Abstract
Several findings relate the hippocampal formation to the behavioural consequences of stress. It contains a high concentration of corticoid receptors and undergoes plastic modifications, including decreased neurogenesis and cellular remodelling, following stress exposure. Various major neurotransmitter systems in the hippocampus are involved in these effects. Serotonin (5-HT) seems to exert a protective role in the hippocampus and attenuates the behavioural consequences of stress by activating 5-HT1A receptors in this structure. These effects may mediate the therapeutic actions of several antidepressants. The role of noradrenaline is less clear and possibly depends on the specific hippocampal region (dorsal vs. ventral). The deleterious modifications induced in the hippocampus by stress might involve a decrease in neurotrophic factors such as brain derived neurotrophic factor (BDNF) following glutamate N-methyl-d-aspartate (NMDA) receptor activation. In addition to glutamate, nitric oxide (NO) could also be related to these effects. Systemic and intra-hippocampal administration of nitric oxide synthase (NOS) inhibitors attenuates stress-induced behavioural consequences. The challenge for the future will be to integrate results related to these different neurotransmitter systems in a unifying theory about the role of the hippocampus in mood regulation, depressive disorder and antidepressant effects.
Introduction
Several hypotheses about the pathophysiology of depression and other stress-related disorders have been proposed. There is an extensive scientific literature describing the involvement of the hippocampal formation in the neurobiology of such disorders, mainly based on observations that similar neurochemical and morphological changes can be found in the hippocampus of stressed animals and depressed humans, which can be prevented or reversed by repeated antidepressant treatment. Much attention has been focused on hippocampal serotonergic and noradrenergic systems. However, recent pieces of evidence has linked other hippocampal neurotransmitters, such as glutamate and nitric oxide (NO), to the development of stress-induced behavioural consequences. In the present work, we review recent data that reinforces the involvement of the hippocampus in behavioural changes induced by stress and how local transmitter systems may interact to mediate stress adaptation and antidepressant effects.
Stress and coping
Selye (Citation1936) first described that exposure to different noxious stimuli could lead to physiologic responses aimed at allowing adaptation of the organism to the new demands. In order to characterize this phenomenon, Selye coined the term stress to describe a potential or real threat to homeostasis imposed by different noxious stimuli that could lead to several physiological alterations and eventually, death. Selye postulated a three stage stereotyped physiologic response in reaction to a stressor, called the general adaptation syndrome. The first stage is the alarm reaction, in which the adrenal medulla releases epinephrine and the adrenal cortex produces glucocorticoids (GCs) to help to restore homeostasis. Restoration of homeostasis leads to the resistance stage, in which defence and adaptation are sustained. If the stressor persists, the adaptive response ceases and the stage of exhaustion follows, with illness and death being possible consequences. Nowadays, it is well recognized that not only physical and chemical agents can act as stressors but also psychological factors, such as novelty and social problems, can cause significant physiological and behavioural changes.
Bruce McEwen and his research team have reinterpreted Selye's alarm response and proposed a new terminology for linking the protective and damaging effects of the biological responses to stressors (McEwen Citation1998, Citation2005a,Citationb; Goldstein and McEwen Citation2002). They proposed the concept of allostasis to refer to a system by which stability is achieved through change—i.e. adaptation. When one's set points vary beyond the limits of homeostatic mechanisms, these variables are referred as being in allostatic states (altered and sustained activity aimed at integrating energetic and the associated behavioural modifications in response to changing environment and challenges). The cumulative results of an allostatic state would then lead to allostatic load, which also provides, within limits, adaptation. However, if the additional load of unpredictable events in the environment is superimposed, then allostatic load can increase and become allostatic overload. Allostatic overload has no useful purpose and predisposes the individual to disease. Thus, according to McEwen, Selye's alarm response could be reinterpreted as the process leading to adaptation, or allostasis, in which GCs, as well as other mediators, promote adaptation to the stressor. But if the alarm response is sustained, with prolonged hormonal secretion, an allostatic state may ensue leading to allostatic overload, which replaces Seley's stage of exhaustion. In other words, Selye's diseases of adaptation would be the result of the allostatic state leading to allostatic overload and resulting in the exacerbation of pathophysiological changes.
A prominent mechanism by which the brain reacts to stress is by activating the hypothalamic–pituitary–adrenal (HPA) axis (Herman and Cullinan Citation1997; Sapolsky et al. Citation2000; Tsigos and Chrousos Citation2002; Carrasco and Van de Kar Citation2003). Neurons in the paraventricular nucleus of the hypothalamus (PVN) secrete corticotrophin-releasing factor (CRF), which stimulates the synthesis and release of adrenocorticotropin (ACTH) from the anterior pituitary. ACTH then stimulates the synthesis and release of GCs (cortisol in humans and corticosterone in rodents). GCs, in turn, exert effects on metabolism and affect behaviour by acting on two specific receptors (glucocorticoid receptors—GRs and mineralocorticoid receptors—MRs) in different brain regions (Fuxe et al. Citation1996; Carrasco and Van de Kar Citation2003). GCs can also exert inhibitory feedback on the HPA axis activity by acting on its receptors in the PVN, hippocampus and cortex (Herman and Cullinan Citation1997; de Kloet Citation2000; Carrasco and Van de Kar Citation2003). Thus, the stress response, with resultant activation of the HPA axis, is meant to be acute or at least of a limited duration. The time-limited duration of this process renders its accompanying antireproductive, antigrowth, catabolic and immunosuppressive effects, temporarily beneficial rather than damaging, allowing adaptation to occur. In contrast, chronicity of the stress system activation would lead to damage and induce pathological states.
In accordance with this proposal, chronic exposure to uncontrollable stresses and consequently, to high GCs levels, has been related to the aetiology of several diseases, including depression and post-traumatic stress disorder (PTSD) (Baungartner et al. Citation1985; Peeters and Broekkamp Citation1994; Heim et al. Citation2000; Sheline Citation2000; Bonne et al. Citation2004; Charney Citation2004; McEwen Citation2005a). For example, an increased prevalence of life stress episodes before the onset of major depression has been documented, suggesting that they could have a main role in its development (Post Citation1992). In laboratory animals, the exposure to uncontrollable and severe stressful events can induce physiological and behavioural alterations that resemble human depression, such as motor deficits, weight and sleep changes, anhedonia, memory impairments and excessive GC secretion (Willner Citation1986, Citation1990; Willner and Mitchell Citation2002; Anisman and Matheson Citation2005). Therefore, most animal models aimed at studying the neurobiology of stress-related disorders, such as depression, are based on behavioural modification induced by exposure to different stressors that can be prevented or reversed by antidepressant drugs (Willner Citation1986, Citation1990; Willner and Mitchell Citation2002).
HPA axis hyperactivity has been implicated in the initiation and maintenance of some stress-induced alterations that could contribute to depression. This hypothesis is supported by several clinical and experimental findings (Checkley Citation1992; Heim et al. Citation2000; Tsigos and Chrousos Citation2002; Keller et al. Citation2006). Abnormal, excessive activation of HPA axis is observed in depressed individuals (Whiteford et al. Citation1987; Gold et al. Citation1995; Keller et al. Citation2006), who often show increased cortisol production that is unresponsive to the administration of the synthetic GC dexamethasone (Baungartner et al. Citation1985). Moreover, there is a high co-morbidity between depression and Cushing's syndrome, which is a disease characterized by high levels of circulating cortisol (Starkman et al. Citation1981, Citation1986, Citation1992). Consistent with these human data are the observations that rodents reared in isolation or submitted to inescapable shocks show abnormalities in HPA axis function (Heidbreder et al. Citation2000) and that chronic GC treatment can lead to depressive-like behaviour in rats (Johnson et al. Citation2006).
However, the mechanisms that mediate the stress effects on behaviour and mood are not fully understood. It has been suggested that the levels of GCs, particularly if sustained over long periods of time, might be high enough to induce toxic effects in some neuronal circuits, especially those related to mood regulation, such as the hippocampus, cortex and amygdala (Sapolsky Citation2000; Sapolsky et al. Citation2000; Lee et al. Citation2002b,Citationa). Impaired functions in these structures might be expected to contribute to some of the cognitive abnormalities observed in depressed patients.
Stress and the hippocampus
The hippocampus is a brain structure that has been traditionally reported to be involved in spatial learning and in episodic, declarative and contextual memory (Fanselow Citation2000; Riedel and Micheau Citation2001; Knierim Citation2003; Shu et al. Citation2003; Buckley Citation2005; Frankland and Bontempi Citation2005; Moscovitch et al. Citation2005, Citation2006). However, the hippocampus is the brain region showing the highest density of receptors for corticosteroids (McEwen et al. Citation1968; Gerlach and McEwen Citation1975; de Kloet Citation2000) and has been consistently linked to the brain's response to stress and to the regulation of the HPA axis activity (de Kloet Citation2003; Herman et al. Citation2005). It has been proposed to play an important role in coping with threatening stimuli. According to Gray and McNaughton (Citation2000), during threatening or novel situations the hippocampus is involved with the detection and resolution of conflicts between incompatible goals or response tendencies. Specifically, when this type of conflict is detected, the hippocampus outputs a signal that increase the weight or valence of affectively negative information, thereby reducing or inhibiting the tendency to approach the goal. During extinction, the hippocampal output would augment the negative affect produced by nonreward (e.g. frustration). In accordance with this proposal, neuroimaging studies have shown hippocampal/parahippocampal activation during perception of several negatively valenced stimuli and/or experiencing of negatively valenced affective states (Blood et al. Citation1999; Isenberg et al. Citation1999; Mirz et al. Citation2000). In addition, the hippocampus could have an important protective role after repeated stress. An impairment of this adaptive effect induced by severe stress could facilitate the development of behavioural deficits and clinical symptoms (Deakin and Graeff Citation1991; Graeff et al. Citation1996).
Stress effects on the hippocampus seem to be mediated largely by the lower affinity GRs which become heavily occupied with corticosteroids in response to stress (Joels Citation2001; de Kloet Citation2003; de Kloet and Derijk Citation2004). GC initial action on these receptors is involved in terminating the endocrine response to stress by attenuating HPA axis activation (Herman and Cullinan Citation1997; Feldman and Weidenfeld Citation1999; Herman et al. Citation2005) and promoting memory storage in preparation for future events (Oitzl and de Kloet Citation1992; Sandi et al. Citation1997; de Kloet et al. Citation1999; Oitzl et al. Citation2001; Avital et al. Citation2006; Diamond et al. Citation2006). Since corticosteroid receptors function as transcriptional regulators, the first step that leads to their ultimate effect on adaptive behaviour involves the altered expression of responsive genes. In the hippocampus, the activation of MRs or GRs leads to the altered expression of several genes that underlie aspects of cell metabolism, structure and synaptic transmission (Datson et al. Citation2001). Tables and summarize the main findings relating hippocampal gene expression changes, stress and antidepressant treatments.
Table I. Gene expression changes in the hippocampal formation induced by stress.
Table II. Gene expression changes in the hippocampal formation induced by antidepressant treatments.
Although GC-mediated processes are adaptive in nature, repeated exposure to high levels of GCs can be deleterious to the structure and function of the hippocampus. This is supported, for example, by numerous studies that consistently report that stress decreases the proliferation of new neurons in the subgranular zone of the hippocampus. Adult neurogenesis is decreased by many different types of stressors, including predator odour (Tanapat et al. Citation2001), social stress (Gould et al. Citation1997; Czeh et al. Citation2001), acute and chronic restraint stress (Pham et al. Citation2003; Rosenbrock et al. Citation2005), inescapable shocks (Malberg and Duman Citation2003; Vollmayr et al. Citation2003; Bland et al. Citation2006) and chronic mild stress (Alonso et al. Citation2004). These stress-induced effects are thought to be corticosteroid-dependent since administration of corticosterone significantly decreases neurogenesis in the hippocampus (Gould et al. Citation1992; Cameron et al. Citation1998) and the blockade of corticosteroid actions prevents stress-induced decrease in neurogenesis (Cameron and Gould Citation1996; Mayer et al. Citation2006). Moreover, it seems that the suppressive action of GCs on cell proliferation is not direct but occurs through an N-methyl-d-aspartate (NMDA) receptor-dependent excitatory pathway (Gould et al. Citation1997; Cameron et al. Citation1998; Gould and Tanapat Citation1999; Nacher and McEwen Citation2006). For a complete and recent review of the literature on the regulation of neurogenesis by stress, see Dranovsky and Hen (Citation2006), Mirescu and Gould (Citation2006) and Schmidt and Duman (Citation2006).
In addition to a decrease in neurogenesis, stress also influences the structure of mature neurons in the adult hippocampus. Repeated stress exerts effects similar to GCs on dendritic remodelling in CA3. Chronic restraint stress results in reduction in apical dendritic length and branch number in rat CA3 pyramidal neurons (Watanabe et al. Citation1992; Vyas et al. Citation2002). Other repeated stress paradigms, such as repeated variable stress and psychosocial stress (Magariños and McEwen Citation1995a; Magariños et al. Citation1996; Fuchs et al. Citation2001), result in similar dendritic remodelling in the CA3 region. Repeated stress-induced dendritic remodelling may also be mediated by sustained high levels of GCs (Sapolsky et al. Citation1990; Magariños and McEwen Citation1995b; Fuchs and Flugge Citation1998; Fuchs et al. Citation2001) and glutamate (Magariños and McEwen Citation1995b; McEwen Citation1996).
Stress also decreases the expression of the brain derived neurotrophic factor (BDNF, Vaidya et al. Citation1997; Nibuya et al. Citation1999; Rasmussen et al. Citation2002; Murakami et al. Citation2005; Smith Citation2005; see Duman and Monteggia (Citation2006) for a complete review). BDNF is required for and may induce neurogenesis (Lee et al. Citation2002a; Scharfman et al. Citation2005), neuronal survival (Sairanen et al. Citation2005) and neuronal branching (Danzer et al. Citation2002; Govindarajan et al. Citation2006). Moreover, BDNF exerts direct effects on membrane excitability (Schinder and Poo Citation2000). Therefore, it is possible that a stress-induced decrease of BDNF levels is involved in neurogenesis, synaptic morphology and membrane excitability changes, thus leading to modification of hippocampal synaptic transmission, connectivity and function. Stress and adrenal steroids may impair long-term potentiation (LTP) in the rodent hippocampus (Foy et al. Citation1987; Shors et al. Citation1990; Pavlides et al. Citation1993; Diamond and Rose Citation1994; Pavlides et al. Citation2002; Kim et al. Citation2005; Aleisa et al. Citation2006; Kim et al. Citation2006), a synaptic model postulated to be fundamental to memory storage (Lynch Citation2004). On the other hand, stress seems to facilitate hippocampal theta-rhythm (Shors et al. Citation1997; Yamamoto Citation1998), a rhythmic waveform that occurs in alert, immobile rats presented with threatening stimuli, possibly facilitating fear memory acquisition and retrieval (Seager et al. Citation2002; Seindenbecher et al. Citation2003; Vertes Citation2005). In agreement with these electrophysiological data, it is reported from studies employing behavioural models that stress exerts complex effects on memory processing, ranging from impairment of hippocampal-dependent spatial memory to facilitation of fear-related learning and memory (see Kim and Diamond (Citation2002) for review).
