Abstract
Anxiety is a complex emotional state associated with sustained heightened autonomic and behavioral arousal and an increase in avoidance behavior. Anxiety-related behavior is a form of risk assessment behavior that is associated with a level of uncertainty or unpredictability regarding the outcome of emotionally salient events, often when both rewarding and aversive outcomes are possible. In this review, we highlight recent advances in our understanding of the neural circuits regulating anxiety states and anxiety-related behavior with an emphasis on the role of brainstem serotonergic systems in modulating anxiety-related circuits. In particular, we explore the possibility that the regulation of anxiety states and anxiety-related behavior by serotonergic systems is dependent on a specific, topographically organized mesolimbocortical serotonergic system that originates in the mid-rostrocaudal and caudal parts of the dorsal raphe nucleus.
Introduction
Aims and scope of review
Anxiety-related physiological and behavioral responses are mediated by specific neural circuits in the brain. Serotonin is an important modulator of these anxiety-related circuits but the mechanisms through which serotonin modulates these circuits and the associated physiological and behavioral responses are not fully understood (Iversen Citation1984; Handley Citation1995; Graeff et al. Citation1996; Graeff et al. Citation1997; Maier and Watkins Citation1998; Zhuang et al. Citation1999Gray and McNaughton Citation2000; Graeff Citation2002; Millan Citation2003; Maier and Watkins Citation2005). In this review, we have three aims. Our first aim is to present operational definitions of anxiety states and anxiety-related behavior in animal models. Our second aim is to briefly define neural systems involved in modulating anxiety- related behaviors. Our third aim is to consider how serotonergic systems specifically modulate these anxiety-related circuits. Anatomical evidence suggests that serotonergic systems are topographically organized within the brainstem raphe nuclei, with serotonergic neurons in different regions of the brainstem raphe nuclei projecting to different functional systems (Imai et al. Citation1986; Lowry Citation2002; Abrams et al. Citation2005). Consequently, we argue that in order to understand the role of serotonergic systems in the regulation of anxiety-related behavior and anxiety states, it will be important to characterize the anatomical distribution and the cellular and functional properties of serotonergic neurons that project to neural structures regulating anxiety-related behaviors. As it is not possible, within the constraints of this review, to consider the effects of serotonergic systems on all components of the neural circuits underlying anxiety-related behaviors in animal models and humans, we will focus on serotonergic systems that project to the basolateral amygdala, a nodal structure in the neural circuits modulating anxiety-related behavior in rodents (Millan Citation2003). In this review, we will not discuss anxiety disorders in humans, which are thought to involve dysfunction of neural systems, including serotonergic systems, underlying anxiety- or fear-related states. For this, the reader is directed to previous reviews on this topic (Nutt et al. Citation1990, Citation1998, Citation1999; Handley Citation1995; Bell and Nutt Citation1998; Nutt Citation2001, Citation2005).
Operational definition of anxiety states and anxiety-related behavior
Anxiety is a complex emotional state associated with sustained heightened autonomic and behavioral arousal and a sustained increase in avoidance behavior in environmental situations characterized by a level of uncertainty, unpredictability, or uncontrollability (Walker et al. Citation2003; Herry et al. Citation2005; Maier and Watkins Citation2005), including situations where a conflict between approach and avoidance exists (Millan Citation2003). An important component of anxiety states is that the associated increases in anxiety-related physiological and behavioral responses are not tied to specific environmental cues but are trans-situational (Maier and Watkins Citation2005), meaning that they can be elicited under diverse environmental contexts. Anxiety-related behavior is associated with a level of uncertainty or unpredictability regarding the outcome of emotionally salient events, often when both rewarding and aversive outcomes are possible. Anxiety-related behavior in animals, which includes increased vigilance and scanning of the environment, has been referred to as “risk assessment” behavior (Blanchard and Blanchard Citation1989). In a complex unpredictable environment, the individual may perceive simultaneously the potential for both rewarding and aversive outcomes of specific behavioral strategies. For example, a black-tailed prairie dog in the shortgrass prairie may desire to exit its burrow to forage on young grass shoots or explore the environment in search of other potential rewards; if upon emerging from the burrow it detects the odor of a fox, a natural predator, then a conflict arises. This conflict, between approaching a potential rewarding stimulus and avoiding a potential aversive stimulus, is central to many anxiety states.
Anxiety states may therefore involve unique neural mechanisms mediating vigilance and scanning behaviors in situations characterized by a level of uncertainty or unpredictability with respect to the outcome of events, leading to the identification of the valence of potential outcomes (positive or negative), and predictions as to the probability of rewarding or aversive outcomes based on different behavioral strategies. A cardinal feature of anxiety-related behaviors as they have classically been defined is that they are benzodiazepine-sensitive; i.e. benzodiazepine drugs can shift the balance between approach and avoidance behaviors, reducing avoidance behaviors and/or increasing approach behaviors (Gray Citation1982; Gray and McNaughton Citation2000). Other behavioral responses that have been associated with anxiety states, such as enhanced startle in a brightly lit open field environment, are also benzodiazepine-sensitive (Walker et al. Citation2003). Behavioral paradigms that involve purely avoidance responses (e.g. escape responses on an elevated T-maze), and therefore, do not involve a conflict between approach and avoidance behaviors or choices between different behavioral strategies, are not sensitive to benzodiazepine drugs (Graeff et al. Citation1998; Zangrossi, Jr. et al. Citation1999).
In the case of anxiety states that involve conflicts between approach and avoidance behaviors, there are multiple mechanisms though which increases in anxiety-related behavior can occur. For example, in an open field test, a commonly used measure of anxiety-related behavior in rodents, there is a conflict between a natural tendency to explore the central region of the novel environment (approach behavior) and the tendency to remain in the relative safety of the sides and corners of the apparatus (avoidance behavior). An increase in avoidance behavior (or a decrease in approach behavior) may be observed as a result of (1) activation of neural mechanisms regulating avoidance behaviors (e.g. increasing the perceived aversive nature of a stimulus or the perceived probability of an aversive outcome), (2) inhibition of neural mechanisms regulating approach behaviors (e.g. decreasing the perceived rewarding nature of a stimulus or the perceived probability of a rewarding outcome), (3) disruptions of the ability to accurately predict potential rewarding or aversive outcomes of specific behavioral strategies, or (4) disruptions of the ability to attach emotional salience to potential rewarding or aversive outcomes. Thus, anxiety-related behavior can be viewed as the output of neural mechanisms involved in assessing the valence, relative value, and emotional salience of stimuli, and the relative probability of the outcomes of specific behavioral strategies.
Experimental models of anxiety-related behavior
Many experimental models of anxiety-related behavior in rodents involve a conflict between approach and avoidance behaviors. In some cases, the conflict between approach and avoidance is immediately apparent. For example, in the Geller-Seifter Conflict Test and the Vogel Conflict Test, food or water deprived rats are simultaneously punished with a mild shock when performing behaviors to obtain a reward (e.g. food, or drink, respectively). In other cases, such as those based on exploration of a novel object or novel environment, the conflict is less obvious. A conflict exists between exploring a novel environment, a conspecific, or an object which is a potential source of reward and the real or perceived risks associated with exploring a novel environment. The balance between approach and avoidance behaviors can be manipulated by the experimenter by altering parameters such as light intensity (Valle Citation1970; Cunha and Masur Citation1978; Hall et al. Citation2000). For rodents, which are nocturnal animals, bright light is more aversive than low light and results in more avoidance behaviors (e.g. due to an increase in the perceived aversive nature of the stimulus). Conversely, in the Geller-Seifter or Vogel Conflict tests, the rewarding properties of food or water, respectively, are increased by food or water deprivation before the test, increasing baseline approach behaviors. The latter manipulations shift the baseline of responding by altering motivational aspects of behavior as opposed to specifically modulating anxiety-related mechanisms.
Although, animal models involving a conflict between approach and avoidance behaviors have been very useful in characterizing the anxiolytic and anxiogenic properties of drugs and in defining the neural systems underlying anxiety states and anxiety-related behaviors (Gray and McNaughton Citation2000; Millan Citation2003), this does not mean that a conflict between approach and avoidance is an essential feature of anxiety states or anxiety-related behaviors. It may be that uncertainty, unpredictability, or uncontrollability in the presence of a potential aversive outcome is sufficient to evoke an anxiety state (Walker et al. Citation2003; Maier and Watkins Citation2005), or that unpredictability per se is the essential stimulus leading to the development of an anxiety state (Herry et al. Citation2005). Recent studies in rats and humans suggest that an unpredictable, relative to a predictable, auditory stimulus leads to activation of the basolateral amygdala, a nodal structure in the neural circuits regulating anxiety-related behavior (see below), and an increase in anxiety-related behavior as measured in the elevated plus-maze (Herry et al. Citation2005). Activation of the afferents from the basolateral amygdala results in direct monosynaptic excitatory postsynaptic responses in layer V pyramidal neurons in the medial prefrontal cortex (mPFC; Orozco-Cabal et al. Citation2005), neurons that are known to project directly to the dorsal raphe nucleus and to control serotonergic neuronal activity (Hajos et al. Citation1998). Both the mPFC and the dorsal raphe nucleus have been implicated in regulating the behavioral consequences of unpredictable or uncontrollable stress (Amat et al. Citation2005; Maier and Watkins Citation2005). Thus, the basolateral amygdala, mPFC, and dorsal raphe may constitute an important circuit determining the behavioral consequences of unpredictable or uncontrollable stress, including increases in avoidance behaviors in anxiety tests (Amat et al. Citation2005; Maier and Watkins Citation2005).
