Abstract
This study examined the underlying neural mechanism of acute stress affecting creative thinking. Twenty-six male participants underwent the Alternative Uses Test before and after acute stress test (Montreal Imaging Stress Task). Compared to before stress, stressed participants responded with higher salivary cortisol and salivary alpha-amylase. The electroencephalogram results indicated a general decrease in upper-frequency alpha power after stress, compared with that of before stress. The decrease in upper-frequency alpha power observed in the first stage after exposure to stress was related to memory and attention. Additionally, stress also reduced the differences between individuals with different creative abilities. In summary, these findings indicated that acute stress impaired creative thinking and primarily affected the earlier phase of the process of creative cognition. This study provides some practical implications for educational practice and corporate innovation in that a more relaxed environment promotes creative output.
Lay Summary
Acute stress impaired creative thinking performance and mainly affects the earlier phase of the process of creative cognition. Additionally, stress seems to reduce the differences between individuals with different creative abilities.
Introduction
Many creative works were completed under stress. Yet, the reason whether we were quick-witted or ineffective in an emergency was unpredictable. It was especially an important issue in modern society as many individuals face psychological stress in various aspects of their lives. Therefore, we investigated the impact of stress on the creative idea generation process.
Stress is accompanied with unpredictable, uncontrollable, and threatening characteristics to adapt to external situations (Byron, Khazanchi, & Nazarian, Citation2010; McEwen, Citation2000). When individuals confront stress, the sympathetic nervous system (SNS) and the hypothalamus–pituitary–adrenal (HPA) axis are activated (Günzel, Citation2014). In humans, salivary alpha-amylase (Nater & Rohleder, Citation2009; Thoma, Kirschbaum, Wolf, & Rohleder, Citation2012) and cortisol (Foley & Kirschbaum, Citation2010; Kirschbaum & Hellhammer, Citation1989) levels are regarded as good indicators of SNS activity and HPA axis activity, respectively.
Creativity is commonly defined as the ability to produce work that is both novel and appropriate (Sternberg & Lubart, Citation1996). Divergent thinking, the core element of creativity (Guilford, Citation1987), has been a topic of interest in creativity research. Divergent problem solving requires answer as many original uses as possible (Christensen, Guilford, & Wilson, Citation1957). Several theoretical models of creativity have been established to describe creative cognition and its possible phases. The number of phases involved in the creative process varies among different theories (Basadur, Graen, & Green, Citation1982; Campbell, Citation1960; Csikszentmihalyi, Citation1996; Finke, Ward, & Smith, Citation1992; Simonton, Citation2011, Citation2013). For example, creative thought depends on the twofold procedure of blind variation (BV) and selective retention (SR), or BVSR (Campbell, Citation1960; Simonton, Citation2011, Citation2013). Similarly, Basadur et al. (Citation1982) proposed that creative thinking begins by generating crudely formed ideas and associations, followed by their exploration through evaluation and testing (Finke et al., Citation1992). Behavioral evidence indicated that individuals’ abilities of creative ideas generation and creative idea evaluation are independent of each other (Groborz & Necka, Citation2003; Ritter, Van Baaren, & Dijksterhuis, Citation2012; Zhu, Ritter, Müller, & Dijksterhuis, Citation2017). Thus, ideas could become more creative over time. Particularly, executive processes, especially those related to memory retrieval and knowledge manipulation could lead to the generation of increasingly remote concepts (Beaty & Silvia, Citation2012; Gilhooly, Fioratou, Anthony, & Wynn, Citation2007; Wang, Hao, Ku, Grabner, & Fink, Citation2017). Several electroencephalography (EEG) studies revealed that electroencephalographic activity was highly sensitive to certain creativity-related factor (Fink & Benedek, Citation2014; Hao et al., Citation2016; Kounios & Beeman, Citation2014; Rominger et al., Citation2018). These studies provided more elaborate descriptions on creative thinking using three isochronous time intervals for the idea generation period by EEG. Alpha power changes during idea generation, followed by a characteristic time course (Schwab, Benedek, Papousek, Weiss, & Fink, Citation2014). In the study by Schwab et al. (Citation2014), the idea generation process was subdivided into three time windows to observe the changes in upper-frequency alpha power. The overall increase in alpha power occurred at the beginning of the idea generation, then decreased, and finally increased again. The time course of brain activity might reflect a distinct stage of idea generation: initially retrieving conventional and obsolete ideas, then overcoming old ideas in psychological simulations and imaginations, and finally generating more novel creative ideas. Similarly, U-shaped pattern for EEG alpha power was observed on reasoning with a distinct final synchronization only in the occipital lobe (Jaarsveld et al., Citation2015). These results corresponded with evidence from functional magnetic resonance studies (Benedek, Schickel, Jauk, Fink, & Neubauer, Citation2014; Mayseless, Eran, & Shamay-Tsoory, Citation2015; Zhao, Li, Chen, Zhou, & Cheng, Citation2015). The studies of neural mechanisms underlying creativity based on BVSR theory showed that the BV stage was mainly accompanied by default mode network (DMN) activation, whereas the SR stage was accompanied by cognitive control network (CCN) activation. In addition, the coupling between the DMN and the salience network regions reflected an increase under the creative task initially and then indicated an increase between the DMN and CCN (Beaty, Benedek, Kaufman, & Silvia, Citation2015; Beaty, Benedek, Silvia, & Schacter, Citation2016; Beaty et al., Citation2018; Kleinmintz et al., Citation2018; Rominger et al., Citation2018). Therefore, examining the creativity process cognitive neuroscience has provided evidence regarding the potential creative cognition mechanism.
The previous researches concerning how stress affects creative thinking were inconsistent. In prior studies, the outcome of the effect of stress on creativity reached a three-category viewpoint: stress hampered creativity (Beversdorf, Hughes, Steinberg, Lewis, & Heilman, Citation1999; Lovelace & Hunter, Citation2013; Probst, Stewart, Gruys, & Tierney, Citation2007), increased creative performance (Baas, De Dreu, & Nijstad, Citation2008; Ohly & Fritz, Citation2009), and showed an inverted U-shaped function (Baer & Oldham, Citation2006; Suedfeld & Vernon, Citation1965; Yeh, Lai, Lin, Lin, & Sun, Citation2015). The controversial results of the previous studies on stress influencing creativity could be attributed to the following reasons. First, the measurements performed during stress induction mainly assess subjective perception (Baer & Oldham, Citation2006; Lovelace & Hunter, Citation2013; Ohly & Fritz, Citation2009). The subjective stress perception is only one of the indicators of successful stress induction. The evidence with respect to the change in biological indicators and brain activation, which measure the intensity and course of stress more precisely, was absent. Also, the change could modulate distinct cognitive systems, such as attention, memory, and creative thinking. Second, the previous researches focused only on the comparison of creativity outcomes and not on the creative process (Beversdorf et al., Citation1999; Suedfeld & Vernon, Citation1965; Yeh et al., Citation2015). Finally, the time course of creative thinking neural mechanism under acute stress remained largely unknown.
