Abstract
Sleep is critically involved in the consolidation of previously acquired memory traces. However, nocturnal sleep is not uniform but is subject to distinct changes in electrophysiological and neuroendocrine activity. Specifically, the first half of the night is dominated by slow wave sleep (SWS), whereas rapid eye movement (REM) sleep prevails in the second half. Concomitantly, hypothalamo-pituitary–adrenal (HPA) activity as indicated by cortisol release is suppressed to a minimum during early sleep, while drastically increasing during late sleep. We have shown that the different sleep stages and the concomitant glucocorticoid release are interactively involved in the consolidation of different types of memories. SWS-rich early sleep has been demonstrated to benefit mainly the consolidation of hippocampus-dependent declarative memories (i.e. facts and episodes). In contrast, REM sleep-rich late sleep was shown to improve in particular emotional memories involving amygdalar function, as well as procedural memories (for skills) not depending on hippocampal or amygdalar function. Enhancing plasma glucocorticoid concentrations during SWS-rich early sleep counteracted hippocampus-dependent declarative memory consolidation, but did not affect hippocampus-independent procedural memory. Preventing the increase in cortisol during late REM sleep-rich sleep by administration of metyrapone impaired hippocampus-dependent declarative memory but enhanced amygdala-dependent emotional aspects of memory. The data underscore the importance of pituitary–adrenal inhibition during early SWS-rich sleep for efficient consolidation of declarative memory. The increase in cortisol release during late REM sleep-rich sleep may counteract an overshooting consolidation of emotional memories.
Introduction
Although we sleep about one-third of our life, the functions of sleep are still obscure. Here, we follow the hypothesis that a primary function of sleep pertains to memory formation, specifically the consolidation of memories (McGaugh Citation2000). The term consolidation, first introduced by German psychologists Müller and Pilzecker (Citation1900), refers to the strengthening of newly encoded memory traces, which is necessary because these new memory traces are thought to be initially labile and to decay easily. In this way, consolidation counteracts forgetting, which would occur without such a strengthening process due to the decay of the fresh trace or due to retroactive interference from subsequently encoded material (Wixted Citation2004). Once stored and sufficiently consolidated, the information is flexibly available for retrieval in appropriate situations. Typically, a test of retrieval is used to probe existence of a memory since the memory trace cannot be directly measured. Encoding and retrieval of information are processes which occur mainly during wakefulness when the organism has to cope acutely with a great diversity of stressors. In contrast, the consolidation of newly acquired memories takes place preferentially during sleep, when the challenge by acute stressors is minimal. We suppose that processes of consolidation are shifted to the sleep phase, because relying on the same cellular networks as those used for the acute processing of stressors, these processes of consolidation would interfere with proper encoding and retrieval operations during wakefulness. Of course, this does not mean that memory consolidation during wakefulness cannot take place at all, but only that it is less effective than during sleep.
Beneficial effects of sleep on memory consolidation
In recent years, the view that sleep serves memory consolidation has received substantial support from a rapidly growing number of studies performed in various species and at different levels of analysis, comprising behavioral, cellular and molecular experiments (Born et al. Citation2006; Frank and Benington Citation2006; Joiner et al. Citation2006; Tononi and Cirelli Citation2006; Hennevin et al. Citation2007). An enhancing effect of sleep on memory consolidation has been demonstrated for major types of neuropsychologically distinct memories, i.e. declarative, procedural and emotional memories. Declarative memory refers to the explicit memories for facts and events, and experimentally is often tested using paired associate learning tasks (e.g. the learning of word pairs). Encoding and, initially, also retrieval of these memories relies essentially on the hippocampus (Squire Citation1992). However, over time retrieval becomes independent of the hippocampus, presumably due to a gradual transfer of these memories to other mainly neocortical networks (Sutherland and McNaughton Citation2000). Procedural memory refers to the memory for sensory and motor skills that are acquired gradually by repeated practice. It does not require hippocampal or amygdalar function but, amongst others, relies primarily on striato-cortical circuitry (Doyon and Benali Citation2005). In studies on sleep-dependent memory formation, procedural memory has been often assessed by the finger sequence tapping task which requires the subject to tap as fast and as accurately as possible a certain sequence of finger taps on a key board (Walker et al. Citation2002, Citation2003). Emotional memory is known to essentially rely on neural circuitries in which the amygdala is critically involved (Cahill et al. Citation1995; LeDoux Citation2000; Hamann Citation2001; McGaugh Citation2004). It is often assessed by the “emotional enhancement”, i.e. the extent to which the memory for an emotional stimulus (e.g. words with aversive meaning) is superior to the memory for a comparable but neutral stimulus (e.g. neutral non-arousing words). The emotional enhancement reflects primarily a modulating influence of the amygdala on hippocampal memory function (Akirav and Richter-Levin Citation2002; Dolcos et al. Citation2004; Phelps Citation2004).
A frequently used design in studies on sleep-associated memory consolidation compares recall after a retention period filled with nocturnal sleep (with learning in the evening before, and recall in the morning after) with recall after a retention period filled with daytime wakefulness (with learning in the morning and recall in the evening of the day). However, this approach confounds circadian phase with effects of sleep. To keep circadian phase constant, other studies compared retention periods across nocturnal periods of sleep vs. wakefulness. With this approach, impaired recall after the nocturnal vigil may reflect fatigue hampering processes of retrieval rather than inferior consolidation during the wake phase. The deprivation of sleep is known to induce a multitude of cognitive impairments that cannot be taken to infer, in reverse, the function of normal sleep (Ellenbogen Citation2005). To avoid confounds of sleep deprivation, designs were used in more recent studies where subjects either slept or remained awake the first night after learning and recall was tested not until after a second night which enabled recovery sleep before retrieval testing. Also, retention was compared for shorter periods of sleep and wakefulness to keep deprivation effects in the waking condition minimal.
Controlling in this way for confounds of the circadian phase and sleep deprivation induced fatigue, recent studies confirmed earlier findings that sleep after learning, in comparison with wakefulness, enhances retention of declarative memory for various materials (e.g. word pairs, spatial locations) (Drosopoulos et al. Citation2005; Fischer et al. Citation2006; Gais et al. Citation2006) and of procedural memories for visual discrimination and motor skills (Fischer et al. Citation2002). Interestingly, for procedural memory tasks a robust gain in performance is observed at a later retrieval testing the size of which has been shown to depend critically on the presence of sleep after initial training. This gain in performance suggests a latent off-line processing of memory representations during sleep that does not only stabilize but in fact enhances skill in the absence of any further practice. Regarding emotional memories, several studies have shown that when neutral and emotional memories have been encoded, sleep preferentially consolidates the emotional contents (Hu et al. Citation2006; Wagner et al. Citation2006) and also modulates emotional reactivity towards negatively valenced stimuli (Wagner et al. Citation2002). Such effects of sleep specifically on the consolidation of emotional memories can be extremely long-lasting. In a recent study we found that subjects were able to remember emotional texts even 4 years after learning when they had slept for only 3 h after learning, while the same texts were forgotten when the 3 h following learning had been spent awake (Wagner et al. Citation2006). Content of the neutral texts was generally forgotten after this long time interval regardless of whether sleep or wakefulness had followed learning.