Neural circuits modulating anxiety-related behavior
As defined above, anxiety-related behavior is a form of risk assessment behavior associated with a level of uncertainty or unpredictability regarding the outcome of emotionally salient events, often when both rewarding and aversive outcomes are possible. This suggests that neural circuits underlying anxiety-related behaviors may encompass neural circuits associated with approach or reward-related behaviors, avoidance behaviors, and decision-making based on the predicted probabilities of the outcomes of specific behavioral strategies.
The neural systems underlying approach behaviors or reward pathways are well characterized, and there are several excellent reviews on this topic (Berridge and Robinson Citation1998; Kalivas and Nakamura Citation1999; Dayan and Balleine Citation2002; Berridge Citation2004; Kelley Citation2004; Morgane et al. Citation2005). An important component of neural circuits underlying reward pathways and approach behaviors is the mesolimbic dopaminergic projection from the ventral tegmental area in the midbrain to the nucleus accumbens. The reward circuitry is modulated by important input from the prefrontal cortex, hippocampus, and amygdala (Kelley Citation2004; Morgane et al. Citation2005).
Attempts also have been made to understand the neural systems involved in the modulation of the conflict between approach and avoidance that is inherent in many forms of anxiety states (Gray Citation1982; Gray and McNaughton Citation1987; Gray and McNaughton Citation2000; Millan Citation2003). Intuitively, it seems that neural systems mediating approach and avoidance behaviors (particularly passive avoidance behaviors; c.f. Gray and McNaughton; Gray Citation1982) must be involved, but that higher order processes are likely to be involved in predicting outcomes of specific behavioral responses and resolving conflicts between two opposing behavioral strategies. For example, Gray has suggested that the septo-hippocampal system plays a critical role in the neurobiology of anxiety states and in particular that it plays a critical role in predicting the outcome of specific behavioral responses, (Gray Citation1982; Gray and McNaughton Citation2000), an idea elaborated in what is called the “comparator model of hippocampal function” (Gray Citation1983; Gray and McNaughton Citation2000; Vinogradova Citation2001). These ideas are consistent with recent findings that hippocampal “place cells” encode not only instantaneous place but also encode information about past and future events [e.g. prospective and retrospective encoding (Ferbinteanu and Shapiro Citation2003; Jeffery Citation2004)]. For example, some hippocampal place cells are modulated (either switched ‘on’ or ‘off’) depending on whether the rat is going to turn right or left in a maze to obtain a reward (prospective coding). This function of the hippocampus may be particularly relevant to risk assessment behavior where predictions about the potential outcome of different behavioral strategies (approach or avoidance) are required. In contrast, the basolateral amygdala may be involved in assigning emotional salience to both potential rewarding and potential aversive outcomes (see below), while the bed nucleus of the stria terminalis (BNST) is thought to be part of a lateral or specific emotional motor system (Holstege Citation1995) that is specifically involved in behavioral responses to diffuse aversive signals such as bright light (Davis Citation1998; Walker et al. Citation2003), and the mPFC is thought to be involved in behavioral suppression (Lacroix et al. Citation2000) and essential for the effects of controllability on dorsal raphe serotonergic systems and behavior (Amat et al. Citation2005). Finally, the anterior cingulate cortex, at least in humans, is thought to have a fundamental role in relating actions to their consequences, both positive reinforcement outcomes and errors, and in making choices between different behavioral strategies, based on their potential outcomes (Rushworth et al. Citation2004). Indeed, a distributed neural system is acknowledged by most investigators to play an important role in the regulation of anxiety-related behavior and anxiety states, including the septohippocampal system, basolateral amygdala, BNST, mPFC, and cingulate cortex (Davis Citation1998; Gray and McNaughton Citation2000; Millan Citation2003; Walker et al. Citation2003). Thus, neural circuits mediating anxiety-related behaviors are likely to encompass aspects of reward-related approach pathways and avoidance pathways but also to involve additional processes associated with predicting outcomes of specific behavioral strategies and making decisions on behavioral strategies based on the predicted outcomes.
The hypothesis that changes in anxiety states involve a highly distributed but anatomically interconnected neural system is supported by recent studies using immunohistochemical staining for the protein product of the immediate-early gene, c-fos. Immunohistochemical staining of Fos is a useful method for identifying neural pathways involved in responses to specific stimuli (Morgan and Curran Citation1991; Kovacs Citation1998). In a series of studies in rats, Singewald and Sharp demonstrated that injections of anxiogenic drugs (i.e. drugs that increase the subjective experience of anxiety in humans and increase avoidance behaviors in rodents in a variety of anxiety tests) with different pharmacological properties increase Fos expression in a distributed neural system (Singewald and Sharp Citation2000; Singewald et al. Citation2003). In the forebrain, all four anxiogenic drugs tested increased Fos expression in the central amygdaloid nucleus, the BNST, lateral septum, paraventricular nucleus of the hypothalamus, lateral hypothalamus, and the infralimbic and prelimbic regions of the mPFC. All drugs, excluding the serotonin agonist m-chlorophenylpiperazine (mCPP), also increased Fos expression in the basolateral and medial amygdala, the dorsomedial hypothalamus, cingulate cortex, and parts of the motor cortex (Singewald et al. Citation2003). Within the brainstem, all drugs increased Fos expression in the periaqueductal gray, locus coeruleus, lateral parabrachial nucleus, and nucleus of the solitary tract and all drugs except the cholecystokinin analogue BOC-CCK4 increased Fos expression in the dorsal raphe nucleus (Singewald and Sharp Citation2000). Most components of this distributed neural system had previously been identified as part of the neural network of brain structures involved in the integration and induction of anxiety states based on other methodological approaches (Gray Citation1982; Gray and McNaughton Citation2000; Millan Citation2003). Similar results have been observed in studies evaluating the effects of exposure to anxiogenic stimuli, such as the chemical component from fox feces, 2,5-dihydro-2,4,5-trimethylthiazoline (TMT) on c-fos mRNA expression in rats (Fendt et al. Citation2003; Day et al. Citation2004) (cf. (Duncan et al. Citation1996).
The basolateral amygdala as a nodal structure in anxiety-related circuits
Although it is recognized that the regulation of anxiety-related behavior will be the product of a distributed neural system, it will be instructive to look in detail at specific components of the system that appear to play a central role in these behaviors. As many anxiety-related behaviors involve conflict between approach and avoidance, it would be particularly instructive to focus on components of anxiety-related neural pathways that are involved in the attribution and/or association of emotional salience (of either a positive or negative nature) to specific environmental stimuli and that are connected anatomically and functionally to neural pathways mediating both approach and avoidance behaviors. One such structure is the basolateral amygdala. Indeed, based on lesion studies the basolateral amygdala has been identified as part of a system responsible for voluntary instrumental choice behavior based on emotional events. The lateral/basolateral nucleus appears to be critical for the sensory relay of the stimulus (LeDoux et al. Citation1990; Campeau and Davis Citation1995) and association and/or attribution of emotional salience to a variety of rewarding (Meil and See Citation1997; Ledford et al. Citation2003; Jackson and Moghaddam Citation2004) as well as aversive cues (Miserendino et al. Citation1990; Mayford et al. Citation1996; Rogan et al. Citation1997; Davis Citation1997; Shumyatsky et al. Citation2002). In simple terms, it has been suggested that the involvement of the basolateral amygdala in reward-related or avoidance-related processes is best characterized as relating to aspects of “liking” (Blundell et al. Citation2003) or “not liking” and the use of this information to modulate voluntary or intentional behavior (Killcross et al. Citation1997). There is also evidence that the basolateral amygdala is integral to emotional learning and disruption of this nucleus disrupts the memory enhancing effects of emotionally salient cues (Roozendaal et al. Citation1999; Schafe et al. Citation2000; Roozendaal et al. Citation2001; Ferry et al. Citation1999; McGaugh Citation2002; Goosens and Maren Citation2003; LaLumiere et al. Citation2003; Roesler et al. Citation2003). It is possible that different neurotransmitters act within the basolateral amygdala to differentially regulate approach and avoidance behaviors. There is accumulating evidence that the stress-related and anxiogenic neuropeptide corticotropin-releasing factor (CRF) acting within the basolateral amygdala may be critical for aversive or negative-cue induced behavioral responses (Lee et al. Citation1994; Heilig et al. Citation1994; Gray and Bingaman Citation1996; Sajdyk et al. Citation1999; Roozendaal et al. Citation2002; Heimer Citation2003). In contrast, dopamine in this nucleus may be more involved in the emotional salience elicited by reward cues (Ledford et al. Citation2003; Jackson and Moghaddam Citation2004). As would be expected based on these functional studies, the basolateral amygdala is anatomically integrated with neural pathways involved in both reward (Kelley Citation2004) and avoidance (Dong et al. Citation2001; Millan Citation2003). Consequently, we and others have focused on the potential role of the basolateral amygdala in regulation of anxiety-related behavior and anxiety states (Pesold and Treit Citation1995; Sanders and Shekhar Citation1995; Campbell and Merchant Citation2003; Etkin et al. Citation2004; Rainnie et al. Citation2004; Sajdyk et al. Citation2004; Abrams et al. Citation2005; Adamec et al. Citation2005).