The initial stage accompanied by DMN activation was responsible for extraction and combination of information from memory and the later stage accompanied by CCN activation was involved in screening and monitoring of high levels of information (Beaty et al., Citation2015, Citation2018; Rominger et al., Citation2018). Under Montreal Imaging Stress Task (MIST) induced stress, individuals under stress recollected cognitive resources to cope with the stressor. Although the activity of the part of CCN was significantly enhanced, the DMN (e.g. the medial temporal prefrontal cortex) showed significant deactivation (Dedovic et al., Citation2009, Citation2014). The deactivation of the DMN was opposite to the requirement under creative cognitive task, which may make it hard for individuals to make freely remote association and get semantic map. Thus, we assumed that stress hampered the early stages in creative process.
Hence, this study aimed to depict and examine the neural mechanisms of creative thinking under acute stress from multiple perspectives using cognitive, behavioral, and EEG methods, as well as physiological and biochemical tests. We used the MIST (Dedovic et al., Citation2005), which is a standard method for inducing stress, and verified that stress was induced by psychophysiological measurements (cortisol and salivary alpha-amylase). The processes of creative thinking were compared in the participants before versus after they were subjected to stress.
Methods
Participants
Thirty-two healthy male students were recruited at Shaanxi Normal University. This study focused on male subjects to maximize the likelihood of obtaining significant effects because the previous research has shown that cognitive functions are less affected by stress in females (Shields, Trainor, Lam, & Yonelinas, Citation2016). Six participants were excluded owing to the absence of a stress effect. The final sample included 26 participants (age: mean = 18.42 years, standard deviation [SD] = 0.74). All participants were selected prior to the study based on their responses to a questionnaire regarding basic information.
The exclusion criteria were a body mass index below 18 kg/m2 or above 27 kg/m2, regular intake of medicine, use of hormonal contraceptives, drug use, coffee or alcohol use, chronic or acute illnesses, and current medical or psychological treatment. Furthermore, participants were advised to refrain from physical exercise and consumption of food and drinks except water within 4 h prior to the start of the test sessions. The exclusion criteria and instructions were defined based on the known factors influencing endogenous cortisol concentrations and were in line with those used in the previous studies, utilizing an acute laboratory stressor (Kudielka, Hellhammer, & Wüst, Citation2009; Kuhlmann, Kirschbaum, & Wolf, Citation2005). This study conformed to the principles of the Declaration of Helsinki (World Medical Association, Citation2013) and was approved by the Academic Committee of the Ministry of Education of Key Laboratory of Modern Teaching Technology, Shaanxi Normal University in China. All participants provided informed consent after the procedures were fully explained and they were compensated monetarily for their participation in the study.
Procedure
Previous studies found differences in individual creativity (Prabhakaran, Green, & Gray, Citation2014). In addition, the impact of stress on the creative process can be influenced by an individual’s personality (Bibbey, Carroll, Roseboom, Phillips, & de Rooij, Citation2013; Liu et al., Citation2013), age, and gender (Allen, Kennedy, Cryan, Dinan, & Clarke, Citation2014; Duan et al., Citation2013). Therefore, between-subject design could confound the experimental results, we used a within-subject before versus after test design (McHugh, Behar, Gutner, Geem, & Otto, Citation2010).
Saliva from the participants was collected at five time points (–40, –8, 0, 6, and 14 min in relation to the onset of the stressful task). The subjects completed the Positive Affect and Negative Affect Scale (PANAS, Watson, Clark, & Tellegen, Citation1988) three times (0, 6, and 14 min postrest). The procedure was summarized in .
Figure 1. Schematic illustration of the procedure. After a rest phase, participants performed AUT before and after the Montreal Imaging Stress Task (MIST). Saliva from the participants for further measurement was collected at five time points (–40, –8, 0, 6, and 14 min in relation to the onset of the stressful task) which was shown on the timeline.

Before and after stress induction, the participants performed the Alternative Uses Test (AUT) tasks. The AUT was administered using a computer and E-Prime 2.0 software (Psychology Software Tools, Inc., Sharpsburg, PA, USA). The order of these tasks was randomized across participants. Test sessions were conducted between 2:00 and 6:00 pm to control for individual differences in circadian rhythm (Izawa, Sugaya, Yamamoto, Ogawa, & Nomura, Citation2010).
Measures
Stress paradigm
During the experimental phase, the difficulty of time limit for the mental arithmetic task exceeded the individual' ability and the negative effects of the feedback increased subject' psychological stress. The screen displayed feedback based on the participant’s response (“correct,” “incorrect,” or “timeout”). There were two performance indicators: one for the individual subject’s performance and one for the average performance of all subjects. If the participant answered three continuous mental arithmetic tasks, then the time limit was reduced to 10% less than the average time of three correctly solved tasks. If the participant answered three continuous mental arithmetic tasks, then the time limit was increased by 10%. In addition, performance on the MIST was monitored by the main female tester (opposited sex to that of the test subjects), who would say “speed up,” “your speed lags behind that of the other students,” and “maintain correctness” during the stress procedure.
Three different types of difficult arithmetic problems were chosen randomly for 6 min. The mental arithmetic tasks used up to four numbers ranging from 0 to 99 and up to four operands (+ for addition, – for subtraction, * for multiplication, and/for division). The solution was an integer from 0 to 9 (selected by only a single keystroke). The difficulty levels of mental arithmetic were divided into three different categories: easiest, medium, and difficult. The easiest level only consisted of three one-digit integers, and the operands limited to + or – (e.g. 3 + 6 – 7). The medium level consisted of three integers (two of these integers in the two-digit range) and operand added * (e.g. 2 * 12 – 19). The difficult level had four integers, the * and / operands were created, and all numbers might be in the two-digit range (e.g.: 14* 16/4 – 52) (Dedovic et al., Citation2009).
Thirty subjects were selected to perform a speed test prior to the pilot experiment. The subjects’ reaction times in this test and the standard deviations were calculated. The formal experiments included another 20% reduction in reaction time to create a sense of urgency, as follows: simple (mean = 1688 ms, SD = 251 ms); medium (mean = 1816 ms, SD = 358 ms); and difficult (mean = 3177 ms, SD = 404 ms).
Divergent creativity
The participants performed the AUT during the EEG measurements. In the AUT, conventional everyday objects were presented on a computer. Participants were asked to think of original uses for these objects. The 30 items in the AUT were divided into two groups: pretest and post-test materials. To determine inter-rater agreement for five raters, tasks were assigned a difficulty level from 1 (“highly simple”) to 5 (“highly difficult”) with an intraclass correlation coefficient of 0.88. A paired-sample t-test revealed that there was no difference between two groups (t(28) = )−1.425, p = 0.17, d = 0.28; mean pre-AUT = 2.71, SD = 0.74; mean post-AUT = 3.05, SD = 0.53).