A sleep-specific improvement in performance has not only been shown for procedural skills but also for other tasks, where sleep supported the gain of explicit (i.e. conscious) knowledge about rules and invariant feature of stimulus materials that had been acquired implicitly (i.e. unconsciously) before sleep (Wagner et al. Citation2004; Drosopoulos et al. Citation2005; Fischer et al. Citation2006). These findings of performance gains at retrieval testing indicate that sleep does not just passively strengthen memories but that memory consolidation during sleep is an active process that re-shapes the newly encoded memory representation and can thereby induce, depending on the type of task, a gain of procedural skill or explicit knowledge. A sleep-specific reorganization of newly encoded memory representations has been confirmed by studies using functional magnetic brain imaging (fMRI). If sleep, compared with wakefulness, followed initial acquisition, later retrieval of declarative memories (of a virtual maze) involved less hippocampal activity but increased striatal activity (Orban et al. Citation2006), later retrieval of emotional memories involved less amygdalar but increased prefrontal and hippocampal activity (Sterpenich et al. Citation2006), and later retrieval of procedural memories (for finger tapping skill) involved less activity in cortical motor areas but increased activity in regions of the basal ganglia (Fischer et al. Citation2005).
Together, all these results demonstrate a crucial role for sleep in the consolidation of various types of memories. However, sleep is not a uniform neurobiological state. On the contrary, sleep is characterized by distinct physiological changes in the patterns of both electrophysiological and neuendocrine activity across the night. These changes and their pertinence to memory functions of sleep are explained in the following sections.
Differential influences of slow wave sleep (SWS) and REM sleep
Electrophysiologically, sleep is characterized by the cyclic occurrence of different sleep stages (Rechtschaffen and Kales Citation1968), with the primary distinction referring to rapid eye movement (REM) sleep on the one hand and non-rapid eye movement (NonREM) sleep, which is further subdivided into sleep stages 1–4, on the other hand (). The deepest stages of NonREM sleep (stages 3 and 4) are termed SWS because of the EEG showing predominant slowly oscillating potential activity ( < 4 Hz). The length of a NonREM–REM sleep cycle is about 90 min. Sleep is entrained to the circadian rhythm and external zeitgebers such that in humans sleep normally occurs during night-time. Due to the coupling to a circadian oscillator, nocturnal sleep in humans can be roughly divided into an early and a late part distinctly differing in sleep architecture. During early sleep SWS is predominant whereas the other core sleep stage, i.e. REM sleep occurs only in marginal amounts. During late sleep this relationship is reversed; REM sleep predominates whereas the amount of SWS is greatly reduced.
Figure 1 (A) Sleep profile (hypnogram) for a typical night of sleep, showing the nocturnal sequence of electrophysiologically defined sleep stages (REM sleep and Non-REM sleep stages S1–S4). The deepest sleep stages S3 and S4 indicate SWS, characterized by slowly oscillating activity ( < 4 Hz) in the EEG. SWS and REM sleep are not equally distributed across the night. While sleep in the first half of the night (left from vertical line) is dominated by SWS, sleep in the second half of the night (right from vertical line) contains high amounts of REM sleep. W, wake; M, movement activity. (B) Due to circadian and sleep-dependent regulation (see text), cortisol release is strongly suppressed during early sleep, but increases distinctly during late sleep, reaching a maximum in the early morning hours. Accordingly, SWS-dominated early is accompanied by minimal levels of circulating cortisol, while basal cortisol levels are high during REM sleep-dominated late sleep. These changing patterns of electrophysiological and endocrine activity across nocturnal sleep interactively affect memory consolidation.
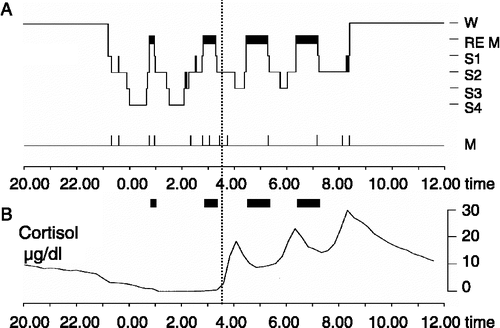
Traditionally, REM sleep has been considered more important for memory consolidation because this sleep stage is linked to dreams and obvious cognitive processing (Aserinsky and Kleitman Citation1953; Maurizi Citation1987; Eiser Citation2005). However, investigations using selective REM sleep deprivation overall were not conclusive (for summaries, Gais and Born Citation2004; Born et al. Citation2006), partly because the repeated arousal from REM sleep imposes a strong stress to subjects, which contaminates later recall performance (Horne and McGrath Citation1984; Cipolli Citation1995; Born and Gais Citation2000). To avoid the adverse effects of REM sleep deprivation procedures, Ekstrand's group developed an effective approach that compares effects of 3–4-h retention intervals filled with early SWS-rich sleep with those of 3–4-h retention intervals filled with late sleep where REM sleep is predominant (Yaroush et al. Citation1971; Barrett and Ekstrand Citation1972; Fowler et al. Citation1973; Ekstrand et al. Citation1977). Effects of early and late sleep are additionally contrasted with those of waking retention intervals during corresponding night-times. This approach revealed that retention of hippocampus-dependent declarative memory benefits particularly, but not exclusively, from SWS-dominated early nocturnal sleep, whereas memories not relying on the hippocampus, i.e. procedural memories as well as amygdala-dependent emotional memories mainly benefit from REM-dominated sleep in the late night (Plihal and Born Citation1997, Citation1999a; Wagner et al. Citation2001). The time spent in lighter NonREM sleep stages 1 and 2, as well as time awake was closely comparable between the early and late periods of retention sleep in these experiments, which excludes a primary role of these stages for the differential effects of early vs. late sleep on memory consolidation (although the possibility of specific influences cooperatively with SWS and REM sleep, respectively, cannot be ruled out).
A central assumption is that consolidation during sleep relies on a covert reactivation during sleep of the neuronal networks that were used for encoding the new memories during prior wakefulness (Buzsáki Citation1998; Sutherland and McNaughton Citation2000; Born et al. Citation2006). Consistent with this hypothesis hippocampal neuron assemblies implicated in encoding of spatial information during maze learning become reactivated in the same temporal order during succeeding SWS in rats (Wilson and McNaughton Citation1994; Ribeiro et al. Citation2004; Ji and Wilson Citation2007). Neuroimaging studies in healthy humans revealed signs of reactivation during sleep after learning, which for declarative tasks (virtual maze learning) occurred in hippocampal regions during SWS whereas for procedural tasks they were found in the cuneus and striatal regions during REM sleep (Maquet et al. Citation2000; Peigneux et al. Citation2003, Citation2004). Brain stimulation in humans that enhanced the slow oscillations characterizing SWS was found to selectively enhance the retention of declarative memories (Marshall et al. Citation2004, Citation2006), corroborating the concept that SWS particularly supports consolidation of hippocampus-dependent memories whereas memories not involving hippocampal function more strongly benefit from REM sleep. Note, however, that the typical experimental tasks do not provide pure measures of declarative, procedural or emotional memory (Peigneux et al. Citation2001). Accordingly, the overall memory benefit is normally determined by both SWS and REM sleep as well as their cyclic occurrence during the night (Giuditta et al. Citation1995; Stickgold et al. Citation1999; Ficca et al. Citation2000).
