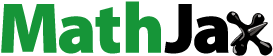
Abstract
Olfactory cues can affect subjective and autonomic manifestations of the human stress response, but evidence of altered endocrine stress reactivity is inconclusive. In the present study, we investigated effects of the odorant Hedione on the human stress response. We exposed 56 women in their follicular phases to a stressor in a room scented with Hedione or no odor. Subjective stress was captured via repeated self-report measurements and the assessment of anticipatory appraisal. As physiological markers of stress, we assessed blood pressure, salivary cortisol and alpha-amylase. The odorant enhanced the cortisol and cardiovascular stress response while leaving subjective stress unaffected. Our results provide evidence for a modulation of the human response to acute psychosocial stress by Hedione. A potential mechanism underlying this effect is Hedione targeting the hypothalamus via binding to the VN1R1 receptor, which is expressed on the human nasal mucosa.
1. Introduction
Depending on their pleasantness and experiences associated with them, odors can affect emotional state positively or negatively, and even exert physiological effects on the stress-responsive autonomic nervous system (ANS; Herz, Citation2009). Another system activated in response to stress is the hypothalamus pituitary adrenal (HPA) axis (Selye, Citation1950; Ulrich-Lai & Herman, Citation2009). Evidence regarding the sensitivity of the human HPA axis to olfactory modulation is inconsistent: A meta-analysis of randomized controlled trials using aromatherapy for stress management (Hur et al., Citation2014) demonstrated positive effects on subjective stress, but not on salivary and serum cortisol, being the end-products of the HPA axis.
Another approach to olfactory modulation of the human stress response is chemosensory communication – the transfer of information between individuals via chemosensory cues (Lübke & Pause, Citation2015). Meta-analytic evidence supports chemosensory communication of stress and threat among humans (de Groot & Smeets, Citation2017). However, evidence of altered endocrine response patterns is inconclusive, and was only investigated for androstadienone, a component of male sweat (e.g. Bensafi, Citation2004).
Speaking of chemosensory communication, the synthetic odorant Hedione requires closer examination. It was discussed as a potential modulator of hormonal release via binding to the VN1R1 receptor (Wallrabenstein et al., Citation2015). This receptor is structurally homologous to a pheromone receptor expressed in the vomeronasal organ (VNO) of most mammals. Despite the lack of a functional VNO, the human olfactory system comprises five vomeronasal-type 1 receptors on the nasal mucosa (Wallrabenstein et al., Citation2015). Their exact function is still subject to investigation. In an fMRI study, Hedione elicited stronger limbic (amygdala, hippocampus) and hypothalamic activation (Wallrabenstein et al., Citation2015) than a common odor (phenylethyl alcohol). Further, two studies indicate that Hedione enhances reciprocity (Berger et al., Citation2017), and reduces subjective vicarious stress (Pützer et al., Citation2019).
In the present study, we investigated the impact of Hedione on the human stress response. Due to its binding to the VN1R1 receptor and the resulting brain activation pattern, we expected Hedione to enhance the autonomic and endocrine stress response. In contrast, we expected it to reduce rather than enhance subjective stress, which would be in line with our previous findings showing that Hedione reduced subjective stress when observing another person in a stressful episode (Pützer et al., Citation2019). To test this, 56 women in their follicular phases were assigned to a group with Hedione or no odor dispersed in the testing room. We induced psychosocial stress in both groups, and captured the course of salivary cortisol, salivary alpha-amylase (sAA), blood pressure (BP), and subjective stress.
2. Methods
2.1. Participants
We tested 56 healthy free-cycling women (n = 29 Hedione group) aged 18 to 32 years with a BMI between 18 and 30 kg/m2 in their follicular phases (day 4–9 of menstrual cycle). For sample size calculation using G-power 3.1.9.2 (Faul et al., Citation2007), the effect size estimate (Hedges g = 0.36, corresponds to f = 0.18) was inferred from a meta-analysis on human fear chemosignalling (de Groot & Smeets, Citation2017). From a TSST study conducted previously in our laboratory, we derived the correlation between repeated cortisol samples for the four time points corresponding to our experiment (r = 0.59). To detect the estimated effect with our 2 (group) × 4 (time) design, assuming a restrictive nonsphericity correction coefficient (ε = 0.5), an alpha error probability of .05 and a power of 80%, 58 participants were required.