The participants underwent testing both before and after exposure to stress. Each of the tests consisted of 15 items. A fixation cross for 10 s (reference period) was first presented on the screen, and then, a stimulus word (common object) appeared for 4 s. Later, a white question mark appeared which indicated that the participants needed to think of useful and original ideas for the given stimulus for 10 s (idea generation period). After this period, the participants reported their ideas within 4 s by recording (cf., Fink, Benedek, Grabner, Staudt, & Neubauer, 2007) and evaluated their answers as “original” or “not original.” The AUT order was randomly presented. clearly showed the experimental procedure of an AUT trial. In , 砖 indicated the word “brick,” 作答 indicated the participant’s response, and 评价 indicated the self-rating of the response.
Figure 2. Procedure of AUT. Each trial began with the presentation of a fixation cross for 10 s (reference period). Then the stimulus word appeared for 4 s on the screen (e.g. brick). During the presentation of the white question mark, the participants were required to think of useful and original ideas for the stimulus for 10 s (activation period). Subsequently, the presentation of “作答” indicated that the participant was required to articulate his or her most original idea within 4 s. Finally, the participants were asked to evaluate their responses as either “original” or “not original” using a keyboard press on the corresponding button (figure adapted from Fink et al., Citation2011).
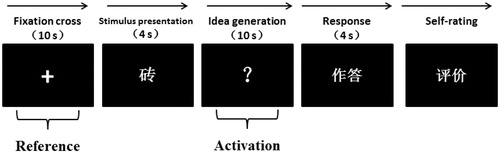
Biological measures
Saliva was collected using Salimetrics® tubes (Swab Storage Tube 5001.05, USA) at five time points: 40 and 8 min before the stress test, and 0, 6, and 8 min after stress induction. All saliva samples were stored at –22 °C and then thawed and centrifuged at 3000 rpm before the cortisol concentration in saliva was determined by enzyme immunoassay (Jianglai, China).
EEG data recording and analysis
The CURRY 7 system (Compumedics Neuroscan, TX, USA) with 64 Ag/AgCl electrodes was used to record scalp voltages. Amplified analog voltages were digitized at 500 Hz. Impedances of individual sensors were maintained below 5 kΩ. The EEG was performed online with reference to the left mastoid and a ground electrode on the frontal area. Eye movements were monitored using bipolar electrodes placed above and below the right eye and at the left and right canthi. The data were later analyzed offline with re-reference to an average of the left and right mastoids.
The EEG data were analyzed using SCAN 4.5 software (Compumedics Neuroscan) and the ERPLAB toolbox in MATLAB (Lopez-Calderon & Luck, Citation2014). The EEG data were digitally filtered using a 0.1–30 Hz bandpass. Eye movements were corrected using an ocular artifact correction algorithm (Gratton, Coles, & Donchin, Citation1983). Artifacts with amplitudes exceeding ±75 μV were removed from the analyses.
The artifacts caused by muscle tension, eye blinks, or eye movements were excluded from further analyses. In addition, the data included trials only with valid answers. After the visual inspection, the EEG signals were filtered for the upper alpha frequency band (10 to 12 Hz) using a fast Fourier transform filter. The band power values (mV2) were then (horizontally) averaged for each trial (Fink, Schwab, & Papousek, Citation2011; Fink et al., Citation2009). According to the method introduced by Schwab et al. (Citation2014), we used 8-s time interval (out of 10 s) in the middle of the reference periods (starting 1 s after the onset of the fixation cross) and the 8-s interval in the middle of the activation period (starting 1 s after the onset of the white question mark) to compute task-related power (TRP). The TRP for each electrode position (i) was computed using the following formula: TRP (logPowi) = log [Powi activation] – log [Powi reference] (Pfurtscheller, Citation1999). The log-transformed power during the reference period (fixation cross) was thus subtracted from the log-transformed power during the activation period (creative generation). Therefore, if the TRP was a positive value (alpha synchronization), it reflected an increase in power. If the TRP was a negative value (desynchronization), it reflected decrease in power. To examine the time course of creative idea generation, the 8-s idea generation intervals were further split into three isochronous 2.67-s time intervals.
Data were analyzed using univariate analyses of variance (ANOVAs). Cortisol and salivary alpha-amylase levels and AUT scores were the within-subject factors. Bonferroni corrections were used to control for multiple comparisons. Partial-eta2 (η2p) was reported as a measure of effect size.
Results
Biological and behavioral data
Negative affect
The ANOVA revealed a significant main negative effect, F (2, 50) = 6.25, p = 0.004, η2p = 0.200. Bonferroni-corrected simple-effects tests at each time point revealed a significant increase in negative effect from Time 1 to Time 2 (p = 0.01), and from Time 1 to Time 3 (p = 0.037) ().
Figure 3. Mean levels of negative effect (from the PANAS questionnaire) as a function of time in minutes following the onset of the stress or control intervention. The intervention began at Time 1 and lasted until Time 2. The experiment ended at Time 3. Results revealed a significant main negative effect (p = 0.004) and a significant increase in negative effect from Time 1 to Time 2 (p = 0.01), and from Time 1 to Time 3 (p = 0.037). Error bars represent SEM. *p < 0.05. **p < 0.01.
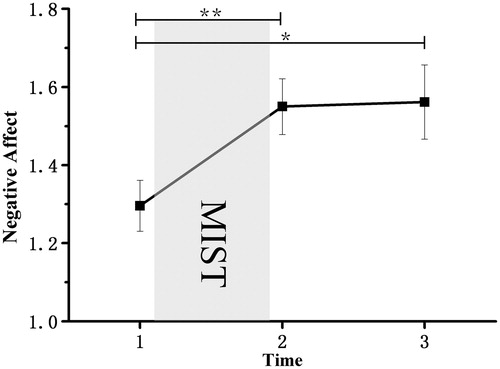
Biological data
The ANOVA of the data obtained from the saliva samples revealed that the levels of cortisol and salivary alpha-amylase increased in response to the MIST. There was a significant main effect of time on both biological indicators for salivary cortisol, F (3,75) = 39.77, p < 0.001, η2p = 0.613 and for salivary alpha-amylase, F (3,75) = 31.44, p < 0.001, η2p = 0.564. Furthermore, the cortisol levels at Time 1 were significantly lower than those at Time 2, Time 3, and Time 4 (p < 0.001 for all). There was also a significant difference in cortisol levels between Time 2 and Time 4 (p < 0.01). The cortisol levels at Time 3 did not show a difference with Time 4. We observed similar findings in the levels of salivary alpha-amylase ().
