isoform composition was determined by sodium dodecyl sulfate polyacrylamide gel electrophoresis (SDS-PAGE), as previously described (De Souza et al., Citation2011). SOL and EDL muscle samples were cut into 12 µm cross-sections using a cryostat (JUNG CM1800, Leica, Germany) at −20 °C. The samples were placed in a solution (0.5 mL) containing glycerol 10% (w/vol), 2-mercaptoethanol 5% (vol/vol), and sodium dodecyl sulfate (SDS) 2.3% (w/vol) in a Tris/HCl buffer 0.9% (pH 6.8) (w/vol). The final solution was shaken for 1 min and heated for 10 min at 60 °C. Small amounts of the extracts (28 µL) were submitted to electrophoresis (SDS-PAGE 7–10%), using a 4% stacking gel, for 26 h at 120 V. The gels were stained with Coomassie Blue and used to identify the MHC isoforms (MHC-I, MHC-IIA, MHC-IID, and MHC-IIB) according to their molecular weight. Images were captured by VDS Software (Pharmacia Biotech, Piscataway, NJ). The MHC isoforms were quantified by densitometry using Image Master VDS Software version 3.0 (Amersham Biosciences Inc. Piscataway, New Jersey), which determined the relative percentage of MHC content. All data were collected blindly.
Statistical analysis
All data, except MHC isoforms in EDL muscle, presented normal distribution (Kolmogorov–Smirnov test) and homoscedasticity (Bartlett’s test). Data with normal distribution were analyzed using parametric statistics (one-way ANOVA followed by Tukey’s post hoc test: with training as factor and weeks as levels of the factor) while non-parametric analysis was applied for MHC isoforms in EDL (Kruskal–Wallis test followed by Dunn’s multiple comparisons). Data were expressed as means ± SEM and significance level was set at .05 (p < .05). Statistical power = 0.8.
Results
The influence of RT on blood lactate is showed in . Higher blood lactate concentration was observed immediately after jump exercise compared to rest period in all weeks. The peak lactate concentration occurred after 20 min of training in weeks 1 and 3, while reduced lactate level was observed in week 5 compared to weeks 1 and 3 (p < .0001; F = 28.69; ). After 40 min of training, lactate concentration was decreased in all weeks (vs. previous times of lactate measurement). Although corticosterone concentration showed higher concentrations in trained rats compared to non-trained in weeks 1 and 3, but the values were not statistically different; however in week 5, we found a significant increase of corticosterone in trained rats compared to non-trained (p = .002; F = 4.79; ). Creatine kinase activity was unchanged in non-trained and trained rats over the weeks ().
Figure 2. Circulating lactate concentration measured at rest, immediately after, 20, 40, and 60 min post-training of trained rats (a). Plasma corticosterone concentrations (b) and creatine kinase activity (c) in non-trained and trained rats after 1, 3, or 5 weeks. Values are expressed as mean ± SEM. One-way ANOVA followed by Tukey’s post-test (p < .05): avs. previous time of lactate measurement; bvs. week 5; &vs. week 5 of non-trained group. n = 8/group.
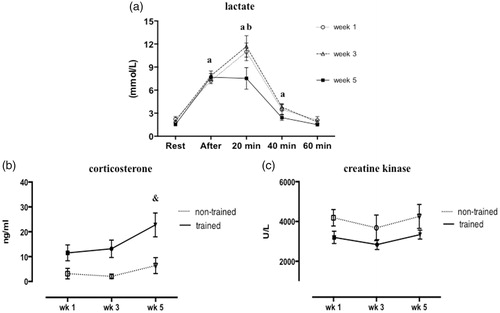
Trained and non-trained groups did not present differences in body weight after week 1 of the experimental period. Non-trained rats gained weight over the weeks (week 3 vs. week 1 and week 5 vs. week 3), whereas body weight of trained rats increased only in week 5, as compared with previous weeks. However, trained rats exhibited lower body weight in week 3 than the matched week of non-trained rats (p < .0001; F = 28.20; ).
Table 2. Body weight, absolute (MW) and relative (MW/BW) weights of soleus and extensor digitorum longus (EDL) muscles of non-trained and trained rats after 1, 3, or 5 weeks.
Actual weights of SOL and EDL muscles increased in the non-trained group after week 3 and 5 in relation to week 1 (p < .0001; F = 18.25 − SOL; F = 20.95 − EDL; ). Weights of both muscles decreased after 3 weeks of jump-training when compared with their matched non-trained rats. However, EDL muscle weight was reduced after 5 weeks of training in comparison with the same week of non-trained rats (p < .0001). MW/BW ratio of SOL was unchanged in both groups over the 5-week experimental period. While MW/BW ratio of EDL did not alter in non-trained rats, 1 and 3 weeks of training elevated MW/BW of EDL in relation to non-trained rats. Noteworthy is that MW/BW of EDL muscle was significantly lower after week 5 weeks in the trained rats than in the non-trained group (p < .0001; F = 11.70; ).