Our choice of a female sample was based on Hedione eliciting a sex-specific activation of the hypothalamus with larger responses in women (Wallrabenstein et al., Citation2015). We expected effects of Hedione to emerge especially in women in their follicular phases, because they show higher sensitivity for social odors (Lundström et al., Citation2006), and their cortisol responses to the TSST are less pronounced than during the luteal phase (Kudielka & Kirschbaum, Citation2005).
Exclusion criteria were a BMI outside the range of 18–30 kg/m2, a history of mental disorders, chronic or acute illnesses, current medical or psychological treatment. Factors relevant for a functional sense of smell and the stress response were considered, including asthma, allergies, common cold, smoking, drug consumption, prior TSST participation, breastfeeding, pregnancy, shift work, as well as vaccination, blood donation or traveling with time shift in the last month. Eligibility was checked before the experiment in a standardized screening. Participants were instructed to avoid alcohol consumption and excessive physical activity 24 h before the experiment, to refrain from using fragrant cosmetics at the testing day, and from eating or drinking anything except for water within one hour before the experiment.
2.2. Ethics statement
The study was in accordance with the Declaration of Helsinki, and approved by the local ethics committee (Faculty of Psychology, Ruhr University Bochum, proposal #434). All participants signed informed consent and were reimbursed with 15€.
2.3. Procedure
Testing was conducted between 12:30 pm and 5:00 pm. The experimental timeline is illustrated in . The testing room (55.2 m³) was either odor-free or scented with Hedione (order # 947325, Firmenich, Meyrin, Switzerland, 5% solution in propylene glycol) via application of 5 ml on an Aroma Stream (TAOASIS GmbH, Detmold, Germany). Reconditioning of the testing room in between the sessions was achieved via window ventilation for 30 min directly after the testing session. No more than one participant was tested per day.
Figure 1. Timeline of the experiment. In the habituation phase (minute 0–15), participants gave informed consent and completed a self-developed questionnaire rating different properties of the experimental room. After 15 min, the first baseline measurement was conducted (minute 15–17), including a salivary sample, the completion of a visual analogue scale (VAS), and a cardiovascular measurement. Then, the Trier Social Stress Test took place (TSST; Kirschbaum et al., Citation1993, minute 17–32). During the preparation period of the TSST, participants completed the Primary Appraisal Secondary Appraisal Scale (PASA; Gaab et al., Citation2005) assessing anticipatory appraisal of the stressful situation. Additional cardiovascular measurements were conducted during the TSST preparation phase and during the stressful task. Following the TSST, three further stress measurements were conducted, directly after the TSST (+15min), 10 min after the end of the TSST (+25min) and 30 min after the end of the TSST (+45min). After this, participants were debriefed and reimbursed.
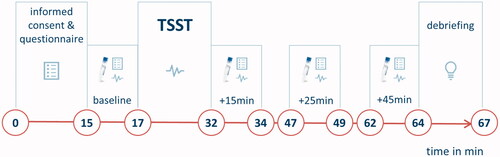
2.3.1. Stress induction and measurement
To induce acute psychosocial stress, a modified version of the Trier Social Stress Test (TSST; Kirschbaum et al., Citation1993) was applied. It consisted of a five minute preparation period, followed by a five minute mock job interview and a five minute mental arithmetic task (counting backwards from 2043 in steps of 17). Participants were informed that they were video-taped and observed by an evaluation panel, consisting of a man and a woman dressed in white lab coats. The panel was trained to act in a neutral way and to respond with standardized verbal phrases.