Figure 4. (A) Mean salivary cortisol (nmol/L) and (B) salivary alpha-amylase (U/ml) as a function of time (minutes following intervention onset) for the stress. The stress intervention began at Time 2 and lasted until Time 3. The experiment started and ended at Time 1 and Time 4. Results revealed a significant main effect of time on both biological indicators for salivary cortisol and salivary alpha-amylase (all p-values < 0.001). Furthermore, the cortisol levels at Time 1 were significantly lower than those at Time 2, Time 3, and Time 4 (p < 0.001 for all). There was also a significant difference in cortisol levels between Time 2 and Time 4 (p < 0.01). The cortisol levels at Time 3 did not show a difference with Time 4. The similar findings were observed in the levels of salivary alpha-amylase. Error bars represent SEM. **p < 0.01. ***p < 0.001
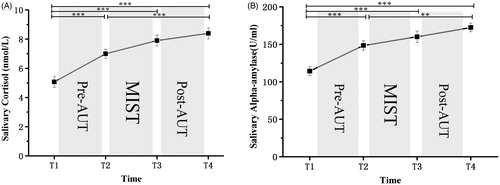
AUT scores
Originality in creative idea generation in the AUT was assessed using the consensual assessment technique (Amabile, Citation1982). The judgment of originality was performed by nine experienced raters on a five-point rating scale ranging from 1 (“highly original”) to 5 (“not original at all”). The intraclass correlation coefficient was 0.87. The pre-AUT scores (mean = 2.47, SD = 0.25) were significantly higher than the post-AUT scores (mean = 2.20, SD = 0.13), t (25) = 6.43, p < 0.001, d = 1.26) ().
EEG data
The TRP values were analyzed using repeated measures ANOVA with STRESS (before stress vs. after stress) TIME (interval 1 [1–3.67 s], interval 2 [3.67–6.33 s], and interval 3 [6.33–9 s]), HEMISPHERE (left vs. right), and POSITION (eight positions in each hemisphere) as within-subject variables. When sphericity assumptions were violated, we used a multivariate approach for the repeated measures variables used (Vasey & Thayer, Citation1987). Bonferroni corrections were used to control for multiple comparisons. Partial-eta2 (η2p) was reported as a measure of effect size.
The ANOVA revealed a significant main effect of STRESS, with larger TRP values observed before stress (mean = –0.007, SD = 0.012) than after stress (mean = –0.066, SD = 0.015), F (1,25) = 21.576, p < 0.001, η2p = 0.463, and a significant main effect of HEMISPHERE, with larger values observed in the right hemisphere (mean = –0.021, SD = 0.014) than left hemisphere (mean = –0.052, SD = 0.012), F (1,25) = 11.258, p = 0.003, η2p = 0.310 and a significant main effect on TIME, F (2,50) = 12.542, p < 0.001, η2p = 0.334. The TRP value in interval 1 (mean = –0.005, SD = 0.015) was higher than interval 2 (mean = –0.043, SD = 0.015) and interval 3(mean = –0.061, SD = 0.012). Furthermore, we found HEMISPHERE × TIME, F (2,50) = 5.612, p = 0.008, η2p = 0.183, HEMISPHERE × POSITION, F (7175) = 3.139, p = 0.015, η2p = 0.112 and STRESS × HEMISPHERE × TIME, F (2,50) = 3.694, p = 0.043, η2p = 0.129, interactions. Bonferroni-corrected simple-effects tests revealed that the TRP values in the right hemisphere were higher than those for the left hemisphere on the parietal and occipital lobes (position on C3C4, T7T8, P3P4, P7P8, and O1O2) (all p-values < 0.05). There were significant differences between before stress and after stress (all p-values < 0.05) except for interval 3 in the right hemisphere. Additionally, the hemisphere differences represented in interval 3 before stress and represented in intervals 2 and 3 after stress were significant (all p-values < 0.01) ().
Figure 6. Means and standard error bars for task-related alpha power changes (10–12 Hz) during creative idea generation ((A) represented before stress and (B) represented after stress) for eight cortical areas in the right and left hemispheres (abbreviations for cortical sites are used according to the international 10-20 system [Jasper, 1958]: FP = frontopolar, F = frontal, C = central, P = parietal, O = occipital, T = temporal; odd numbers represent cortical sites in the left hemisphere, whereas even numbers correspond to cortical sites in the right hemisphere). The ANOVA revealed a significant main effect of STRESS, with larger TRP values observed before stress than after stress (p < 0.001) and a significant main effect of HEMISPHERE, with larger values observed in the right hemisphere than left hemisphere (p = 0.003) and a significant main effect on TIME (p < 0.001). Furthermore, TRP values in interval 1 were higher than interval 2 and interval 3 (all p-values < 0.001). In addition, we found HEMISPHERE × TIME (p = 0.008), HEMISPHERE × POSITION (p = 0.015) and STRESS × HEMISPHERE × TIME (p = 0.043) interactions. Bonferroni-corrected simple-effects tests revealed that the TRP values in the right hemisphere were higher than those for the left hemisphere on the parietal and occipital lobes (position on C3C4, T7T8, P3P4, P7P8, and O1O2) (all p-values < 0.05). There were significant differences between before stress and after stress (all p-values < 0.05) except for interval 3 in the right hemisphere. Additionally, the hemisphere differences represented in interval 3 before stress and represented in intervals 2 and 3 after stress (all p-values < 0.01).
![Figure 6. Means and standard error bars for task-related alpha power changes (10–12 Hz) during creative idea generation ((A) represented before stress and (B) represented after stress) for eight cortical areas in the right and left hemispheres (abbreviations for cortical sites are used according to the international 10-20 system [Jasper, 1958]: FP = frontopolar, F = frontal, C = central, P = parietal, O = occipital, T = temporal; odd numbers represent cortical sites in the left hemisphere, whereas even numbers correspond to cortical sites in the right hemisphere). The ANOVA revealed a significant main effect of STRESS, with larger TRP values observed before stress than after stress (p < 0.001) and a significant main effect of HEMISPHERE, with larger values observed in the right hemisphere than left hemisphere (p = 0.003) and a significant main effect on TIME (p < 0.001). Furthermore, TRP values in interval 1 were higher than interval 2 and interval 3 (all p-values < 0.001). In addition, we found HEMISPHERE × TIME (p = 0.008), HEMISPHERE × POSITION (p = 0.015) and STRESS × HEMISPHERE × TIME (p = 0.043) interactions. Bonferroni-corrected simple-effects tests revealed that the TRP values in the right hemisphere were higher than those for the left hemisphere on the parietal and occipital lobes (position on C3C4, T7T8, P3P4, P7P8, and O1O2) (all p-values < 0.05). There were significant differences between before stress and after stress (all p-values < 0.05) except for interval 3 in the right hemisphere. Additionally, the hemisphere differences represented in interval 3 before stress and represented in intervals 2 and 3 after stress (all p-values < 0.01).](/cms/asset/617efa86-c41a-4e8a-a53f-26a366e539da/ists_a_1604665_f0006_c.jpg)
Originality as assessed using EEG
Before stress
For each of the 26 participants, we divided all of the ideas generated in the AUT before the application of stress into trials in which more versus less original ideas were generated using a median split strategy (median = 2.50). This classification was based on the external ratings. This distinction was used as a within-subject factor in further analysis. A paired-samples t-test, (t (12) = –7.04, p < 0.001, d = 2.09; mean for less original ideas = 2.67, SD = 0.15; mean for more original ideas = 2.27, SD = 0.14) revealed that the average score for the more original ideas was significantly lower (denoting higher originality) than the average score for the less original ideas.