These findings have been related to several neuropsychiatric diseases, especially depression and PTSD. In agreement with this hypothesis, several studies have found a significant reduction in hippocampal volume in patients suffering from these disorders (Sheline et al. Citation1996, Citation1999, Citation2003; Stein et al. Citation1997; Bremner et al. Citation2000; Mervaala et al. Citation2000; Steffens et al. Citation2000; Frodl et al. Citation2002; MacQueen et al. Citation2003; Smith Citation2005; Karl et al. Citation2006). Interestingly, Fuchs and co-workers (Ohl et al. Citation1999, Citation2000; Fuchs et al. Citation2001) have shown that chronic psychological stress in primates reduces the proliferation of new cells in the hippocampus, causing a decrease of neuronal function and viability. This was associated with a trend towards a decrease in total hippocampal volume. Again, this seems to be mediated by high corticosteroid levels, since it can also be induced by long-term cortisol exposure (Lupien et al. Citation1998; Ohl et al. Citation1999) and reduced hippocampal volume can be observed in Cushing's patients (Starkman et al. Citation1992). Recent evidence, on the other hand, suggests that in PTSD a small hippocampus probably precedes the symptoms and predicts the susceptibility to develop the disorder after severe stress exposure (Gilbertson et al. Citation2002; Gross and Hen Citation2004). Together, however, these results support the proposal that in depression and PTSD there is an impairment of a stress protective role played by the hippocampus.
The fact that chronic antidepressant treatment is able to attenuate these stress-induced effects strengthens the involvement of hippocampal atrophy in pathophysiology of stress-related disorders (Sheline et al. Citation2003; Bremner Citation2006). Depression, therefore, could be a consequence of stress-induced neuronal death and decreased cellular resilience within the hippocampal circuitry with consequent alteration in information processing. Antidepressants would work by restoring normal connectivity and function in this region (Castrén Citation2005). According to this hypothesis, increased BDNF release would select those neurons and connections that mediate useful neuronal activity to the target neurons and helps to eliminate connections that produce random noise. Therefore, “although antidepressant-induced neurogenesis increases overall activity, only those neurons that best mediate useful neuronal activity to the target cells would be selected and prevail in the competition. Increased granule neuronal turnover produced by antidepressant treatments indicates that there are more competing neurons available for selection, which may improve the ability of the hippocampus to rapidly adapt to emerging environmental challenges” (Sairanen et al. Citation2005). Corroborating this hypothesis, it was shown that behavioural effects of antidepressants depend on intact BDNF signalling (Saarelainen et al. Citation2003) and hippocampal neurogenesis (Santarelli et al. Citation2003). Moreover, hippocampal BDNF administration prevents cognitive impairment induced by stress (Radechi et al. Citation2005) and induces antidepressant-like effects in animal models (Sciuciak et al. Citation1997; Shirayama et al. Citation2002).
In summary, a wealth of evidence indicates that impairment of normal hippocampal functions could lead to stress-related disorders, maybe by impairing protective mechanisms and enhancing the processing of aversive memories, predisposing the individual towards negatively valenced affective states. The increase in cell turnover and connectivity induced by antidepressants would restore hippocampal function.
Stress, serotonin and the hippocampus
Since the discovery that the selective inhibition of serotonin reuptake induces antidepressant effects, several studies have investigated the role of the serotonergic neurotransmission in the neurobiology of depression. Serotonin was shown to be essential to the effects of antidepressant drugs by Delgado et al. (Citation1990). They reported that remitted depressed patients receiving antidepressants experience an acute symptom relapse after a procedure (tryptophan depletion) that decreases serotonin brain levels (Delgado et al. Citation1990; Heninger et al. Citation1996). More recently, it was shown that reducing serotonin synthesis induced depressive-like symptoms in normal subjects submitted to uncontrollable stress (Richell et al. Citation2005). Therefore, it seems that serotonin is not only necessary for the therapeutic effects of antidepressants but it is also required to facilitate resilience to uncontrollable stress. Also, the acute relapse of depressive symptoms induced by serotonin depletion suggests that other mechanisms in addition to increased neurogenesis and cellular resilience are involved in the therapeutic effects of antidepressants.
Deakin and Graeff (Citation1991) proposed that the serotonergic pathways originated in the dorsal raphe nuclei (DRN) regulate adaptive responses aimed at terminating acute stress, such as escape, fight and flight reactions. However, in case of chronic exposure to inescapable aversive stimuli, where coping with stress is needed the pathway connecting the median raphe nucleus (MRN) to the hippocampus would help adaptation to occur by inhibiting the consolidation of stressful memories and thus disconnecting the aversive events from the behavioural outcomes (Deakin and Graeff Citation1991). A failure in this mechanism would favour the development of stress-induced behavioural deficits in animals and depression in humans. Several experimental findings have supported this hypothesis (see Graeff et al. (Citation1996) for review). For example, electrolytic lesions of the MRN significantly enhanced plasma corticosterone levels and incidence of gastric ulcers (Andrade et al. Citation1999) and lesions of serotonin neurons located in the MRN impaired the behavioural adaptation to repeated restraint stress (Netto et al. Citation2002). Moreover, adaptation to chronic mild stress was followed by increased levels of serotonin in the dorsal hippocampus (Storey et al. Citation2006) and post-stress facilitation of hippocampal serotonergic neurotransmission prevented the development of stress-induced behavioural deficits (Guimarães et al. Citation1993; Padovan and Guimarães Citation1993; Joca et al. Citation2003, ), findings which are consistent with the hypothesis that increased serotonin release in the hippocampus may be implicated in the mechanisms underlying coping with inescapable stress.
Figure 1 Post-stress facilitation of serotonin-mediated neurotransmission in the dorsal hippocampus prevents the behavioural consequences of stress. Male Wistar rats (n = 7–23) were submitted to inescapable footshocks (40 shocks, 1 mA, 10 s) or habituation (30 min) in a shuttle box. Immediately afterwards, they received bilateral intra-hippocampal injections of saline (Sal) or zimelidine (Zim, 20 or 100 nmols/0.5 μl), a selective serotonin reuptake inhibitor, and were tested 24 h later wit escapable footshocks (30 sound-signalled shocks, 0.8 mA, 10 s). Data are expressed as the mean ± SEM number of escape and/or avoidance failures in each block (summation of five individual trials). * Indicates significant difference from respective Sal-treated group (p < 0.05, ANOVA followed by Duncan test, modified from Joca et al. (Citation2003), with kind permission from Elsevier).
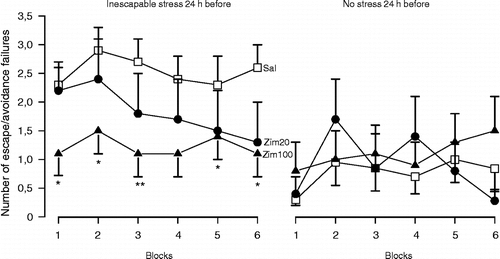
In agreement with these data, it was shown in rodents that behavioural adaptation to repeated restraint stress is followed by serotonergic supersensitivity (Kennett et al. Citation1985a,Citationb) and that post-stress facilitation of serotonergic neurotransmission by 5-HT1A agonist administration is able to prevent the development of stress-induced behavioural consequences (Kennett et al. Citation1987). These results, therefore, suggest that adaptation to stress involves augmentation of 5-HT1A receptor function.
Hippocampal 5-HT1A receptors are likely to be the main target of the MRN-serotonergic pathway to promote stress adaptation. Post-stress microinjections of a selective serotonin reuptake inhibitor (SSRI) or 5-HT1A agonists into the dorsal hippocampus reversed the deficits induced by restraint stress of open arm exploration in an elevated plus-maze (Guimarães et al. Citation1993, Netto and Guimarães Citation1996). More recently, we showed similar effects in the learned helplessness model (Joca and Guimarães Citation2006, ).
The mechanisms by which 5-HT1A receptors mediate adaptation to stress are still unknown. One possible mechanism could be interference with consolidation of stressful memories (Guimarães et al. Citation1993). Activation of 5-HT1A receptors negatively modulates LTP in the hippocampus (Corradetti et al. Citation1992; Sakai and Tanaka Citation1993; Kojima et al. Citation2003; Tachibana et al. Citation2004), a phenomenon traditionally linked to learning and memory (Vertes Citation2005). Consistent with these physiological data, systemic or intra-hippocampal administration of 5-HT1A agonists impaired acquisition and consolidation of spatial and fear-related memories (Carli and Samanin Citation1992; Carli et al. Citation1992a,Citationb; Stiedl et al. Citation2000). Moreover, mice with knockout of 5-HT1A receptors show impaired hippocampal-dependent learning and memory (Sarnyai et al. Citation2000), and their fear response is biased toward threatening cues (Gross et al. Citation2000; Klemenhagen et al. Citation2006). Therefore, activation of hippocampal 5-HT1A receptors could help adaptation to stress by attenuating the emotional impact of aversive stimuli and consequently inhibiting the consolidation of stressful memories. Another possibility is that the activation of 5-HT1A receptors could attenuate hippocampal hyperactivity, which can be observed in stressed rats (Petty and Sherman Citation1981; Shumake et al. Citation2002) and depressed humans (Mayberg et al. Citation2000; Goldapple et al. Citation2004), by attenuating the release of glutamate (Matsuyama et al. Citation1996; Strosznajder et al. Citation1996). Finally, 5-HT1A mediates some trophic actions attributed to serotonin, such as the increase in neurogenesis (Brezun and Daszuta Citation1999; Brezun and Daszuta Citation2000; Radley and Jacobs Citation2002; Banasr et al. Citation2004) and the release of neurotrophic factors (Galter and Unsicker Citation1996). It is possible that these effects could protect hippocampal circuitry from the deleterious effects induced by repeated exposure to stress and GCs.
Under conditions of repeated exposure to severe inescapable stress and high corticosteroid levels 5-HT1A-mediated functions could be impaired, thus predisposing to the development of behavioural deficits. In fact, different kinds of severe stressors can induce down-regulation and desensitization of 5-HT1A receptors within the hippocampus (Flugge Citation1995; Karten et al. Citation1999; van Riedel et al. Citation2003). This seems to be corticoid-dependent, since the administration of corticosterone to rats induces the same effect (Mendelson and McEwen Citation1992; Meijer and de Kloet Citation1994; Beck et al. Citation1996; Neumaier et al. Citation2000; Bijak et al. Citation2001) and removal of the adrenal glands enhances 5-HT1A expression in the hippocampus (Chalmers et al. Citation1993). Besides, MR, GR and 5-HT1A receptors are highly colocalized within the hippocampus (Lopez et al. Citation1998, Lopez et al. Citation1999), which strengthens the possibility that 5-HT1A expression is under the control of GCs. However, altered 5-HT1A function and hypocortisolemia are also observed after repeated exposure to severe stress (Harvey et al. Citation2003, Citation2004a). Harvey and co-workers suggested that this could be the result of a hypersensitive feedback regulation on HPA-axis regulation that would follow the acute hypercortisolemia induced by the first stress exposure. The hypocortisolemia over sustained periods could also contribute to altered 5-HT1A function and abnormal behaviour. This can be of special importance in the neurobiology of PTSD, where hypocortisolemia is a hallmark characteristic of the disorder. The finding that stressed rats show cognitive impairment along with 5-HT1A receptor changes (Harvey et al. Citation2004a) and that chronic antidepressant treatment reverses the stress effects upon 5-HT1A receptors (Bijak et al. Citation2001) further suggest that 5-HT1A-mediated functions are important for normal behaviour and mood.
Several clinical studies suggest an impairment of hippocampal 5-HT1A-mediated neurotransmission in depression. Post-mortem brains of depressed suicides show reduced number of hippocampal post-synaptic 5-HT1 receptors (Cheetham et al. Citation1990) and an increased number of 5-HT inhibitory autoreceptors (Stockmeier et al. Citation1998). In addition, depressed patients have a widespread reduction in 5-HT1A receptor binding measured by positron emission tomography scans with [C]WAY-100635 (Drevets et al. Citation2000; Sargent et al. Citation2000) and show a marked attenuation of prolactin release in response to intravenous tryptophan (Deakin et al. Citation1990). Finally, most effective antidepressants progressively enhance serotonin function in the hippocampus (Blier and De Montigny Citation1994, Haddjeri et al. Citation1998; Blier Citation2003; Castro et al. Citation2003).
Therefore, it can be suggested that severe inescapable stress impairs the hippocampal serotonergic system, particularly 5-HT1A receptors, which would limit adaptation to subsequent aversive stimuli, leading to helpless-like behaviour and depressive symptoms in humans. Chronic antidepressant treatment, by facilitating 5-HT1A-mediated transmission within the hippocampus, would ameliorate these behavioural changes.
Beyond serotonin
In addition to serotonin, several neurotransmitter systems in the hippocampal formation may also participate in stress responses. Ninety per cent of pyramidal and granule cells within the hippocampus are glutamatergic and 10% are GABA-producing interneurons (Vizi and Kiss Citation1998). Moreover, the hippocampal neuropil is enriched by noradrenergic, serotonergic, dopaminergic and cholinergic axon terminals. These systems are afferent pathways originating from the locus coeruleus, raphe nuclei, ventral tegmental area (VTA) and septal nuclei, respectively (reviewed in Vizi and Kiss Citation1998).
Noradrenergic system
The noradrenergic system has long been related to behavioural responses to stress (Leonard Citation2001; Morilak et al. Citation2005) and to the mechanism of action of antidepressant drugs (Heninger et al. Citation1996; Brunello et al. Citation2002; Nutt Citation2006). Noradrenergic neurons are excited in response to stress, leading to enhanced noradrenaline release in several brain regions, including limbic regions thought to be involved in mediating a variety of behavioural, cognitive, affective, autonomic and neuroendocrine responses to stress (Morilak et al. Citation2005).
However, noradrenaline and serotonin may play different roles in the hippocampus. In several behavioural paradigms (open-field, passive avoidance and Geller-Seifter), intra dorsal-hippocampus administration of serotonin or noradrenaline induced opposite behavioural effects (Plaznick et al. 1983). Serotonin and noradrenaline also show differences in the regulation of hippocampal functional activity. While serotonin inhibits theta rhythm (Hajos-Korcsok et al. Citation2000) and LTP (Corradetti et al. Citation1992), noradrenaline seems to facilitate both processes (Katsuki et al. Citation1997; Hajos et al. Citation2003; Almaguer-Melian et al. Citation2005). In this regard, noradrenaline release into the hippocampus in response to aversive stimuli would signal relevant biological outcomes (“alarm-system”), promoting arousal and storage of fear-related memories (Gray and McNaughton Citation2000). Thus, the persistence of this system in the active mode could lead to the development of behavioural disorders, such as anxiety and depression. In accordance with this hypothesis, the anxiogenic drug FG-7142 facilitates noradrenergic transmission in the dorsal hippocampus (Ida et al. Citation1991). Similar effects were reported for animals that showed potentiated behavioural response to stress (Rosario and Abercrombie Citation1999). Moreover, facilitation of noradrenergic neurotransmission within the dorsal hippocampus failed to show antidepressant-like effects and seems to facilitate helpless-like behaviour in non-stressed rats ( and , Joca et al. Citation2006), suggesting that the blockade of the noradrenergic system in this region could prevent the development of stress-induced behavioural deficits. In agreement with this proposal, systemic administration of a β-adrenergic receptor antagonist attenuated the development of PTSD in humans that had recently been exposed to severe trauma (Vaiva et al. Citation2003).