As a biomarker of HPA axis activity, salivary cortisol was measured at the four stress measurements (see ) using Salivettes (Sarstedt, Nümbrecht, Germany). In between the analysis of the first and second load of salivary samples, a change of the assay in the laboratory was required. Thus, for 26 participants (n = 12 control group, n = 14 Hedione group), salivary cortisol was determined using commercial enzyme-linked immunosorbent assays (ELISAs; free cortisol in saliva; Demeditec, Germany), analyzed on a Synergy2 plate reader (Biotek, USA). Intra- and inter-assay variability were lower than 4.61% and 7.02%. The second load of salivary samples (n = 15 of each group) was analyzed with a time-resolved fluorescence immunoassay (IBL; Hamburg, Germany). Intra-assay variation was below 5%, and inter-assay variation below 11%.
Capturing the sympathetic response, the enzyme sAA was determined from the saliva (Nater & Rohleder, Citation2009). To this end, a colorimetric test using the substrate reagent 2-chloro-4-nitrophenyl-α-maltrotriosoide (CNP-G3) was applied. Intra- and inter-assay variabilities were below 9%. As cardiovascular indicators, heart rate, systolic and diastolic BP were measured on the non-dominant arm using Critikon Dinamap Vital Daten Monitor 1846 SX (Critikon, Inc., Tampa, FL). They were assessed along with the four stress measurements and additionally, during preparation period and TSST.
For subjective stress, participants completed a visual analogue scale (VAS; from 0 = not stressed to 100 = extremely stressed) at the four stress measurements (see ). During the preparation period, anticipatory stress was assessed via the Primary Appraisal Secondary Appraisal scale (PASA; Gaab et al., Citation2005), consisting of 24 items related to primary and secondary appraisal of the stressful situation.
2.4. Confounding variables
Participants believed our intention to be investigating the effect of different experimental rooms on the stress response. Thus, they were sensitized toward features of the environment without being aware of the hypotheses. Via a self-developed questionnaire assessing valence of features of the experimental room (size, temperature, illumination, smell, wall colour) on a 9-point pictorial scale (from 1 = unpleasant to 9 = pleasant), we could compare valence of the ambient odor between the groups.
2.5. Statistical analysis
Statistical analyses were conducted using R (version 3.6.3) and RStudio (version 1.2.5042). Differences between the groups were checked via independent t-tests or, when the assumption of normality was violated, non-parametric Man-WhitneyUTests. Whether cortisol data were affected by the assay was tested in a 2(assay) × 4(time) ANOVA. For sAA, one participant was excluded due to extreme values exceeding 3SD above the mean at all time points.
Participants showing at least 15.5% increase in salivary cortisol from baseline to +25min were defined as cortisol responders (Miller et al., Citation2013). Pearson’s Chi2 test was used to compare cortisol responder rates between the groups. To compare stress responses between the groups, 4 × 2 ANCOVAs with the within-subjects factor time (baseline, +15min, +25min, +45min), the between-subjects factor group (Hedione/control), and the covariates age, BMI and assay were conducted for salivary cortisol. The same analyses, without assay as a covariate, were conducted for sAA and the VAS. For heart rate, systolic and diastolic BP, the within-subjects factor time had six levels (baseline, preparation, TSST, +15min, +25min, +45min). Comparing the groups regarding the PASA stress index, an independent t-test was performed. The assumption of normality was violated for salivary cortisol, sAA, and VAS, which were thus log-transformed. In case of non-sphericity, Greenhouse Geisser correction was applied. To confirm the robustness of our results, all analyses were additionally conducted without covariates and are reported in the Supplementary material.