The TRP values were analyzed using repeated measures ANOVA with TIME (interval 1 [1–3.67 s], interval 2 [3.67–6.33 s], and interval 3 [6.33–9 s]), and HEMISPHERE (left vs. right) as within-subject variables and ORIGINALITY (more vs. less original ideas) as between-subject variables. We observed main effects of ORIGINALITY, F (1,24) = 6.489, p = .018 η2p = 0.213; TIME, F (2,48) = 11.254, p < 0.001, η2p = 0.319, and HEMISPHERE, F (1,24) = 4.967, p = 0.035, η2p = 0.171. The stronger increase in TRP on the right hemisphere was more pronounced in trials with more (vs. less) original ideas. In addition, the participants with more original ideas had higher TRP values than those with less original ideas in every interval. Furthermore, we found HEMISPHERE × TIME had a significant difference (F (2,48) = 5.820, p = 0.01, η2p = 0.195). Bonferroni-corrected simple-effects tests revealed a significant difference between interval 1 (mean = 0.028, SD = 0.017), interval 2 (mean = –0.027, SD = 0.016), and interval 3 (mean = –0.056, SD = 0.011) in the left hemisphere, p < 0.05. Also, there was a significant difference between interval 1 (mean = 0.035, SD = 0.017) and interval 2 (mean = –0.014, SD = 0.016) in the right hemisphere (p = .005) ().
Figure 7. Means and standard error bars of task-related alpha power changes (10–12 Hz) during creative idea generation for more versus less original ideas before stress (A) and after stress (B). The results before stress (A) revealed a main effect of ORIGINALITY (p = .018), TIME (p < 0.001) and HEMISPHERE (p = 0.035). The stronger increase in TRP in the right hemisphere was more pronounced in trials with more (vs. less) original ideas. In addition, the participants with more original ideas had higher TRP values than those with less original ideas in every interval. There was also a significant interaction effect on HEMISPHERE × TIME (p = 0.01). Furthermore, there was a significant difference between interval 1, interval 2, and interval 3 in the left hemisphere (all p-values < 0.05) and a significant difference between interval 1 and interval 2 in the right hemisphere (p = 0.005). The results after stress (B) revealed no main effect of idea originality (p = 0.197). However, there was a main effect of HEMISPHERE (p = 0.004) and TIME (p = 0.048). Post-hoc results showed that changes in the right hemisphere were more pronounced than left hemisphere, whereas there was no difference in intervals 1, 2, or 3.
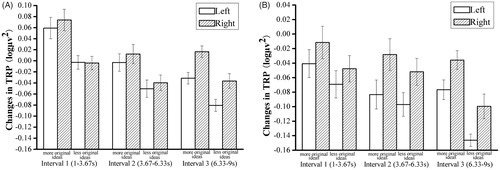
After stress
The TRP values were analyzed as they were for the before-stress test. We found no main effect of idea originality (F (1,24) = 1.760, p = 0.197, η2p = 0.068). However, there was a main effect of HEMISPHERE, F (1,24) = 10.471, p = 0.004, η2p = 0.304, and TIME, F (2,48) = 3.741, p = 0.048, η2p = 0.135. Post-hoc results showed that changes in the right hemisphere (mean = –0.046, SD = 0.016) were more pronounced than those in the left hemisphere (mean = –0.085, SD = 0.016), whereas there was no difference in intervals 1, 2, or 3 ().
Discussion
Our findings revealed that acute stress impaired creative thinking. Our current findings were consistent with the viewpoint that stress decreases creativity (Beversdorf et al., Citation1999; Lovelace & Hunter, Citation2013; Probst et al., Citation2007). The EEG findings suggested that acute stress has a broad impact on the process of creative ideation. The change in task-related EEG alpha power over time showed a characteristic pattern. The creative activity EEG without stress showed a general increase in alpha power initially, followed by a decrease in alpha power. The participants showed alpha synchronization only during the first test before the application of stress, whereas there was no alpha synchronization at any time point after the induction of stress. The upper-frequency alpha power activity in creativity indicated an increase in internal attentional demand, a focus on defocusing, to ensure control of access and retrieval from memory (Klimesch, Citation2012) without internal interference (Benedek, 2018; Rominger et al., Citation2018). More specifically, the increased alpha power activity in the frontal lobe reflected a reduction in executive control (Martindale, Citation1999). The increase in alpha power activity in the right brain reflected more divergent semantically encoded information that activated a broader semantic map (Fink & Benedek, Citation2014; Kounios & Beeman, Citation2014).
According to the time course of alpha band activity, we concluded that the initial phase of creative idea generation was typically characterized by the retrieval of dominant responses from memory (Beaty et al., Citation2015; Benedek et al., Citation2014; Gilhooly et al., Citation2007; Mayseless et al., Citation2015). Therefore, it seemed that the bilateral alpha synchronization at the beginning of creative idea generation indicated the typical first phase of the creative generation before stress, such as recalling common thoughts from memory (Benedek et al., Citation2014; Schwab et al., Citation2014). Subsequently, there was a decrease in alpha power compared with the last stage. In addition, this phase was characterized by the onset of hemispheric lateralization, which further increased in the last stage. It was reported that alpha synchronization of the right posterior cortex was specific to creative thinking (Benedek et al., Citation2014; Fink et al., Citation2007) and was interpreted as a marker for centralizing internal attention for fueling the imagination during creative idea generation (Fink & Benedek, Citation2014). When the individuals experienced stressful situations, the entire creative process was desynchronized, although in the second stage, there was a tendency to restore synchronization. In the last stage, the whole brain still showed less desynchronization. Combined with the previous studies discussed above, the finding indicated that individuals experienced stress were in a bottom-up stimulus-driven state accompanied by CCN activation during the initial stages of creativity and unable to effectively retrieve memory resources by inhibiting irrelevant information. However, in the second stage, individuals tried to find an effective semantic connection focused on a trend of synchronization. It may be because of the deactivation of the DMN causing unsuccessful retrieval of information from memory when subjected to a stressful situation (Dedovic et al., Citation2009, Citation2014).
In contrast to the findings of other reports (Schwab et al., Citation2014), there was no lateralization tendency, except in the right parietal-frontal (F7, F8) and parietal-cortical (C3, C4) synchronization. This might have been owing to the fast-paced trial or that the event-related potential device made the participant too nervous to complete the experiment normally and freely. Almost the entire creative course was affected by stress. There were hemisphere differences represented in interval 3 before stress and represented in intervals 2 and 3 after stress. However, these effects were observed as a state of desynchronization. It is expected that an organism would devote attentional resources to focus on a current threatening stimulus, so that it would lead to an inhibitory state of the cortex. Sustained stress resulted in prefrontal desynchronization and lateralization of parieto-occipital signals in interval 3 of the AUT (Bogdanov & Schwabe, Citation2016; Dedovic et al., Citation2009, Citation2014; Lee et al., Citation2014; Smeets et al., Citation2006). Such circumstances impaired creative thinking, as divergent thinking required a widespread semantic network.
Thus, acute stress impaired creative thinking and primarily affected the earlier phase of the creative cognition process. Stress induced a negative effect, which would in turn attract the individual to focus on the current threat, and then lead to a narrow attention scope. Individual thinking became rigid when there was competition between emotional and cognitive stimuli for cognitive resources and eventually leads to decreased creativity (Huntsinger, Citation2013).