Histological mages of SOL and EDL muscles are shown in . SOL and EDL CSAs increased in the untrained group over the weeks (week 3 vs. week 1 and week 5 vs. week 3) (). In trained rats, CSA of SOL muscle was reduced after 3 weeks of training when compared with the matched week of untrained rats (p < .0001; F = 11.09 − SOL; ). After 5 weeks of training, both types of muscle showed marked reduction of CSA in relation to week 5 in non-trained rats (p = .0002; F = 6.94 − EDL; ).
Figure 3. Images of soleus (SOL) and extensor digitorum longus (EDL) muscles stained with hematoxylin and eosin (H&E) after 1, 3, or 5 weeks of the experimental period. Representative images were taken at 40x magnification. Groups: non-trained and trained rats. *vs. week 1 of the same group; #vs. week 3 of non-trained group; &vs. week 5 of non-trained group. n = 8/group.
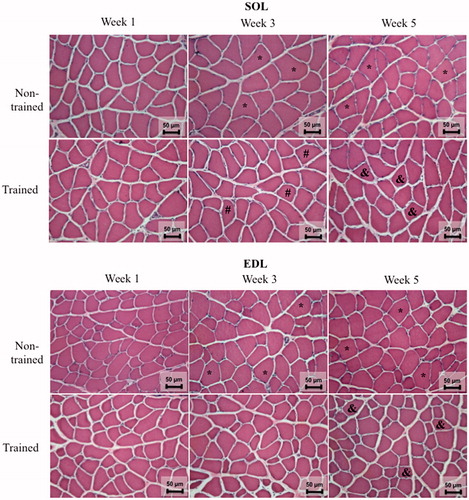
Table 3. Cross-sectional area (CSA) of soleus and extensor digitorum longus (EDL) muscles of non-trained and trained rats after 1, 3, or 5 weeks.
In SOL muscle, no changes were observed in MHC-I and MHC-IIA contents after weeks 1 and 3. However, exercise training decreased MHC-I and MHC-IIA contents in SOL muscle of trained rats after week 5 when compared with the matched week of non-trained rats (trained vs. non-trained) (p = .01; F = 9.35; ). Expression of MHC-IIb and MHC-IId in SOL muscle was not detected. In EDL muscle, no significant differences were observed in MHC isoforms (I, IIA, IID, and MHC-IIB) (Kruskal–Wallis test p > .05, ).
Figure 4. Relative myosin heavy chain (MHC) isoform distribution of MHC-I (a) and MHC-IIA (b) in soleus (SOL) muscle of non-trained and trained rats after 1, 3, or 5 weeks. Values are expressed as means ± SEM. One-way ANOVA followed by Tukey’s post-test (p < .05): &vs. week 5 of non-trained group. n = 8/group.
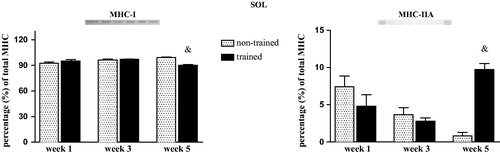
Figure 5. Relative myosin heavy chain (MHC) isoform expression of MHC-I (a), MHC-IIA (b), MHC-IID (c) and MHC-IIB (d) in extensor digitorum longus (EDL) muscle of non-trained and trained rats after 1, 3, or 5 weeks. Values are expressed as means ± SEM. No statistically significant differences: Kruskal–Wallis (p > .05). n = 8/group.
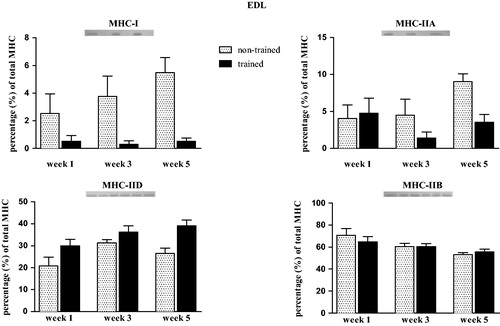
Discussion
Our major findings reveal the detrimental effects of a jump-training protocol (characterized by high-load, large volume, and short rest intervals) on skeletal muscle adaptations and metabolic stress. We provide clear evidence that pronounced metabolic stress and impairment of muscle growth might take place when variables of exercise training are not appropriately manipulated. Whereas some caution is warranted regarding the manipulation of the training variables, it is important to highlight that we have extensively studied jump exercise as a suitable model of RT for rats (Cunha et al., Citation2005a; Citation2006; Das Neves et al., Citation2013; Sousa et al., Citation2016; Tanno et al., Citation2011). In this study, we observed that jump-training induced a higher peak of blood lactate after 20 min of training in weeks 1 and 3, even though a lactate level peak was not observed after 20 min of training in week 5 (). Creatine kinase, in turn, was unchanged between the groups over the weeks. Jump-training also induced negative effects on hypertrophy of type-I and II muscle fibers, while plasma concentrations of corticosterone were elevated after 5 weeks of training.