Figure 2 Post-stress facilitation of noradrenaline-mediated neurotransmission in the dorsal hippocampus does not prevent the behavioural consequences of stress. Male Wistar rats (n = 11–14) were submitted to inescapable footshocks (40 shocks, 1 mA, 10 s) or habituation (30 min) in a shuttle box. Immediately afterwards, they received bilateral intra-hippocampal injections of Sal or desipramine (DIM, 3 or 30 nmols/0.5 μl), a selective noradrenaline reuptake inhibitor and were tested 24 h later with escapable footshocks (30 sound-signalled shocks, 0.8 mA, 10 s). Data are expressed as the mean ± SEM number of escape and/or avoidance failures in each block (summation of five individual trials). There was no significant difference between treatments (modified from Joca et al. (Citation2006), with kind permission from Elsevier).
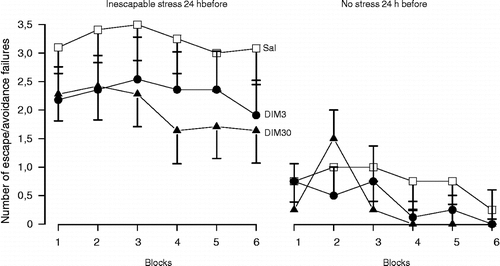
Figure 3 Pre-stress facilitation of noradrenaline-mediated neurotransmission in the dorsal hippocampus also does not prevent the behavioural consequences of stress. However, it facilitates helpless behaviour in control (non-stressed) animals. Male Wistar rats (n = 7–19) received bilateral intra-hippocampal injections of Sal or desipramine (DIM, 30 nmols/0.5 μl) and immediately afterwards were submitted to inescapable footshocks (40 shocks, 1 mA, 10 s) or habituation (30 min) in a shuttle box. All animals were tested 24 h later with escapable footshocks (30 sound-signalled shocks, 0.8 mA, 10 s). Data are expressed as the mean ± SEM number of escape and/or avoidance failures in each block (summation of five individual trials). * Indicates significant difference from respective Sal-treated group (t-test, p < 0.05, (modified from Joca et al. (Citation2006), with kind permission from Elsevier).
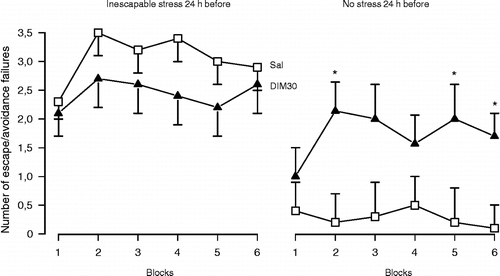
On the other hand, facilitation of noradrenergic neurotransmission within the ventral hippocampus seems to protect against stress effects (Petty and Sherman Citation1980; Sherman and Petty Citation1980) and prevents learned helpless development. Rats with enhanced noradrenaline levels within this region do not develop learned helplessness in response to inescapable footshocks (Petty and Sherman Citation1980; Sherman and Petty Citation1980). Moreover, noradrenaline release is increased in the ventral hippocampus during habituation to repeated stress (Hajos-Korcsok et al. Citation2000).
These data are in agreement with recent evidence indicating the existence of important functional differences between dorsal and ventral regions of the hippocampus, the first being closely related to learning/memory processes while the latter would be more involved in anxiety (Kjelstrup et al. Citation2002; Bannerman et al. Citation2004; Degroot and Treit Citation2004; Bertoglio et al. Citation2006). Thus, it could be speculated that the facilitation of noradrenergic neurotransmission in the ventral hippocampus, but not in the dorsal hippocampus, could attenuate the stress-induced aversive state and, as a consequence, its emotional impact, leading to antidepressant-like effects.
Another possibility to explain the involvement of noradrenergic drugs in stress adaptation would be the facilitation of serotonergic neurotransmission in the hippocampal formation. Mongeau et al. (Citation1997) proposed that the main mechanism of antidepressant treatments in the hippocampus would be to enhance 5-HT1A and/or to reduce β-adrenergic-mediated neurotransmission. Both effects could be produced by antidepressant treatments through parallel or serial mechanisms involving interactions between the serotonergic and noradrenergic systems.
Noradrenaline, therefore, could play a different role in modulating stress responses depending on the hippocampal region. Antidepressant effects induced by noradrenergic drugs could involve either an interference with noradrenergic neurotransmission in the ventral hippocampus or a facilitation of serotonergic neurotransmission in the dorsal hippocampus. Moreover, the behavioural effects of antidepressants may also involve interference with noradrenergic neurotransmission in other brain structures apart from the hippocampal formation such as the amygdala (Strange et al. Citation2003).
Dopaminergic system
Dopamine is another monoamine proposed to play an important role in the regulation of mood and behaviour, particularly in motivated and reward-related behaviour (Koob Citation1996; Berridge and Robinson Citation1998; Hyman et al. Citation2006). It is also implicated in the neurobiology of depression as well as in the mechanism of action of antidepressants (Gambarana et al. Citation1995; D'Aquila et al. Citation2000; Basso et al. Citation2005; Gershon et al. Citation2006; Nestler and Carlezon Citation2006). However, much of the attention aimed at understanding the role of dopamine in the pathophysiology of depression has been directed to the mesolimbic dopamine pathways, where a dopaminergic hypofunction has been related to the decreased motivation and/or anhedonia observed in human depressive states (Nelson and Charney Citation1981; Cabib and Puglisi-Allegra Citation1996; Willner Citation1997; D'Aquila et al. Citation2000; Nestler and Carlezon Citation2006).
Not much is known about the role of hippocampal dopaminergic neurotransmission in the neurobiology of stress-related disorders. Dopamine levels are altered in the hippocampus in response to stress (Vijayakumar and Meti Citation1999; Zangen et al. Citation1999; Harvey et al. Citation2005; Dronjak and Gavrilovic Citation2006). These levels are increased in congenitally “depressive” rats (Zangen et al. Citation1999; Yadid et al. Citation2000) or in rats submitted to an acute exposure to different inescapable stressors (Harvey et al. Citation2005). On the other hand, chronic exposure to stress induces significant reduction of dopamine content in the hippocampus (Sunanda et al. Citation2000; Dronjak and Gavrilovic Citation2006). Chronic antidepressant treatment can counteract the acute neurochemical imbalances induced by stress (Zangen et al. Citation1999; Yadid et al. Citation2000), increasing and decreasing the responsiveness of hippocampal dopamine D2 and D1-like receptors, respectively (Bijak and Smialowski Citation1988; Bijak Citation1993). In accordance with these data, the mRNA for D5 receptors (D1 family) is increased in the hippocampus of patients suffering from unipolar depression (Knable et al. Citation2004)
Several pieces of evidence indicate that the facilitation of dopaminergic neurotransmission induces neuroprotective effects in the hippocampus (Takata et al. Citation2000; Chlan-Fourney et al. Citation2002; Bai et al. Citation2003). Subchronic treatment with D2 and D3 receptor agonists, for example, induces a significant increase in expression of the anti-apoptotic protein Bcl-2 in the hippocampus (Takata et al. Citation2000). Corroborating these results, several groups have shown that chronic treatment with D2 antagonists induces significant decreases in the hippocampal expression of BDNF mRNA and protein (Angelucci et al. Citation2000; Lipska et al. Citation2001; Chlan-Fourney et al. Citation2002; Bai et al. Citation2003) and TrkB (Angelucci et al. Citation2000). These results suggest that the facilitation of dopaminergic neurotransmission within the hippocampus may enhance BDNF expression, which is believed to mediate the behavioural effects of antidepressants (Saarelainen et al. Citation2003). Studies using chronic treatment with preferential inhibitors of dopamine uptake that possess antidepressant properties could test this hypothesis. These data support the involvement of the hippocampal dopaminergic system in the neurobiology of stress-related disorders and in the behavioural effects of antidepressant drugs. However, additional evidence is needed to clarify the role of hippocampal dopaminergic neurotransmission in adaptation to stress and in the mechanism of action of antidepressants. For example, infusion of drugs that modulate dopaminergic neurotransmission into the hippocampus of rats submitted to appropriate models predictive of antidepressant effects could further clarify the role of hippocampal dopamine in stress neurobiology and in the mechanism of antidepressant drugs.
Glutamatergic and nitrergic systems
Several preclinical studies have indicated that repeated treatment with NMDA receptor antagonists possesses antidepressant-like properties in different animal models (Sofia and Harakal Citation1975, Trullas and Skolnick Citation1990; Petrie et al. Citation1999; Berman et al. Citation2000). These data are supported by clinical evidence showing that drugs that reduce glutamatergic tone may play a role in the treatment of depression (Sanacora et al. Citation2004, Citation2006; Zarate et al. Citation2004, Citation2006). For example, chronic treatment with riluzole, a drug that decreases glutamate release (Wang et al Citation2004) and increases glutamate uptake (Azbill et al. Citation2000; Frizzo et al. Citation2004), induces antidepressant and anxiolytic effects (Zarate et al. Citation2004; Mathew et al. Citation2005). It also improves the mood of depressed patients resistant to antidepressants (Sanacora et al. Citation2006).
Although the mechanisms responsible for these effects are not still understood, several lines of evidence have pointed to the hippocampus as an important target for these drugs. The hippocampal glutamatergic system is activated by stress leading to local increase of glutamate release (Moghaddam Citation1993; Sunanda et al. Citation2000) through a mechanism that seems to be corticoid-dependent (Abraham et al. Citation1998). This increased glutamate level has been proposed to mediate stress-induced morphological damage to the hippocampus (Magariños and McEwen Citation1995b; McEwen Citation1996; Gould et al. Citation1997; Cameron et al. Citation1998; Gould and Tanapat Citation1999; Nacher and McEwen Citation2006). Moreover, glutamate is crucial for LTP (Lynch Citation2004) and positively modulates hippocampal theta rhythm (Puma and Bizot Citation1999; Bonansco et al. Citation2002; Leung and Shen Citation2004), thus facilitating learning/memory processes. Systemic administration of a NMDA receptor antagonist prevents the activation of the hippocampus in response to inescapable stress (Titze-de-Almeida et al. Citation1994a,Citationb). Also, blockade of hippocampal glutamatergic neurotransmission disrupts the acquisition of fear conditioning (Cammarota et al. Citation2004; Quinn et al. Citation2005; Roesler et al. Citation2006). Therefore, stress-induced glutamate release within the hippocampus may facilitate the formation of aversive memories and at high levels, promote hippocampal damage. These processes would then contribute to the development of stress-induced behavioural changes.
The aforementioned hypothesis is further supported by the observation that intra-hippocampal administration of NMDA antagonists prevents the anxiogenic effect induced by stress (Padovan et al. Citation2000) and induces antidepressant-like effects in rats (Padovan and Guimarães Citation2004). In accordance with these behavioural data, chronic treatment with antidepressants reduces glutamate release in hippocampal synaptosomes (Bonanno et al. Citation2005), downregulates NMDA receptors (Boyer et al. Citation1998) and decreases glutamate responsiveness (Zahorodna and Bijak Citation1999). Besides, antidepressant drugs that reduce glutamate levels, such as tianeptine, prevent stress-induced alterations in hippocampal neuronal morphology (McEwen and Chattarji Citation2004; Reagan et al. Citation2004) whereas drugs that block glutamate receptors enhance neurogenesis (Cameron et al. Citation1995, Citation1998; Yoshimizu and Chaki Citation2004; Nacher and McEwen Citation2006) and prevent stress-induced atrophy (Magariños and McEwen Citation1995b; McEwen Citation1996). Therefore, modulation of the glutamatergic system seems to play a key role in the regulation of synaptic plasticity in the hippocampus. Normalization of stress-induced alterations in glutamate function, on the other hand, would produce antidepressant effects. This normalization could be achieved via mechanisms targeting the glutamatergic synapse directly or indirectly, for example, involving other neurotransmitters such as serotonin, noradrenaline, or GABA. All these systems closely interact in the hippocampal circuitry (Vizi and Kiss Citation1998).
The observation that glutamate, by acting on NMDA receptors, can evoke the release of NO (Garthwaite et al. Citation1988) lead to the suggestion that the antidepressant-like effects produced by NMDA antagonists would result from reduced NO production in the brain. Jefferys and Funder (Citation1996) first reported that systemic inhibition of the enzyme nitric oxide synthase (NOS) produces an antidepressant-like effect in the forced swimming test (FST) in rats, an effect reversed by pretreatment with l-arginine, the substrate for NOS. Besides this observation, it was reported that chronic treatment with nitro-l-arginine, a NOS inhibitor, induces down-regulation of cortical β-adrenoceptors, an effect observed in rodents following chronic treatment with many clinically effective antidepressants (Karolewicz et al. Citation1999). These effects seem to be due to the inhibition of neuronal NOS (nNOS) because the administration of preferential inhibitors of this isoform also induced dose-dependent antidepressant-like effects in rodents (Yildiz et al. Citation2000).
Experimental and clinical studies suggest the existence of a dysregulation of the nitrergic system in stress-related disorders. Depressed patients show elevated plasma nitrate levels (Suzuki et al. Citation2001) and significant mood improvement in response to the systemic administration of methylene blue, a drug that inhibits NOS/ guanylate cyclase (Naylor et al. Citation1987). Moreover, enhanced hippocampal expression of the nNOS enzyme is reported in post-mortem brain of depressed patients (de Oliveira et al. Citation2000a,Citationb). Again, stress seems to contribute to this neurochemical dysregulation, since repeated exposure to inescapable stress leads to enhanced expression of NOS and enhanced levels of nitrogen oxides in the hippocampus (Harvey et al. Citation2004b, Citation2005).
Systemic administration of clinically effective antidepressants reduces NOS activity in the hippocampus (Luo and Tan Citation2001; Wegener et al. Citation2003) and local administration of a nNOS inhibitor immediately before or after an inescapable stress induces antidepressant-like effects in rats (Joca and Guimarães Citation2006). Increased NO levels in the hippocampus, therefore, could contribute to the development of stress-induced behavioural consequences. The mechanisms mediating these effects, however, are not completely understood. NO is proposed to modulate synaptic transmission in several ways, the most common being through activation of soluble guanylate cyclase (sGC) and nitrosilation of proteins and enzymes (Snyder and Ferris Citation2000; Prast and Philippu Citation2001). As a result, NO can modulate neuronal excitability and neurotransmitter release (Snyder and Ferris Citation2000; Prast and Philippu Citation2001). It can decrease serotonin levels in several brain regions (Kiss Citation2000; Prast and Philippu Citation2001), including the hippocampus (Weneger et al. Citation2000). The antidepressant-like profile of NOS inhibitors in the FST was similar to that of serotonin selective reuptake inhibitors and depended on intact serotonin neurotransmission (Harkin et al. Citation2003). Taken together, these data suggest that the increase in NO production that follows stressful situations can impair serotonergic transmission in the brain, interfering with the adaptation to repeated stress exposure. On the other hand, activation of 5-HT1A receptors negatively regulates glutamate release (Dijk et al. Citation1995; Matsuyama et al. Citation1996) and NO synthesis (Strosznajder et al. Citation1996), suggesting that these systems mutually interact in the hippocampal formation to modulate behaviour under stressful conditions.
NO could also change neuronal function by direct neurotoxic effects. In primary cortical culture administration of NOS inhibitors prevents cell death elicited by NMDA and related excitatory amino acids, thus suggesting that NO mediates glutamate-induced neurotoxicity (Dawson et al. Citation1991). Besides, in cultured hippocampal neurons, NO can decrease BDNF release and inhibition of nNOS enhances hippocampal BDNF expression (Canossa et al. Citation2002). We observed similar effects in vivo after treatment with a nNOS inhibitor that produced antidepressant-like effects in the FST (). Moreover, systemic inhibition of NO is reported to increase hippocampal neurogenesis (Pacher et al. Citation2003; Cardenas et al. Citation2005; Zhu et al. Citation2006). These data suggest that stress-induced release of NO could mediate pathological processes within the hippocampus that account for the plastic changes and behavioural alterations seen in stressed animals. Therefore, inhibition of NO synthesis could protect the hippocampus from stress-induced effects, thus allowing behavioural adaptation to occur in situations of chronic exposure to stress.