Specifically, the findings indicated that ideas with high originality could be more easily affected than less original ideas. Stronger alpha synchronization at right hemispheric sites were observed in individuals or in association with ideas with higher (vs. lower) originality before the application of stress (Fink et al., Citation2009; Kounios & Beeman, Citation2014). Here, the results showed that individuals with higher originality develop lateralization earlier than those with less originality. However, after the application of stress, there was no significant difference between higher originality and lower originality. Notably, individuals with higher originality couldnot take full advantage of their creativity in the same manner during the creative process before stress. In other words, stress reduced the differences between individuals with different creative abilities. Highly creative individuals might not be able to complete creative tasks as usual under stressful conditions, whereas less creative individuals also had impaired creativity, but the effects of stress were smaller among these individuals when compared to the more creative subjects. Also, highly creative individuals might be better able to display creative behavior in more relaxed environments, whereas less creative individuals would not have similar benefits.
Our findings suggested that acute stress impaired creative thinking performance and mainly affected the earlier phase of the process of creative cognition. This study provided some practical implications for educational practice and corporate innovation. For educational practice, a more relaxed classroom atmosphere was more likely to generate creative ideas; for corporate innovation, a relaxed work environment can stimulate employees’ creative product output. Therefore, timely stress management systems could reduce the negative effects of stress to a large extent.
Future directions
There was a lack of direct evidence regarding the cognitive mechanism underlying creativity under stress. Future studies should be performed to either directly measure specific cognitive ability (such as inhibition) or modify the inhibitory capacity of transcranial direct current stimulation (Nozari, Woodard, & Thompson-Schill, Citation2014; Zmigrod, Zmigrod, & Hommel, Citation2016). Such studies would enable us to learn more about the pathway involved in our observations. Besides, we found individual differences in creativity under stress in our study. Future research should also be specifically performed in individuals with more and less creativity. These studies could enable the assessment of brain functional connectivity as a predictor of individual creative ability under acute stress (Beaty et al., Citation2018).
Disclosure statement
No potential conflict of interest was reported by the authors.
Additional information
Funding
References
- Allen, A.P., Kennedy, P.J., Cryan, J.F., Dinan, T.G., & Clarke, G. (2014). Biological and psychological markers of stress in humans: focus on the trier social stress test. Neuroscience and Biobehavioral Reviews, 38, 94–124. doi:10.1016/j.neubiorev.2013.11.005
- Amabile, T.M. (1982). Children’s artistic creativity: Detrimental effects of competition in a field setting. Personality and Social Psychology Bulletin, 8, 573–578. doi:10.1177/0146167282083027
- Baas, M., De Dreu, C.K., & Nijstad, B.A. (2008). A meta-analysis of 25 years of mood-creativity research: Hedonic tone, activation, or regulatory focus? Psychological Bulletin, 134, 779. doi:10.1037/a0012815
- Baer, M., & Oldham, G.R. (2006). The curvilinear relation between experienced creative time pressure and creativity: Moderating effects of openness to experience and support for creativity. Journal of Applied Psychology, 91, 963. doi:10.1037/0021-9010.91.4.963
- Basadur, M., Graen, G.B., & Green, S.G. (1982). Training in creative problem solving: Effects on ideation and problem finding and solving in an industrial research organization. Organizational Behavior and Human Performance, 30, 41–70. doi:10.1016/0030-5073(82)90233-1
- Beaty, R.E., Benedek, M., Kaufman, S.B., & Silvia, P.J. (2015). Default and executive network coupling supports creative idea production. Scientific Reports, 5, 10964. doi:10.1038/srep10964
- Beaty, R.E., Benedek, M., Silvia, P.J., & Schacter, D.L. (2016). Creative cognition and brain network dynamics. Trends in Cognitive Sciences, 20, 87–95. doi:10.1016/j.tics.2015.10.004
- Beaty, R.E., Kenett, Y.N., Christensen, A.P., Rosenberg, M.D., Benedek, M., Chen, Q., … Silvia, P.J. (2018). Robust prediction of individual creative ability from brain functional connectivity. Proceedings of the National Academy of Sciences USA, 115, 1087–1092. doi:10.1073/pnas.1713532115
- Beaty, R.E., & Silvia, P.J. (2012). Why do ideas get more creative across time? An executive interpretation of the serial order effect in divergent thinking tasks. Psychology of Aesthetics, Creativity, and the Arts, 6, 309. doi:10.1037/a0029171
- Benedek, M. (2018). Internally directed attention in creative cognition. In R. E. Jung, O. Vartanian (Eds.), The Cambridge Handbook of the Neuroscience of Creativity. (pp. 180–194). New York: Cambridge University Press. doi: 10.1017/9781316556238.011
- Benedek, M., Jauk, E., Fink, A., Koschutnig, K., Reishofer, G., Ebner, F., & Neubauer, A.C. (2014). To create or to recall? Neural mechanisms underlying the generation of creative new ideas. NeuroImage, 88, 125–133. doi:10.1016/j.neuroimage.2013.11.021
- Benedek, M., Schickel, R.J., Jauk, E., Fink, A., & Neubauer, A.C. (2014). Alpha power increases in right parietal cortex reflects focused internal attention. Neuropsychologia, 56, 393–400. doi:10.1016/j.neuropsychologia.2014.02.010
- Beversdorf, D.Q., Hughes, J.D., Steinberg, B.A., Lewis, L.D., & Heilman, K.M. (1999). Noradrenergic modulation of cognitive flexibility in problem solving. NeuroReport, 10, 2763–2767. doi:10.1097/00001756-199909090-00012
- Bibbey, A., Carroll, D., Roseboom, T.J., Phillips, A.C., & de Rooij, S.R. (2013). Personality and physiological reactions to acute psychological stress. International Journal of Psychophysiology, 90, 28–36. doi:10.1016/j.ijpsycho.2012.10.018
- Bogdanov, M., & Schwabe, L. (2016). Transcranial stimulation of the dorsolateral prefrontal cortex prevents stress-induced working memory deficits. The Journal of Neuroscience, 36, 1429–1437. doi:10.1523/JNEUROSCI.3687-15.2016
- Byron, K., Khazanchi, S., & Nazarian, D. (2010). The relationship between stressors and creativity: A meta-analysis examining competing theoretical models. Journal of Applied Psychology, 95, 201. doi:10.1037/a0017868
- Campbell, D.T. (1960). Blind variation and selective retentions in creative thought as in other knowledge processes. Psychological Review, 67, 380. doi:10.1037/h0040373
- Christensen, P.R., Guilford, J.P., & Wilson, R.C. (1957). Relations of creative responses to working time and instructions. Journal of Experimental Psychology, 53, 82–88. doi:10.1037/h0045461
- Csikszentmihalyi, M. (1996). Flow and the psychology of discovery and invention. New York: Harper Collins.