Modulation of anxiety-related circuits by serotonin
In order to understand the role of serotonin in the regulation of anxiety-related behavior, it will be important to determine the effects of serotonin on the neural circuits that mediate anxiety-related behaviors. This represents a significant challenge for investigators. The effects of serotonin on these neural circuits are likely to depend on several variables. These include the time course of serotonin action, the extracellular concentrations of serotonin (which may determine which serotonin receptor populations are affected based on anatomical proximity to the site of release or the affinity of specific serotonin receptors for the ligand), the type and presynaptic or postsynaptic location of serotonin receptors within each specific brain region, systems level interactions among different nodes in the neural circuitry [e.g. interactions between the mPFC and the amygdala (Berretta et al. Citation2005; Pezawas et al. Citation2005), or the mPFC and the dorsal raphe nucleus (Amat et al. Citation2005)], differential proteolytic processing and co-release of peptidergic co-transmitters in serotonergic neurons (Blackmore et al. Citation2001), or context dependent changes in presynaptic and post-synaptic mechanisms regulating serotonergic signaling [initiated, for example, by glucocorticoid hormones (Stutzmann et al. Citation1998; Tronche et al. Citation1999)].
Despite this complexity, several lines of evidence suggest that serotonergic systems play an important role in modulating anxiety-related behaviors. In animal models of anxiety that involve conflict, drugs or lesions that reduce serotonergic activity can have anxiolytic effects that are qualitatively similar to the anxiolytic effects of benzodiazepines (Kahn et al. Citation1988; Graeff Citation1990; Griebel Citation1995; Graeff et al. Citation1996; Gray and McNaughton Citation2000). Microinfusions directly into the dorsal raphe nucleus of anxiogenic drugs that increase serotonergic activity, like the benzodiazepine receptor partial inverse agonists N-methyl-beta-carboline-3-carboxamide (FG-7142) (Lista et al. Citation1990), methyl 6,7-dimethoxy-4-ethyl-beta-carboline-3-carboxylate (DMCM) (Maier et al. Citation1995), and the anxiety-related neuropeptide urocortin 2 (Hammack et al. Citation2003; Amat et al. Citation2004; Maier and Watkins Citation2005) increase anxiety-related behavioral responses. In contrast, microinfusions of 5-HT or 5-HT1A agonists into the dorsal raphe decrease anxiety-related behavioral responses, presumably via activation of somatodendritic 5-HT1A autoreceptors on serotonergic neurons resulting in decreased 5-HT neurotransmission (Higgins et al. Citation1988; Higgins et al. Citation1992; Schreiber and De Citation1993). Likewise, intra-dorsal raphe microinfusions of other drugs expected to decrease serotonergic activity (e.g., the selective neurotoxin 5,7-dihydroxytryptamine, benzodiazepines, and the inhibitory neurotransmitter GABA) have all been reported to reduce behavioral measures of anxiety [see for review, Maier and Watkins (Citation1998)]. Consistent with these findings, selective lesion of serotonergic systems in the amygdala results in an anxiolytic effect in a punished drinking test (Sommer et al. Citation2001). Together, these findings are consistent with the classic hypothesis that serotonergic systems can facilitate anxiety-related behavior (Wise et al. Citation1972).
Effects of serotonin within the basolateral amygdala
Evidence suggests that the basolateral amygdala is an important site for the effects of serotonin on anxiety circuits. Electrical stimulation of the rat dorsal raphe serotonergic pathways or direct iontophoretic application of serotonin inhibits spontaneous neuronal activity within the cortical and basolateral amygdala in vivo (Wang and Aghajanian Citation1977) and activation of serotonin 5-HT1A and 5-HT2 receptors within the basolateral amygdala alters the electrophysiological properties of identified basolateral amygdala interneurons and projection neurons in vitro (Rainnie Citation1999; Stein et al. Citation2000). These findings suggest that serotonin modulates basolateral amygdala function. Evidence for both anxiogenic and anxiolytic effects of serotonin receptor activation within the basolateral amygdala has been described. For example, bilateral intra-basolateral amygdala injection of mCPP, a mixed 5-HT2 agonist, reduces exploratory behavior in an open field while increasing the latency to investigate a novel object. These responses are attenuated by prior treatment with SB-242084, a 5-HT2C receptor-specific antagonist (Campbell and Merchant Citation2003). 5-HT2C receptor activation within the basolateral amygdala may have long-term effects on anxiety-related behaviors as in vitro electrophysiological studies have demonstrated that 5-HT2 receptor activation initiates long-term potentiation that can be prevented by a selective 5-HT2C antagonist (Chen et al. Citation2003). Similarly bilateral intra-basolateral amygdala injection of 8-OH-DPAT, a 5-HT1A receptor agonist, increased anxiety-like behavior in the social interaction test (Gonzalez et al. Citation1996). This response was reversed by treatment with a 5-HT1A receptor antagonist. In contrast, intra-basolateral amygdala injections of 8-OH-DPAT had no effect on inhibitory avoidance behavior in the elevated plus-maze (Gonzalez et al. Citation1996), and had the opposite (anxiolytic) effect in the elevated T-maze test (Zangrossi, Jr. et al. Citation2001). These disparate findings might be expected as the basolateral amygdala is involved in assigning emotional salience to both potential rewarding and potential aversive stimuli and altering basolateral amygdala function is likely to modulate both approach and avoidance behaviors. In addition, it is possible that the actions of serotonin in the basolateral amygdala are involved in both the induction and the termination of anxiety-related behaviors via actions on different serotonin receptors or different postsynaptic targets. In summary, evidence suggests that 5-HT receptor activation within the basolateral amygdala does alter anxiety-related behavior, but additional studies will be required to develop a clear picture of the mechanisms involved. An important step in this direction is to understand the properties of serotonergic neurons that innervate anxiety-related circuits, including the basolateral amygdala. This includes their location within the brainstem raphe complex, their afferent regulatory mechanisms, and their unique molecular, cellular, and functional properties.
Functional subsets of serotonergic neurons
Serotonergic systems are topographically organized
Serotonergic systems within the brainstem raphe complex give rise to topographically organized projections to forebrain targets (Imai et al. Citation1986; Lowry Citation2002; Insel et al. Citation2003; Abrams et al. Citation2005). In other words, each region of the forebrain that is innervated by brainstem serotonergic systems has its own unique representation in the brainstem raphe complex. For example, neurons that project to the caudate putamen and substantia nigra are located within rostral regions of the dorsal raphe nucleus while those that project to the amygdala are concentrated in the mid-rostrocaudal region of the dorsal raphe nucleus, and those that project to the hippocampus are located in the most caudal regions of the dorsal raphe nucleus, the interfascicular part of the dorsal raphe, and the median raphe nucleus (Steinbusch et al. Citation1981; Köhler and Steinbusch Citation1982; Imai et al. Citation1986; Abrams et al. Citation2005). dorsal raphe neurons that project to the caudate putamen do not project to the hippocampus and vice versa (Imai et al. Citation1986). Individual dorsal raphe neurons projecting to the caudate putamen also project to other forebrain and brainstem structures involved in motor function including the substantia nigra (van der Kooy and Hattori Citation1980), and nucleus reticularis gigantocellularis pars α (Li et al. Citation2001). These findings suggest that individual serotonergic neurons give rise to collateral projections to distributed but functionally related targets. If we make the assumption that the subset of serotonergic neurons projecting to anxiety-related forebrain circuits plays a unique role in modulating anxiety-related behaviors and anxiety states, then these anatomical findings suggest that it may be possible to identify unique anatomical, morphological, and molecular properties of these serotonergic neurons.
Subsets of serotonergic neurons have different behavioral correlates
The presence of multiple subsets of serotonergic neurons with unique behavioral correlates within the dorsal raphe nucleus (Rasmussen et al. Citation1984; Sakai and Crochet Citation2001) is consistent with the hypothesis that subsets of serotonergic neurons may have functional properties linked to context-dependent forms of behavioral arousal (e.g. ambulatory locomotion versus anxiety-related behaviors). For example, a subpopulation (approximately 25% of those studied) of serotonergic neurons predominantly localized in ventral portions of the dorsal raphe nucleus is strongly activated in association with oral-buccal movements during chewing, licking, and grooming behaviors (Fornal et al. Citation1996). Subsequent studies in behaving rats have identified six different subtypes (types I-A, I-B, I-C, II-A, II-B, and II-C) of presumed serotonergic neurons based on their behavioral correlates (Sakai and Crochet Citation2001), several of which show distinct patterns of topographical organization within the dorsal raphe nucleus. The finding that anxiety-related behaviors are associated with increases in extracellular serotonin concentrations in the basolateral amygdala (Gonzalez et al. Citation2004), hippocampus, cortex, and septum, but not in the striatum (Beekman et al. Citation2005), is consistent with the hypothesis that mesolimbocortical and mesostriatal serotonergic systems can be independently regulated (Daugherty et al. Citation2001; Lowry Citation2002; Insel et al. Citation2004)
Distribution of serotonergic neurons projecting to anxiety-related circuits
Imai and colleagues have reported a rostrocaudal topography within the dorsal raphe with neurons projecting to the caudate putamen and substantia nigra located in the rostral region of the dorsal raphe nucleus, neurons projecting to the amygdala located in the mid-rostrocaudal dorsal raphe nucleus, and neurons projecting to the locus coeruleus and hippocampus in the caudal dorsal raphe nucleus (Imai et al. Citation1986). Although, it is unlikely that functional subsets of serotonergic neurons will be confined to subdivisions of the dorsal raphe nucleus that have been defined based on cytoarchitectonic criteria, these findings and others (Kohler et al. Citation1980; Ottersen Citation1981; Köhler and Steinbusch Citation1982; Köhler et al. Citation1982; Petrov et al. Citation1992, Citation1994; Van Bockstaele et al. Citation1993; Commons et al. Citation2003; Abrams et al. Citation2005) suggest that serotonergic neurons projecting to structures associated with neural circuits modulating anxiety states are concentrated within the mid-rostrocaudal and caudal dorsal raphe nucleus ().