Regulation of the hypothalamo-pituitary axis (HPA) during sleep
The electrophysiological dynamics in sleep architecture are paralleled by distinct temporal changes in endocrine activity across the night embracing almost all hormonal systems (Steiger Citation2003). Regarding stress hormone regulation, hypothalamo-pituitary–adrenal axis (HPA) activity is distinctly suppressed in the first half of the night, but disinhibited in the second half of the night (Born and Fehm Citation1998). Consequently, early SWS-dominated sleep is associated with very low levels of circulating cortisol, while late REM-dominated sleep is associated with a high cortisol level, which reaches its circadian maximum around the time of morning awakening (). This circadian pattern changes only marginally during conditions of continuous 24-h wakefulness. However, sleep actively and synergistically adds to both the inhibition of ACTH (corticotropin) and cortisol release during early sleep and also to the activation of the HPA system during late sleep. The former effect can be unmasked by stimulating pituitary–adrenal activity during sleep through secretagogues like corticotropin-releasing hormone (CRH) and vasopressin (Späth-Schwalbe et al. Citation1993; Bierwolf et al. Citation1997). The secretory response to the releasing hormones when injected during SWS in early sleep is distinctly reduced in comparison with the response to these substances injected at the same dose and the same time of night while the subject is awake. This inhibitory action of SWS during early sleep on the release of ACTH and cortisol is likely mediated via the release of a release-inhibiting factor during this period. The molecular structure of this factor is presently unknown.
There is evidence from another study (Späth-Schwalbe et al. Citation1991) that late sleep, by disinhibiting the HPA system, likewise contributes to the morning increase in cortisol concentration. In this study, subjects were aroused after a 3-h period of early nocturnal sleep and then stayed awake. Arousing the subject induces a transient increase in ACTH and cortisol concentrations (Born et al. Citation1999; Wilhelm et al. Citation2007). However, during the succeeding waking period, concentrations of ACTH and cortisol were significantly lower than during the corresponding time interval of the control condition during which subjects continued sleeping. Because the pituitary–adrenal secretory response to intravenous CRH was not affected by late sleep (Späth-Schwalbe et al. Citation1993), the disinhibition during this sleep interval is likely mediated via mechanisms acting at the hypothalamic or a superordinate level of HPA regulation.
Although less clear, there is evidence that plasma catecholamine concentrations are also regulated to some extent by sleep processes. Sleep, and particularly REM sleep, reduces blood concentrations of norepinephrine and epinephrine (Dodt et al. Citation1997; Lechin et al. Citation2004; Wagner et al. Citation2005). In general, however, the clear-cut dissociation of circadian and sleep-related mechanisms in the regulation of the sympatho-adrenal and the HPA systems is methodologically difficult because of possible confounding variables like motor activity, body position, light exposure and food intake that interact with circadian and sleep-related mechanisms of hormonal regulation.
The modulating role of glucocorticoids in sleep-associated memory consolidation
To what extent does the nocturnal change in HPA activity, specifically the changing cortisol release, contribute to the differential pattern of memory consolidation during early and late sleep? This question is tempting particularly with respect to the consolidation of declarative and emotional memories, as these memory systems strongly rely on hippocampal and amygdalar function, i.e. two regions well-known to express corticosteroid receptors at high density (Joels and De Kloet Citation1994; De Kloet et al. Citation1998; Johnson et al. Citation2005). These receptors are essentially implicated in the well-documented effects of glucocorticoids on memory function during wakefulness (for overviews, see Lupien and McEwen (Citation1997), McGaugh and Roozendaal (Citation2002), Sauro et al. (Citation2003) and Het et al. (Citation2005)) and, hence, are most likely involved also in mediating respective effects on consolidation of memory during sleep. In an ongoing series of experiments in humans we have been aiming to characterize the role of glucocorticoidregulation specifically for sleep-associated memory consolidation.
Plihal and Born (Citation1999b) investigated effects of elevated cortisol plasma concentrations on retention of memories during early SWS-rich sleep (). Prior to retention sleep (i.e. 22.15–23.00 h), subjects learned to a criterion on a declarative word pair associate learning task and a procedural mirror tracing task. After learning, in one of the experimental nights cortisol was intravenously infused, and in a control night vehicle was infused. Infusions started at 23.00 h when lights were turned off to enable the 3-h period of retention sleep, which was followed by retrieval testing 15 min later. The dose of cortisol was low, starting at a rate of 16 mg/h during the first 15 min and of 4 mg/h during the remaining time, which induced cortisol concentrations in plasma of about 16 μg/dl during retention sleep, i.e. a level comparable with that observed naturally during the early morning hours or during mild stress (). Infusions were discontinued 30 min before awakening so that plasma cortisol concentrations differed between placebo and cortisol conditions only during retention sleep, but were again nearly identical during retrieval testing after sleep.
Figure 2 (A) Top: Experimental design for studying the influence of cortisol on memory consolidation during early nocturnal sleep, illustrated by an individual sleep profile. Before sleep, subjects learned memory tasks to a criterion. Lights were turned off at 23.00 h to enable a 3-h period of SWS-rich early sleep. Recall was tested 15–30 min after awakening. Infusion of cortisol (vs. placebo) started at 23.00 h and was discontinued after 2.5 h. Bottom: Mean( ± SEM) plasma cortisol concentrations during the Placebo (dotted line) and Cortisol conditions (solid line). Note that in the cortisol condition plasma concentrations of the hormone were enhanced only during the period of retention sleep, but did not differ from placebo at recall testing. (B) Cortisol infusion during consolidation sleep, compared with placebo, impaired retention of hippocampus-dependent declarative memories for word pairs across sleep (top), but did not affect speed in procedural mirror tracing skills not relying on the hippocampus (bottom). n = 14. *p < 0.05, **p < 0.01. Data from Plihal and Born (Citation1999b).
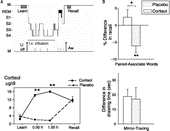
The main outcome of this study was that cortisol distinctly impaired consolidation of declarative memories for the word pairs. Learning performance before sleep was closely comparable between placebo and cortisol conditions. However, striking differences developed at retrieval testing after sleep. Whereas after infusion of placebo the subjects' recall performance of word pairs was improved on average by +2.4 ± 2.2% (with reference to performance on the criterion trial at learning before sleep), following infusion of cortisol the subjects had even forgotten a significant number of word pairs learned before sleep, with this impairment averaging − 6.1 ± 1.8% (p < 0.01). Mirror tracing skills, i.e. speed and error rate during tracing figures as trained before sleep, improved across sleep in both conditions, but was not influenced by cortisol, indicating that the hormone selectively impaired hippocampus-dependent declarative memory function, while leaving procedural memory unaffected. Whereas higher doses of cortisol can change the distribution of sleep stages towards increased SWS and reduced REM sleep (Born et al. Citation1987, Citation1989; Friess et al. Citation1994), the relatively low dose given here did not significantly change time spent in the different sleep stages in comparison with placebo. Thus, the influence of cortisol administration on declarative memory cannot be reduced to effects of changes in gross sleep architecture, which further strengthens the interpretation of a direct memory-impairing influence of cortisol on hippocampal receptors.