- Dedovic, K., Duchesne, A., Engert, V., Lue, S.D., Andrews, J., Efanov, S.I., … Pruessner, J.C. (2014). Psychological, endocrine and neural responses to social evaluation in subclinical depression. Social Cognitive and Affective Neuroscience, 9, 1632–1644. doi:10.1093/scan/nst151
- Dedovic, K., Renwick, R., Mahani, N.K., Engert, V., Lupien, S.J., & Pruessner, J.C. (2005). The Montreal Imaging Stress Task: Using functional imaging to investigate the effects of perceiving and processing psychosocial stress in the human brain. Journal of Psychiatry and Neuroscience, 30, 319–326.
- Dedovic, K., Rexroth, M., Wolff, E., Duchesne, A., Scherling, C., Beaudry, T., … Pruessner, J.C. (2009). Neural correlates of processing stressful information: An event-related fMRI study. Brain Research, 1293, 49–60. doi:10.1016/j.brainres.2009.06.044
- Duan, H., Yuan, Y., Zhang, L., Qin, S., Zhang, K., Buchanan, T.W., & Wu, J. (2013). Chronic stress exposure decreases the cortisol awakening response in healthy young men. Stress, 16, 630–637. doi:10.3109/10253890.2013.840579
- Fink, A., & Benedek, M. (2014). EEG alpha power and creative ideation. Neuroscience & Biobehavioral Reviews, 44, 111–123. doi:10.1016/j.neubiorev.2012.12.002
- Fink, A., Benedek, M., Grabner, R.H., Staudt, B., & Neubauer, A.C. (2007). Creativity meets neuroscience: Experimental tasks for the neuroscientific study of creative thinking. Methods, 42, 68–76. doi:10.1016/j.ymeth.2006.12.001
- Fink, A., Grabner, R.H., Benedek, M., Reishofer, G., Hauswirth, V., Fally, M., … Neubauer, A.C. (2009). The creative brain: Investigation of brain activity during creative problem solving by means of EEG and fMRI. Human Brain Mapping, 30, 734–748. doi:10.1002/hbm.20538
- Fink, A., Schwab, D., & Papousek, I. (2011). Sensitivity of EEG upper alpha activity to cognitive and affective creativity interventions. International Journal of Psychophysiology, 82, 233–239. doi:10.1016/j.ijpsycho.2011.09.003
- Finke, R.A., Ward, T.M., & Smith, S.M. (1992). Creative cognition: Theory, research and applications. Cambridge, MA: MIT Press.
- Foley, P., & Kirschbaum, C. (2010). Human hypothalamus-pituitary-adrenal axis responses to acute psychosocial stress in laboratory settings. Neuroscience and Biobehavioral Reviews, 35, 91–96. doi:10.1016/j.neubiorev.2010.01.010
- Gilhooly, K.J., Fioratou, E., Anthony, S.H., & Wynn, V. (2007). Divergent thinking: Strategies and executive involvement in generating novel uses for familiar objects. British Journal of Psychology, 98, 611–625. doi:10.1111/j.2044-8295.2007.tb00467.x
- Gratton, G., Coles, M.G., & Donchin, E. (1983). A new method for off-line removal of ocular artifact. Electroencephalography and Clinical Neurophysiology, 55, 468–484. doi:10.1016/0013-4694(83)90135-9
- Groborz, M., & Necka, E. (2003). Creativity and cognitive control: Explorations of generation and evaluation skills. Creativity Research Journal, 15, 183–197. doi:10.1207/S15326934CRJ152&3_09
- Guilford, J.P. (1987). Creativity research: Past, present and future. In S.G. Isaksen (Ed.), Frontiers of creativity research: Beyond the basics (pp. 33–65). Buffalo: Bearly Limited.
- Günzel, F.M. (2014). The impact of stress on stimulus response learning (Unpublished doctoral dissertation). Ruhr University Bochum, Germany.
- Hao, N., Ku, Y., Liu, M., Hu, Y., Bodner, M., Grabner, R.H., & Fink, A. (2016). Reflection enhances creativity: Beneficial effects of idea evaluation on idea generation. Brain and Cognition, 103, 30–37. doi:10.1016/j.bandc.2016.01.005
- Huntsinger, J.R. (2013). Does emotion directly tune the scope of attention? Current Directions in Psychological Science, 22, 265–270. doi:10.1177/0963721413480364
- Izawa, S., Sugaya, N., Yamamoto, R., Ogawa, N., & Nomura, S. (2010). The cortisol awakening response and autonomic nervous system activity during nocturnal and early morning periods. Neuroendocrinology Letters, 31, 685.
- Jaarsveld, S., Fink, A., Rinner, M., Schwab, D., Benedek, M., & Lachmann, T. (2015). Intelligence in creative processes: An EEG study. Intelligence, 49, 171–178. doi:10.1016/j.intell.2015.01.012
- Kleinmintz, O.M., Abecasis, D., Tauber, A., Geva, A., Chistyakov, A.V., Kreinin, I., … Shamay-Tsoory, S.G. (2018). Participation of the left inferior frontal gyrus in human originality. Brain Structure and Function, 223, 329–341. doi:10.1007/s00429-017-1500-5
- Kirschbaum, C., & Hellhammer, D.H. (1989). Salivary cortisol in psychobiological research: An overview. Neuropsychobiology, 22, 150–169. doi:10.1159/000118611
- Klimesch, W. (2012). Alpha-band oscillations, attention, and controlled access to stored information. Trends in Cognitive Sciences, 16, 606–617. doi:10.1016/j.tics.2012.10.007
- Kounios, J., & Beeman, M. (2014). The cognitive neuroscience of insight. Annual Review of Psychology, 65, 71–93. doi:10.1146/annurev-psych-010213-115154
- Kudielka, B.M., Hellhammer, D.H., & Wüst, S. (2009). Why do we respond so differently? Reviewing determinants of human salivary cortisol responses to challenge. Psychoneuroendocrinology, 34, 2–18. doi:10.1016/j.psyneuen.2008.10.004
- Kuhlmann, S., Kirschbaum, C., & Wolf, O.T. (2005). Effects of oral cortisol treatment in healthy young women on memory retrieval of negative and neutral words. Neurobiology of Learning and Memory, 83, 158–162. doi:10.1016/j.nlm.2004.09.001
- Lee, M.R., Cacic, K., Demers, C.H., Haroon, M., Heishman, S., Hommer, D.W., … Salmeron, B.J. (2014). Gender differences in neural-behavioral response to self-observation during a novel fMRI social stress task. Neuropsychologia, 53, 257–263. doi:10.1016/j.neuropsychologia.2013.11.022
- Liu, Q., Zhou, R., Oei, T.P., Wang, Q., Zhao, Y., & Liu, Y. (2013). Variation in the stress response between high-and low-neuroticism female undergraduates across the menstrual cycle. Stress, 16, 503–509. doi:10.3109/10253890.2013.797958
- Lopez-Calderon, J., & Luck, S.J. (2014). ERPLAB: An open-source toolbox for the analysis of event-related potentials. Frontiers in Human Neuroscience, 8, 213.