Variables of exercise training including intensity, volume, duration, and exercise modality are potential factors that alter body composition (Xiao & Fu, Citation2015). Considering that trained rats gained less weight than their non-trained counterparts over the weeks, as well as body weight of trained rats being lower after 3 weeks of training, we might suggest that the jump exercise protocol, at least with regard to rat growth plays a crucial role in whole-body composition, as supported by our previous studies (Cunha, Tanno, Costa Sampaio Moura, & Marcondes, Citation2005b; Das Neves et al., Citation2013).
There has been a controversy about whether RT-induced high plasma creatine kinase levels reflects skeletal muscle damage (Baird, Graham, Baker, & Bickerstaff, Citation2012). We did not observe any changes in plasma creatine kinase activity after 1, 3, or 5 weeks of training, suggesting that creatine kinase activity might not represent a direct responsive biomarker of muscle damage, and indeed, previous studies have shown no association between CK activity and muscle damage (Komulainen, Takala, & Vihko, Citation1995; Magal et al., Citation2010).
In order to investigate glucose metabolism in response to jump-training over the weeks, blood lactate concentration was measured in trained rats. While ATP is produced faster anaerobically (by glycolysis or glycogenolysis) when glucose is metabolized to lactate (Shulman, Citation2005), lactate removal might be indicative of adaptation to exercise training (Watanabe et al., Citation1992). Increased lactate concentration after 20 min of jump exercise was observed after weeks 1 and 3. However, lactate level after 20 min of jump exercise was significantly reduced in week 5 (vs. weeks 1 and 3), suggesting a transition from glucose metabolism to lipid oxidation and/or muscle triglyceride utilization (Bielinski, Schutz, & Jéquier, Citation1985). In this sense, lower lactate concentration in week 5 might be an indicator of over-training/over-reaching, as reported in other studies (Bosquet, Léger, & Legros, Citation2001; Jeukendrup & Hesselink, Citation1994). Indeed, over-training syndrome has been shown to induce oxidative stress and inhibit skeletal muscle growth (Xiao, Chen, & Dong, Citation2012; Zoppi & MacEdo, Citation2007). Accordingly, changes in intracellular mechanisms have been observed during post-exercise recovery, including enhanced lactate clearance (Menzies et al., Citation2010), lactate transport by monocarboxylate transporters (MCTs), lactate oxidation by skeletal muscle (mainly type-I fiber and myocardium) and alteration in lactate dehydrogenase (LDH) activity (Bangsbo, Graham, Johansen, & Saltin, Citation1994; Brooks, Citation1986; van Hall, Citation2010). Moreover, our research group has demonstrated overexpression of LDH protein in cardiac tissue from rats submitted to jump-training (Sousa et al., Citation2016), which might support our findings. However, further analyses are warranted to better characterize the phenomenon.
We observed increases in plasma corticosterone after week 5, suggesting an effect of training duration on metabolic stress. It has been shown that combination of high-frequency and elevated duration of exercise leads to an exhaustive condition for rats and thereafter impairs muscle beneficial adaptations (Contarteze et al., Citation2008; Demirel, Powers, Naito, Hughes, & Coombes, Citation1999). Moreover, increases in plasma corticosterone are consistently documented in different exercise training models (Contarteze et al., Citation2008; Gong et al., Citation2015). Of note, while exercise protocols in an aquatic environment could induce metabolic stress per se, we exclude this possibility, as trained rats were submitted to an adaptation period (over 1 week). Higher corticosterone levels could imply onset of a stress condition in response to the exercise protocol, given that corticosterone is evidently a more adaptation-related stress biomarker than cortisol during chronic stress (Gong et al., Citation2015). However, we did not observe increase corticosterone levels after week 1 of training, which suggests that duration and volume of training play an important role in the metabolic stress.