3. Results
3.1. Sample description
Despite random assignment, the groups differed in age (t = 2.37, p=.03, d= −.63) and BMI (w = 226, p=.01, r= −0.37). Participants in the control group were slightly older (M = 26 years, SD = 2.97) than in the Hedione group (M = 23.83 years, SD = 3.81), and had a slightly lower BMI (M = 20.96 kg/m2, SD = 1.74) than in the Hedione group (M = 23.21 kg/m2, SD = 3.41). Valence of the room odor was not perceived differently (w = 436.5, p=.46, r = 0.1) between the control (M = 6.48, SD = 2.01) and Hedione (M = 6.00, SD = 2.15) groups. Cortisol data differed depending on the assay, as revealed by a main effect of assay (F(1,53)=6.68, p = 0.01, =.11), and a time*assay interaction (F(1.36, 1.73)=1.73, p=.04,
=.07) in the 2(assay) × 4(time) ANOVA.
3.2. Effects of Hedione on salivary cortisol
Of all participants, 62.5% showed a cortisol response exceeding 15.5% increase from baseline to +25min after the TSST. The chi2 test (χ2(1) = 4.58, p = .03) revealed more responders in the Hedione (75.86%) than in the control group (48.15%, ).
Figure 2. Cortisol stress response displayed by group. Cortisol responder rates (A) were higher in the Hedione than in the control group. The time course of cortisol levels (B) differed between the Hedione (red) and control group (blue). Note. **p < .01. ***p < .001. Shaded errors represent the SEM.
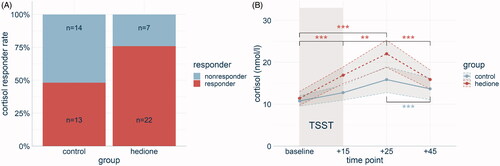
The 4 (time) × 2 (group) ANCOVA for salivary cortisol with assay, age, and BMI as covariates revealed a main effect of time (F(1.32, 70.37) = 7.80, p < .001, =.25). Pairwise comparisons showed a cortisol increase from baseline to +15 min (p < .001) with peak levels at +25min, being higher than at any of the other three time points (p’s <.001). No main effect of group was revealed (F(1,53) = 0.19, p = .66,
= .00), but a time*group interaction that barely missed significance after Greenhouse Geisser correction (F(1.32, 70.37) = 1.54, p = .05,
= .06). Following up this effect with separate repeated measures ANCOVAs for each group, we observed main effects of time for the Hedione (F(2.52, 3.95) = 7.80, p < .001,
=.25) and control group (F(0.42, 3.55) = 1.42, p < .001,
= .11). However, paired t-tests revealed different time courses of salivary cortisol in the two groups, which are visualized in .
3.3. Effects of Hedione on the autonomic nervous system
The 4 (time) × 2 (group) ANCOVA for sAA with age and BMI as covariates resulted in a main effect of time (F(2.35, 124.58) = 15.52, p < .001, = .27). Pairwise t-tests revealed higher sAA levels at +15min than at all other time points and higher sAA levels at +25min than at baseline. No main effect of group (F(1,53) = 0.09, p = .76,
= .00), and no time*group interaction (F(2.35, 124.58) = 0.15, p = .86,
=.00) were observed ().
Figure 3. Response of the autonomic nervous system displayed by group. The time course of systolic blood pressure (BP) differed between the two groups, with a stronger increase in the Hedione group (A). The time course of salivary alpha-amylase levels did not differ between the groups (B). Note. *p < .05. Shaded errors represent the SEM.