Glucocorticoids have been found to exert an impairing effect specifically on retrieval (de Quervain et al. Citation1998, Citation2000). However, this effect cannot satisfactorily explain the impaired recall of word pairs after cortisol infusion during sleep, because cortisol infusion in the study by Plihal and Born (Citation1999b) stopped almost 1 h before retrieval testing and plasma cortisol concentrations measured at retrieval, like those at learning before sleep, were nearly identical for the placebo and cortisol conditions. A delayed action of cortisol on retrieval operations is also unlikely in light of the rapid temporal dynamics of the effects on retrieval in previous studies, suggesting a non-genomic mediation of the effect (De Kloet and Reul Citation1987).
Mineralocorticoid receptors vs. glucocorticoid receptors
Cortisol acts on the brain via two different receptors, i.e. high affinity mineralocorticoid receptors (MR) and low affinity glucocorticoid receptors (GR) (De Kloet et al. Citation1998, Citation2005). Due to the distinctly higher affinity, 70–90% of MRs are continuously occupied even at nadir cortisol concentrations during early nocturnal sleep. Whereas GR are widely expressed throughout the brain, expression of MR is particularly high in limbic regions including the hippocampus and amygdala. Available findings indicate that the impairing effect of cortisol on declarative memory consolidation is mediated via an activation of GR.
Plihal et al. (Citation1999) examined the effects of the synthetic glucocorticoid dexamethasone (DEX; 2 mg) on memory consolidation during sleep. DEX preferentially binds GR. Because of its slow pharmacodynamics, DEX was administered orally 7 h before periods of retention sleep that covered 3-h intervals of SWS-rich early or REM sleep-rich late nocturnal sleep. As in the study described above on effects of cortisol in the early night (Plihal and Born Citation1999b), memory was tested using a word pair associate learning task and a mirror tracing task. Like cortisol, DEX did not affect the time in SWS during early retention sleep, but distinctly impaired retention of word pairs. During late retention sleep, DEX reduced the amount of REM sleep but did not affect consolidation of word pair memories. Further, DEX did not affect memory for mirror tracing skill during early or late retention sleep, although late REM sleep-rich sleep was, as expected, associated with a superior gain of mirror tracing skill. Since DEX did not change learning of word pairs before retention sleep, a confounding influence on encoding of memories is unlikely. Also, a primary impairing effect of DEX on retrieval processes could be ruled out in this study, because in this case the impairing effect of DEX on declarative word recall would be expected to occur independently of the timing of retention sleep. However, DEX impaired recall of word pairs selectively after early but not late retention sleep. In combination, these data support the notion that enhanced glucocorticoid activity during early sleep impairs consolidation of newly acquired hippocampus-dependent memories through predominant activation of GR.
MR may also contribute to declarative memory consolidation during sleep, as suggested by a recent study investigating effects of metyrapone, which blocks cortisol synthesis (Wagner et al. Citation2005). Metyrapone was given in the evening after subjects had learned texts that were either neutral or emotionally aversive. Compared with the effects of placebo, metyrapone distinctly reduced cortisol levels already during early SWS-rich sleep to values less than half of those during the placebo condition (). Due to the slow pharmacodynamics of metyrapone the cortisol suppression persisted throughout the night and did not cease until the next morning. Retrieval testing took place at 11.00 a.m., when cortisol levels did not differ any more between metyrapone and placebo conditions. Notably, in conjunction with distinctly reduced SWS during early sleep, metyrapone impaired retention of the neutral texts, representing pure hippocampus-dependent memory without amygdalar modulation. REM sleep during the late night remained unchanged, but amygdala-dependent aspects of emotional memory formation were enhanced (see below).
Figure 3 (A) Mean( ± SEM) plasma cortisol concentrations following oral administration of the cortisol synthesis inhibitor metyrapone (3 g, solid line) and placebo (dotted line) before an 8-h period of nocturnal retention sleep (shaded area). Memory tasks (neutral and emotional texts, mirror tracing) were learned before sleep. Retrieval was tested at 11.00 h the next day. (B) Metyrapone impaired retention (percent with reference to performance at learning before sleep) of hippocampus-dependent declarative memories for neutral texts across sleep (left), but augmented amygdala-dependent emotional enhancement in declarative memory, i.e. the superiority of emotional as compared to neutral text recall (middle). Consolidation of procedural memories for mirror tracing was unaffected by the treatment (right). n = 14. *p < 0.05, **p < 0.01, ***p < 0.001. Data from Wagner et al. (Citation2005).
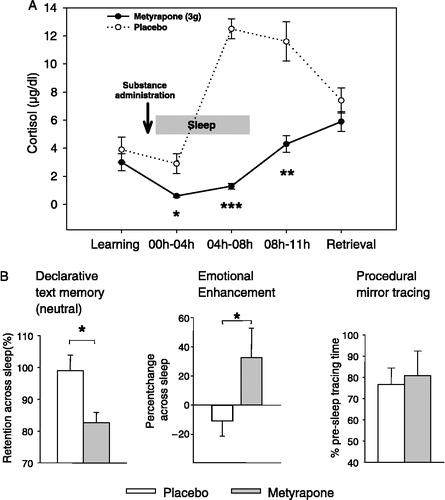
The combined suppression of SWS and consolidation of neutral texts likely reflects insufficient MR activation following metyrapone (Neylan et al. Citation2003). As mentioned, during early nocturnal sleep cortisol concentrations reach a minimum which is associated with preferential binding of high-affinity MRs (70–90%) whereas occupation of low-affinity GRs is marginal. Hence, a further >50% reduction of cortisol concentrations during this time, as observed after metyrapone, primarily reduces MR rather than GR occupation. An involvement of GRs is unlikely also because reduced GR activation would be expected to improve rather than impair memory consolidation (as discussed above). Metyrapone has been also demonstrated to diminish declarative memory function during wakefulness (Lupien et al. Citation2002a,Citationb; Maheu et al. Citation2004). However, in those studies metyrapone was administered in such a way that it was active both at the time of learning and in the hours following learning, which makes it difficult to disentangle treatment effects on memory acquisition and on memory consolidation. A direct comparison of the specific effects of metyrapone on memory consolidation occurring during sleep vs. wakefulness remains to be performed. Also, the hypothesis of primary MR involvement in the sleep-associated metyrapone effects on declarative memory needs to be substantiated in future studies.
The role of the cortisol rise during late sleep
Aside from nadir cortisol concentrations during early sleep, the role of the rise in cortisol during late sleep for memory consolidation has been investigated. As mentioned previously, late sleep, dominated by REM sleep, supports consolidation particularly of emotional and procedural memories. Increasing activation of GR during a period of late REM sleep-rich sleep by administration of DEX did not affect consolidation of procedural mirror tracing skill, although DEX reduced substantially the time in REM sleep (Plihal et al. Citation1999). This negative outcome is in line with previous findings (Kirschbaum et al. Citation1996) suggesting that procedural memory function relying on cortico-striatal circuitry is generally much less sensitive to effects of corticosteroids.