In terms of muscle adaptations, EDL muscle seems to be negatively affected by the duration of training, as observed in our findings ( and ). We hypothesized that EDL responses induced by jump-training after 5 weeks would be related to higher plasma corticosterone levels observed in the same week (), as there is a strong correlation between glucocorticoids and protein muscle catabolism (Pereira & Freire de Carvalho, Citation2011; Schakman, Gilson, & Thissen, Citation2008). However, Aguiar et al. demonstrated that jump-training did not elicit changes in SOL weight and SOL weight/body weight ratio, which might be related to longer rest interval used in the study (40 s) (Aguiar et al., Citation2010). By contrast, climbing a ladder (Hornberger & Farrar, Citation2004) and squat-training (Tamaki, Uchiyama, & Nakano, Citation1992) have been shown to induce muscle hypertrophy in rats. In this study, SOL and EDL muscles were used to investigate the effects of jump-training on fiber types (SOL – slow-twitch fiber or type I; EDL – fast-twitch fiber or type II) (Delp & Duan, Citation1996). Pronounced reduction of MW/BW ratio was observed in both muscles of trained rats over the 5-week training period. Furthermore, while SOL weight seems to be affected only after 3 weeks of training, MW/BW of EDL muscle was reduced after weeks 3 and 5, suggesting that frequency of training plays a detrimental role in muscle fibers, particularly those composed predominantly of type II fibers. This might be supported, at least in part, by our findings from CSA measurements in EDL muscle. Reduced CSA of EDL muscle was observed after 5 weeks of training, while 3 and 5 weeks of training-induced lower CSA of SOL muscle (). However, SOL weight (actual MW and MW/BW ratio) was not reduced in week 3. Indeed, inconsistent findings might be explained by different characteristics of experimental models, RT protocols and muscle growth measurements. For example, muscle weight has been used to study muscle mass (Kobayashi et al., Citation2016), which might include connective tissues (Tamaki & Uchiyama, Citation1995). CSA, in turn, reflects muscle development (hypertrophy or atrophy) by measuring single muscle fiber size (diameter or circumference) (Tamaki & Uchiyama, Citation1995). In this regard, ladder climbing exercise resulted in increased CSA of flexor hallucis longus, but not in plantar flexors (SOL, plantaris, gastrocnemius, and quadriceps), suggesting that muscle activation depends on the specific exercise (Hornberger & Farrar, Citation2004). As glucocorticoid-induced muscle atrophy affects mainly fast-twitch muscle fibers (type II), predominantly IIb fibers (Schakman et al., Citation2008), it appears that corticosterone play a key role in the EDL responses, even though our findings do not rely on a mechanistic approach, as several factors contribute to the muscle atrophy in trained rats (Kwon et al., Citation2018). The underlying mechanisms by which glucocorticoids inhibit muscle protein synthesis have been consistently described (Schakman et al., Citation2008).
MHC isoforms represent a feasible marker of fiber type transition in response to duration of exercise training (Demirel et al., Citation1999). Indeed, both reduction in MHC-I and increases in MHC-IIA contents were seen after 5 weeks in SOL muscle of trained rats (), which are in accordance with others using endurance training (Demirel et al., Citation1999). In addition, RT increased MHC-IIA in SOL muscle of rats (Aguiar et al., Citation2010). SOL muscle has been shown to be highly recruited after jump-training, as supported by others (Aguiar et al., Citation2010). However, no differences were found in MHC contents of EDL muscle (). Nevertheless, the findings about EDL muscle could be explained by the conservative statistical analysis (Kruskal–Wallis test followed by Dunn’s multiple comparisons). Endurance training, in turn, was shown to decrease percentage of MHC-IIB, while increases in MHC-I, IIA, and IID were observed in EDL muscle (Demirel et al., Citation1999). Endurance training induced a decrease in the percentage of MHC-IIB and an increase in the MHC-IIA of plantaris muscle (Sullivan et al., Citation1995). Whereas plantaris muscle is composed of a mixture of fibers (Delp & Duan, Citation1996), and is activated in response to jump (De Souza et al., Citation2011) or squat training (Tamaki et al., Citation1992), no studies have investigated previously the effects of jump-training on growth of SOL and EDL muscles. Accordingly, our findings also suggest that muscle atrophy is dependent upon fiber type composition. A limitation of our study was that we did not measure muscle hypertrophy after 2, 4, or 6 weeks of training. Moreover, future research related to anabolic signaling (testosterone, growth factors, mammalian target of rapamycin, -mTOR, and p70S6 kinase), proteolysis processes (proinflammatory cytokines and ubiquitin ligases), gene regulation and protein expression is warranted to extend our findings.
Although detrimental effects on skeletal muscle and elevated metabolic stress were observed when high loads, large volume, and short rest intervals are applied in the jump-training, we have previously shown several beneficial effects of the jump-training protocol, including endothelial function (Cunha et al., Citation2005a; Guzzoni et al., Citation2018), cardiac remodeling and function (Tanno et al., Citation2011) and reduced plasma triglyceride levels (Cunha et al., Citation2005b). Hence, a better understanding of the effects of a RT program might provide important insights in order to preclude stress to rats and lead to important clinical insights in terms of exercise prescription.
Conclusion
Five weeks of jump exercise-induced marked wasting of hind-limb muscles (type I and II fibers) concomitant with elevated plasma corticosterone. Our results demonstrate that pronounced metabolic stress and impairment of muscle development might take place when variables of exercise training are not appropriately manipulated (i.e. high-load, large volume, and short rest intervals).
Disclosure statement
No potential conflict of interest was reported by the author.
Additional information
Funding
Notes on contributors
Vinicius Guzzoni
Vinicius Guzzoni, Larissa Briet, Maeli Dal-Pai-Silva, Tatiana S. Cunha and Fernanda K. Marcondes elaborated the study.