Figure 4 Subchronic treatment with a NOS inhibitor increases BDNF expression in the hippocampus. The figure shows stained cells for BDNF in the hippocampal formation after (a) vehicle, (b) imipramine (15 mg/kg) and (c) 7-nitro-indazole (60 mg/kg), a preferential nNOS inhibitor, treated rats. They received three i.p. injections (0, 5 and 24 h) and were killed 24 h after the last injection, the hippocampus was removed and processed for BDNF immunohistochemistry (primary anti-BDNF antibody: rabbit polyclonal antibody raised against a peptide mapping at the N-terminus of BDNF of human origin, Santa Cruz Biotechnology, Santa Cruz, CA, USA; 1:800). Note BDNF signal (dark stained cells) only in (b) and (c). DG, dentate gyrus; CA, ammons horn. Bar = 150 μm.
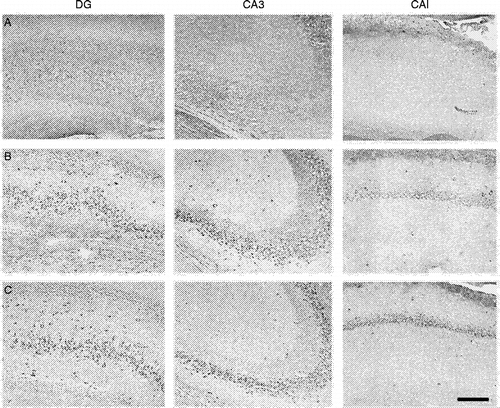
Based on these data, it is possible to speculate that an enhanced NMDA/NO-pathway facilitates the development in animals of behavioural deficits induced by stress and thus may contribute to the pathophysiology of stress-related disorders, such as depression and PTSD. Drugs that attenuate NMDA and/or NO-mediated neurotransmission would offer an alternative treatment for these disorders, either alone or in combination with antidepressant drugs (Zarate et al. Citation2006).
Conclusion
Stress-induced behavioural changes are a complex phenomenon that certainly involves more than one structure. However, numerous pieces of evidence have pointed to the hippocampal formation as an important target for stress and antidepressant-induced effects. Several main neurotransmitter systems in the hippocampus are related to stress effects. Serotonin, by acting on 5-HT1A receptors in the dorsal hippocampus, facilitates adaptation to severe inescapable stress. A failure in this system induced by severe stress and/or high corticoid levels would predispose to the development of stress-induced behavioural deficits. This process would be facilitated by glutamate and NO. Drugs that facilitate 5-HT1A- or attenuate glutamatergic/nitrergic-mediated neurotransmission in the hippocampal formation, on the other hand, would promote adaptation to stress and induce antidepressant-like effects (). The role of noradrenaline in these processes is less clear and may differ depending on the specific hippocampal region (dorsal vs. ventral).
Figure 5 Severe stress, activating the NMDA/NO pathway, can impair normal hippocampal functioning, predisposing to helplessness and depressive symptoms. Conversely, serotonin can counteract the stress-induced damage to the hippocampus, restoring normal functioning and allowing coping and stress adaptation. These effects are probably mediated by 5-HT1A receptors. GCs have a permissive role in NMDA damaging effects and at high levels, can impair 5-HT1A-mediated neurotransmission. Clinical studies using tryptophan depletion and results obtained with direct intra-hippocampal drug injections suggest that serotonin, glutamate and NO can also interfere with hippocampal function independently of cellular remodelling and neurogenesis.
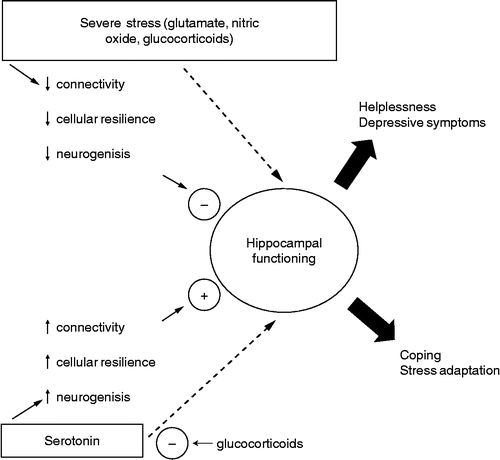
Acknowledgements
The authors acknowledge J. C. de Aguiar and E. L. T. Gomes for their helpful technical assistance. This research was supported by grants from CAPES, CNPq and FAPESP (02/13197-2 and 04/01738-4).
Behavioral procedures described in this manuscript were conducted in conformity with the Brazilian Society of Neuroscience and Behavior guidelines for the care and use of laboratory animals, which are in compliance with international laws and policies. The experimental protocols were approved by the local Ethical Committee and all efforts were made to minimize animal suffering.
References
- Abraham I, Juhasz G, Kekesi KA, Kovacs KJ. Corticosterone peak is responsible for stress-induced elevation of glutamate in the hippocampus. Stress 1998; 2(3)171–181
- Aguilar-Valles A, Sanchez E, de Gortari P, Balderas I, Ramirez-Amaya V, Bermudez-Rattoni F, Joseph-Bravo P. Analysis of the stress response in rats trained in the water-maze: Differential expression of corticotropin-releasing hormone, CRH-R1, glucocorticoid receptors and brain-derived neurotrophic factor in limbic regions. Neuroendocrinology 2005; 82(5–6)306–319
- Aleisa AM, Alzoubi KH, Gerges NZ, Alkadhi KA. Chronic psychosocial stress-induced impairment of hippocampal LTP: Possible role of BDNF. Neurobiol Dis 2006; 22(3)453–462
- Alfonso J, Pollevick GD, Van Der Hart MG, Flugge G, Fuchs E, Frasch AC. Identification of genes regulated by chronic psychosocial stress and antidepressant treatment in the hippocampus. Eur J Neurosci 2004; 19(3)659–666
- Alfonso J, Frick LR, Silberman DM, Palumbo ML, Genaro AM, Frasch AC. Regulation of hippocampal gene expression is conserved in two species subjected to different stressors and antidepressant treatments. Biol Psychiatry 2006; 59(3)244–251
- Almaguer-Melian W, Rojas-Reyes Y, Alvare A, Rosillo JC, Frey JU, Bergado JA. Long-term potentiation in the dentate gyrus in freely moving rats is reinforced by intraventricular application of norepinephrine, but not oxotremorine. Neurobiol Learn Mem 2005; 83(1)72–78
- Alonso R, Griebel G, Pavone G, Stemmelin J, Le Fur G, Soubrie P. Blockade of CRF1 or V1B receptors reverses stress-induced suppression of neurogenesis in a mouse model of depression. Mol Psychiatry 2004; 9: 278–286
- Andrade TG, Silva AM, Silva CL, Graeff FG. Effect of electrolytic lesion of the median raphe nucleus on behavioral and physiological measures of stress. Acta Physiol Pharmacol Ther Latinoam 1999; 49(4)279–289
- Angelucci F, Mathe AA, Aloe L. Brain-derived neurotrophic factor and tyrosine kinase receptor Trk B in rat brain are significantly altered after haloperidol and risperidone administration. J Neurosci Res 2000; 60: 783–794
- Anisman H, Matheson K. Stress, depression, and anhedonia: Caveats concerning animal models. Neurosci Biobehav Rev 2005; 29(4–5)525–546
- Avital A, Segal M, Richter-Levin G. Contrasting roles of corticosteroid receptors in hippocampal plasticity. J Neurosci 2006; 26(36)9130–9134
- Azbill RD, Mu X, Springer JE. Riluzole increases high-affinity glutamate uptake in rat spinal cord synaptosomes. Brain Res 2000; 871: 175–180
- Bai O, Chlan-Fourney J, Bowen R, Keegan D, Li XM. Expression of brain-derived neurotrophic factor mRNA in rat hippocampus after treatment with antipsychotic drugs. J Neurosci Res 2003; 71(1)127–131
- Banasr M, Hery M, Printemps R, Daszuta A. Serotonin-induced increases in adult cell proliferation and neurogenesis are mediated through different and common 5-ht receptor subtypes in the dentate gyrus and the subventricular zone. Neuropsychopharmacology 2004; 29: 450–460
- Bannerman DM, Rawlins JN, Mchugh SB, Deacon RM, Yee BK, Bast T, Zhang WN, Othuizen HH, Feldon J. Regional dissociations within the hippocampus-memory and anxiety. Neurosci Biobehav Rev 2004; 28(3)273–283
- Basso AM, Glagher NA, Bratcher NA, Brioni JD, Moreland RB, Hsieh GC, Drescher K, Fox GB, Decker MW, Rueter LE. Antidepressant-like effects of D2/3 receptor-, but not D4 receptor-activation in the rat forced swimming test. Neuropsychopharmacology 2005; 30: 1257–1268
- Baungartner A, Graf KJ, Kurten I. The dexamethasone suppression test in depression in schizophrenia, and during emotional stress. Biol Psychiat 1985; 20: 675–679
- Beck SG, Choi KC, List TJ, Okuhara DY, Birnstiel S. Corticosterone alters 5-HT1A receptor-mediated hyperpolarization in area CA1 hippocampal pyramidal neurons. Neuropsychopharmacology 1996; 14: 27–33
- Berman RM, Cappiello A, Anand A, Oren DA, Heninger GR, Charney DS, Krystal JH. Antidepressant effects of ketamine in depressed patients Biol. Psychiatry 2000; 47(4)351–354
- Berridge KC, Robinson TE. What is the role of dopamine in reward: Hedonic impact, reward learning, or incentive salience?. Brain Res Brain Res Rev 1998; 28(3)309–369
- Bertoglio LJ, Joca SR, Guimarães FS. Further evidence that anxiety and memory are regionally dissociated within the hippocampus. Behav Brain Res 2006, in press
- Bijak M. Prolonged treatment with antidepressant drugs increases the excitatory effect of quinpirole in hippocampal slices. Pol J Pharmacol 1993; 45(4)381–390
- Bijak M, Smialowski A. The effect of acute and prolonged treatment with citalopram on the action of dopamine and SKF 38393 in rat hippocampal slices. Eur J Pharmacol 1988; 149(1–2)41–47
- Bijak M, Zahorodna A, Tokarski K. Opposite effects of antidepressants and corticosterone on the sensitivity of hippocampal CA1 neurons to 5-HT1A and 5-HT4 receptor activation. Arch Pharmacol 2001; 363: 491–498
- Bjartmar L, Johansson IM, Marcusson J, Ross SB, Seckl JR, Olsson T. Selective effects on NGFI-A, MR, GR and NGFI-B hippocampal mRNA expression after chronic treatment with different subclasses of antidepressants in the rat. Psychopharmacology 2000; 151(1)7–12
- Bland ST, Schmid MJ, Greenwood BN, Watkins LR, Maier SF. Behavioral control of the stressor modulates stress-induced changes in neurogenesis and fibroblast growth factor-2. Neuroreport 2006; 17(6)593–597
- Blier P. The pharmacology of putative early-onset antidepressant strategies. Eur Neuropsychopaharmacol 2003; 13: 57–66
- Blier P, De Montigny C. Currents advances and trends in the treatment of depression. Trends Pharmacol Sci 1994; 15: 220–226
- Blood AJ, Zatorre RJ, Bermudez P, Evans AC. Emotional responses to pleasant and unpleasant music correlate with activity in paralimbic brain regions. Nat Neurosci 1999; 2: 382–387
- Bonanno G, Giambelli R, Raiteri L, Tiraboschi E, Zappettini S, Musazzi L, Raiteri M, Racagni G, Popoli M. Chronic antidepressants reduce depolarization-evoked glutamate release and protein interactions favoring formation of SNARE complex in hippocampus. J Neurosci 2005; 25(13)3270–3279
- Bonansco C, Gonzalez de la Vega A, Gonzalez Alegre P, Borde M, Garca-Segura LM, Buno W. Tetanic stimulation of schaffer collaterals induces rhythmic bursts via NMDA receptor activation in rat CA1 pyramidal neurons. Hippocampus 2002; 12(4)434–446
- Bonne O, Grillon C, Vythilingam M, Neumeister A, Charney DS. Adaptive and maladaptive psychobiological responses to severe psychological stress: Implications for the discovery of novel pharmacotherapy. Neuroendocrine Biobehav Rev 2004; 28: 65–94
- Boyer PA, Skolnick P, Fossom LH. Chronic administration of imipramine and citalopram alters the expression of NMDA receptor subunit mRNAs in mouse brain. A quantitative in situ hybridization study. J Mol Neurosci 1998; 10(3)219–233
- Bremner JD. The relationship between cognitive and brain changes in posttraumatic stress disorder. Ann NY Acad Sci 2006; 1071: 80–86
- Bremner J, Narayan M, Anderson ER, Staib LH, Miller HL, Charney DS. Hippocampal volume reduction in major depression. Am J Psychiatry 2000; 157: 115–118
- Brezun JM, Daszuta A. Depletion in serotonin decreases neurogenesis in the dentate gyrus and the subventricular zone of adult rats. Neuroscience 1999; 89: 999–1002
- Brezun JM, Daszuta A. Serotonergic reinnervation reverses lesion-induced decreases in PSA-NCAM labeling and proliferation of hippocampal cells in adult rats. Hippocampus 2000; 10: 37–46
- Brunello N, Mendlewicz J, Kasper S, Leonard B, Montgomery S, Nelson J, Paykel E, Versiani M, Racagni G. The role of noradrenaline and selective noradrenaline reuptake inhibition in depression. Eur Neuropsychopharmacol 2002; 12(5)461–475
- Buckley MJ. The role of perirhinal cortex and the hippocampus in learning, memory, and perception. Q J Exp Psychol B 2005; 58(3–4)246–268
- Cabib S, Puglisi-Allegra S. Stress, depression and the mesolimbic dopamine system. Psychopharmacology 1996; 128(4)331–342
- Cameron H, Gould E. Distinct populations of cells in the adult dentate gyrus undergo mitosis or apoptosis in response to adrenalectomy. J Comp Neurol 1996; 369: 56–63
- Cameron HA, McEwen BS, Gould E. Regulation of adult neurogenesis by excitatory input and NMDA receptor activation in the dentate gyrus. J Neurosci 1995; 15: 4687–4692
- Cameron H, Tanapat P, Gould E. Adrenal steroids and N-methyl-d-aspartate receptor activation regulate neurogenesis in the dentate gyrus of adult rats through a common pathway. Neuroscience 1998; 82: 349–354
- Cammarota M, Bevilaqua LR, Bonini JS, Rossatto JI, Medina JH, Izquierdo N. Hippocampal glutamate receptors in fear memory consolidation. Neurotox Res 2004; 6(3)205–212
- Canossa M, Giordano E, Cappello S, Guarnieri C, Ferri S. Nitric oxide down-regulates brain-derived neurotrophic factor secretion in cultured hippocampal neurons. Proc Natl Acad Sci USA 2002; 99(5)3282–3287
- Cardenas A, Moro MA, Hurtado O, Leza JC, Lizasoain I. Dual role of nitric oxide in adult neurogenesis. Brain Res Brain Res Rev 2005; 50(1)1–6
- Carli M, Samanin R. 8-Hydroxy-2-(di-n-propylamino)tetralin impairs spatial learning in a water maze: Role of post-synaptic 5-HT1A receptors. Br J Pharmacol 1992; 105: 720–726
- Carli M, Lazarova M, Tatarczynska E, Samanin R. Stimulation of 5-HT1A receptors in the dorsal hippocampus impairs acquisition and performance of a spatial task in a water maze. Brain Res 1992a; 595: 50–56