However, there is evidence that the increase in cortisol concentration during late sleep affects processing of emotional memories depending on amygdalar function (Wagner et al. Citation2005; ). In this study, mentioned above, administration of metyrapone diminished the naturally enhanced cortisol concentrations during late sleep to levels comparable with those normally observed during early sleep. Interestingly, retention of emotional texts was completely unaffected by the detrimental effect of metyrapone on retention of the neutral texts. As an indirect measure aiming to extract the “pure” amygdala contribution in the case of emotional text recall, we determined additionally the so-called “emotional enhancement”, i.e. the relative superiority of emotional over neutral memory recall. This emotional enhancement, which has been shown in numerous previous studies to be mediated by the amygdala (Cahill and McGaugh Citation1998; Hamann et al. Citation1999), turned out to even be augmented across sleep after metyrapone compared to placebo. Apparently, the normally occurring late night rise in cortisol does not facilitate but rather dampens amygdala-dependent emotional processing during REM sleep. In this way, cortisol release during late sleep could prevent over-consolidation of emotional memories. This effect is mediated via GR, as the late night increase in cortisol activates preferentially this type of corticosteroid receptor. Moreover, the effect of cortisol suppression is presumably directly related to limbic processing of emotional representations with only an indirect influence on brain stem centers generating REM sleep, since REM sleep per se remained unchanged by metyrapone. In addition to effects mediated by corticosteroid receptors, secondary influences of metyrapone could contribute to the effect on emotional enhancement in memory. Here, of particular relevance is the feedback-induced elevation of CRH which is likewise known to modulate emotional processing in the amygdala (Heilig et al. Citation1994; Roozendaal et al. Citation2002). In contrast to hippocampus- and amygdala-dependent memory, procedural mirror tracing skills not relying on hippocampal or amygdalar function were not affected by the suppression of cortisol synthesis after metyrapone treatment, which is consistent with findings indicating a general insensitivity of procedural memory to glucocorticoids (Plihal and Born Citation1999b; Plihal et al. Citation1999).
The finding of a facilitating role of low cortisol levels for amygdala-dependent emotional memory consolidation appears to be at variance with findings in humans and animals indicating a supportive effect of glucocorticoids on emotional memory (Roozendaal Citation2000; Buchanan and Lovallo Citation2001; Abercrombie et al. Citation2003; Maheu et al. Citation2004). However, none of these studies specifically referred to the role of sleep in memory consolidation so that, in humans at least, memory consolidation was always tested during daytime wakefulness. Sleep, as an offline mode of processing characterized by downregulated sensory inputs, relies on mechanisms basically different from those subserving memory function during wakefulness (Stickgold Citation2005; Born et al. Citation2006), which may also affect glucocorticoid action. Interestingly, in most rodent studies glucocorticoids were administered during daytime which, in contrast to humans, corresponds to the rest phase in these animals. The fact that these studies, likewise in opposition to the effects of glucocorticoids during sleep in humans, typically revealed memory enhancement after post-learning administration of glucocorticoids (for overviews, see, e.g. Lupien and McEwen Citation1997; De Kloet et al. Citation1998; Roozendaal Citation2000), points to the additional possibility of species-specific influences of corticosteroids on memory consolidation. However, to the best of our knowledge the actual amount of sleep during the consolidation interval has never been controlled in these animal studies.
Clinically, the finding of specific enhancement of emotional aspects in sleep-associated memory consolidation with lower cortisol activity seems to be particularly pertinent to the mechanisms in post-traumatic stress disorder (PTSD), a psychiatric disease with primary symptoms of hyper-emotionality in memory, as reflected by intrusive memories, flashbacks and nightmares (Charney et al. Citation1993; vanOyen Citation1997; Yehuda Citation2002). These symptoms are thought to reflect a pathological enhancement of amygdala-mediated memory processing in combination with impaired hippocampal functioning (van der Kolk Citation1994; Elzinga and Bremner Citation2002; Yehuda Citation2002). Interestingly, a typical neurobiological marker in PTSD are distinctly reduced basal cortisol levels (Mason et al. Citation1986; Yehuda et al. Citation1990, Citation1995; Kanter et al. Citation2001; Rohleder et al. Citation2004), and 24-hours profiles have shown that during sleep this cortisol reduction is particularly pronounced in the second half of the night (Yehuda et al. Citation1996). Moreover, reductions of SWS were found in PTSD patients in several studies (Glaubman et al. Citation1990; Fuller et al. Citation1994; Neylan et al. Citation2003), which likewise parallels the effects of metyrapone in our study. Thus, our pattern of metyrapone-induced effects on sleep and associated memory consolidation provide a useful model for several features characterizing PTSD and suggest that reduced cortisol levels, especially during sleep, play a crucial role for the development of the “overconsolidation” (Pitman Citation1989) of emotional memories in this disease.
A model of memory consolidation during sleep
There is converging evidence that covert reactivation of recently acquired memories represents a key mechanism of memory consolidation during sleep. A causative role of reactivation during SWS for consolidation of hippocampus-dependent memories has been recently demonstrated by Rasch et al. (Citation2007) using odors as contextual stimuli at learning that were re-exposed during subsequent sleep. Drawing on this evidence for a central role of reactivations, the consolidation of hippocampus-dependent memories during sleep has been conceptualized in the framework of a dialogue between neocortex and hippocampus, which also allows for integrating effects of stress hormones (Buzsáki Citation1998; Gais and Born Citation2004; Born et al. Citation2006; ). According to this model, during wakefulness information to be stored is encoded into both neocortical and hippocampal networks. The hippocampus serves as an intermediate buffer which encodes certain aspects of an experienced event very quickly but holds this information only temporarily. During subsequent SWS the newly encoded representations are repeatedly reactivated in the hippocampus and, in a coordinate way, also in neocortical networks (Sutherland and McNaughton Citation2000; Ji and Wilson Citation2007). Eventually, this reactivation stimulates a gradual transfer of the information from hippocampal to neocortical networks, with the latter serving as long-term store for the information. Hippocampal memory reactivation is driven by the slow oscillations ( < 1 Hz) that originate from neocortical circuitry and dominate SWS. In parallel with driving hippocampal memory reactivation the slow oscillations exert a grouping influence on thalamo-cortical (10–15 Hz) spindle activity, which presumably facilitates neuronal plastic processes within neocortical networks by stimulating calcium-dependent intracellular mechanisms (Sejnowski and Destexhe Citation2000; Sirota et al. Citation2003; Mölle et al. Citation2006). Through synchronizing the generation of thalamic spindle activity and hippocampal memory reactivation slow oscillations enable that feedback inputs from these structures (i.e. thalamo-cortical spindle activity and hippocampo-cortical memory transfer) arrive at the same time within neocortical networks. The co-occurrence of these feedback inputs from thalamus, hippocampus and probably also from further structures (e.g. locus coeruleus) that are subjected to the synchronizing influence of the slow oscillation, might eventually be the factor that facilitates the formation of longer-term memories within respective neocortical networks.
Figure 4 Model of declarative memory consolidation during sleep. During wakefulness information is encoded into neocortical networks and in part in hippocampal networks (gray arrow). During SWS, newly encoded information in the hippocampus is repeatedly reactivated. Reactivations are accompanied by hippocampal sharp wave-ripples. They are driven by slow oscillations which originate in neocortical networks (preferentially in those that were used for encoding at learning) and synchronize hippocampal memory reactivation with the occurrence of spindle activity in thalamo-cortical circuitry. Hippocampal reactivation stimulates a transfer of the newly encoded information back to neocortical networks (black arrow). The hippocampal input arriving in synchrony with spindle input at neocortical circuitry can induce long-term plastic changes selectively at those synapses previously used for encoding, thereby forming a long-term memory of the information in neocortical networks. High concentrations of cortisol inhibit hippocampal memory reactivation and transfer to neocortex via activation of GR. Likewise, memory reactivation and transfer is suppressed in the hippocampus with insufficient occupation of MR. The amygdala enhances hippocampal memory consolidation, depending on the emotional impact of the learning material. As a result of this amygdalar modulation, emotional material is generally better remembered than neutral material. Whether glucocorticoids affect emotional memory formation via an influence on amygdalar function or primarily via hippocampal function is not yet clear.