Figure 1 Diagrammatic illustration of the topographical distribution of serotonergic neurons projecting to the caudate putamen and substantia nigra (blue) and serotonergic neurons projecting to brain structures implicated in the regulation of anxiety-related behavior (yellow) in rat brain (for references, see text). Although this is an oversimplification, these cell populations have a differential distribution in the dorsal raphe nucleus (DR), with serotonergic cells projecting to the caudate putamen and substantia nigra located in more rostral regions of the DR. In some cases, individual serotonergic neurons project to functionally related targets [e.g. CP/ SN (van der Kooy and Hattori Citation1980), mPFC/ Acb (Van Bockstaele et al. Citation1993)]. In some cases, serotonergic neurotransmission of the dorsal part of the mid-rostrocaudal and caudal DR can be independently regulated (Macedo et al. Citation2005), suggesting that although the innervation arises in part from the same brain region, it could also arise in part from different populations of serotonergic neurons. In the mid-rostrocaudal DR, serotonergic neurons projecting to the CP are highly concentrated within compact cell clusters in the DRD “core” and DRV “ellipse” regions. Numbers below illustrations of rostral, mid-rostrocaudal, and caudal DR indicate stereotaxic coordinates with reference to Bregma. Scale bar, 500 μm. Abbreviations, Acb, nucleus accumbens; BL, basolateral amygdala; BNST, bed nucleus of the stria terminalis; CE, central amygdaloid nucleus; CP, caudate putamen; DA, dorsal hypothalamic area; DRD, DR, dorsal part; DRI, DR, interfascicular part; DRV, DR, ventral part; DRVL, DR ventrolateral part; LC, locus coeruleus; LPB, lateral parabrachial nucleus; mPFC, medial prefrontal cortex; SN, substantia nigra.
![Figure 1 Diagrammatic illustration of the topographical distribution of serotonergic neurons projecting to the caudate putamen and substantia nigra (blue) and serotonergic neurons projecting to brain structures implicated in the regulation of anxiety-related behavior (yellow) in rat brain (for references, see text). Although this is an oversimplification, these cell populations have a differential distribution in the dorsal raphe nucleus (DR), with serotonergic cells projecting to the caudate putamen and substantia nigra located in more rostral regions of the DR. In some cases, individual serotonergic neurons project to functionally related targets [e.g. CP/ SN (van der Kooy and Hattori Citation1980), mPFC/ Acb (Van Bockstaele et al. Citation1993)]. In some cases, serotonergic neurotransmission of the dorsal part of the mid-rostrocaudal and caudal DR can be independently regulated (Macedo et al. Citation2005), suggesting that although the innervation arises in part from the same brain region, it could also arise in part from different populations of serotonergic neurons. In the mid-rostrocaudal DR, serotonergic neurons projecting to the CP are highly concentrated within compact cell clusters in the DRD “core” and DRV “ellipse” regions. Numbers below illustrations of rostral, mid-rostrocaudal, and caudal DR indicate stereotaxic coordinates with reference to Bregma. Scale bar, 500 μm. Abbreviations, Acb, nucleus accumbens; BL, basolateral amygdala; BNST, bed nucleus of the stria terminalis; CE, central amygdaloid nucleus; CP, caudate putamen; DA, dorsal hypothalamic area; DRD, DR, dorsal part; DRI, DR, interfascicular part; DRV, DR, ventral part; DRVL, DR ventrolateral part; LC, locus coeruleus; LPB, lateral parabrachial nucleus; mPFC, medial prefrontal cortex; SN, substantia nigra.](/cms/asset/89e61659-101d-4893-bd16-f492fa77f7ae/ists_a_149261_f0001_b.jpg)
In order to increase our understanding of the distribution of dorsal raphe neurons projecting to neural circuits modulating anxiety-related behaviors, we conducted a detailed analysis of the distribution of dorsal raphe neurons that project to the basolateral amygdala (Abrams et al. Citation2005). Although, dorsal raphe neurons projecting to the basolateral amygdala were widely distributed throughout the rostrocaudal extent of the dorsal raphe nucleus, these neurons were concentrated in the dorsal part of the middle and caudal dorsal raphe nucleus. Consistent with this observation, following a small injection of retrograde tracer into the anterior part of the basolateral amygdala, retrogradely labeled cells were largely restricted to the dorsal part of the mid-rostrocaudal dorsal raphe nucleus (). Comparison of the distribution of dorsal raphe neurons projecting to the basolateral amygdala with the distribution of dorsal raphe neurons projecting to other components of the neural circuits implicated in the regulation of anxiety-related behavior (particularly those neurons with collateral projections to the prefrontal cortex and nucleus accumbens) reveals that these neurons are concentrated within the dorsomedial part of the mid-rostrocaudal dorsal raphe nucleus ().
Figure 2 Labeling of dorsal raphe neurons following injection of the retrograde tracer FluoroGold into the anterior part of the rat basolateral amygdala. (a) illustration of the distribution of retrogradely labeled cells within the dorsal raphe nucleus (coronal sections). Box in -8.00 mm illustration indicates the region illustrated at higher magnification in the photograph in (c). (b) photomicrograph illustrating the injection site restricted to the anterior part of the basolateral amygdaloid nucleus (-3.30 mm Bregma). (c) photomicrograph illustrating dorsal raphe neurons retrogradely labeled with FluoroGold. Retrogradely labeled dorsal raphe neurons were concentrated in the dorsal part of the mid-rostrocaudal dorsal raphe nucleus [gray shading in panel (a), levels -8.00 and -8.24 mm]. Numbers below illustrations in panel (a) indicate stereotaxic coordinates with reference to Bregma. Arrows in (c) indicate FluoroGold labeled neurons in the dorsal raphe nucleus. Abbreviations: BLA, basolateral amygdaloid nucleus, anterior part; BLP, basolateral amygdaloid nucleus, posterior part; BLV, basolateral amygdaloid nucleus, ventral part; BMP, basomedial amygdaloid nucleus, posterior part; CeC, central amygdaloid nucleus, capsular part; CeL, central amygdaloid nucleus, lateral division; LaDL, lateral amygdaloid nucleus, dorsolateral part; LaVL, lateral amygdaloid nucleus, ventrolateral part; LaVM, lateral amygdaloid nucleus, ventromedial part. Scale bar, (a) 873 μm, (b) 800 μm, (c) 50 μm.
![Figure 2 Labeling of dorsal raphe neurons following injection of the retrograde tracer FluoroGold into the anterior part of the rat basolateral amygdala. (a) illustration of the distribution of retrogradely labeled cells within the dorsal raphe nucleus (coronal sections). Box in -8.00 mm illustration indicates the region illustrated at higher magnification in the photograph in (c). (b) photomicrograph illustrating the injection site restricted to the anterior part of the basolateral amygdaloid nucleus (-3.30 mm Bregma). (c) photomicrograph illustrating dorsal raphe neurons retrogradely labeled with FluoroGold. Retrogradely labeled dorsal raphe neurons were concentrated in the dorsal part of the mid-rostrocaudal dorsal raphe nucleus [gray shading in panel (a), levels -8.00 and -8.24 mm]. Numbers below illustrations in panel (a) indicate stereotaxic coordinates with reference to Bregma. Arrows in (c) indicate FluoroGold labeled neurons in the dorsal raphe nucleus. Abbreviations: BLA, basolateral amygdaloid nucleus, anterior part; BLP, basolateral amygdaloid nucleus, posterior part; BLV, basolateral amygdaloid nucleus, ventral part; BMP, basomedial amygdaloid nucleus, posterior part; CeC, central amygdaloid nucleus, capsular part; CeL, central amygdaloid nucleus, lateral division; LaDL, lateral amygdaloid nucleus, dorsolateral part; LaVL, lateral amygdaloid nucleus, ventrolateral part; LaVM, lateral amygdaloid nucleus, ventromedial part. Scale bar, (a) 873 μm, (b) 800 μm, (c) 50 μm.](/cms/asset/0ddb3bf2-c0c6-4915-8336-5f0dfa61f64e/ists_a_149261_f0002_b.jpg)
Figure 3 Diagrammatic illustration of the distribution of dorsal raphe neurons projecting to anxiety circuits. (a) illustration (coronal sections) of the location of injections of retrograde tracer into the prelimbic (PrL) and infralimbic (IL) parts of the medial prefrontal cortex (mPFC, left), nucleus accumbens (middle), and the basolateral amygdala (right). (b) illustration of the distribution of retrogradely labeled neurons in the dorsal raphe nucleus. Open circles represent single-labeled neurons following injections of retrograde tracer in the mPFC; open triangles represent single-labeled neurons following injections of retrograde tracer in the nucleus accumbens (AcbC and AcbSh regions); grey squares represent double-labeled neurons following injections of retrograde tracers into the mPFC and nucleus accumbens; black circles represent single-labeled neurons following injections of retrograde tracer in the basolateral amygdala. The dorsal part of the mid-rostrocaudal dorsal raphe nucleus (gray shading) contains concentrations of neurons with collateral projections to both the mPFC and nucleus accumbens (grey squares), and neurons projecting to the basolateral amygdala (black circles). Abbreviations: ac, anterior commissure; AOP, anterior olfactory nucleus, posterior part; BL, basolateral amygdala; AcbC, nucleus accumbens, core; AcbSh, nucleus accumbens, shell; CPu, caudate putamen; f, fornix; mPFC, medial prefrontal cortex; LV, lateral ventricle; LS, lateral septum; mt, mammillothalamic tract; opt, optic tract. Scale bar, (a) 264 μm, (b) 1 mm. Adapted from Van Bockstaele et al. Citation1993 and Abrams et al. Citation2005, with permission.