- Carli M, Tranchina S, Samanin R. 8-Hydroxy-2-(di-n-propylamino)tetralin, a 5-HT1A receptor agonist, impairs performance in a passive avoidance task. Eur J Pharmacol 1992b; 211: 227–234
- Carrasco G, Van de Kar LD. Neuroendocrine Pharmacology of stress. Eur J Pharmacol 2003; 463: 235–272
- Castrén E. Is mood chemistry?. Nat Rev Neurosci 2005; 6(3)241–246
- Castro ME, Diaz A, Del Olmo E, Pazos A. Chronic fluoxetine induces opposite changes in G protein coupling at pre and postsynaptic 5-HT1A receptors in rat brain. Neuropharmacol 2003; 44: 93–101
- Chalmers DT, Kwak A, Mansour A, Akil H, Watson SJ. Corticosteroids regulate brain hippocampal 5-HT1A receptor mRNA expression. J Neurosci 1993; 13(3)914–923
- Charney DS. Psychobiological mechanisms of resilience and vulnerability: Implications for successful adaptation to extreme stress. Am J Psychiatry 2004; 161: 195–216
- Checkley SA. Neuroendocrine mechanisms and the precipitation of depression by life events. Br J Psychiatry 1992; 160(supl. 15)7–17
- Cheetham SC, Crompton MR, Katona CLE, Horton RW. Brain 5-HT1 binding site in depressed suicides. Psychopharmacology 1990; 102: 544–548
- Chlan-Fourney J, Ashe P, Nylen K, Juorio AV, Li XM. Differential regulation of hippocampal BDNF mRNA by typical and atypical antipsychotic administration. Brain Res 2002; 954(1)11–20
- Coppell AL, Pei Q, Zetterstrom TS. Bi-phasic change in BDNF gene expression following antidepressant drug treatment. Neuropharmacology 2003; 44(7)903–910
- Corradetti R, Ballerini A, Pugliese AM, Pepeu G. Serotonin blocks the long-term potentiation induced by primed burst stimulation in the CA1 region of rat hippocampal slices. Neuroscience 1992; 46(3)511–518
- Czeh B, Michaelis T, Watanabe T, Frahm J, de Biurrun G, van Kampen M, Bartololomucci A, Fuchs E. Stress-induced changes in cerebral metabolites, hippocampal volume, and cell proliferation are prevented by antidepressant treatment with tianeptine. Proc Natl Acad Sci USA 2001; 98: 12796–12801
- D'Aquila PF, Collu M, Gessa LG, Serra G. The role of dopamine in the mechanism of action of antidepressant drugs. Eur J Pharmacol 2000; 405: 365–373
- Danzer SC, Crooks KRC, Lo DC, McNamara JO. Increased expression of brain-derived neurotrophic factor induces formation of basal dendrites and axonal branching in dentate granule cells in hippocampal explant cultures. J Neurosci 2002; 22(22)9754–9763
- Datson NA, van der Perk J, de Kloet ER, Vreugdenhil E. Identification of corticosteroid-responsive genes in rat hippocampus using serial analysis of gene expression. Eur J Neurosci 2001; 14: 675–689
- Dawson VL, Dawson TM, London ED, Bredt DS, Snyder SH. Nitric oxide mediates glutamate neurotoxicity in primary cortical cultures. Proc Natl Acad Sci USA 1991; 88: 6368–6371
- de Kloet ER. Stress in the brain. Eur J Pharmacol 2000; 405: 187–198
- de Kloet ER. Hormones, brain and stress. Endocr Regul 2003; 37(2)51–68
- de Kloet ER, Derijk R. Signaling pathways in brain involved in predisposition and pathogenesis of stress-related disease: Genetic and kinetic factors affecting the MR/GR balance. Ann NY Acad Sci 2004; 1032: 14–34
- de Kloet ER, Oitzl MS, Joels M. Stress and cognition: Are corticosteroids good or bad guys?. Trends Neurosci 1999; 22(10)422–426
- de Medeiros MA, Carlos Reis L, Eugenio Mello L. Stress-induced c-Fos expression is differentially modulated by dexamethasone, diazepam and imipramina. Neuropsychopharmacology 2005; 30(7)1246–1256
- de Oliveira RM, Del Bel EA, Mamede-Rosa ML, Padovan CM, Deakin JF, Guimarães FS. Expression of neuronal nitric oxide synthase mRNA in stress-related brain areas after restraint in rats. Neurosci Lett 2000a; 289(2)123–126
- de Oliveira RMW, Deakin JF, Guimarães FS. Neuronal nitric oxide synthase (NOS) expression in the hippocampal formation of patients with schizophrenia and affective disorder. J Psychopharmacol 2000b; 14(suppl)8
- Deakin JFW, Graeff FG. 5-HT and mechanisms of defense. J Psychopharmacol 1991; 5: 305–315
- Deakin JFW, Pennel I, Upadhyaya AK, Lofthouse R. A neuroendocrine study of serotonin function in depression: Evidence for biological mechanisms of endogenous and psychosocial causation. Psychopharmacology 1990; 101: 85–92
- Degroot A, Treit D. Anxiety is functionally segregated within the septo-hippocampal system. Brain Res 2004; 1001(1–2)60–71
- Del Bel EA, Silveira MC, Graeff FG, Garcia-Cairasco N, Guimarães FS. Differential expression of c-fos mRNA and Fos protein in the rat brain after restraint stress or pentylenetetrazol-induced seizures. Cell Mol Neurobiol 1998; 18(3)339–346
- Delgado PL, Charney DS, Price LH, Aghjanian GK, Landis H, Heninger GR. Serotonin function and mechanism of antidepressant action. Reversal of antidepressant-induced remission by rapid depletion of plasma tryptophan. Arch Gen Psychiatry 1990; 47: 411–418
- Diamond DM, Rose GM. Stress impairs LTP and hippocampal-dependent memory. Ann NY Acad Sci 1994; 746: 411–414
- Diamond DM, Park CR, Campbell AM, Woodson JC. Competitive interactions between endogenous LTD and LTP in the hippocampus underlie the storage of emotional memories and stress-induced amnesia. Hippocampus 2006; 15(8)1006–1025
- Dijk SN, Francis PT, Stratmann GC, Bowen DM. NMDA-induced glutamate and aspartate release from rat cortical pyramidal neurones: Evidence for modulation by a 5-HT1A antagonist. Br J Pharmacol 1995; 115(7)1169–1174
- Dranovsky A, Hen R. Hippocampal neurogenesis: Regulation by stress and antidepressants. Biol Psychiatry 2006; 59(12)1136–1143
- Drevets WC, Frank E, Price JC, Kupfer DJ, Greer PJ, Mathis C. Serotonin type-1A-receptor imaging in depression. Nuclear Med Biol 2000; 27: 499–507
- Drigues N, Poltyrev T, Bejar C, Weinstock M, Youdim MB. cDNA gene expression profile of rat hippocampus after chronic treatment with antidepressant drugs. J Neural Transm 2003; 110(12)1413–1436
- Dronjak S, Gavrilovic L. Effects of stress on catecholamine stores in central and peripheral tissues of long-term socially isolated rats. Braz J Med Biol Res 2006; 39(6)785–790
- Duman RS, Monteggia LM. A neurotrophic model for stress-related mood disorders. Biol Psychiatry 2006; 59(12)1116–1127
- Dwivedi Y, Rizavi HS, Pandey GN. Antidepressants reverse corticosterone-mediated decrease in brain-derived neurotrophic factor expression: Differential regulation of specific exons by antidepressants and corticosterone. Neuroscience 2006; 139(3)1017–1029
- Ejchel-Cohen TF, Wood GE, Wang JF, Barlow K, Nobrega JN, McEwen BS, Young TL. Chronic restraint stress decreases the expression of glutathione S-transferase pi2 in the mouse hippocampus. Brain Res 2006; 1090(1)156–162
- Fanselow MS. Contextual ear, gestalt memory, and the hippocampus. Behav Brain Res 2000; 110: 73–81
- Feldman S, Weidenfeld J. Glucocorticoid receptor antagonists in the hippocampus modify the negative feedback following neural stimuli. Brain Res 1999; 821(1)33–37
- Flugge G. Dynamics of central nervous 5-HT1A receptors under psychosocial stress. J Neurosci 1995; 15(11)7132–7140
- Foy MR, Stanton ME, Levine S, Thompson RF. Behavioral stress impairs long-term potentiation in rodent hippocampus. Behav Neural Biol 1987; 48(1)138–149
- Frankland PW, Bontempi B. The organization of recent and recent memories. Nat Rev Neurosci 2005; 6(20)119–130
- Frechilla D, Otano A, Del Rio J. Effect of chronic antidepressant treatment on transcription factor binding activity in rat hippocampus and frontal cortex. Prog Neuropsychopharmacol Biol Psychiatry 1998; 22(5)787–802
- Frizzo ME, Dall'Onder LP, Dalcin KB, Souza DO. Riluzole enhances glutamate uptake in rat astrocyte cultures. Cell Mol Neurobiol 2004; 24: 123–128
- Frodl T, Meisenzahl EM, Zetzsche T, Born C, Groll C, Jager M, Leinsinger G, Bottlender R, Hahn K, Moller HJ. Hippocampal changes in patients with a first episode of major depression. Am J Psychiatry 2002; 159: 1112–1118
- Fuchs E, Flugge G. Stress, glucocorticoids and structural plasticity of the hippocampus. Neurosci Biobehav Rev 1998; 23(2)295–300
- Fuchs E, Flugge G, Ohl F, Lucassen P, Vollmann-Honsdorf GK, Michaelis T. Psychosocial stress, glucocorticoids, and structural alterations in the tree shrew hippocampus. Physiol Behav 2001; 73(3)285–291
- Fuxe K, Diaz R, Cintra A, Bhatnagar M, Tinner B, Gustafsson JA, Ogren SO, Agnati LF. On the role of glucocorticoid receptors in brain plasticity. Cell Mol Neurobiol 1996; 16(2)239–258
- Galter D, Unsicker K. Sequential activation of the 5-HT1(A) serotonin receptor and TrkB induces the serotonergic neuronal phenotype. Mol Cell Neurosci 1996; 15(5)446–455
- Gambarana C, Ghigheri O, Tagliamonte A, D'Alessandro N, de Montis MG. Crucial role of D1 dopamine receptors in mediating the antidepressant effect of imipramine. Pharmacol Biochem Behav 1995; 50: 147–151
- Garthwaite J, Charles SL, Chess-Williams R. Endothelium-450 derived relaxing factor release on activation of NMDA 451 receptors suggests role as intercellular messenger in the brain. Nature 1988; 336(6197)385–388
- Gerlach JL, McEwen BS. Rat brain binds adrenal steroid hormone: Radioautography of hippocampus with corticosterone. Science 1975; 175: 1133–1136
- Gershon AA, Vishne T, Grunhaus L. Dopamine D2-like receptors and the antidepressant response. Biol Psychiatry 2006, in press
- Gilbertson MW, Shenton ME, Ciszewski A, Kasai K, Lasko NB, Orr SP, Pitman RK. Smaller hippocampal volume predicts pathologic vulnerability to pathological trauma. Nat Neurosci 2002; 5: 1242–1247
- Givalois L, Arancibia S, Tapia-Arancibia L. Concomitant changes in CRH mRNA levels in rat hippocampus and hypothalamus following immobilization stress. Brain Res Mol Brain Res 2000; 75(1)166–171
- Goldapple K, Segal Z, Garson C, Lau M, Bieling P, Kennedy S, Mayberg H. Modulation of cortical-limbic pathways in major depression: Treatment-specific effects of cognitive behavior therapy. Arch Gen Psychiatry 2004; 61(1)34–41
- Goldstein DS, McEwen B. Allostasis, homeostasis, and the nature of stress. Stress 2002; 5(1)55–58
- Gold PW, Licino J, Wong MA, Chrousos GP. Corticotropin releasing hormone in the pathophysiology of melancholic and atypical depression and in the mechanism of action of antidepressant drugs. Ann NY Acad Sci 1995; 771: 716–729
- Gould E, Tanapat P. Stress and hippocampal Neurogenesis. Biol Psychiatry 1999; 46(11)1472–1479
- Gould E, Cameron HA, Daniels DC, Wooley CS, McEwen BS. Adrenal hormones suppress cell division in the adult rat dentate gyrus. J Neurosci 1992; 12: 3642–3650
- Gould E, McEwen BS, Tanapat P, Galea LAM, Fuchs E. Neurogenesis in the dentate gyrus of the adult tree shrew is regulated by psychosocial stress and NMDA receptor activation. J Neurosci 1997; 17(7)2492–2498
- Govindarajan A, Rao BS, Nair D, Trinh M, Mawjee N, Tonegawa S, Chattarji S. Transgenic brain-derived neurotrophic factor expression causes both anxiogenic and antidepressant effects. Proc Natl Acad Sci USA 2006; 103(35)13208–13213
- Graeff FG, Guimarães FS, Andrade GCS, Deakin JFW. Role of 5-HT in stress, anxiety and depression. Pharmacol Biochem Behav 1996; 54(1)129–141
- Gray J, McNaughton N. The neuropsychology of anxiety. Oxford University Press. 2000; 2–36
- Gross C, Hen R. The developmental origins of anxiety. Nat Rev Neurosci 2004; 5: 545–552
- Gross C, Santarelli L, Brunner D, Zhuang X, Hen R. Altered fear circuits in 5-HT1A receptor KO mice. Biol Psychiatry 2000; 48: 1157–1163
- Guimarães FS, Del Bel EA, Padovan CM, Mendonça Netto S, Titze De Almeida R. Hippocampal 5-HT receptors and consolidation of stressful memories. Behav Brain Res 1993; 58: 133–139
- Haddjeri N, Blier P, De Montigny C. Long term antidepressant treatments result in a tonic activation of forebrain 5HT1a receptors. J Neurosci 1998; 18(23)10150–10156
- Hajos-Korcsok E, Mctavish SF, Sharp T. Effect of a selective 5-hydroxytryptamine reuptake inhibitor on brain extracellular noradrenaline: Microdialysis studies using paroxetine. Eur J Pharmacol 2000; 407(1–2)101–107
- Hajos M, Hoffman WE, Robinson DD, Yu JH, Hajos-Korcsok E. Norepinephrine but not serotonin reuptake inhibitors enhance theta and gamma activity of the septo-hippocampal system. Neuropsychopharmacology 2003; 28(5)857–864
- Harkin AJ, Connor TJ, Walsh M, St Jonh N, Kelly JP. Serotonergic mediation of the antidepressant-like effects of nitric oxide inhibitors. Neuropharmacology 2003; 44: 616–623
- Harvey BH, Naciti C, Brand L, Stein DJ. Endocrine, cognitive and hippocampal/cortical 5HT1A/2A receptor changes evoked by a time-dependent sensitization (TDS) stress model in rats. Brain Res 2003; 983: 97–107
- Harvey BH, Naciti C, Brand L, Stein DJ. Serotonin and stress: Protective or malevolent actions in the biobehavioral response to repeated trauma?. Ann NY Acad Sci 2004a; 1032: 267–272
- Harvey BH, Oosthuizen F, Brand L, Wegener G, Stein DJ. Stress-restress evokes sustained iNOS activity and altered GABA levels and NMDA receptors in rat hippocampus. Psychopharmacology 2004b; 175(4)494–502
- Harvey BH, Bothma T, Nel A, Wegener G, Stein DJ. Involvement of the NMDA receptor, NO-cyclic GMP and nuclear factor K-beta in an animal model of repeated trauma. Hum Psychopharmacol 2005; 20(5)367–373