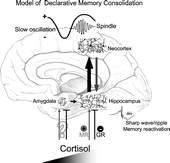
Hormones exert an impact mainly on the hippocampus to modulate declarative memory consolidation. Cortisol presumably affects hippocampal reactivation and hippocampo-neocortical memory transfer by fine-tuning local neuronal excitability and synaptic potentiation via activation of MR and GR (Joels et al. Citation1995). GR activation was found to inhibit glucose transport into hippocampal neurons and glia cells, to suppress hippocampal glutamatergic neurotransmission and excitatory output from CA1 neurons of the hippocampus (Horner et al. Citation1990; De Kloet et al. Citation1998, Citation2005). Predominant activation of GR has been found to suppress long-term potentiation (LTP) and primed burst potentiation in hippocampal cells, but to stimulate long-term synaptic depression and depotentiation (Pavlides et al. Citation1995, Citation1996; Pavlides and McEwen Citation1999; Alfarez et al. Citation2002). Blockade of GR prolonged maintenance of LTP in hippocampal dentate gyrus (Korz and Frey Citation2003; Avital et al. Citation2006). Together, these data indicate that enhanced GR activation globally suppresses excitatory neurotransmission as well as the induction and maintenance of LTP within hippocampal circuitry, i.e. conditions that likely counteract effective reactivation and transfer of memories to neocortical regions during sleep. In contrast, activation of MR has been found to change membrane properties of pyramidal CA1 neurons towards enhanced excitability (Joels and De Kloet Citation1992) and to prolong hippocampal LTP (Korz and Frey Citation2003). Blockade of MR suppressed the ability to induce LTP in dentate gyrus (Pavlides et al. Citation1996; Avital et al. Citation2006). These data concur with the view that sufficient activation of high affinity MR in hippocampal circuitry is a prerequisite for proper reactivation and transfer of memory during SWS.
Basically the same mechanisms may account for a glucocorticoid-induced suppression of emotional memory formation during REM sleep. Consistent with the view that emotional memories are reactivated during REM sleep, human imaging studies revealed increased amygdalar activation during this sleep stage (Maquet et al. Citation1996; Nofzinger et al. Citation1997). Recent evidence indicates that sleep-dependent consolidation of emotional memories is associated with a transfer and shift of the respective neural representations towards increased involvement of prefrontal cortex and hippocampus, while amygdalar involvement declines (Sterpenich et al. Citation2006). However, little is known so far about the underlying effects of corticosteroids on neuronal excitability and synaptic potentiation in the amygdala (Kavushansky and Richter-Levin Citation2006). Our data on metyrapone-induced effects on emotional memory formation (Wagner et al. Citation2005) suggest that cortisol might suppress the putative processes of memory reactivation and transfer occurring in the amygdala during REM sleep in the same way as hippocampal memory processing during SWS. Another possibility is that the corticosteroid effect on emotional memory consolidation results from an indirect influence on hippocampal networks due to an interaction with amygdalar networks during memory reactivation (Cahill and McGaugh Citation1998; Akirav and Richter-Levin Citation2002; Paz et al. Citation2006). According to this view, like activation during wakefulness, reactivation of the basolateral amygdala during REM sleep following an emotional experience feeds into hippocampal networks to enhance respective memory representations. This emotional enhancement of hippocampal memories is counteracted by activation of hippocampal GR due to elevated levels of cortisol during REM sleep. As hippocampal portions of the representation per se are enhanced during SWS (at nadir cortisol concentration), the modulating effects of cortisol during SWS-rich early and during REM sleep-rich late sleep would act in concert to support the maintenance of a declarative memory representation while attenuating its emotional impact.
In conclusion, we propose that stress hormone regulation influences memory consolidation during sleep primarily by an action on memory reactivation occurring in the hippocampus and amygdala during SWS and REM sleep, respectively. Inasmuch as the periods of reactivation in sleep share relevant features with memory reactivation during memory retrieval, our findings are also consistent with reports of negative glucocorticoid effects on retrieval processes (de Quervain et al. Citation1998, Citation2000; Kuhlmann et al. Citation2005). Here, our view that stress hormones critically influence sleep-associated memory consolidation has been specifically substantiated with respect to HPA activity, but needs further validation by extension to signals of the sympatho-adrenal stress system like norepinephrine, which is likewise known as a potent modulator of memory function (McGaugh and Roozendaal Citation2002; Southwick et al. Citation2002). During SWS, activity of norepinephrine is in between the levels observed during wakefulness and REM sleep, where release of catecholamines reaches a minimum (Dodt et al. Citation1997; Hobson and Pace-Schott Citation2002). Phasic increases in norepinephrine release during SWS may represent conditions favorable for synaptic plastic processes presumed to underlying the formation of long-term memories in neocortical networks (Cirelli and Tononi Citation2000). On the other hand, suppressed release of catecholamines during REM sleep might act in concert with increased cortisol concentrations to counteract a possible overshooting consolidation of emotional aspects of memory representations.
Last but not least, it is to be noted that memory formation represents a more general biological function that pertains not only to the neurobehavioral system but also to other systems, like the immune system, that likewise need to adapt to stressors (in this case, antigens) in the long-term. Some studies indeed support the notion that sleep supports immunological memory formation as well (Lange et al. Citation2003, Citation2006; Dimitrov et al. Citation2007), and this influence might likewise depend critically on the regulation of stress hormones during sleep.