Vinicius Guzzoni, Larissa Briet, Rafaela Costa, Rodrigo W. A. Souza and Fernanda R. Carani performed the experiments.
Vinicius Guzzoni, Larissa Briet, Rafaela Costa, Rodrigo W. A. Souza, Fernanda R. Carani, Maeli Dal-Pai-Silva, Kleiton A. S. Silva, Tatiana S. Cunha and Fernanda K. Marcondes analysed the results and wrote the manuscript.
Larissa Briet
Vinicius Guzzoni, Larissa Briet, Maeli Dal-Pai-Silva, Tatiana S. Cunha and Fernanda K. Marcondes elaborated the study.
Vinicius Guzzoni, Larissa Briet, Rafaela Costa, Rodrigo W. A. Souza and Fernanda R. Carani performed the experiments.
Vinicius Guzzoni, Larissa Briet, Rafaela Costa, Rodrigo W. A. Souza, Fernanda R. Carani, Maeli Dal-Pai-Silva, Kleiton A. S. Silva, Tatiana S. Cunha and Fernanda K. Marcondes analysed the results and wrote the manuscript.
Rafaela Costa
Vinicius Guzzoni, Larissa Briet, Rafaela Costa, Rodrigo W. A. Souza and Fernanda R. Carani performed the experiments.
Vinicius Guzzoni, Larissa Briet, Rafaela Costa, Rodrigo W. A. Souza, Fernanda R. Carani, Maeli Dal-Pai-Silva, Kleiton A. S. Silva, Tatiana S. Cunha and Fernanda K. Marcondes analysed the results and wrote the manuscript.
Rodrigo W. A. Souza
Vinicius Guzzoni, Larissa Briet, Rafaela Costa, Rodrigo W. A. Souza and Fernanda R. Carani performed the experiments.
Vinicius Guzzoni, Larissa Briet, Rafaela Costa, Rodrigo W. A. Souza, Fernanda R. Carani, Maeli Dal-Pai-Silva, Kleiton A. S. Silva, Tatiana S. Cunha and Fernanda K. Marcondes analysed the results and wrote the manuscript.
Fernanda R. Carani
Vinicius Guzzoni, Larissa Briet, Maeli Dal-Pai-Silva, Tatiana S. Cunha and Fernanda K. Marcondes elaborated the study.
Vinicius Guzzoni, Larissa Briet, Rafaela Costa, Rodrigo W. A. Souza, Fernanda R. Carani, Maeli Dal-Pai-Silva, Kleiton A. S. Silva, Tatiana S. Cunha and Fernanda K. Marcondes analysed the results and wrote the manuscript.
Maeli Dal-Pai-Silva
Vinicius Guzzoni, Larissa Briet, Maeli Dal-Pai-Silva, Tatiana S. Cunha and Fernanda K. Marcondes elaborated the study.
Vinicius Guzzoni, Larissa Briet, Rafaela Costa, Rodrigo W. A. Souza, Fernanda R. Carani, Maeli Dal-Pai-Silva, Kleiton A. S. Silva, Tatiana S. Cunha and Fernanda K. Marcondes analysed the results and wrote the manuscript.
Kleiton A. S. Silva
Vinicius Guzzoni, Larissa Briet, Rafaela Costa, Rodrigo W. A. Souza, Fernanda R. Carani, Maeli Dal-Pai-Silva, Kleiton A. S. Silva, Tatiana S. Cunha and Fernanda K. Marcondes analysed the results and wrote the manuscript.
Fernanda K. Marcondes
Vinicius Guzzoni, Larissa Briet, Rafaela Costa, Rodrigo W. A. Souza and Fernanda R. Carani performed the experiments.
Vinicius Guzzoni, Larissa Briet, Rafaela Costa, Rodrigo W. A. Souza, Fernanda R. Carani, Maeli Dal-Pai-Silva, Kleiton A. S. Silva, Tatiana S. Cunha and Fernanda K. Marcondes analysed the results and wrote the manuscript.