- Heidbreder CA, Weiss IC, Domeney AM, Pryce C, Homberg J, Hedou G, Feldon J, Moran MC, Nelson P. Behavioral, neurochemical and endocrinological characterization of the early social isolation syndrome. Neuroscicence 2000; 100(4)749–768
- Heim C, Newport DJ, Heit S, Graham YP, Wicox M, Bonsall R, Miller AH, Nemeroff CB. Pituitary–adrenal and autonomic responses to stress in women after sexual and physical abuse childhood. JAMA 2000; 284(5)592–597
- Heninger GR, Delgado PL, Charney DS. The revised monoamine theory of depression: A modulatory role for monoamines, based on new findings from monoamine depletion experiments in humans. Pharmacopsychiatry 1996; 29(1)2–11
- Herman JP, Cullinan WE. Neurocircuitry of stress: Central control of the hypothalamus–pituitary–adrenal axis. Trends Neurosci 1997; 20: 78–84
- Herman JP, Watson SJ. Stress regulation of mineralocorticoid receptor heteronuclear RNA in rat hippocampus. Brain Res 1995; 677(2)243–249
- Herman JP, Ostrander MM, Mueller NK, Figueiredo H. Limbic system mechanisms of stress regulation: Hypothalamo–pituitary–adrenocortical axis. Prog Neuropsychopharmacol Biol Psychiatry 2005; 29(8)1201–1213
- Hyman SE, Malenka RC, Nestler EJ. Neural mechanisms of addiction: The role of reward-related learning and memory. Annu Rev Neurosci 2006; 29: 565–598
- Ida Y, Elsworth JD, Roth RH. Anxiogenic beta-carboline FG 7142 produces activation of noradrenergic neurons in specific brain regions of rats. Pharmacol Biochem Behav 1991; 39(3)791–793
- Isenberg N, Silberswieg D, Engelien A, Emmrich S, Malavade K, et al. Linguistic threat activates the human amygdala. Proc Natl Acad Sci USA 1999; 96: 10456–10459
- Jefferys D, Funder J. Nitric oxide modulates retention of immobility in the forced swimming test in rats. Eur J Pharmacol 1996; 295: 131–135
- Joca SR, Guimarães FS. Inhibition of neuronal nitric oxide synthase in the rat hippocampus induces antidepressant-like effects. Psychopharmacology 2006; 185(3)298–305
- Joca SR, Padovan CM, Guimarães FS. Activation of post-synaptic5-HT1A receptors in the dorsal hippocampus prevents learned helplessness development. Brain Res 2003; 978: 177–184
- Joca SR, Zanelati T, Guimarães FS. Post-stress facilitation of serotonergic, but not noradrenergic, neurotransmission in the dorsal hippocampus prevents learned helplessness development in rats. Brain Res 2006; 1087(1)67–74
- Joels M. Corticosteroid actions in the hippocampus. J Neuroendocrinol 2001; 13(8)657–669
- Johnson SA, Fournier NM, Kalynchuk LE. Effect of different doses of corticosterone on depression-like behavior and HPA axis responses to a novel stressor. Behav Brain Res 2006; 168(2)280–288
- Karl A, Schaefer M, Malta LS, Dorfel D, Rohleder N, Werner A. A meta-analysis of structural brain abnormalities in PTSD. Neurosci Biobehav Rev 2006; 30(7)1004–1031
- Karolewicz B, Bruce KH, Lee B, Paul IA. Nitric oxide synthase inhibitors have antidepressant-like properties in mice 2. Chronic treatment results in downregulation of cortical β-adrenoceptors. Eur J Pharmacol 1999; 372: 215–220
- Karten YGJ, Nair SM, van Esses L, Sibug R, Joels M. Long-term exposure to high corticosterone levels attenuates serotonin responses in rat hippocampal CA1 neurons. Proc Natl Acad Sci USA 1999; 26(23)13456–13461
- Katsuki H, Izumi Y, Zorumski CF. Noradrenergic regulation of synaptic plasticity in the hippocampal CA1 region. J Neurophysiol 1997; 77(6)3013–3020
- Keller J, Flores B, Gomez RG, Solvason HB, Kenna H, Williams GH, Schatzberg AF. Cortisol circadian rhythm alterations in psychotic major depression. Biol Psychiatry 2006; 60(3)275–281
- Kennett GA, Dickinson SL, Curzon G. Central serotonergic responses and behavioral adaptation to repeated immobilisation: The effect of the corticosterone synthesis inhibitor metyrapone. Eur J Pharmacol 1985a; 119: 143–152
- Kennett GA, Dickinson SL, Curzon G. Enhancement of some 5-HT-dependent behavioral responses following repeated immobilization in rats. Brain Res 1985b; 330: 253–263
- Kennett GA, Dourish CT, Curzon G. Antidepressant-like action of 5-HT1A agonists and conventional antidepressants in an animal model of depression. Eur J Pharmacol 1987; 134: 265–274
- Khawaja X, Xu J, Liang JJ, Barrett JE. Proteomic analysis of protein changes developing in rat hippocampus after chronic antidepressant treatment: Implications for depressive disorders and future therapies. J Neurosci Res 2004; 75(4)451–460
- Kim JJ, Diamond DM. The stressed hippocampus, synaptic plasticity and lost memories. Nat Rev Neurosci 2002; 3: 453–462
- Kim JJ, Koo JW, Lee HJ, Han JS. Amygdalar inactivation blocks stress-induced impairments in hippocampal long-term potentiation and spatial memory. J Neurosci 2005; 25(6)1532–1539
- Kim JJ, Song EY, Kosten TA. Stress effects in the hippocampus: Synaptic plasticity and memory. Stress 2006; 9(1)1–11
- Kiss JP. Role of nitric oxide in the regulation of monoaminergic neurotransmission. Brain Res Bull 2000; 52(6)459–466
- Kitraki E, Karandrea D, Kittas C. Long-lasting effects of stress on glucocorticoid receptor gene expression in the rat brain. Neuroendocrinology 1999; 69(5)331–338
- Kjelstrup KG, Tuvnes FA, Steffenach HA, Murison R, Moser EI, Moser MB. Reduced fear expression after lesions of the ventral hippocampus. Proc Natl Acad Sci USA 2002; 99(16)10825–10830
- Klemenhagen KC, Gordon JA, Davids DJ, Hen R, Gross CT. Increased fear response to contextual cues in mice lacking the 5-HT1A receptor. Neuropsychopharmacology 2006; 31: 101–111
- Knable MB, Barci BM, Webster MJ, Meador-Woodruff J, Torrey EF. Stanley neuropathology consortium. Molecular abnormalities of the hippocampus in severe psychiatric illness: Postmortem findings from the Stanley Neuropathology Consortium. Mol Psychiatry 2004; 9(6)609–620
- Knierim JJ. Hippocampus and memory. Can we have our place and fear it too?. Neuron 2003; 37(3)372–374
- Kohen R, Kirov S, Navaja GP, Happe HK, Hamblin MW, Snoddy JR, Neumaier JF, Petty F. Gene expression profiling in the hippocampus of learned helpless and nonhelpless rats. Pharmacogenomics J 2005; 5(5)278–291
- Kojima T, Matsumoto M, Togashi H, Tachibana K, Kemmotsu O, Yoshioka M. Fluvoxamine suppresses the long-term potentiation in the hippocampal CA1 field of anesthetized rats: An effect mediated via 5-HT1A receptors. Brain Res 2003; 959(1)165–168
- Koob GF. Hedonic valence, dopamine and motivation. Mol Psychiatry 1996; 1(3)186–189
- Lee J, Duan W, Mattson MP. Evidence that brain derived neurotrophic factor is required for basal neurogenesis and mediates, in part, the enhancement of neurogenesis by dietary restriction in the hippocampus of adult mice. J Neurochem 2002a; 82: 1367–1375
- Lee AL, Ogle WO, Sapolsky RM. Stress and depression: Possible links to neuron death in the hippocampus. Bipolar Disord 2002b; 4(2)117–128
- Legutko B, Szewczyk B, Pomierny-Chamiolo L, Nowak G, Pilc A. Effect of MPEP treatment on brain-derived neurotrophic factor gene expression. Pharmacol Rep 2006; 58(3)427–430
- Leonard BE. Stress, norepinephrine and depression. J Psychiatry Neurosci 2001; 26: S11–S16
- Lesch KP, Aulakh CS, Wolozin BL, Tolliver TJ, Hill JL, Murphy DL. Regional brain expression of serotonin transporter mRNA and its regulation by reuptake inhibiting antidepressants. Brain Res Mol Brain Res 1993; 17(1–2)31–35
- Leung LS, Shen B. Glutamatergic synaptic transmission participates in generating the hippocampal EEG. Hippocampus 2004; 14(4)510–525
- Liberzon I, Lopez JF, Flagel SB, Vazquez DM, Young EA. Differential regulation of hippocampal glucocorticoid receptors mRNA and fast feedback: Relevance to post-traumatic stress disorder. J Neuroendocrinol 1999; 11(1)11–17
- Lipska BK, Khaing ZZ, Weickert CS, Weinberger DR. BDNF mRNA expression in rat hippocampus and prefrontal cortex: Effects of neonatal ventral hippocampal damage and antipsychotic drugs. Eur J Neurosci 2001; 14: 135–144
- Liu D, Diorio J, Tannenbaum B, Caldji C, Francis D, Freedman A, Sharma S, Pearson D, Plotsky PM, Meaney MJ. Maternal care, hippocampal glucocorticoid receptors, and hypothalamic-pituitary-adrenal responses to stress. Science 1997; 277(5332)1659–1662
- Lopez JF, Chalmers DT, Little KY, Watson SJ. Regulation of 5-HT1A, glucocorticoid receptor in rat and human hippocampus: Implications for the neurobiology of depression. Biol Psychiat 1998; 43(8)547–573
- Lopez JF, Liberzon I, Vazquez DM, Young EA, Watson SJ. Serotonin 1A receptor messenger RNA regulation in the hippocampus after acute stress. Biol Psychiatry 1999; 45(7)934–937
- Lopez JF, Akil H, Watson SJ. Role of biological and psychological factors in early development and their impact on adult life-neural circuits mediating stress. Biol PsychiatRY 1999; 46: 1461–1471
- Luo L, Tan RX. Fluoxetine inhibits dendrite atrophy of hippocampal neurons by decreasing nitric oxide synthase expression in rat depression model. Acta Pharmacol Sin 2001; 22(10)865–870
- Lupien SJ, De Leon M, De Santi S, Convit A, Tarshish C, Nair NPV, Thakur M, McEwen B, Hauger R, Meaney MJ. Cortisol levels during human aging predict hippocampal atrophy and memory deficits. Nat Neurosci 1998; 1(1)69–72
- Lynch MA. Long-term potentiation and memory. Physiol Rev 2004; 84(1)87–136
- MacQueen G, Campbell S, McEwen BS, Macdonald K, Amano S, Joffe RT, Nahmias C, Young LT. Course of illness, hippocampal function, and hippocampal volume in major depression. Proc Natl Acad Sci USA 2003; 100: 1387–1392
- Magariños AM, McEwen BS. Stress-induced atrophy of apical dendrites of hippocampal CA3c neurons: Comparison of stressors. Neuroscience 1995a; 69(1)83–88
- Magariños AM, McEwen BS. Stress-induced atrophy of apical dendrites of hippocampal CA3c neurons: Involvement of glucocorticoid secretion and excitatory amino acid receptors. Neuroscience 1995b; 69(1)89–98
- Magariños AM, McEwen BS, Flugge G, Fuchs E. Chronic psychosocial stress causes apical dendritic atrophy of hippocampal CA3 pyramidal neurons in subordinate tree shrews. J Neurosci 1996; 16(10)3534–3540
- Malberg JE, Duman RS. Cell proliferation in adult hippocampus is decreased by inescapable stress: Reversal by fluoxetine treatment. Neuropsychopharmacology 2003; 28(9)1562–1571
- Marini F, Pozzato C, Andreetta V, Jansson B, Arban R, Domenici E, Carboni L. Single exposure to social defeat increases corticotropin-releasing factor and glucocorticoid receptor mRNA expression in rat hippocampus. Brain Res 2006; 1067(1)25–35
- Marmigere F, Givalois L, Rage F, Arancibia S, Tapia-Arancibia L. Rapid induction of BDNF expression in the hippocampus during immobilization stress challenge in adult rats. Hippocampus 2003; 13(5)646–655
- Mathew SJ, Amiel JM, Coplan JD, Fitterling HA, Sackeim HA, Gorman JM. Open-label trial of riluzole in generalized anxiety disorder. Am J Psychiatry 2005; 162: 2379–2381
- Matsuda S, Peng H, Yoshimura H, Wen TC, Fukuda T, Sakanaka M. Persistent c-fos expression in the brains of mice with chronic social stress. Neurosci Res 1996; 26(2)157–170
- Matsuyama S, Nei K, Tanaka C. Regulation of glutamate release via NMDA and 5-HT1A receptors in guinea pig dentate gyrus. Brain Res 1996; 728(2)175–180
- Mayberg HS, Brannan SK, Tekell JL, Silva JA, Mahurin RK, McGinnis S, Jerabek PA. Regional metabolic effects of fluoxetine in major depression: Serial changes and relationship to clinical response. Biol Psychiatry 2000; 48(8)830–843
- Mayer JL, Klumpers L, Maslam S, de Kloet ER, Joels M, Lucassen PJ. Brief treatment with the glucocorticoid receptor antagonist mifepristone normalises the corticosterone-induced reduction of adult hippocampal neurogenesis. J Neuroendocrinol 2006; 18(8)629–631
- McEwen BS. Gonadal and adrenal steroids regulate neurochemical and structural plasticity of the hippocampus via cellular mechanisms involving NMDA receptors. Cell Mol Neurobiol 1996; 16(2)103–116
- McEwen BS. Stress, adaptation and disease. Allostasis and allostatic load. Ann NY Acad Sci 1998; 840: 33–44
- McEwen BS. Glucocorticoids, depression and mood disorders: Structural remodelling in the brain. Metabolism 2005a; 54(Suppl 1)20–23
- McEwen BS. Stressed or stressed out: What is the difference?. J Psychiatry Neurosci 2005b; 30(5)315–317
- McEwen BS, Chattarji S. Molecular mechanisms of neuroplasticity and pharmacological implications: The example of tianeptine. Eur Neuropsychopharmacol 2004; 14(Suppl 5)S497–S502
- McEwen BS, Weiss JM, Schwartz LS. Selective retention of corticosterone by limbic structure in rat brain. Nature 1968; 220: 911–912
- Meijer OC, de Kloet ER. Corticosterone suppresses the expression of 5-HT1A receptor mRNA in rat hippocampus. Eur J Pharmacol 1994; 266: 255–261
- Melia KR, Ryabinin AE, Schroeder R, Bloom FE, Wilson MC. Induction and habituation of immediate early gene expression in rat brain by acute and repeated restraint stress. J Neurosci 1994; 14(10)5929–5938
- Mendelson SD, McEwen BS. Autoradiographic analysis of the effects of adrenalectomy and corticosterone on 5-HT1A and 5-HT1B receptors in the dorsal hippocampus and cortex of rats. Neuroendocrinology 1992; 55: 444–450