References
- Abercrombie HC, Kalin NH, Thurow ME, Rosenkranz MA, Davidson RJ. Cortisol variation in humans affects memory for emotionally laden and neutral information. Behav Neurosci 2003; 117: 505–516
- Akirav I, Richter-Levin G. Mechanisms of amygdala modulation of hippocampal plasticity. J Neurosci 2002; 22: 9912–9921
- Alfarez DN, Wiegert O, Joels M, Krugers HJ. Corticosterone and stress reduce synaptic potentiation in mouse hippocampal slices with mild stimulation. Neuroscience 2002; 115: 1119–1126
- Aserinsky E, Kleitman N. Regularly occurring periods of eye motility and concomitant phenomena during sleep. Science 1953; 118: 273–274
- Avital A, Segal M, Richter-Levin G. Contrasting roles of corticosteroid receptors in hippocampal plasticity. J Neurosci 2006; 26: 9130–9134
- Barrett TR, Ekstrand BR. Effect of sleep on memory. 3. Controlling for time-of-day effects. J Exp Psychol 1972; 96: 321–327
- Bierwolf C, Struve K, Marshall L, Born J, Fehm HL. Slow wave sleep drives inhibition of pituitary–adrenal secretion in humans. J Neuroendocrinol 1997; 9: 479–484
- Born J, Fehm HL. Hypothalamus–pituitary–adrenal activity during human sleep: A coordinating role for the limbic hippocampal system. Exp Clin Endocrinol Diabetes 1998; 106: 153–163
- Born J, Gais S. REM sleep deprivation: The wrong paradigm leading to wrong conclusions. Behav Brain Sci 2000; 23: 912–913, Ref Type: Abstract
- Born J, Zwick A, Roth G, Fehm-Wolfsdorf G, Fehm HL. Differential effects of hydrocortisone, fluocortolone, and aldosterone on nocturnal sleep in humans. Acta Endocrinol (Copenh) 1987; 116: 129–137
- Born J, Spath-Schwalbe E, Schwakenhofer H, Kern W, Fehm HL. Influences of corticotropin-releasing hormone, adrenocorticotropin, and cortisol on sleep in normal man. J Clin Endocrinol Metab 1989; 68: 904–911
- Born J, Hansen K, Marshall L, Molle M, Fehm HL. Timing the end of nocturnal sleep. Nature 1999; 397: 29–30
- Born J, Rasch B, Gais S. Sleep to remember. Neuroscientist 2006; 12: 410–424
- Buchanan TW, Lovallo WR. Enhanced memory for emotional material following stress-level cortisol treatment in humans. Psychoneuroendocrinology 2001; 26: 307–317
- Buzsáki G. Memory consolidation during sleep: A neurophysiological perspective. J Sleep Res 1998; 7: 17–23
- Cahill L, McGaugh JL. Mechanisms of emotional arousal and lasting declarative memory. Trends Neurosci 1998; 21: 294–299
- Cahill L, Babinsky R, Markowitsch HJ, McGaugh JL. The amygdala and emotional memory. Nature 1995; 377: 295–296
- Charney DS, Deutch AY, Krystal JH, Southwick SM, Davis M. Psychobiologic mechanisms of posttraumatic stress disorder. Arch Gen Psychiatry 1993; 50: 295–305
- Cipolli C. Cognitive processes and sleep disturbances: Sleep, dreams and memory: An overview. J Sleep Res 1995; 4: 2–9
- Cirelli C, Tononi G. Differential expression of plasticity-related genes in waking and sleep and their regulation by the noradrenergic system. J Neurosci 2000; 20: 9187–9194
- De Kloet ER, Reul JM. Feedback action and tonic influence of corticosteroids on brain function: A concept arising from the heterogeneity of brain receptor systems. Psychoneuroendocrinology 1987; 12: 83–105
- De Kloet ER, Vreugdenhil E, Oitzl MS, Joels M. Brain corticosteroid receptor balance in health and disease. Endocr Rev 1998; 19: 269–301
- De Kloet ER, Joels M, Holsboer F. Stress and the brain: From adaptation to disease. Nat Rev Neurosci 2005; 6: 463–475
- de Quervain DJ, Roozendaal B, McGaugh JL. Stress and glucocorticoids impair retrieval of long-term spatial memory. Nature 1998; 394: 787–790
- de Quervain DJ, Roozendaal B, Nitsch RM, McGaugh JL, Hock C. Acute cortisone administration impairs retrieval of long-term declarative memory in humans. Nat Neurosci 2000; 3: 313–314
- Dimitrov S, Lange T, Nohroudi K, Born J. 2007. Number and function of circulating human antigen presenting cells regulated by sleep, in press, 00. Ref Type: Abstract
- Dodt C, Breckling U, Derad I, Fehm HL, Born J. Plasma epinephrine and norepinephrine concentrations of healthy humans associated with nighttime sleep and morning arousal. Hypertension 1997; 30: 71–76
- Dolcos F, LaBar KS, Cabeza R. Interaction between the amygdala and the medial temporal lobe memory system predicts better memory for emotional events. Neuron 2004; 42: 855–863
- Doyon J, Benali H. Reorganization and plasticity in the adult brain during learning of motor skills. Curr Opin Neurobiol 2005; 15: 161–167
- Drosopoulos S, Wagner U, Born J. Sleep enhances explicit recollection in recognition memory. Learn Mem 2005; 12: 44–51
- Eiser AS. Physiology and psychology of dreams. Semin Neurol 2005; 25: 97–105
- Ekstrand BR, Barrett TR, West JN, Meier WG. The effect of sleep on human long-term memory. Neurobiology of sleep and memory, RR Drucker-Colin, JL McGaugh. Academic Press, New York 1977; 419–438
- Ellenbogen JM. Cognitive benefits of sleep and their loss due to sleep deprivation. Neurology 2005; 64: E25–E27
- Elzinga BM, Bremner JD. Are the neural substrates of memory the final common pathway in posttraumatic stress disorder (PTSD)?. J Affect Disord 2002; 70: 1–17
- Ficca G, Lombardo P, Rossi L, Salzarulo P. Morning recall of verbal material depends on prior sleep organization. Behav Brain Res 2000; 112: 159–163
- Fischer S, Hallschmid M, Elsner AL, Born J. Sleep forms memory for finger skills. Proc Natl Acad Sci USA 2002; 99: 11987–11991
- Fischer S, Nitschke MF, Melchert UH, Erdmann C, Born J. Motor memory consolidation in sleep shapes more effective neuronal representations. J Neurosci 2005; 25: 11248–11255
- Fischer S, Drosopoulos S, Tsen J, Born J. Implicit learning—explicit knowing: A role for sleep in memory system interaction. J Cogn Neurosci 2006; 18: 311–319
- Fowler MJ, Sullivan MJ, Ekstrand BR. Sleep and memory. Science 1973; 179: 302–304
- Frank MG, Benington JH. The role of sleep in memory consolidation and brain plasticity: Dream or reality?. Neuroscientist 2006; 12: 477–488
- Friess E, Bardeleben V, Wiedemann K, Lauer CJ, Holsboer F. Effects of pulsatile cortisol infusion on sleep-EEG and nocturnal growth hormone release in healthy men. J Sleep Res 1994; 3: 73–79
- Fuller KH, Waters WF, Scott O. An investigation of slow-wave sleep processes in chronic PTSD patients. J Anxiety Disord 1994; 8: 227–236
- Gais S, Born J. Declarative memory consolidation: Mechanisms acting during human sleep. Learn Mem 2004; 11: 679–685
- Gais S, Lucas B, Born J. Sleep after learning aids memory recall. Learn Mem 2006; 13: 259–262
- Giuditta A, Ambrosini MV, Montagnese P, Mandile P, Cotugno M, Grassi ZG, et al. The sequential hypothesis of the function of sleep. Behav Brain Res 1995; 69: 157–166
- Glaubman H, Mikulincer M, Porat A, Wasserman O, Birger M. Sleep of chronic post-traumatic patients. J Trauma Stress 1990; 3: 255–263
- Hamann S. Cognitive and neural mechanisms of emotional memory. Trends Cogn Sci 2001; 5: 394–400