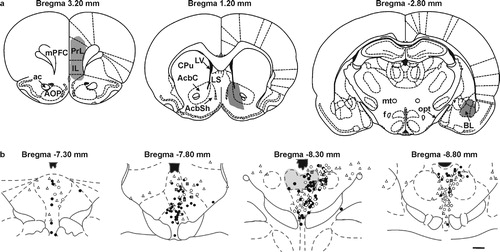
Based on the knowledge that there is a topographical organization throughout the rostrocaudal axis of the dorsal raphe nucleus, we predicted that anxiety-related stimuli may have selective actions on topographically organized subsets of serotonergic neurons in regions of the dorsal raphe nucleus with projections to anxiety circuits. We investigated the effects of different anxiety-related stimuli on the expression of Fos throughout the dorsal raphe nucleus (Abrams et al. Citation2005; Staub et al. Citation2005). Increased behavioral arousal and vigilance behaviors following treatment with different anxiogenic drugs including the adenosine receptor antagonist caffeine, the serotonin 5-HT2A/2C receptor agonist m-chlorophenyl piperazine (mCPP), and the benzodiazepine receptor partial inverse agonist N-methyl-beta-carboline-3-carboxamide (FG-7142) were associated with increases in Fos expression in the dorsal part of the mid-rostrocaudal and caudal dorsal raphe nucleus but not other areas of the dorsal raphe nucleus studied (Abrams et al. Citation2005). Likewise, treatment with the anxiety-related neuropeptide, urocortin 2 (a selective CRF type 2 receptor agonist) (Staub et al. Citation2005), or exposure to social defeat (Gardner et al. Citation2005), also selectively increased Fos expression in the same region (Staub et al. Citation2005). It should be pointed out that the studies using anxiogenic drugs did not verify that the drug-induced increases in Fos expression in brainstem serotonergic neurons were associated with increases in anxiety-related behaviors in validated behavioral tests of anxiety. Therefore, it is uncertain if the anxiogenic drug-induced increases in Fos expression are associated with (1) the performance of anxiety-related behaviors, (2) the development of an anxiety-like emotional state (see below), or (3) physiological or behavioral responses unrelated to the performance of anxiety-related behaviors or the development of a drug-induced anxiety-like emotional state. Future studies should be able to resolve these issues.
The dorsal part of the mid-rostrocaudal dorsal raphe nucleus
Although the neural mechanisms underlying this anatomical specificity are not clear, retrograde tracing studies have revealed that the dorsal part of the mid-rostrocaudal dorsal raphe nucleus is neurochemically distinct (Commons et al. Citation2003) and receives unique afferent input (Peyron et al. Citation1998). Following retrograde tracer injections in the dorsal part of the mid-rostrocaudal dorsal raphe nucleus, large numbers of retrogradely-labelled cells were seen in the lateral, ventral and medial parts of the BNST, whereas only a small to moderate number was visualized after injections in the other dorsal raphe subdivisions. Several lines of evidence suggest that the bed nucleus of the stria terminalis plays an important role in mediating anxiety-related behavior (Davis Citation1998; Gewirtz et al. Citation1998; Walker et al. Citation2003; Levita et al. Citation2004) and therefore the very specific afferents from the BNST to the dorsal part of the mid-rostrocaudal dorsal raphe region may account for the topographical specificity of the effects of anxiety-related drugs, the anxiety-related neuropeptide urocortin 2, or social defeat on Fos expression in serotonergic neurons within this region. Although, it is not possible, using Fos immunohistochemical methods, to establish cause and effect relationships, several lines of evidence suggest that serotonergic neurons within the dorsal part of the mid-rostrocaudal and caudal dorsal raphe may play an important role in the modulation of anxiety states.
Experimental models of anxiety states
As defined above, anxiety is a complex emotional state associated with sustained heightened autonomic and behavioral arousal and a sustained, trans-situational increase in avoidance behavior, often in environmental situations where a conflict between approach and avoidance exists. Although anxiety-related behavior is an innate behavioral response, a number of stimuli have been identified that result in sustained, trans-situational increases in avoidance behaviors. These include a number of pharmacologically distinct anxiogenic drugs, anxiogenic neuropeptides, aversive stimuli such as bright light, and inescapable shock. All of these stimuli have been shown to increase anxiety-related behavior in diverse environmental contexts and therefore, are thought to induce a generalized anxiety state as opposed to specific anxiety-related behaviors. It should be emphasized that although much of what we know about neural mechanisms underlying anxiety-related behavior is based on animal models involving conflict between approach and avoidance behaviors [e.g. (Millan Citation2003)], changes in anxiety states are likely to result in changes in many other physiological and behavioral responses. The distinct temporal profiles of different anxiety-related states and the potential role of serotonergic systems in the development of these anxiety states are outlined below.
Models of anxiety states with distinct temporal profiles
An important component of anxiety states is that the sustained increases in anxiety-related physiological and behavioral responses are not tied to specific environmental cues but are trans-situational, meaning that they can be elicited under diverse environmental contexts. Several models of anxiety states with very distinct temporal profiles have been developed, suggesting that the mechanisms underlying the development and maintenance of these anxiety states may be different. For example, bright light, which is aversive to rats and increases anxiety-related behaviors in a variety of experimental contexts (Valle Citation1970; Cunha and Masur Citation1978; Hall et al. Citation2000), also increases the magnitude of startle responses following exposure of rats to startle-eliciting noise bursts [reviewed in Walker et al. (Citation2003)]. This effect is disrupted by both benzodiazepine and non-benzodiazepine anxiolytics (Walker and Davis Citation1997; de Jongh R. et al. Citation2002; Walker and Davis Citation2002), supporting the possibility that the effects of bright light on startle responses are due to development of an anxiety state. The effect of bright light takes several minutes to develop in order to evoke a maximal response and, following a 20 min exposure to bright light, is present for at least another 20 min (Walker et al. Citation2003). Thus, as pointed out by Walker and colleagues, light-enhanced startle requires a long-duration stimulus and the effect of the stimulus outlasts its duration. The behavioral effects of the stress and anxiety-related neuropeptide CRF on startle responses and other anxiety-related behaviors develop within 30 min and persist for several hours (Dunn and Berridge Citation1990; Walker et al. Citation2003), a similar time course compared to several anxiogenic drugs (Millan Citation2003). In contrast, exposure to inescapable stress appears to result in an anxiety-like emotional state that lasts for a period of approximately 24-48 h (Maier and Watkins Citation2005), while other experimental models of anxiety are associated with increased anxiety-related behavioral responses that are sustained for several weeks (Gewirtz et al. Citation1998; Sajdyk and Shekhar Citation2000; Sajdyk et al. Citation2004). The different time courses involved in these different animal models of anxiety states suggest that they are associated with different underlying neural mechanisms. There is evidence supporting the hypothesis that at least some of these anxiety-related states are dependent on activation of serotonergic systems in the dorsal raphe nucleus.
A role for serotonergic systems in the development of anxiety states
Currently, the most convincing evidence for a role for serotonergic systems in the development and maintenance of anxiety states is based on studies involving the administration of anxiogenic drugs (see discussion above) and exposure of rats to inescapable stress. Exposure of rats to inescapable stress results in a constellation of behavioral consequences 24–48 h later, including increases in anxiety-related behavior in the social interaction and circular elevated maze tests of anxiety [reviewed in Maier and Watkins (Citation2005)]. Several lines of evidence suggest that the anxiety state that follows exposure to inescapable stress is dependent on activation of serotonergic neurons within the dorsal raphe nucleus, and these arguments have been outlined recently in an excellent review by Maier and Watkins (Citation2005). Determining the role of serotonergic systems in the development and maintenance of chronic anxiety states with different temporal profiles is an important objective for future studies.
Summary and future directions
Although a clear understanding of the neural mechanisms underlying anxiety states and the essential stimulus parameters for the development of anxiety states have proven elusive, evidence suggests that elements of uncertainty, unpredictability, or uncontrollability play a central role (Walker et al. Citation2003; Herry et al. Citation2005; Maier and Watkins Citation2005). Interestingly, a neural circuit including the basolateral amygdala, mPFC, and dorsal raphe may play a central role in detecting unpredictability or uncontrollability as a stimulus parameter in rodents and humans (Herry et al. Citation2005; Amat et al. Citation2005; Maier and Watkins Citation2005). Evidence suggests that serotonergic neurons within the dorsal part of the mid-rostrocaudal and caudal dorsal raphe nucleus are selectively activated by a variety of anxiety-related stimuli. This region of the dorsal raphe nucleus, compared to other regions of the dorsal raphe nucleus, is neurochemically distinct and receives unique afferent input from the BNST, a structure that has been implicated in the regulation of anxeity-related behaviour. This region of the dorsal raphe nucleus in turn gives rise to collateral projections to multiple nodes within a distributed neural system modulating anxiety-related behaviors including the basolateral amygdala and mPFC. These findings suggest that different subsets of serotonergic neurons may differentially influence neural processes underlying the initiation, maintenance, or termination of anxiety-related behaviors and anxiety states. In future studies it will be important to determine which subpopulations of serotonergic neurons within the dorsal part of the mid-rostrocaudal and caudal dorsal raphe are affected by anxiogenic drugs or anxiogenic stimuli (e.g. identified based on their projections to other brain regions, or their unique molecular, morphological, or physiological properties). It will also be important to determine the specific roles that these serotonergic neurons play in the modulation of anxiety states, and the time course of these effects. Identification of the molecular, cellular, and functional properties of different subsets of serotonergic neurons may lead to a better understanding of the role of serotonergic systems in modulation of complex emotional states including anxiety.
Acknowledgements
We are grateful to Dr Miles Orchinik, Dr Katharine Semsar, Dr Paul Gasser, Andrew Evans, and Daniel Staub for critical comments on this manuscript. This work was supported by NIMH RO1 MH065702 and RO1 MH52619 to A.S.; C. A. Lowry is a Wellcome Trust Research Fellow (RCDF 068558/Z/02/Z).