References
- Aguiar, A.F., Aguiar, D.H., Felisberto, A.D.S., Carani, F.R., Milanezi, R.C., Padovani, C.R., & Dal-Pai-Silva, M. (2010). Effects of creatine supplementation during resistance training on myosin heavy chain (MHC) expression in rat skeletal muscle fibers. Journal of Strength and Conditioning Research, 24, 88–96. doi: 10.1519/JSC.0b013e3181aeb103
- Bågenhammar, S., & Hansson, E.E. (2007). Repeated sets or single set of resistance training - A systematic review. Advances in Physiotherapy, 9, 154–160. doi:10.1080/14038190701554277
- Baird, M.F., Graham, S.M., Baker, J.S., & Bickerstaff, G.F. (2012). Creatine-kinase- and exercise-related muscle damage implications for muscle performance and recovery. Journal of Nutrition and Metabolism, 2012, 1–13. doi: 10.1155/2012/960363
- Bangsbo, J., Graham, T., Johansen, L., & Saltin, B. (1994). Muscle lactate metabolism in recovery from intense exhaustive exercise: Impact of light exercise. Journal of Applied Physiology, 77, 1890–1895. doi: 10.1152/jappl.1994.77.4.1890
- Bielinski, R., Schutz, Y., & Jéquier, E. (1985). Energy metabolism during the postexercise recovery in man. The American Journal of Clinical Nutrition, 42, 69–82. doi: 10.1093/ajcn/42.1.69
- Bosquet, L., Léger, L., & Legros, P. (2001). Blood lactate response to overtraining in male endurance athletes. European Journal of Applied Physiology, 84, 107–114. doi:10.1007/s004210000343
- Brooks, G.A. (1986). The lactate shuttle during exercise and recovery. Medicine and Science in Sports and Exercise, 18, 360–368. doi: 10.1249/00005768-198606000-00019
- Burd, N.A., West, D.W.D., Staples, A.W., Atherton, P.J., Baker, J.M., Moore, D.R., … Phillips, S.M. (2010a). Low-load high volume resistance exercise stimulates muscle protein synthesis more than high-load low volume resistance exercise in young men. PLoS One, 5, e12033. doi:10.1371/journal.pone.0012033
- Burd, N.A., Holwerda, A.M., Selby, K.C., West, D.W.D., Staples, A.W., Cain, N.E., … Phillips, S.M. (2010b). Resistance exercise volume affects myofibrillar protein synthesis and anabolic signalling molecule phosphorylation in young men. The Journal of Physiology, 588, 3119–3130. doi: 10.1113/jphysiol.2010.192856
- Cholewa, J., Guimarães-Ferreira, L., da Silva Teixeira, T., Naimo, M.A., Zhi, X., de Sá, R.B., … Zanchi, N.E. (2014). Basic models modeling resistance training: An update for basic scientists interested in study skeletal muscle hypertrophy. Journal of Cellular Physiology, 229, 1148–1156. doi: 10.1002/jcp.24542
- Contarteze, R.V.L., Manchado, F.D.B., Gobatto, C.A., & De Mello, M.A R. (2008). Stress biomarkers in rats submitted to swimming and treadmill running exercises. Comparative Biochemistry and Physiology Part A: Molecular & Integrative Physiology, 151, 415–422. doi: 10.1016/j.cbpa.2007.03.005
- Cunha, T.S., José, M., Moura, C.S., Bernardes, C.F., Tanno, A.P., & Marcondes, F.K. (2005a). Vascular sensitivity to phenylephrine in rats submitted to anaerobic training and nandrolone treatment. Hypertension, 46, 1010–1015. doi: 10.1161/01.HYP.0000174600.51515.e7
- Cunha, T.S., Tanno, A.P., Costa Sampaio Moura, M.J., & Marcondes, F.K. (2005b). Influence of high-intensity exercise training and anabolic androgenic steroid treatment on rat tissue glycogen content. Life Science, 77, 1030–1043. doi: doi:10.1016/j.lfs.2005.03.001
- Cunha, T.S., Tanno, A.P., Marcondes, F.K., Perez, S.E.A., & Selistre-Araújo, H.S. (2006). A administração de nandrolona não promove hipertrofia do músculo sóleo em ratos. Arquivos Brasileiros de Endocrinologia & Metabologia, 50, 532–540. doi: 10.1590/S0004-27302006000300017
- Das Neves, V.J., Tanno, A.P., Cunha, T.S., Fernandes, T., Guzzoni, V., Da Silva, C.A., … Marcondes, F.K. (2013). Effects of nandrolone and resistance training on the blood pressure, cardiac electrophysiology, and expression of atrial β-adrenergic receptors. Life Science, 92, 1029–1035. doi: 10.1016/j.lfs.2013.04.002
- De Souza, R.W.A., Aguiar, A.F., Carani, F.R., Campos, G.E.R., Padovani, C.R., & Silva, M.D.P. (2011). High-intensity resistance training with insufficient recovery time between bouts induce atrophy and alterations in myosin heavy chain content in rat skeletal muscle. The Anatomical Record: Advances in Integrative Anatomy and Evolutionary Biology, 294, 1393–1400. doi: 10.1002/ar.21428