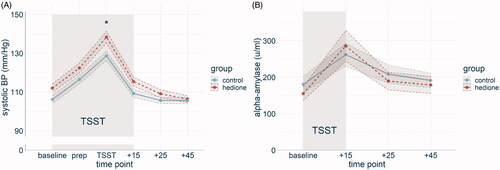
For systolic BP (), the 6 (time) × 2 (group) ANCOVA with age and BMI as covariates revealed a main effect of time (F(3.36, 174.82) = 88.91, p < .001, = .72). Post-hoc pairwise t-tests showed that systolic BP was higher during the TSST than at all other time points (p’s < .001) and higher at the preparatory period than at baseline, +25min and +45min (p’s < .001). There was no main effect of group (F(1, 52) = 1.15, p = .29,
= .02). A significant time*group interaction (F(3.36, 174.82) = 1.91, p = .03,
=.05) was followed up by comparing both groups across all time points. Only during TSST, the difference between the groups was significant (t(52) = −2.60, p = .01, d = .71). Diastolic BP changed over time (F(3.4, 176.9) = 59.33, p < .001,
= .63), but neither a main effect of group (F(1,52) = 0.09, p = .92,
=.00), nor a time*group interaction (F(3.4, 176.9) = 0.72, p = .38,
= .02) were found. Likewise, heart rate changed over time (F(2.6, 135.4) = 41.9, p < .001,
= .61). Post-hoc pairwise t-tests showed that in the preparation phase, and during the TSST, it was higher than at all other time points (p’s < .001). Neither a main effect of group (F(1,52) = 2.50, p = .12,
= .05), nor a time*group interaction (F(2.6, 135.4) = 0.32, p = .59,
= .01) were found.
3.4. Effects of Hedione on subjective stress
The PASA stress index did not differ between the groups (t(54) = .25, p = .80, d= −.07, ). A main effect of time for the VAS (F(2.44, 131.97) = 53.14, p < .001, = .55) was driven by higher VAS scores at +15 than at any other time point and lower VAS scores at +45 than at any other time point. No main effect of group (F(1, 54) = 0.09, p = .70,
= .00), and no time*group interaction (F(2.44, 131.97) = .91, p = .34,
= .02) were found ().
4. Discussion
In the present study, we investigated the impact of Hedione on the human stress response. For this purpose, we captured stress responses of women in their follicular phases, who were exposed to the TSST in a room scented with Hedione or no odor. Our results show more cortisol responders in the Hedione group, along with a strong trend indicating enhanced cortisol responses in the Hedione group. Systolic BP increased more strongly in the Hedione than in the control group. Neither sAA, nor subjective stress were affected by exposure to Hedione.
Our findings show enhanced cortisol secretion in response to an acute psychosocial stressor when exposed to the odorant Hedione. This could be an adaptive mechanism, fostering the reestablishment of homeostasis after a challenging situation (Selye, Citation1950; Ulrich-Lai & Herman, Citation2009). Hedione appears to exert its effect on the HPA axis only in response to acute stress, since baseline cortisol levels did not differ between the groups. Such adjustment of the cortisol stress response can go along with adaptive psychological outcomes, such as reduced negative affect (Het et al., Citation2012) or enhanced emotion regulation (Langer et al., Citation2021). A potential pathway for the effects of Hedione on the HPA axis stress response could be Hedione binding to the VN1R1 receptor, resulting in enhanced hypothalamic activation (Wallrabenstein et al., Citation2015) – with the paraventricular nucleus of the hypothalamus being the starting point of the HPA axis stress response. The absence of an effect on subjective stress and of differences in odor valence support the assumption that Hedione exerts its effects due to odorant-specific features, and not due to a mere psychological mechanism (Herz, Citation2009).
Hedione further differentially affected aspects of the ANS. It enhanced reactivity of systolic BP to the acute psychosocial stressor, while leaving sAA unaffected. Since correlations between sAA and cardiovascular measures are relatively small (Nater & Rohleder, Citation2009), this argues for a selective targeting of the cardiovascular response. Elevated reactivity of systolic BP by Hedione could be adaptive in a stressful situation, guaranteeing the availability of oxygen and energy in the body (Cannon, Citation1932; Ulrich-Lai & Herman, Citation2009). Increased stress reactivity of systolic BP is further considered the strongest cardiovascular predictor of health-related outcomes, and was associated with better self-perceived health (Wright et al., Citation2014).