References
- Abrams JK, Johnson PL, Hay-Schmidt A, Mikkelsen JD, Shekhar A, Lowry CA. Serotonergic systems associated with arousal and vigilance behaviors following administration of anxiogenic drugs. Neuroscience 2005; 133: 983–997
- Adamec R, Shallow T, Burton P. Anxiolytic and anxiogenic effects of kindling–role of baseline anxiety and anatomical location of the kindling electrode in response to kindling of the right and left basolateral amygdala. Behav Brain Res 2005; 159: 73–88
- Amat J, Baratta MV, Paul E, Bland ST, Watkins LR, Maier SF. Medial prefrontal cortex determines how stressor controllability affects behavior and dorsal raphe nucleus. Nat Neurosci 2005; 8: 365–371
- Amat J, Tamblyn JP, Paul ED, Bland ST, Amat P, Foster AC, Watkins LR, Maier SF. Microinjection of urocortin 2 into the dorsal raphe nucleus activates serotonergic neurons and increases extracellular serotonin in the basolateral amygdala. Neuroscience 2004; 129: 509–519
- Beekman M, Flachskamm C, Linthorst AC. Effects of exposure to a predator on behaviour and serotonergic neurotransmission in different brain regions of C57bl/6N mice. Eur J Neurosci 2005; 21: 2825–2836
- Bell CJ, Nutt DJ. Serotonin and panic. Br J Psychiatry 1998; 172: 465–471
- Berretta S, Pantazopoulos H, Caldera M, Pantazopoulos P, Pare D. Infralimbic cortex activation increases c-fos expression in intercalated neurons of the amygdala. Neuroscience 2005; 132: 943–953
- Berridge KC. Motivation concepts in behavioral neuroscience. Physiol Behav 2004; 81: 179–209
- Berridge KC, Robinson TE. What is the role of dopamine in reward: Hedonic impact, reward learning, or incentive salience?. Brain Res Rev 1998; 28: 309–369
- Blackmore CG, Varro A, Dimaline R, Bishop L, Gallacher DV, Dockray GJ. Measurement of secretory vesicle pH reveals intravesicular alkalinization by vesicular monoamine transporter type 2 resulting in inhibition of prohormone cleavage. J Physiol 2001; 531: 605–617
- Blanchard RJ, Blanchard DC. Attack and defense in rodents as ethoexperimental models for the study of emotion. Prog Neuropsychopharmacol Biol Psychiatry 1989; 13 Suppl: S3–14
- Blundell P, Hall G, Killcross S. Preserved sensitivity to outcome value after lesions of the basolateral amygdala. J Neurosci 2003; 23: 7702–7709
- Campbell BM, Merchant KM. Serotonin 2C receptors within the basolateral amygdala induce acute fear-like responses in an open-field environment. Brain Res 2003; 993: 1–9
- Campeau S, Davis M. Involvement of the central nucleus and basolateral complex of the amygdala in fear conditioning measured with fear-potentiated startle in rats trained concurrently with auditory and visual conditioned stimuli. J Neurosci 1995; 15: 2301–2311
- Chen A, Hough CJ, Li H. Serotonin type II receptor activation facilitates synaptic plasticity via N-methyl-d-aspartate-mediated mechanism in the rat basolateral amygdala. Neuroscience 2003; 119: 53–63
- Commons KG, Connolley KR, Valentino RJ. A neurochemically distinct dorsal raphe-limbic circuit with a potential role in affective disorders. Neuropsychopharmacology 2003; 28: 206–215
- Cunha JM, Masur J. Evaluation of psychotropic drugs with a modified open field test. Pharmacology 1978; 16: 259–267
- Daugherty WP, Corley KC, Phan TH, Boadle-Biber MC. Further studies on the activation of rat median raphe serotonergic neurons by inescapable sound stress. Brain Res 2001; 923: 103–111
- Davis M. Are different parts of the extended amygdala involved in fear versus anxiety?. Biol Psychiatr 1998; 44: 1239–1247
- Davis M. Neurobiology of fear responses: The role of the amygdala. J Neuropsychiatry Clin Neurosci 1997; 9: 382–402
- Day HE, Masini CV, Campeau S. The pattern of brain c-fos mRNA induced by a component of fox odor, 2,5-dihydro-2,4,5-trimethylthiazoline (TMT), in rats, suggests both systemic and processive stress characteristics. Brain Res 2004; 1025: 139–151
- Dayan P, Balleine BW. Reward, motivation, and reinforcement learning. Neuron 2002; 36: 285–298
- de Jongh R, Groenink L, van der GJ, Olivier B. The light-enhanced startle paradigm as a putative animal model for anxiety: Effects of chlordiazepoxide, flesinoxan and fluvoxamine. Psychopharmacology (Berl) 2002; 159: 176–180
- Dong HW, Petrovich GD, Swanson LW. Topography of projections from amygdala to bed nuclei of the stria terminalis. Brain Res Rev 2001; 38: 192–246
- Duncan GE, Knapp DJ, Breese GR. Neuroanatomical characterization of Fos induction in rat behavioral models of anxiety. Brain Res 1996; 713: 79–91
- Dunn AJ, Berridge CW. Physiological and behavioral responses to corticotropin-releasing factor administration: Is CRF a mediator of anxiety or stress responses?. Brain Res Rev 1990; 15: 71–100
- Etkin A, Klemenhagen KC, Dudman JT, Rogan MT, Hen R, Kandel ER, Hirsch J. Individual differences in trait anxiety predict the response of the basolateral amygdala to unconsciously processed fearful faces. Neuron 2004; 44: 1043–1055
- Fendt M, Endres T, Apfelbach R. Temporary inactivation of the bed nucleus of the stria terminalis but not of the amygdala blocks freezing induced by trimethylthiazoline, a component of fox feces. J Neurosci 2003; 23: 23–28
- Ferbinteanu J, Shapiro ML. Prospective and retrospective memory coding in the hippocampus. Neuron 2003; 40: 1227–1239
- Ferry B, Roozendaal B, McGaugh JL. Basolateral amygdala noradrenergic influences on memory storage are mediated by an interaction between beta- and alpha1-adrenoceptors. J Neurosci 1999; 19: 5119–5123
- Fornal CA, Metzler CW, Marrosu F, Ribiero-do-Valle LE, Jacobs BL. A subgroup of dorsal raphe serotonergic neurons in the cat is strongly activated during oral-buccal movements. Brain Res 1996; 716: 123–133
- Gardner KL, Thrivikraman KV, Lightman SL, Plotsky PM, Lowry CA. Early life experience alters behavior during social defeat: Focus on serotonergic systems. Neuroscience 2005; 136: 181–191
- Gewirtz JC, McNish KA, Davis M. Lesions of the bed nucleus of the stria terminalis block sensitization of the acoustic startle reflex produced by repeated stress, but not fear-potentiated startle. Prog Neuropsychopharmacol Biol Psychiatry 1998; 22: 625–648
- Gonzalez LE, Andrews N, File SE. 5-HT1A and benzodiazepine receptors in the basolateral amygdala modulate anxiety in the social interaction test, but not in the elevated plus-maze. Brain Res 1996; 732: 145–153
- Gonzalez LE, Quinonez B, Rangel A, Pino S, Hernandez L. Tonic and phasic alteration in amygdala 5-HT, glutamate and GABA transmission after prefrontal cortex damage in rats. Brain Res 2004; 1005: 154–163
- Goosens KA, Maren S. Pretraining NMDA receptor blockade in the basolateral complex, but not the central nucleus, of the amygdala prevents savings of conditional fear. Behav Neurosci 2003; 117: 738–750
- Graeff FG. Brain defense systems and anxiety. Handbook of Anxiety, M Roth, GD Burrows, R Noyes. Elsevier, Amsterdam 1990; 3: 307–354
- Graeff FG. On serotonin and experimental anxiety. Psychopharmacology (Berl) 2002; 163: 467–476
- Graeff FG, Guimaraes FS, De Andrade TG, Deakin JF. Role of 5-HT in stress, anxiety, and depression. Pharmacol Biochem Behav 1996; 54: 129–141
- Graeff FG, Netto CF, Zangrossi H, Jr. The elevated T-maze as an experimental model of anxiety. Neurosci Biobehav Rev 1998; 23: 237–246
- Graeff FG, Viana MB, Mora PO. Dual role of 5-HT in defense and anxiety. Neurosci Biobehav Rev 1997; 21: 791–799
- Gray JA. A theory of anxiety: The role of the limbic system. Encephale 1983; 9: 161B–166B
- Gray JA. The neuropsychology of anxiety: An enquiry into the functions of the septo-hippocampal system. Clarendon Press, Oxford 1982
- Gray JA, McNaughton N. The neuropsychology of anxiety: Reprise. Perspectives on Anxiety, Panic, and Fear, RA Dienstbier, DA Hope. University of Nebraska Press, Lincoln, Nebraska 1987; 61–134
- Gray JA, McNaughton N. The Neuropsychology of Anxiety, Second Edition. Oxford Medical Publications, Oxford 2000; 1–424
- Gray TS, Bingaman EW. The amygdala: Corticotropin-releasing factor, steroids, and stress. Crit Rev Neurobiol 1996; 10: 155–168
- Griebel G. 5-Hydroxytryptamine-interacting drugs in animal models of anxiety disorders: More than 30 years of research. Pharmacol Ther 1995; 65: 319–395
- Hajos M, Richards CD, Szekely AD, Sharp T. An electrophysiological and neuroanatomical study of the medial prefrontal cortical projection to the midbrain raphe nuclei in the rat. Neuroscience 1998; 87: 95–108