- Delp, M.D., & Duan, C. (1996). Composition and size of type I, IIA, IID/X, and IIB fibers and citrate synthase activity of rat muscle. Journal of Applied Physiology, 80, 261–270. doi: 10.1152/jappl.1996.80.1.261
- Demirel, H. A., Powers, S.K., Naito, H., Hughes, M., & Coombes, J.S. (1999). Exercise-induced alterations in skeletal muscle myosin heavy chain phenotype: Dose-response relationship. Journal of Applied Physiology, 86, 1002–1008. doi: 10.1152/jappl.1999.86.3.1002
- Fink, J.E., Schoenfeld, B.J., Kikuchi, N., & Nakazato, K. (2017). Acute and long-term responses to different rest intervals in low-load resistance training. International Journal of Sports Medicine, 38, 118–124. doi: 10.1055/s-0042-119204
- Gong, S., Miao, Y.L., Jiao, G.Z., Sun, M.J., Li, H., Lin, J., … Tan, J.H. (2015). Dynamics and correlation of serum cortisol and corticosterone under different physiological or stressful conditions in mice. PLoS One, 10, 1–14. doi:10.1371/journal.pone.0117503
- Grgic, J., Lazinica, B., Mikulic, P., Krieger, J.W., & Schoenfeld, B.J. (2017). The effects of short versus long inter-set rest intervals in resistance training on measures of muscle hypertrophy: A systematic review. European Journal of Sport Science, 17, 1–11. doi:10.1080/17461391.2017.1340524
- Guzzoni, V., Cunha, T.S., das Neves, V.J., Briet, L., Costa, R., Moura, M.J.C.S., … Marcondes, F.K. (2018). Nandrolone combined with strenuous resistance training reduces vascular nitric oxide bioavailability and impairs endothelium-dependent vasodilation. Steroids, 131, 7–13. doi: 10.1016/j.steroids.2017.12.013
- Halle, J. (2001). Changes in human skeletal muscle contractility and hormone status during 2 weeks of heavy strength training. European Journal of Applied Physiology, 84, 54–63. doi:10.1007/s004210000328
- Hasselgren, P.O. (1999). Glucocorticoids and muscle catabolism. Current Opinion in Clinical Nutrition and Metabolic Care, 2, 201–205. doi: 10.1097/00075197-199905000-00002
- Hornberger, T.A., & Farrar, R.P. (2004). Physiological hypertrophy of the FHL muscle following 8 weeks of progressive resistance exercise in the rat. Canadian Journal of Applied Physiology, 29, 16–31. doi: 10.1139/h04-002
- Ikezoe, T., Kobayashi, T., Nakamura, M., & Ichihashi, N. (2017). Effects of low-load, higher-repetition versus high-load, lower-repetition resistance training not performed to failure on muscle strength, mass, and echo intensity in healthy young men: A time-course study. The Journal of Strength & Conditioning Research, 1. doi: 10.1519/JSC.0000000000002278
- Jeukendrup, A.E., & Hesselink, M.K. (1994). Overtraining–what do lactate curves tell us? British Journal of Sports Medicine, 28, 239–240. doi: 10.1136/bjsm.28.4.239
- Kobayashi, Y., Somoto, Y., Mitsuyama, E., Tanaka, A., Yuda, N., Nakada, H., … Nagasawa, T. (2016). Supplementation of protein-free diet with whey protein hydrolysates prevents skeletal muscle mass loss in rats. Journal of Nutrition & Intermediary Metabolism, 4, 1–5. doi: 10.1016/j.jnim.2016.03.001
- Komulainen, J., Takala, T.E., & Vihko, V. (1995). Does increased serum creatine kinase activity reflect exercise-induced muscle damage in rats? International Journal of Sports Medicine, 16, 150–154. doi: 10.1055/s-2007-972983
- Kwon, I., Jang, Y., Cho, J.Y., Jang, Y.C., & Lee, Y. (2018). Long-term resistance exercise-induced muscular hypertrophy is associated with autophagy modulation in rats. The Journal of Physiological Sciences, 68, 1–12. doi:10.1007/s12576-017-0531-2
- Lorenz, D.S., Reiman, M.P., & Walker, J.C. (2010). Periodization: Current review and suggested implementation for athletic rehabilitation. Sports Health: A Multidisciplinary Approach, 2, 509–518. doi: 10.1177/1941738110375910
- Magal, M., Dumke, C.L., Urbiztondo, Z.G., Cavill, M.J., Triplett, N.T., Quindry, J.C., … Epstein, Y. (2010). Relationship between serum creatine kinase activity following exercise-induced muscle damage and muscle fibre composition. Journal of Sports Sciences, 28, 257–266. doi: 10.1080/02640410903440892
- McKendry, J., Pérez-López, A., McLeod, M., Luo, D., Dent, J.R., Smeuninx, B., … Breen, L. (2016). Short inter-set rest blunts resistance exercise-induced increases in myofibrillar protein synthesis and intracellular signalling in young males. Experimental Physiology, 101, 866–882. doi: 10.1113/EP085647