- Mervaala E, Fohr J, Kononen M, Valkonen-Korhonen M, Vainio P, Partanen K, Partanen J, Tiihanen J, Viinamaki H, Karjalainen AK, Lehtonen J. Quantitative MRI of the hippocampus and amygdala in severe depression. Psychol Med 2000; 30: 117–125
- Meyer U, van Kampen M, Isovich E, Flugge G, Fuchs E. Chronic psychosocial stress regulates the expression of both GR and MR mRNA in the hippocampal formation of tree shrews. Hippocampus 2001; 11(3)329–336
- Mikkelsen JD, Larsen MH. Effects of stress and adrenalectomy on activity-regulated cytoskeleton protein (Arc) gene expression. Neurosci Lett 2006; 403(3)239–243
- Mirescu C, Gould E. Stress and adult neurogenesis. Hippocampus 2006; 16(3)233–238
- Mirz F, Gjedde A, Sodkilde-Jorgensen H, Pedersen CB. Functional brain imaging of tinnitus-like perception induced by aversive auditory stimuli. Neuroreport 2000; 11: 633–637
- Moghaddam B. Stress preferentially increases extraneuronal levels of excitatory amino acids in the prefrontal cortex: Comparison to hippocampus and basal ganglia. J Neurochem 1993; 60(5)1650–1657
- Molteni R, Calabrese F, Bedogni F, Tongiorgi E, Fumagalli F, Racagni G, Riva MA. Chronic treatment with fluoxetine up-regulates cellular BDNF mRNA expression in rat dopaminergic regions. Int J Neuropsychopharmacol 2006; 9(3)307–317
- Mongeau R, Blier P, De Montigny C. The serotonergic and noradrenergic systems of the hippocampus: Their interactions and their effects of antidepressant treatments. Brain Res Rev 1997; 23: 145–195
- Morilak DA, Barrera G, Echevarria DJ, Garcia AS, Hernandez A, Ma S, Petre CO. Role of brain norepinephrine in the behavioral response to stress. Prog Neuropsychopharmacol Biol Psychiatry 2005; 29(8)1214–1224
- Morinobu S, Fujimaki K, Kawano K, Tanaka K, Takahashi J, Ohkawa M, Yamawaki S, Kato N. Influence of immobilization stress on the expression and phosphatase activity of protein phosphatase 2A in the rat brain. Biol Psychiatry 2003; 54(10)1060–1066
- Moscovitch M, Rosenbaum RS, Gilboa A, Addis DR, Westmacott R, Grady C, McAndrews MP, Levine B, Black S, Winocur G, Nadel L. Functional neuroanatomy of remote episodic, semantic and spatial memory: A unified account based on multiple trace theory. J Anat 2005; 207(1)35–66
- Moscovitch M, Nadel L, Winocur G, Gilboa A, Rosenbaum RS. The cognitive neuroscience of remote episodic, semantic and spatial memorial memory. Curr Opin Neurobiol 2006; 16(2)179–190
- Murakami S, Imbe H, Morikawa Y, Kubo C, Senba E. Chronic stress, as well as acute stress, reduces BDNF mRNA expression in the rat hippocampus but less robustly. Neurosci Res 2005; 53(2)129–139
- Nacher J, McEwen BS. The Role of N-methyl-d-asparate receptors in neurogenesis. Hippocampus 2006; 16: 267–270
- Naylor GJ, Smith AH, Connelly P. A controlled trial of methylene blue in severe depressive illness. Biol Psychiatry 1987; 22(5)657–689
- Nelson JC, Charney DS. The symptoms of major depressive illness. Am J Psychiatry 1981; 138(1)1–13
- Nestler EJ, Carlezon WA. The mesolimbic dopamine reward circuit in depression. Biol Psychiatry 2006; 59: 1151–1159
- Netto SM, Guimarães FS. Role of hippocampal 5-HT1A receptors on elevated plus maze exploration after a single restraint experience. Behav Brain Res 1996; 77(1–2)215–218
- Netto SM, Silveira R, Coimbra NC, Joca SR, Guimarães FS. Anxiogenic effect of median raphe nucleus lesion in stressed rats. Prog Neuropsychopharmacol Biol Psychiatry 2002; 26(6)1135–1141
- Neumaier JF, Sexton TJ, Hamblim MW, Beck SG. Corticosteroids regulate 5-HT1A but not 5-HT1B receptor mRNA in rat hippocampus. Mol Brain Res 2000; 82: 65–73
- Nibuya M, Takahashi M, Russel DS, Duman RS. Repeated stress increases catalytic TrkB mRNA in rat hippocampus. Neurosci Lett 1999; 267: 81–84
- Nichols NR, Masters JN, Finch CE. Changes in gene expression in hippocampus in response to glucocorticoids and stress. Brain Res Bull 1990; 24(5)659–662
- Nutt DJ. The role of dopamine and norepinephrine in depression and antidepressant treatment. J Clin Psychiatry 2006; 67(Suppl 6)3–8
- Ohl F, Michaelis T, Fujimori H, Frahm J, Rensing S, Fuchs E. Volumetric MRI measurements of the tree shrew hippocampus. J Neurosci Method 1999; 88(2)189–193
- Ohl F, Michaelis T, Vollmann-Honsdorf GK, Kirschbaum C, Fuchs E. Effect of chronic psychosocial stress and long-term cortisol treatment on hippocampus-mediated memory and hippocampal volume: A pilot-study in tree shrews. Psychoneuroendocrinology 2000; 25(4)357–363
- Oitzl MS, de Kloet ER. Selective corticosteroid antagonists modulate specific aspects of spatial orientation learning. Behav Neurosci 1992; 106: 62–71
- Oitzl MS, Reichardt HM, Joëls M, de Kloet ER. Point mutation in the mouse glucocorticoid receptor preventing DNA binding impairs spatial memory. Proc Natl Acad Sci USA 2001; 98: 12790–12795
- Okugawa G, Omori K, Suzukawa J, Fujiseki Y, Kinoshita T, Inagaki C. Long-term treatment with antidepressants increases glucocorticoid receptor binding and gene expression in cultured rat hippocampal neurones. J Neuroendocrinol 1999; 11(11)887–895
- Packer MA, Stasiv Y, Benraiss A, Chmielnicki E, Grinberg A, Westphal H, Goldman SA, Enikolopov G. Nitric oxide negatively regulates mammalian adult neurogenesis. Proc Natl Acad Sci USA 2003; 100(16)9566–9571
- Padovan CM, Guimarães FS. Attenuation of behavioral consequences of immobilization stress by intra-hippocampal microinjection of zimelidine. Braz J Med Biol Res 1993; 26(10)1085–1089
- Padovan CM, Guimarães FS. Antidepressant-like effects of NMDA-receptor antagonist injected into the dorsal hippocampus of rats. Pharmacol Biochem Behav 2004; 77(1)15–19
- Padovan CM, Del Bel EA, Guimarães FS. Behavioral effects in the elevated plus maze of an NMDA antagonist injected into the dorsal hippocampus: Influence of restraint stress. Pharmacol Biochem Behav 2000; 67(2)325–330
- Park C, Cho K, Ryu JH, Shin KS, Kim J, Ahn H, Huh Y. 7-Nitroindazole upregulates phosphorylated cAMP response element binding protein, polysialylated-neural cell adhesion molecule and tryptophan hydroxylase expression in the adult rat hippocampus. Brain Res 2004; 1008(1)120–125
- Pavlides C, Watanabe Y, McEwen BS. Effects of glucocorticoids on hippocampal long-term potentiation. Hippocampus 1993; 3(2)183–192
- Pavlides C, Nivon LG, McEwen BS. Effects of chronic stress on hippocampal long-term potentiation. Hippocampus 2002; 12(2)245–257
- Peeters BWMM, Broekkamp CLE. Involvement of corticosteroids in the processing of stressful life-events. A possible implication for the development of depression. J Steroid Mol Biol 1994; 49(4–6)417–427
- Pei Q, Zetterstrom TS, Sprakes M, Tordera R, Sharp T. Antidepressant drug treatment induces Arc gene expression in the rat brain. Neuroscience 2003; 121(4)975–982
- Petrie RX, Reid IC, Stewart CA. The N-methyl-d-aspartate receptor, synaptic plasticity, and depressive disorder. A critical review. Pharmacol Ther 1999; 87(1)11–25
- Petty F, Sherman AD. Regional aspects of the prevention of learned helplessness by desipramine. Life Sci 1980; 26: 1447–1452
- Petty F, Sherman AD. 1981. GABAergic modulation of learned helplessness.
- Pham K, Nacher J, Hof PR, McEwen BS. Repeated restraint stress suppresses neurogenesis and reduces biphasic PSA-NCAM expression in the adult rat dentate gyrus. Eur J Neurosci 2003; 17: 879–886
- Popova JS, Petkov VV. Changes in 5-HT1 receptors in different brain structures rats with isolation syndrome. Gen Pharmacol 1990; 21: 23–25
- Post RM. Transduction of psychosocial stress into the neurobiology of recurrent affective disorder. Am J Psych 1992; 149(8)999–1010
- Prast H, Philippu A. Nitric oxide as a modulator of neuronal function. Prog Neurobiol 2001; 64: 51–56
- Puma C, Bizot JC. Hippocampal theta rhythm in anesthetized rats: Role of AMPA glutamate receptors. Neuroreport 1999; 10(11)2297–2300
- Quinn JJ, Loya F, Ma QD, Fanselow MS. Dorsal hippocampus NMDA receptors differentially mediate trace and contextual fear conditioning. Hippocampus 2005; 15(5)665–674
- Radecki DT, Brown LM, Martinez J, Teyler TJ. BDNF protects against stress-induced impairments in spatial learning and memory and LTP. Hippocampus 2005; 15(2)246–253
- Radley JJ, Jacobs BL. 5-HT1A receptor antagonist administration decreases cell proliferation in the dentate gyrus. Brain Res 2002; 955(1–2)264–267
- Rasmussen AM, Libin S, Duman R. Down regulation mRNA in the hippocampal dentate gyrus after exposure to cues previously associated with footshock. Neuropsychopharmacology 2002; 27: 133–142
- Reagan LP, Rosell DR, Wood GE, Spedding M, Munoz C, Rothstein J, McEwen BS. Chronic restraint stress up-regulates GLT-1 mRNA and protein expression in the rat hippocampus: Reversal by tianeptine. Proc Natl Acad Sci USA 2004; 101(7)2179–2184
- Richell RA, Deakin JF, Anderson IM. Effect of acute tryptophan depletion on the response to controllable and uncontrollable noise stress. Biol Psychiatry 2005; 57(3)295–300
- Riedel G, Micheau J. Function of the hippocampus in memory formation: Desperately seeking resolution. Prog Neuropsychopharmacol Biol Psychiatry 2001; 25(4)835–853
- Roceri M, Hendriks W, Racagni G, Ellenbroek BA, Riva MA. Early maternal deprivation reduces the expression of BDNF and NMDA receptor subunits in rat hippocampus. Mol Psychiatry 2002; 7(6)609–616
- Roesler R, Vianna MR, Schroder N, Ferreira MB, Quevedo J. Aversive learning under different training conditions: Effects of NMDA receptor blockade in area CA1 of the hippocampus. Neurochem Res 2006; 31(5)679–683
- Rogoz Z, Legutko B. Combined treatment with imipramine and metyrapone induces hippocampal and cortical brain-derived neurotrophic factor gene expression in rats. Pharmacol Rep 2005; 57(6)840–844
- Rogoz Z, Skuza G, Legutko B. Repeated treatment with mirtazepine induces brain-derived neurotrophic factor gene expression in rats. J Physiol Pharmacol 2005; 56(4)661–671
- Rosario LA, Abercrombie ED. Individual differences in behavioral reactivity: Correlation with stress-induced norepinephrine efflux in the hippocampus of Sprague-Dawley rats. Brain Res Bull 1999; 48(6)595–602
- Rosenbrock H, Koros E, Bloching A, Podhorna J, Borsini F. Effect of chronic intermittent restraint stress on hippocampal expression of marker proteins for synaptic plasticity and progenitor cell proliferation in rats. Brain Res 2005; 1040(1/2)55–63
- Saaralainen T, Hendolin P, Lucas G, Koponen E, Sairane M, MacDonald E, Agerman K, Haapasalo A, Nawa H, Aloyz R, Ernfors P, Castrén E. Activation of the trkB neurotrophin receptor is induced by antidepressant drugs and requires for antidepressant-induced behavioural effects. J Neurosci 2003; 23(1)349–357
- Sairanen M, Lucas G, Ernfors P, Castrén M, Castrén E. Brain-derived neurotrophic factor and antidepressant drugs have different but coordinated effects on neuronal turnover, proliferation, and survival in the adult dentate gyrus. J Neurosci 2005; 25: 1089–1094
- Sakai N, Tanaka C. Inhibitory modulation of long-term potentiation via the 5-HT1A receptor in slices of the rat hippocampal dentate gyrus. Brain Res 1993; 613: 326–330
- Sanacora G, Kendell SF, Fenton L, Coric V, Krystal JH. Riluzole augmentation for treatment-resistant depression. Am J Psychiatry 2004; 161: 2132
- Sanacora G, Kendell SF, Levin Y, Simen AA, Fenton LR, Coric V, Krystal JH. Preliminary Evidence of riluzole efficacy in antidepressant-treated patients with residual depressive symptoms. Biol Psychiatry 2006, in press
- Sandi C, Loscertales M, Guaza C. Experience dependent facilitating effect of corticosterone on spatial memory formation in the water maze. Eur J Neurosci 1997; 9: 637–642
- Santarelli L, Saxe M, Gross C, Surget A, Battaglia F, Dulawa S, Weisstaub N, Lee J, Duman R, Arancio O, Belzung C, Hen R. Requirement of hippocampal neurogenesis for the behavioral effects of antidepressant. Science 2003; 301: 805–809
- Sapolsky RM. Glucocorticoids and hippocampal atrophy in neuropsychiatric disorders. Arch Gen Psychiatry 2000; 57(10)925–935
- Sapolsky RM, Uno H, Rebert CS, Finch CE. Hippocampal damage associated with prolonged glucocorticoid exposure in primates. J Neurosci 1990; 10(9)2897–2902
- Sapolsky RM, Romero M, Munck AU. How do glucocorticoids influences stress responses? Integrating permissive, suppressive, stimulatory, and preparative actions. Endocr Rev 2000; 21(1)55–89
- Sargent PA, Kjaer KH, Bench CJ, Rabiner EA, Messa C, Meyer J, Gun RN, Grasby PM, Cowen P. Brain serotonin1A receptor binding measured by positron emission topography with (11C) WAY100635. Arch Gen Psychiatry 2000; 57: 174–180
- Sarnyai Z, Sibille E, Pavlides C, Fenster RJ, McEwen BS. Impaired hippocampal-dependent learning and functional abnormalities in the hippocampus in mice lacking serotonin1A receptors. Proc Natl Acad Sci USA 2000; 97(26)14731–14736
- Scharfman H, Goodman J, Macleod A, Phani S, Antonelli C, Croll S. Increased neurogenesis and the ectopic granule cells after intrahippocampal BDNF infusion in adult rats. Exp Neurol 2005; 192(2)348–356
- Schinder AF, Poo M. The neurotrophin hypothesis for synaptic plasticity. Trends Neurosci 2000; 23(12)639–645
- Schmidt JL, Duman RS. Hippocampal neurogenesis: Opposing effects of stress and antidepressant treatment. Hippocampus 2006; 16(3)239–249