- Hall FS, Huang S, Fong GW, Sundstrom JM, Pert A. Differential basis of strain and rearing effects on open-field behavior in Fawn Hooded and Wistar rats. Physiol Behav 2000; 71: 525–532
- Hammack SE, Schmid MJ, LoPresti ML, Der-Avakian A, Pellymounter MA, Foster AC, Watkins LR, Maier SF. Corticotropin releasing hormone type 2 receptors in the dorsal raphe nucleus mediate the behavioral consequences of uncontrollable stress. J Neurosci 2003; 23: 1019–1025
- Handley SL. 5-Hydroxytryptamine pathways in anxiety and its treatment. Pharmacol Ther 1995; 66: 103–148
- Heilig M, Koob GF, Ekman R, Britton KT. Corticotropin-releasing factor and neuropeptide Y: Role in emotional integration. Trends Neurosci 1994; 17: 80–85
- Heimer L. A new anatomical framework for neuropsychiatric disorders and drug abuse. Am J Psychiatry 2003; 160: 1726–1739
- Herry C, Bach D, Scheffler K, Seifritz E, Luthi A. The amygdala as a detector of unpredictability in mice and humans. 2005 Abstract Viewer/Itinerary Planner. Washington, DC 2005, Online. 416.1. 2005
- Higgins GA, Bradbury AJ, Jones BJ, Oakley NR. Behavioural and biochemical consequences following activation of 5HT1-like and GABA receptors in the dorsal raphe nucleus of the rat. Neuropharmacology 1988; 27: 993–1001
- Higgins GA, Jones BJ, Oakley NR. Effect of 5-HT1A receptor agonists in two models of anxiety after dorsal raphe injection. Psychopharmacology (Berl) 1992; 106: 261–267
- Holstege G. The basic, somatic, and emotional components of the motor system in mammals. The Rat Nervous System, G Paxinos. Academic Press, San Diego 1995; 137–154
- Imai H, Steindler da, Kitai ST. The organization of divergent axonal projections from the midbrain raphe nuclei in the rat. J Comp Neurol 1986; 243: 363–380
- Insel TR, Volkow ND, Landis SC, Li TK, Battey JF, Sieving P. Limits to growth: Why neuroscience needs large-scale science. Nat Neurosci 2004; 7: 426–427
- Insel TR, Volkow ND, Li TK, Battey JF, Jr, Landis SC. Neuroscience networks: Data-sharing in an information age. PLoS Biol 2003; 1: E17
- Iversen SD. 5-HT and anxiety. Neuropharmacology 1984; 23: 1553–1560
- Jackson ME, Moghaddam B. Stimulus-specific plasticity of prefrontal cortex dopamine neurotransmission. J Neurochem 2004; 88: 1327–1334
- Jeffery KJ. Remembrance of futures past. Trends Cogn Sci 2004; 8: 197–199
- Kahn RS, van Praag HM, Wetzler S, Asnis GM, Barr G. Serotonin and anxiety revisited. Biol Psychiatry 1988; 23: 189–208
- Kalivas PW, Nakamura M. Neural systems for behavioral activation and reward. Curr Opin Neurobiol 1999; 9: 223–227
- Kelley AE. Memory and addiction: Shared neural circuitry and molecular mechanisms. Neuron 2004; 44: 161–179
- Killcross S, Robbins TW, Everitt BJ. Different types of fear-conditioned behaviour mediated by separate nuclei within amygdala. Nature 1997; 388: 377–380
- Köhler C, Chan-Palay V, Haglund L, Steinbusch H. Immunohistochemical localization of serotonin nerve terminals in the lateral entorhinal cortex of the rat: Demonstration of two separate patterns of innervation from the midbrain raphe. Anat Embryol (Berl) 1980; 160: 121–129
- Köhler C, Chan-Palay V, Steinbusch H. The distribution and origin of serotonin-containing fibers in the septal area: A combined immunohistochemical and fluorescent retrograde tracing study in the rat. J Comp Neurol 1982; 209: 91–111
- Köhler C, Steinbusch H. Identification of serotonin and non-serotonin-containing neurons of the mid-brain raphe projecting to the entorhinal area and the hippocampal formation A combined immunohistochemical and fluorescent retrograde tracing study in the rat brain. Neuroscience 1982; 7: 951–975
- Kovacs KJ. c-Fos as a transcription factor: A stressful (re)view from a functional map. Neurochem Int 1998; 33: 287–297
- Lacroix L, Spinelli S, Heidbreder CA, Feldon J. Differential role of the medial and lateral prefrontal cortices in fear and anxiety. Behav Neurosci 2000; 114: 1119–1130
- LaLumiere RT, Buen TV, McGaugh JL. Post-training intra-basolateral amygdala infusions of norepinephrine enhance consolidation of memory for contextual fear conditioning. J Neurosci 2003; 23: 6754–6758
- Ledford CC, Fuchs RA, See RE. Potentiated reinstatement of cocaine-seeking behavior following D-amphetamine infusion into the basolateral amygdala. Neuropsychopharmacology 2003; 28: 1721–1729
- LeDoux JE, Cicchetti P, Xagoraris A, Romanski LM. The lateral amygdaloid nucleus: Sensory interface of the amygdala in fear conditioning. J Neurosci 1990; 10: 1062–1069
- Lee Y, Schulkin J, Davis M. Effect of corticosterone on the enhancement of the acoustic startle reflex by corticotropin releasing factor (CRF). Brain Res 1994; 666: 93–98
- Levita L, Hammack SE, Mania I, Li XY, Davis M, Rainnie DG. 5-hydroxytryptamine1A-like receptor activation in the bed nucleus of the stria terminalis: Electrophysiological and behavioral studies. Neuroscience 2004; 128: 583–596
- Li YQ, Kaneko T, Mizuno N. Collateral projections of nucleus raphe dorsalis neurones to the caudate-putamen and region around the nucleus raphe magnus and nucleus reticularis gigantocellularis pars alpha in the rat. Neurosci Lett 2001; 299: 33–36
- Lista A, Blier P, de Montigny C. Benzodiazepine receptors modulate 5-hydroxytryptamine neurotransmission in the rat hippocampus: In vivo electrophysiological evidence. J Pharmacol Exp Ther 1990; 254: 318–323
- Lowry CA. Functional subsets of serotonergic neurones: Implications for control of the hypothalamic-pituitary-adrenal axis. J Neuroendocrinol 2002; 14: 911–923
- Macedo CE, Martinez RC, de Souza Silva MA, Brandao ML. Increases in extracellular levels of 5-HT and dopamine in the basolateral, but not in the central, nucleus of amygdala induced by aversive stimulation of the inferior colliculus. Eur J Neurosci 2005; 21: 1131–1138
- Maier SF, Busch CR, Maswood S, Grahn RE, Watkins LR. The dorsal raphe nucleus is a site of action mediating the behavioral effects of the benzodiazepine receptor inverse agonist DMCM. Behav Neurosci 1995; 109: 759–766
- Maier SF, Watkins LF. Stressor controllability, anxiety, and serotonin. Cognitive Therapy Res 1998; 22: 595–613
- Maier SF, Watkins LR. Stressor controllability and learned helplessness: The roles of the dorsal raphe nucleus, serotonin, and corticotropin-releasing factor. Neurosci Biobehav Rev 2005; 29: 829–841
- Mayford M, Bach ME, Huang YY, Wang L, Hawkins RD, Kandel ER. Control of memory formation through regulated expression of a CaMKII transgene. Science 1996; 274: 1678–1683
- McGaugh JL. Memory consolidation and the amygdala: A systems perspective. Trends Neurosci 2002; 25: 456
- Meil WM, See RE. Lesions of the basolateral amygdala abolish the ability of drug associated cues to reinstate responding during withdrawal from self-administered cocaine. Behav Brain Res 1997; 87: 139–148
- Millan MJ. The neurobiology and control of anxious states. Prog Neurobiol 2003; 70: 83–244
- Miserendino MJ, Sananes CB, Melia KR, Davis M. Blocking of acquisition but not expression of conditioned fear-potentiated startle by NMDA antagonists in the amygdala. Nature 1990; 345: 716–718
- Morgan JI, Curran T. Stimulus-transcription coupling in the nervous system: Involvement of the inducible proto-oncogenes fos and jun. Annu Rev Neurosci 1991; 14: 421–451
- Morgane PJ, Galler JR, Mokler DJ. A review of systems and networks of the limbic forebrain/limbic midbrain. Prog Neurobiol 2005; 75: 143–160
- Nutt DJ. Neurobiological mechanisms in generalized anxiety disorder. J Clin Psychiatry 2001; 62(Suppl 11)22–27
- Nutt DJ. Overview of diagnosis and drug treatments of anxiety disorders. CNS Spectr 2005; 10: 49–56
- Nutt DJ, Bell CJ, Malizia AL. Brain mechanisms of social anxiety disorder. J Clin Psychiatry 1998; 59(Suppl 17)4–11
- Nutt DJ, Forshall S, Bell C, Rich A, Sandford J, Nash J, Argyropoulos S. Mechanisms of action of selective serotonin reuptake inhibitors in the treatment of psychiatric disorders. Eur Neuropsychopharmacol 1999; 9(Suppl 3)S81–S86
- Nutt DJ, Glue P, Lawson C. The neurochemistry of anxiety: An update. Prog Neuropsychopharmacol Biol Psychiatry 1990; 14: 737–752
- Orozco-Cabal L, Pollandt S, Liu J, Vergara L, Shinnick-Gallagher P, Gallagher JP. A novel rat medial prefrontal cortical slice preparation to investigate synaptic transmission from amygdala to layer V prelimbic pyramidal neurons. J Neurosci Methods 2005, Sep 7; [Epub ahead of print]