- Menzies, P., Menzies, C., McIntyre, L., Paterson, P., Wilson, J., & Kemi, O.J. (2010). Blood lactate clearance during active recovery after an intense running bout depends on the intensity of the active recovery. Journal of Sports Science, 28, 975–982. doi: 10.1080/02640414.2010.481721
- Ogasawara, R., Arihara, Y., Takegaki, J., Nakazato, K., & Ishii, N. (2017). Relationship between exercise volume and muscle protein synthesis in a rat model of resistance exercise. Journal of Applied Physiology, 123, 710–716. doi: 10.1152/japplphysiol.01009.2016
- Pereira, R.M.R., & Freire de Carvalho, J. (2011). Glucocorticoid-induced myopathy. Joint Bone Spine, 78, 41–44. doi: 10.1016/j.jbspin.2010.02.025
- Ratamess, N.A., Falvo, M.J., Mangine, G.T., Hoffman, J.R., Faigenbaum, A.D., & Kang, J. (2007). The effect of rest interval length on metabolic responses to the bench press exercise. European Journal of Applied Physiology, 100, 1–17. doi: 10.1007/s00421-007-0394-y
- Schakman, O., Gilson, H., & Thissen, J.P. (2008). Mechanisms of glucocorticoid-induced myopathy. Journal of Endocrinology, 197, 1–10. doi: 10.1677/JOE-07-0606
- Shulman, R.G. (2005). Glycogen turnover forms lactate during exercise. Exercise and Sport Sciences Reviews, 33, 157–162. doi: 10.1097/00003677-200510000-00002
- Simoes, H.G., Gampbell, C.S.G., Kushnick, M.R., Nakamura, A., Katsanos, C.S., Baldissera, V., & Moffatt, R.J. (2003). Blood glucose threshold and the metabolic responses to incremental exercise tests with and without prior lactic acidosis induction. Journal of Applied Physiology, 89, 603–611. doi: 10.1007/s00421-003-0851-1
- Sousa, P., Mayumi, M., Dinéia, J., Lucia, R., Watanabe, H., César, F., … Cunha, T.S. (2016). Unraveling the role of high-intensity resistance training on left ventricle proteome: Is there a shift towards maladaptation? Life Science, 152, 156–164. doi: 10.1016/j.lfs.2016.03.040
- Sullivan, V.K., Powers, S.K., Criswell, D.S., Tumer, N., Larochelle, J.S., & Lowenthal, D. (1995). Myosin heavy chain composition in young and old rat skeletal muscle: Effects of endurance exercise. Journal of Applied Physiology, 78, 2115–2120. doi: 10.1152/jappl.1995.78.6.2115
- Tamaki, T., & Uchiyama, S. (1995). Absolute and relative growth of rat skeletal muscle. Physiology & Behavior, 57, 913–919. doi: 10.1016/0031-9384(94)00359-D Retrieved from 10.1016/0031-9384(94)00359-D
- Tamaki, T., Uchiyama, S., & Nakano, S. (1992). A weight-lifting exercise model for inducing hypertrophy in the hindlimb muscles of rats. Medicine & Science in Sports & Exercise, 24, 881–886. doi:10.1249/00005768-199208000-00009
- Tanno, A.P., Neves, V.J.D., Rosa, K.T., Cunha, T.S., Giordano, F.C.L., Calil, C.M., … Moura, M.J.C.S. (2011). Nandrolone and resistance training induce heart remodeling: Role of fetal genes and implications for cardiac pathophysiology. Life Science, 89, 631–637. doi: 10.1016/j.lfs.2011.08.004
- Tharp, G.D. (1975). The role of glucocorticoids in exercise. Medicine and Science in Sports, 7, 6–11. Retrieved from http://www.ncbi.nlm.nih.gov/pubmed/1143055
- Vahl, T.P., Ulrich-Lai, Y.M., Ostrander, M.M., Dolgas, C.M., Elfers, E.E., Seeley, R.J., … Herman, J.P. (2005). Comparative analysis of ACTH and corticosterone sampling methods in rats. American Journal of Physiology-Endocrinology and Metabolism, 289, E823–E828. doi: 10.1152/ajpendo.00122.2005
- van Hall, G. (2010). Lactate kinetics in human tissues at rest and during exercise. Acta Physiologica, 199, 499–508. doi: 10.1111/j.1748-1716.2010.02122.x
- Watanabe, T., Morimoto, A., Sakata, Y., Tan, N., Morimoto, K., & Murakami, N. (1992). Running training attenuates the ACTH responses in rats to swimming and cage-switch stress. Journal of Applied Physiology, 73, 2452–2456. doi: 10.1152/jappl.1992.73.6.2452
- Xiao, W., Chen, P., & Dong, J. (2012). Effects of overtraining on skeletal muscle growth and gene expression. International Journal of Sports Medicine, 33, 846–853. doi: 10.1055/s-0032-1311585
- Xiao, T., & Fu, Y.F. (2015). Resistance training vs. aerobic training and role of other factors on the exercise effects on visceral fat. European Review for Medical and Pharmacological Sciences, 19, 1779–1784. http://www.ncbi.nlm.nih.gov/pubmed/26044220
- Zoppi, C.C., & MacEdo, D.V. (2007). Overreaching-induced oxidative stress, enhanced HSP72 expression, antioxidant and oxidative enzymes downregulation. Scandinavian Journal of Medicine & Science in Sports, 18, 67–76. doi: 10.1111/j.1600-0838.2006.00630.x