- Lovelace, J.B., & Hunter, S.T. (2013). Charismatic, ideological, and pragmatic leaders’ influence on subordinate creative performance across the creative process. Creativity Research Journal, 25, 59–74. doi:10.1080/10400419.2013.752228
- Martindale, C. (1999). Biological bases of creativity. In R. Sternberg (Ed.), Handbook of creativity (pp. 137–152). Cambridge: Cambridge University Press.
- Mayseless, N., Eran, A., & Shamay-Tsoory, S.G. (2015). Generating original ideas: The neural underpinning of originality. NeuroImage, 116, 232–239. doi:10.1016/j.neuroimage.2015.05.030
- McEwen, B.S. (2000). The neurobiology of stress: From serendipity to clinical relevance. Brain Research, 886, 172–189. doi:10.1016/S0006-8993(00)02950-4
- McHugh, R.K., Behar, E., Gutner, C.A., Geem, D., & Otto, M.W. (2010). Cortisol, stress, and attentional bias toward threat. Anxiety, Stress, and Coping, 23, 529–545. doi:10.1080/10615801003596969
- Nater, U.M., & Rohleder, N. (2009). Salivary alpha-amylase as a non-invasive biomarker for the sympathetic nervous system: Current state of research. Psychoneuroendocrinology, 34, 486–496. doi:10.1016/j.psyneuen.2009.01.014
- Nozari, N., Woodard, K., & Thompson-Schill, S.L. (2014). Consequences of cathodal stimulation for behavior: When does it help and when does it hurt performance? PLoS One, 9, e84338. doi:10.1371/journal.pone.0084338
- Ohly, S., & Fritz, C. (2009). Work characteristics, challenge appraisal, creativity, and proactive behavior: A multi‐level study. Journal of Organizational Behavior, 31, 543–565. doi:10.1002/job.633
- Pfurtscheller, G. (1999). Quantifikation of ERD and ERS in the time domain. In G. Pfurtscheller and F. H. Lopes da Silva (Ed.), Event-Related Desynchronization. Handbook of Elektroencephalic and Clinical Neurophysiology (pp. 89–105). Amsterdam: Elsevier.
- Prabhakaran, R., Green, A.E., & Gray, J.R. (2014). Thin slices of creativity: Using single-word utterances to assess creative cognition. Behavior Research Methods, 46, 641–659. doi:10.3758/s13428-013-0401-7
- Probst, T.M., Stewart, S.M., Gruys, M.L., & Tierney, B.W. (2007). Productivity, counterproductivity and creativity: The ups and downs of job insecurity. Journal of Occupational and Organizational Psychology, 80, 479–497. doi:10.1348/096317906X159103
- Rominger, C., Papousek, I., Perchtold, C.M., Weber, B., Weiss, E.M., & Fink, A. (2018). The creative brain in the figural domain: Distinct patterns of EEG alpha power during idea generation and idea elaboration. Neuropsychologia, 118, 13–19. doi:10.1016/j.neuropsychologia.2018.02.013
- Ritter, S.M., Van Baaren, R.B., & Dijksterhuis, A. (2012). Creativity: The role of unconscious processes in idea generation and idea selection. Thinking Skills and Creativity, 7, 21–27. doi:10.1016/j.tsc.2011.12.002
- Schwab, D., Benedek, M., Papousek, I., Weiss, E.M., & Fink, A. (2014). The time-course of EEG alpha power changes in creative ideation. Frontiers in Human Neuroscience, 8, 310.
- Shields, G.S., Trainor, B.C., Lam, J.C., & Yonelinas, A.P. (2016). Acute stress impairs cognitive flexibility in men, not women. Stress, 19, 542–546. doi:10.1080/10253890.2016.1192603
- Simonton, D.K. (2011). Creativity and discovery as blind variation: Campbell’s (1960) BVSR model after the half-century mark. Review of General Psychology, 15, 158. doi:10.1037/a0022912
- Simonton, D.K. (2013). Creative problem solving as sequential BVSR: Exploration (total ignorance) versus elimination (informed guess). Thinking Skills and Creativity, 8, 1–10. doi:10.1016/j.tsc.2012.12.001
- Smeets, T., Jelicic, M., Merckelbach, H., Peters, M., Fett, A., Taverniers, J., … Dautzenberg, J. (2006). Enhanced memory performance on an internal-internal source monitoring test following acute psychosocial stress. Behavioral Neuroscience, 120, 1204–1210. doi:10.1037/0735-7044.120.6.1204
- Sternberg, R.J., & Lubart, T.I. (1996). Investing in creativity. American Psychologist, 51, 677. doi:10.1037//0003-066X.51.7.677
- Suedfeld, P., & Vernon, J. (1965). Stress and verbal originality in sensory deprivation. The Psychological Record, 15, 567–570. doi:10.1007/BF03393631
- Thoma, M.V., Kirschbaum, C., Wolf, J.M., & Rohleder, N. (2012). Acute stress responses in salivary alpha-amylase predict increases of plasma norepinephrine. Biological Psychology, 91, 342–348. doi:10.1016/j.biopsycho.2012.07.008
- Vasey, M.W., & Thayer, J.F. (1987). The continuing problem of false positives in repeated measures ANOVA in psychophysiology: A multivariate solution. Psychophysiology, 24, 479–486. doi:10.1111/j.1469-8986.1987.tb00324.x
- Wang, M., Hao, N., Ku, Y., Grabner, R.H., & Fink, A. (2017). Neural correlates of serial order effect in verbal divergent thinking. Neuropsychologia, 99, 92–100. doi:10.1016/j.neuropsychologia.2017.03.001
- Watson, D., Clark, L.A., & Tellegen, A. (1988). Development and validation of brief measures of positive and negative affect: The PANAS scales. Journal of Personality and Social Psychology, 54, 1063. doi:10.1037//0022-3514.54.6.1063
- World Medical Association (WMA). (2013). World Medical Association Declaration of Helsinki. Ethical Principles for Medical Research Involving Human Subjects (www.wma.net/en/30publications/10policies/b3.htm.).
- Yeh, Y.C., Lai, G.J., Lin, C.F., Lin, C.W., & Sun, H.C. (2015). How stress influences creativity in game-based situations: Analysis of stress hormones, negative emotions, and working memory. Computers & Education, 81, 143–153. doi:10.1016/j.compedu.2014.09.011
- Zhao, Q., Li, S., Chen, S., Zhou, Z., & Cheng, L. (2015). Dynamic neural processing mode of creative problem solving. Advances in Psychological Science, 23, 375–384. doi:10.3724/SP.J.1042.2015.00375
- Zhu, Y., Ritter, S.M., Müller, B.C., & Dijksterhuis, A. (2017). Creativity: Intuitive processing outperforms deliberative processing in creative idea selection. Journal of Experimental Social Psychology, 73, 180–188. doi:10.1016/j.jesp.2017.06.009
- Zmigrod, S., Zmigrod, L., & Hommel, B. (2016). Transcranial direct current stimulation (tDCS) over the right dorsolateral prefrontal cortex affects stimulus conflict but not response conflict. Neuroscience, 322, 320–325. doi:10.1016/j.neuroscience.2016.02.046