- Ottersen OP. Afferent connections of the amygdaloid complex of the rat with some observations in the cat. III. Afferents from the lower brain stem. J Comp Neurol 1981; 202: 335–356
- Pesold C, Treit D. The central and basolateral amygdala differentially mediate the anxiolytic effects of benzodiazepines. Brain Res 1995; 671: 213–221
- Petrov T, Krukoff TL, Jhamandas JH. The hypothalamic paraventricular and lateral parabrachial nuclei receive collaterals from raphe nucleus neurons: A combined double retrograde and immunocytochemical study. J Comp Neurol 1992; 318: 18–26
- Petrov T, Krukoff TL, Jhamandas JH. Chemically defined collateral projections from the pons to the central nucleus of the amygdala and hypothalamic paraventricular nucleus in the rat. Cell Tissue Res 1994; 277: 289–295
- Peyron C, Petit J-M, Rampon C, Jouvet M, Luppi P-H. Forebrain afferents to the rat dorsal raphe nucleus demonstrated by retrograde and anterograde tracing methods. Neuroscience 1998; 82: 443–468
- Pezawas L, Meyer-Lindenberg A, Drabant EM, Verchinski BA, Munoz KE, Kolachana BS, Egan MF, Mattay VS, Hariri AR, Weinberger DR. 5-HTTLPR polymorphism impacts human cingulate-amygdala interactions: A genetic susceptibility mechanism for depression. Nat Neurosci 2005; 8: 828–834
- Rainnie DG. Serotonergic modulation of neurotransmission in the rat basolateral amygdala. J Neurophysiol 1999; 82: 69–85
- Rainnie DG, Bergeron R, Sajdyk TJ, Patil M, Gehlert DR, Shekhar A. Corticotrophin releasing factor-induced synaptic plasticity in the amygdala translates stress into emotional disorders. J Neurosci 2004; 24: 3471–3479
- Rasmussen K, Heym J, Jacobs BL. Activity of serotonin-containing neurons in nucleus centralis superior of freely moving cats. Exp Neurol 1984; 83: 302–317
- Roesler R, Schroder N, Vianna MR, Quevedo J, Bromberg E, Kapczinski F, Ferreira MB. Differential involvement of hippocampal and amygdalar NMDA receptors in contextual and aversive aspects of inhibitory avoidance memory in rats. Brain Res 2003; 975: 207–213
- Rogan MT, Staubli UV, LeDoux JE. Fear conditioning induces associative long-term potentiation in the amygdala. Nature 1997; 390: 604–607
- Roozendaal B, Brunson KL, Holloway BL, McGaugh JL, Baram TZ. Involvement of stress-released corticotropin-releasing hormone in the basolateral amygdala in regulating memory consolidation. Proc Natl Acad Sci U S A 2002; 99: 13908–13913
- Roozendaal B, de Quervain DJ, Ferry B, Setlow B, McGaugh JL. Basolateral amygdala-nucleus accumbens interactions in mediating glucocorticoid enhancement of memory consolidation. J Neurosci 2001; 21: 2518–2525
- Roozendaal B, Nguyen BT, Power AE, McGaugh JL. Basolateral amygdala noradrenergic influence enables enhancement of memory consolidation induced by hippocampal glucocorticoid receptor activation. Proc Natl Acad Sci USA 1999; 96: 11642–11647
- Rushworth MF, Walton ME, Kennerley SW, Bannerman DM. Action sets and decisions in the medial frontal cortex. Trends Cogn Sci 2004; 8: 410–417
- Sajdyk TJ, Schober DA, Gehlert DR, Shekhar A. Role of corticotropin-releasing factor and urocortin within the basolateral amygdala of rats in anxiety and panic responses. Behav Brain Res 1999; 100: 207–215
- Sajdyk TJ, Shekhar A. Sodium lactate elicits anxiety in rats after repeated GABA receptor blockade in the basolateral amygdala. Eur J Pharmacol 2000; 394: 265–273
- Sajdyk TJ, Shekhar A, Gehlert DR. Interactions between NPY and CRF in the amygdala to regulate emotionality. Neuropeptides 2004; 38: 225–234
- Sakai K, Crochet S. Differentiation of presumed serotonergic dorsal raphe neurons in relation to behavior and wake-sleep states. Neuroscience 2001; 104: 1141–1155
- Sanders SK, Shekhar A. Regulation of anxiety by GABAA receptors in the rat amygdala. Pharmacol Biochem Behav 1995; 52: 701–706
- Schafe GE, Atkins CM, Swank MW, Bauer EP, Sweatt JD, LeDoux JE. Activation of ERK/MAP kinase in the amygdala is required for memory consolidation of pavlovian fear conditioning. J Neurosci 2000; 20: 8177–8187
- Schreiber R, De VJ. Neuronal circuits involved in the anxiolytic effects of the 5-HT1A receptor agonists 8-OH-DPAT ipsapirone and buspirone in the rat. Eur J Pharmacol 1993; 249: 341–351
- Shumyatsky GP, Tsvetkov E, Malleret G, Vronskaya S, Hatton M, Hampton L, Battey JF, Dulac C, Kandel ER, Bolshakov VY. Identification of a signaling network in lateral nucleus of amygdala important for inhibiting memory specifically related to learned fear. Cell 2002; 111: 905–918
- Singewald N, Salchner P, Sharp T. Induction of c-Fos expression in specific areas of the fear circuitry in rat forebrain by anxiogenic drugs. Biol Psychiatry 2003; 53: 275–283
- Singewald N, Sharp T. Neuroanatomical targets of anxiogenic drugs in the hindbrain as revealed by Fos immunocytochemistry. Neuroscience 2000; 98: 759–770
- Sommer W, Moller C, Wiklund L, Thorsell A, Rimondini R, Nissbrandt H, Heilig M. Local 5,7-dihydroxytryptamine lesions of rat amygdala: Release of punished drinking, unaffected plus-maze behavior and ethanol consumption. Neuropsychopharmacology 2001; 24: 430–440
- Staub DR, Spiga F, Lowry CA. Urocortin 2 increases c-Fos expression in topographically organized subpopulations of serotonergic neurons in the rat dorsal raphe nucleus. Brain Res 2005; 1044: 176–189
- Stein C, Davidowa H, Albrecht D. 5-HT(1A) receptor-mediated inhibition and 5-HT(2) as well as 5-HT(3) receptor-mediated excitation in different subdivisions of the rat amygdala. Synapse 2000; 38: 328–337
- Steinbusch HW, Nieuwenhuys R, Verhofstad AA, van der KD. The nucleus raphe dorsalis of the rat and its projection upon the caudatoputamen. A combined cytoarchitectonic, immunohistochemical and retrograde transport study. J Physiol (Paris) 1981; 77: 157–174
- Stutzmann GE, McEwen BS, LeDoux JE. Serotonin modulation of sensory inputs to the lateral amygdala: Dependency on corticosterone. J Neurosci 1998; 18: 9529–9538
- Tronche F, Kellendonk C, Kretz O, Gass P, Anlag K, Orban PC, Bock R, Klein R, Schutz G. Disruption of the glucocorticoid receptor gene in the nervous system results in reduced anxiety. Nat Genet 1999; 23: 99–103
- Valle FP. Effects of strain, sex, and illumination on open-field behavior of rats. Am J Psychol 1970; 83: 103–111
- Van Bockstaele EJ, Biswas A, Pickel VM. Topography of serotonin neurons in the dorsal raphe nucleus that send axon collaterals to the rat prefrontal cortex and nucleus accumbens. Brain Res 1993; 624: 188–198
- van der Kooy D, Hattori T. Dorsal raphe cells with collateral projections to the caudate-putamen and substantia nigra: A fluorescent retrograde double labeling study in the rat. Brain Res 1980; 186: 1–7
- Vinogradova OS. Hippocampus as comparator: Role of the two input and two output systems of the hippocampus in selection and registration of information. Hippocampus 2001; 11: 578–598
- Walker DL, Davis M. Anxiogenic effects of high illumination levels assessed with the acoustic startle response in rats. Biol Psychiatry 1997; 42: 461–471
- Walker DL, Davis M. Light-enhanced startle: Further pharmacological and behavioral characterization. Psychopharmacology (Berl) 2002; 159: 304–310
- Walker DL, Toufexis DJ, Davis M. Role of the bed nucleus of the stria terminalis versus the amygdala in fear, stress, and anxiety. Eur J Pharmacol 2003; 463: 199–216
- Wang RY, Aghajanian GK. Inhibition of neurons in the amygdala by dorsal raphe stimulation: Mediation through a direct serotonergic pathway. Brain Res 1977; 120: 85–102
- Wise CD, Berger BD, Stein L. Benzodiazepines: Anxiety-reducing activity by reduction of serotonin turnover in the brain. Science 1972; 177: 180–183
- Zangrossi H, Jr, Viana MB, Graeff FG. Anxiolytic effect of intra-amygdala injection of midazolam and 8-hydroxy-2-(di-n-propylamino)tetralin in the elevated T-maze. Eur J Pharmacol 1999; 369: 267–270
- Zangrossi H, Jr, Viana MB, Zanoveli J, Bueno C, Nogueira RL, Graeff FG. Serotonergic regulation of inhibitory avoidance and one-way escape in the rat elevated T-maze. Neurosci Biobehav Rev 2001; 25: 637–645
- Zhuang X, Gross C, Santarelli L, Compan V, Trillat AC, Hen R. Altered emotional states in knockout mice lacking 5-HT1A or 5-HT1B receptors. Neuropsychopharmacology 1999; 21: 52S–60S