Our findings raise interesting questions for future research. First, the stress-enhancing effect of Hedione occurred in response to an acute psychosocial stressor. Thus, like human chemosignals (de Groot et al., Citation2017; Lübke & Pause, Citation2015), Hedione might have a social communicative function, i.e. enhancing the sensitivity for social signals. It should be tested whether enhanced stress-reactivity would also occur in response to acute physiological or cognitive stressors. Besides, a systematic investigation of sex and menstrual cycle phase is warranted, since they both modulate stress and olfaction (Kudielka & Kirschbaum, Citation2005, Lundström et al., Citation2006). For now, it remains unclear whether the effects of Hedione on the human stress response are restricted to women in their follicular phases or whether they would generalize across sex, menstrual cycle phase or even other potential modulators, such as age. Moreover, studies including a stress-free control group, as well as a control odor condition will help to attribute the effects to this particular odorant. Further, it is important to consider when interpreting our findings that the effect sizes indicate rather small effects of Hedione on the human stress response. This corresponds to the meta-analytic effect size estimate of stress-related chemosignalling in general (de Groot et al., Citation2017). It will be necessary to validate these effect sizes both within and outside of the laboratory setting.
Taken together, our study provides initial evidence for a modulation of the human response to acute psychosocial stress by Hedione. The odorant enhanced the cortisol and cardiovascular stress response, possibly reflecting the binding of Hedione to the VN1R1 receptor.
Supplemental Material
Download PDF (415.3 KB)Acknowledgements
We would like to thank Jana Böttner, Dennis Pomrehn, and Niklas Schürmann for their help with data collection.
Disclosure statement
The authors report no conflict of interest.
Additional information
Funding
Notes on contributors
Anika Pützer
Anika Pützer, PhD is a postdoc at the Department of Cognitive Psychology at Ruhr University Bochum, Germany. Her research focuses on stress, olfaction, and memory.
Oliver T. Wolf
Oliver T. Wolf is full Professor and head of the Department of Cognitive Psychology at Ruhr University Bochum, Germany (since 2007). For more than 25 years he has investigated the impact of acute and chronic stress on cognitive processes in humans taking a psychoneuroendocrine perspective.
References
- Bensafi, M. (2004). Sniffing a human sex-steroid derived compound affects mood and autonomic arousal in a dose-dependent manner. Psychoneuroendocrinology, 29(10), 1290–1299. https://doi.org/https://doi.org/10.1016/j.psyneuen.2004.03.007
- Berger, S., Hatt, H., & Ockenfels, A. (2017). Exposure to Hedione increases reciprocity in humans. Frontiers in Behavioral Neuroscience, 11, 79. https://doi.org/https://doi.org/10.3389/fnbeh.2017.00079
- Cannon, W. (1932). The wisdom of the body. Norton and Co.
- de Groot, J. H. B., & Smeets, M. A. M. (2017). Human fear chemosignaling: Evidence from a meta-analysis. Chemical Senses, 42(8), 663–673. https://doi.org/https://doi.org/10.1093/chemse/bjx049
- Faul, F., Erdfelder, E., Lang, A., & Buchner, A. (2007). G* Power 3: A flexible statistical power analysis program for the social, behavioral, and biomedical sciences. Behavior Research Methods, 39(2), 175–191. https://doi.org/https://doi.org/10.3758/BF03193146
- Gaab, J., Rohleder, N., Nater, U. M., & Ehlert, U. (2005). Psychological determinants of the cortisol stress response: The role of anticipatory cognitive appraisal. Psychoneuroendocrinology, 30(6), 599–610. https://doi.org/https://doi.org/10.1016/j.psyneuen.2005.02.001