- Schreiber SS, Tocco G, Shors TJ, Thompson RF. Activation of immediate early genes after acute stress. Neuroreport 1991; 2(1)17–20
- Schwendt M, Jezova D. Gene expression of two glutamate receptor subunits in response to repeated stress exposure in rat hippocampus. Cell Mol Neurobiol 2000; 20(3)319–329
- Sciuciak J, Lewis DR, Wiegand SJ, Lindsay R. Antidepressant like effect of brain derived neurotrophic factor (BDNF). Pharmacol Biochem Behav 1997; 56(1)131–137
- Seager MA, Johnson LD, Chabot ES, Asaka Y, Berry SD. Oscillatory brain states and learning: Impact of hippocampal theta-contingent training. Proc Natl Acad Sci USA 2002; 99(3)1616–1620
- Seindenbecher T, Laxmi R, Stork O, Pape HC. Amygdalar and hippocampal theta rhythm synchronization during fear memory retrieval. Science 2003; 301: 846–850
- Selye H. A syndrome produced by diverse nocuous agents. Nature 1936; 138: 32
- Sheline Y. 3D MRI studies of neuroanatomic changes in unipolar major depression: The role of stress and medical comorbidity. Biol Psychiatry 2000; 48: 791–800
- Sheline Y, Wany P, Gado MH, Csernansky JG, Vannier MW. Hippocampal atrophy in recurrent major depression. Proc Natl Acad Sci USA 1996; 93: 3908–3913
- Sheline Y, Sanghavi M, Mintun MA, Gado MH. Depression duration but not age predicts hippocampal volume loss in medically healthy women with recurrent major depression. J Neurosci 1999; 19: 5034–5043
- Sheline Y, Gado M, Kraemer HC. Untreated depression and hippocampal volume loss. Am J Psychiatry 2003; 160: 1516–1518
- Sherman A, Petty F. Neurochemical basis of the action of antidepressants on learned helplessness. Behav Neural Biol 1980; 30: 119–134
- Shirayama Y, Chen ACH, Russel DS, Duman RS. Brain derived neurotrophic factor produces antidepressant effects in behavioural models of depression. J Neurosci 2002; 22(8)3251–3361
- Shors TJ, Foy MR, Levine S, Thompson RF. Unpredictable and uncontrollable stress impairs neuronal plasticity in the rat hippocampus. Brain Res Bull 1990; 24(5)663–667
- Shors TJ, Gallegos RA, Breindl A. Transient and persistent consequences of acute stress on long-term potentiation (LTP), synaptic efficacy, theta rhythms and bursts in area CA1 of the hippocampus. Synapse 1997; 26(3)209–217
- Shumake J, Edwards E, Gonzalez-Lima F. Dissociation of septo-hippocampal metabolism in the congenitally helpless rat. Neuroscience 2002; 114(2)373–377
- Shu SY, Wu YM, Bao XM, Leonard B. Interactions among memory-related centers in the brain. J Neurosci Res 2003; 71(5)609–616
- Smith ME. Bilateral hippocampal volume reduction in adults with post-traumatic stress disorder: A meta-analysis of structural MRI studies. Hippocampus 2005; 15(6)798–807
- Smith MA, Makino S, Kvetnansky R, Post RM. Stress alters the express of brain-derived neurotrophic factor and neurotrophin-3 mRNAs in the hippocampus. J Neurosci 1995; 15: 1768–1777
- Snyder SH, Ferris CD. Novel neurotransmitters and their psychiatric relevance. Am J Psychiatry 2000; 157(11)1738–1751
- Sofia RD, Harakal JJ. Evaluation of ketamine HCl for anti-depressant activity. Arch Int Pharmacodyn Ther 1975; 214(1)68–74
- Starkman MN, Schteingart DE, Schork MA. Depressed mood and other psychiatric manifestations of Cushing's syndrome: Relationship to hormone levels. Psychosom Med 1981; 43(1)3–18
- Starkman MN, Schteingart DE, Schork MA. Cushing's syndrome after treatment: Changes in cortisol and ACTH levels, and amelioration of the depressive syndrome. Psychiatry Res 1986; 19(3)177–188
- Starkman MN, Gebarski SS, Berent S, Schteingart DE. Hipocampal formation volume, memory dysfunction, and cortisol levels in patients with Cushing's syndrome. Biol Psychiatry 1992; 32(9)756–765
- Steffens D, Byrum CE, McQuoid DR, Greenberg DL, Payne ME, Blitchington TF, MacFall JR, Krishnan KR. Hippocampal volume in geriatric depression. Biol Psychiatry 2000; 48: 301–309
- Stein MB, Hanna C, Koverola C, Torchia M, McClarty B. Structural brain changes in PTSD. Does trauma alter neuroanatomy?. Ann NY Acad Sci 1997; 821: 76–83
- Stiedl O, Misane I, Spiess J, Ogren SO. Involvement of the 5-HT1A receptors in classical fear conditioning in C57BL/6J mice. J Neurosci 2000; 20(22)8515–8527
- Stockmeier CA, Shapiro LA, Dilley GE, Kolli TN, Friedman L, Rajkowska G. Increase in serotonin-1A autoreceptors in the midbrain of suicide victims with major depression-postmortem evidence for decreased serotonin activity. J Neurosci 1998; 18(18)7394–7401
- Storey JD, Robertson DA, Beattie JE, Reid IC, Mitchell SN, Balfour DJ. Behavioural and neurochemical responses evoked by repeated exposure to an elevated open platform. Behav Brain Res 2006; 166(2)220–229
- Strange BA, Hurlemann R, Dolan RJ. An emotion-induced retrograde amnesia in humans is amygdala- and beta-adrenergic-dependent. Proc Natl Acad Sci USA 2003; 100(23)13626–13631
- Strosznajder J, Chalimoniuk M, Samochocki M. Activation of serotonergic 5-HT1A receptor reduces Ca(2+)- and glutamatergic receptor-evoked arachidonic acid and No/cGMP release in adult hippocampus. Neurochem Int 1996; 28(4)439–444
- Sunanda, Rao BS, Raju TR. Restraint stress-induced alterations in the levels of biogenic amines, amino acids, and AChE activity in the hippocampus. Neurochem Res 2000; 25(12)1547–1552
- Suzuki E, Yagi G, Nakaki T, Kanba S, Asai M. Elevated plasma nitrate levels in depressive states. J Affect Disord 2001; 63: 221–224
- Tachibana K, Matsumoto M, Togashi H, Kojima T, Morimoto Y, Kemmotsu O, Yoshioka M. Milnacipran, a serotonin and noradrenaline reuptake inhibitor, suppresses long-term potentiation in the rat hippocampal CA1 field via 5-HT1A receptors and alpha 1-adrenoceptors. Neurosci Lett 2004; 357(2)91–94
- Takata K, Kitamura Y, Kakimura J, Kohno Y, Taniguchi T. Repeated administration of talipexole or pramipexole may have neuroprotective effect. Brain Res 2000; 872(1–2)236–241
- Tanapat P, Hastings NB, Rydel TA, Galea LA, Gould E. Exposure to fox odor inhibits cell proliferation in the hippocampus of adult rats via an adrenal hormone-dependent mechanism. Comp Neurol 2001; 437(4)496–504
- Thome J, Sakai N, Shin K, Steffen C, Zhang YJ, Impey S, Storm D, Duman RS. cAMP response element-mediated gene transcription is upregulated by chronic antidepressant treatment. J Neurosci 2000; 20(11)4030–4036
- Thome J, Pesold B, Baader M, Hu M, Gewirtz JC, Duman RS, Henn FA. Stress differentially regulates synaptophysin and synaptotagmin expression in hippocampus. Biol Psychiatry 2001; 50(10)809–812
- Titze-de-Almeida R, de Oliveira CL, Shida HW, Guimarães FS, Del Bel EA. Midazolam and the N-methyl-d-aspartate (NMDA) receptor antagonist 2-amino-7-phosphonoheptanoic acid (AP-7) attenuate stress-induced expression of c-fos mRNA in the dentate gyrus. Cell Mol Neurobiol 1994a; 14(4)373–380
- Titze-de-Almeida R, Shida H, Guimarães FS, Del-Bel EA. Stress-induced expression of the c-fos proto-oncogene in the hippocampal formation. Braz J Med Biol Res 1994b; 27(4)1083–1088
- Tohda M, Watanabe H. Imipramine-induced increase in 5-HT2C receptor mRNA level in the rat brain. Neurosci Res 1996; 24(2)189–193
- Trullas R, Skolnick P. Functional antagonists at the NMDA receptor complex exhibit antidepressant action. Eur J Pharmacol 1990; 185: 1–10
- Tsigos C, Chrousos GP. Hypothalamic–pituitary–adrenal axis, neuroendocrine factors and stress. J Psychosomatic Res 2002; 53: 865–871
- Uz T, Manev H. Chronic fluoxetine administration increases the serotonin N-acetyltransferase messenger RNA content in rat hippocampus. Biol Psychiatry 1999; 45(2)175–179
- Uz T, Ahmed R, Akhisaroglu M, Kurtuncu M, Imbesi M, Dirim Arslan A, Manev H. Effect of fluoxetine and cocaine on the expression of clock genes in the mouse hippocampus and striatum. Neuroscience 2005; 134(4)1309–1316
- Vaidya V, Marek GJ, Aghajanian GA, Duman RS. 5-HT2A receptor mediated regulation of BDNF mRNA in the hippocampus and the neocortex. J Neurosci 1997; 17: 2785–2795
- Vaiva G, Ducrocq F, Jezequel K, Averland B, Lestavel P, Brunet A, Marmar CRR. Immediate treatment with propranolol decreases posttraumatic stress disorder two months after trauma. Biol Psychiatry 2003; 54(9)947–949
- van Riedel E, Meijer OC, Steebergen PJ, Joels M. Chronic unpredictable stress causes attenuation of serotonin responses in cornu ammonis 1 pyramidal neurons. Neuroscience 2003; 120: 649–658
- Vazquez DM, Van Oers H, Levine S, Akil H. Regulation of glucocorticoid and mineralocorticoid receptor mRNAs in the hippocampus of the maternally deprived infant rat. Brain Res 1996; 731(1–2)79–90
- Vertes RP. Hippocampal theta rhythm: A tag for short-term memory. Hippocampus 2005; 15(7)923–935
- Vijayakumar M, Meti BL. Alterations in the levels of monoamines in discrete brain regions of clomipramine-induced animal model of endogenous depression. Neurochem Res 1999; 24(3)345–349
- Vizi ES, Kiss JP. Neurochemistry and pharmacology of the major hippocampal transmitter systems: Synaptic and nonsynaptic interactions. Hippocampus 1998; 8(6)566–607
- Vollmayr B, Simonis C, Weber S, Gass P, Henn F. Reduced cell proliferation in the dentate gyrus is not correlated with the development of learned helplessness. Biol Psychiatry 2003; 54: 1035–1040
- Vyas A, Mitra R. Shankaranarayana Rao B.S., Chattarji, S. Chronic stress induces contrasting patterns of dendritic remodeling in hippocampal and amygdaloid neurons. J Neurosci 2002; 22(15)6810–6818
- Wang SJ, Wang KY, Wang WC. Mechanisms underlying the riluzole inhibition of glutamate release from rat cerebral cortex nerve terminals (synaptosomes). Neuroscience 2004; 125: 191–201
- Watanabe Y, Gould E, McEwen BS. Stress induces atrophy of apical dendrites of hippocampal CA3 pyramidal neurons. Brain Res 1992; 588: 341–345
- Wegener G, Volke V, Harvey BH, Rosenberg R. Local, but not systemic, administration of serotonergic antidepressants decreases hippocampal nitric oxide synthase activity. Brain Res 2003; 959(1)128–134
- Weneger G, Volke V, Rosenberg R. Endogenous nitric oxide decreases hippocampal level of serotonin and dopamine in vivo. Br J Pharmacol 2000; 130(3)575–580
- Whiteford H, Peaboy C, Csermansky J, Warner M, Berger P. Elevated baseline and postdexamethasone cortisol levels. A reflection of severity or endogeneity?. J Affect Disord 1987; 12: 199–202
- Willner P. Validation criteria for animal models of human mental disorders: Learned helplessness as a paradigm case. Prog Neuropsychopharmacol Biol Psychiatry 1986; 10: 677–690
- Willner P. Animal models of depression: An overview. Pharmac Ther 1990; 45: 425–455
- Willner P. The mesolimbic dopamine system as a target for rapid antidepressant action. Int Clin Psychopharmacol 1997; Suppl 3: S7–14
- Willner P, Mitchell PJ. The validity of animal models of predisposition to depression. Behav Pharmacol 2002; 13(3)169–188
- Yadid G, Nakash R, Deri I, Tamar G, Kinor N, Gispan I, Zangen A. Elucidation of the neurobiology of depression: Insights from a novel genetic animal model. Prog Neurobiol 2000; 62(4)353–378
- Yamamoto J. Relationship between hippocampal theta-wave frequency and emotional behaviors in rabbits produced with stresses or psychotropic drugs. Jpn J Pharmacol 1998; 76(1)125–127
- Yau JL, Noble J, Hibberd C, Seckl JR. Short-term administration of fluoxetine and venlafaxine decreases corticosteroid receptor mRNA expression in the rat hippocampus. Neurosci Lett 2001; 306(3)161–164
- Yau JL, Noble J, Chapman KE, Seckl JR. Differential regulation of variant glucocorticoid receptor mRNAs in the rat hippocampus by the antidepressant fluoxetine. Brain Res Mol Brain Res 2004; 129(1–2)189–192
- Yildiz F, Erden BF, Ulak G, Utkan T, Gacar N. Antidepressant-like effect of 7-nitroindazole in the forced swimming test in rats. Psychopharmacology 2000; 149: 41–44
- Yoshimizu T, Chaki S. Increased cell proliferation in the adult mouse hippocampus following chronic administration of group II metabotropic glutamate receptor antagonist MGS0039. Biochem Biophys Res Commun 2004; 315(2)493–496
- Zahorodna A, Bijak M. An antidepressant-induced decrease in the responsiveness of hippocampal neurons to group I metabotropic glutamate receptor activation. Eur J Pharmacol 1999; 386(2–3)173–179
- Zangen A, Overstreet DH, Yadid G. Increased catecholamine levels in specific brain regions of a rat model of depression: Normalization by chronic antidepressant treatment. Brain Res 1999; 824(2)243–250
- Zarate CA, Jr, Payne JL, Quiroz J, Sporn J, Denicoff KK, Luckenbaugh D, Charney DS, Manji HK. An open-label trial of riluzole in patients with treatment-resistant major depression. Am J Psychiatry 2004; 161(1)171–174
- Zarate CA, Jr, Singh JB, Carlson PJ, Brutsche NE, Ameli R, Luckenbaugh DA, Charney DS, Manji HK. A randomized trial of an N-methyl-d-aspartate antagonist in treatment-resistant major depression. Arch Gen Psychiatry 2006; 63(8)856–864
- Zhu XJ, Hua Y, Jiang J, Zhou QG, Luo CX, Han X, Lu YM, Zhu DY. Neuronal nitric oxide synthase-derived nitric oxide inhibits neurogenesis in the adult dentate gyrus by down-regulating cyclic AMP response element binding protein phosphorylation. Neuroscience 2006; 141(2)827–836