- Herz, R. S. (2009). Aromatherapy facts and fictions: A scientific analysis of olfactory effects on mood, physiology and behavior. International Journal of Neuroscience, 119(2), 263–290. https://doi.org/https://doi.org/10.1080/00207450802333953
- Het, S., Schoofs, D., Rohleder, N., & Wolf, O. T. (2012). Stress-induced cortisol level elevations are associated with reduced negative affect after stress: Indications for a mood-buffering cortisol effect. Psychosomatic Medicine, 74(1), 23–32. https://doi.org/https://doi.org/10.1097/PSY.0b013e31823a4a25
- Hur, M.-H., Song, J.-A., Lee, J., & Lee, M. S. (2014). Aromatherapy for stress reduction in healthy adults: A systematic review and meta-analysis of randomized clinical trials. Maturitas, 79(4), 362–369. https://doi.org/https://doi.org/10.1016/j.maturitas.2014.08.006
- Kirschbaum, C., Pirke, K.-M., & Hellhammer, D. H. (1993). The ‘Trier social stress test’—A tool for investigating psychobiological stress responses in a laboratory setting. Neuropsychobiology, 28(1–2), 76–81. https://doi.org/https://doi.org/10.1159/000119004
- Kudielka, B. M., & Kirschbaum, C. (2005). Sex differences in HPA axis responses to stress: A review. Biological Psychology, 69(1), 113–132. https://doi.org/https://doi.org/10.1016/j.biopsycho.2004.11.009
- Langer, K., Jentsch, V. L., & Wolf, O. T. (2021). Cortisol promotes the cognitive regulation of high intensive emotions independent of timing. European Journal of Neuroscience, 1–15. https://doi.org/https://doi.org/10.1111/ejn.15182
- Lübke, K. T., & Pause, B. M. (2015). Always follow your nose: The functional significance of social chemosignals in human reproduction and survival. Hormones and Behavior, 68, 134–144. https://doi.org/https://doi.org/10.1016/j.yhbeh.2014.10.001
- Lundström, J. N., McClintock, M. K., & Olsson, M. J. (2006). Effects of reproductive state on olfactory sensitivity suggest odor specificity. Biological Psychology, 71(3), 244–247. https://doi.org/https://doi.org/10.1016/j.biopsycho.2005.07.001
- Miller, R., Plessow, F., Kirschbaum, C., & Stalder, T. (2013). Classification criteria for distinguishing cortisol responders from nonresponders to psychosocial stress: Evaluation of salivary cortisol pulse detection in panel designs. Psychosomatic Medicine, 75(9), 832–840. https://doi.org/https://doi.org/10.1097/PSY.0000000000000002
- Nater, U. M., & Rohleder, N. (2009). Salivary alpha-amylase as a non-invasive biomarker for the sympathetic nervous system: Current state of research. Psychoneuroendocrinology, 34(4), 486–496. https://doi.org/https://doi.org/10.1016/j.psyneuen.2009.01.014
- Pützer, A., Brüne, M., Hatt, H., & Wolf, O. T. (2019). Hedione Reduces Subjective Vicarious Stress. Frontiers in Behavioral Neuroscience, 13, 297. https://doi.org/https://doi.org/10.3389/fnbeh.2019.00297
- Selye, H. (1950). Stress and the general adaptation syndrome. British Medical Journal, 1(4667), 1383–1392. https://doi.org/https://doi.org/10.1136/bmj.1.4667.1383
- Ulrich-Lai, Y. M., & Herman, J. P. (2009). Neural regulation of endocrine and autonomic stress responses. Nature Reviews. Neuroscience, 10(6), 397–409. https://doi.org/https://doi.org/10.1038/nrn2647
- Wallrabenstein, I., Gerber, J., Rasche, S., Croy, I., Kurtenbach, S., Hummel, T., & Hatt, H. (2015). The smelling of Hedione results in sex-differentiated human brain activity. NeuroImage, 113, 365–373. https://doi.org/https://doi.org/10.1016/j.neuroimage.2015.03.029
- Wright, B. J., O'Brien, S., Hazi, A., & Kent, S. (2014). Increased systolic blood pressure reactivity to acute stress is related with better self-reported health. Scientific Reports, 4(1), 6882 https://doi.org/https://doi.org/10.1038/srep06882