- Hamann SB, Ely TD, Grafton ST, Kilts CD. Amygdala activity related to enhanced memory for pleasant and aversive stimuli. Nat Neurosci 1999; 2: 289–293
- Heilig M, Koob GF, Ekman R, Britton KT. Corticotropin-releasing factor and neuropeptide Y: Role in emotional integration. Trends Neurosci 1994; 17: 80–85
- Hennevin E, Huetz C, Edeline JM. Neural representations during sleep: From sensory processing to memory traces. Neurobiol Learn Mem 2007; 87: 416–440
- Het S, Ramlow G, Wolf OT. A meta-analytic review of the effects of acute cortisol administration on human memory. Psychoneuroendocrinology 2005; 30: 771–784
- Hobson JA, Pace-Schott EF. The cognitive neuroscience of sleep: Neuronal systems, consciousness and learning. Nat Rev Neurosci 2002; 3: 679–693
- Horne JA, McGrath MJ. The consolidation hypothesis for REM sleep function: Stress and other confounding factors—a review. Biol Psychol 1984; 18: 165–184
- Horner HC, Packan DR, Sapolsky RM. Glucocorticoids inhibit glucose transport in cultured hippocampal neurons and glia. Neuroendocrinology 1990; 52: 57–64
- Hu P, Stylos-Allan M, Walker MP. Sleep facilitates consolidation of emotional declarative memory. Psychol Sci 2006; 17: 891–898
- Ji D, Wilson MA. Coordinated memory replay in the visual cortex and hippocampus during sleep. Nat Neurosci 2007; 10: 100–107
- Joels M, De Kloet ER. Control of neuronal excitability by corticosteroid hormones. Trends Neurosci 1992; 15: 25–30
- Joels M, De Kloet ER. Mineralocorticoid and glucocorticoid receptors in the brain. Implications for ion permeability and transmitter systems. Prog Neurobiol 1994; 43: 1–36
- Joels M, Hesen W, De Kloet ER. Long-term control of neuronal excitability by corticosteroid hormones. J Steroid Biochem Mol Biol 1995; 53: 315–323
- Johnson LR, Farb C, Morrison JH, McEwen BS, LeDoux JE. Localization of glucocorticoid receptors at postsynaptic membranes in the lateral amygdala. Neuroscience 2005; 136: 289–299
- Joiner WJ, Crocker A, White BH, Sehgal A. Sleep in Drosophila is regulated by adult mushroom bodies. Nature 2006; 441: 757–760
- Kanter ED, Wilkinson CW, Radant AD, Petrie EC, Dobie DJ, McFall ME, et al. Glucocorticoid feedback sensitivity and adrenocortical responsiveness in posttraumatic stress disorder. Biol Psychiatry 2001; 50: 238–245
- Kavushansky A, Richter-Levin G. Effects of stress and corticosterone on activity and plasticity in the amygdala. J Neurosci Res 2006; 84: 1580–1587
- Kirschbaum C, Wolf OT, May M, Wippich W, Hellhammer DH. Stress- and treatment-induced elevations of cortisol levels associated with impaired declarative memory in healthy adults. Life Sci 1996; 58: 1475–1483
- Korz V, Frey JU. Stress-related modulation of hippocampal long-term potentiation in rats: Involvement of adrenal steroid receptors. J Neurosci 2003; 23: 7281–7287
- Kuhlmann S, Kirschbaum C, Wolf OT. Effects of oral cortisol treatment in healthy young women on memory retrieval of negative and neutral words. Neurobiol Learn Mem 2005; 83: 158–162
- Lange T, Perras B, Fehm HL, Born J. Sleep enhances the human antibody response to hepatitis A vaccination. Psychosom Med 2003; 65: 831–835
- Lange T, Dimitrov S, Fehm HL, Westermann J, Born J. Shift of monocyte function toward cellular immunity during sleep. Arch Intern Med 2006; 166: 1695–1700
- Lechin F, Pardey-Maldonado B, van der DB, Benaim M, Baez S, Orozco B, et al. Circulating neurotransmitters during the different wake-sleep stages in normal subjects. Psychoneuroendocrinology 2004; 29: 669–685
- LeDoux JE. Emotion circuits in the brain. Annu Rev Neurosci 2000; 23: 155–184
- Lupien SJ, McEwen BS. The acute effects of corticosteroids on cognition: Integration of animal and human model studies. Brain Res Rev 1997; 24: 1–27
- Lupien SJ, Wilkinson CW, Briere S, Menard C, Ng Ying Kin NM, Nair NP. The modulatory effects of corticosteroids on cognition: Studies in young human populations. Psychoneuroendocrinology 2002a; 27: 401–416
- Lupien SJ, Wilkinson CW, Briere S, Ng Ying Kin NM, Meaney MJ, Nair NP. Acute modulation of aged human memory by pharmacological manipulation of glucocorticoids. J Clin Endocrinol Metab 2002b; 87: 3798–3807
- Maheu FS, Joober R, Beaulieu S, Lupien SJ. Differential effects of adrenergic and corticosteroid hormonal systems on human short- and long-term declarative memory for emotionally arousing material. Behav Neurosci 2004; 118: 420–428
- Maquet P, Peters J, Aerts J, Delfiore G, Degueldre C, Luxen A, et al. Functional neuroanatomy of human rapid-eye-movement sleep and dreaming. Nature 1996; 383: 163–166
- Maquet P, Laureys S, Peigneux P, Fuchs S, Petiau C, Phillips C, et al. Experience-dependent changes in cerebral activation during human REM sleep. Nat Neurosci 2000; 3: 831–836
- Marshall L, Molle M, Hallschmid M, Born J. Transcranial direct current stimulation during sleep improves declarative memory. J Neurosci 2004; 24: 9985–9992
- Marshall L, Helgadottir H, Molle M, Born J. Boosting slow oscillations during sleep potentiates memory. Nature 2006; 444: 610–613
- Mason JW, Giller EL, Kosten TR, Ostroff RB, Podd L. Urinary free-cortisol levels in posttraumatic stress disorder patients. J Nerv Ment Dis 1986; 174: 145–149
- Maurizi CP. The function of dreams (REM sleep): Roles for the hippocampus, melatonin, monoamines, and vasotocin. Med Hypotheses 1987; 23: 433–440
- McGaugh JL. Memory—a century of consolidation. Science 2000; 287: 248–251
- McGaugh JL. The amygdala modulates the consolidation of memories of emotionally arousing experiences. Annu Rev Neurosci 2004; 27: 1–28
- McGaugh JL, Roozendaal B. Role of adrenal stress hormones in forming lasting memories in the brain. Curr Opin Neurobiol 2002; 12: 205–210
- Mölle M, Yeshenko O, Marshall L, Sara SJ, Born J. Hippocampal sharp wave-ripples linked to slow oscillations in rat slow-wave sleep. J Neurophysiol 2006; 96: 62–70
- Müller GE, Pilzecker A. Experimentelle Beiträge zur Lehre vom Gedächtnis. Z Psychol Ergänzungsbd 1900; 1: 1–300
- Neylan TC, Lenoci M, Maglione ML, Rosenlicht NZ, Metzler TJ, Otte C, et al. Delta sleep response to metyrapone in post-traumatic stress disorder. Neuropsychopharmacology 2003; 28: 1666–1676
- Nofzinger EA, Mintun MA, Wiseman M, Kupfer DJ, Moore RY. Forebrain activation in REM sleep: An FDG PET study. Brain Res 1997; 770: 192–201
- Orban P, Rauchs G, Balteau E, Degueldre C, Luxen A, Maquet P, et al. Sleep after spatial learning promotes covert reorganization of brain activity. Proc Natl Acad Sci USA 2006; 103: 7124–7129
- Pavlides C, McEwen BS. Effects of mineralocorticoid and glucocorticoid receptors on long-term potentiation in the CA3 hippocampal field. Brain Res 1999; 851: 204–214
- Pavlides C, Kimura A, Magarinos AM, McEwen BS. Hippocampal homosynaptic long-term depression/depotentiation induced by adrenal steroids. Neuroscience 1995; 68: 379–385
- Pavlides C, Ogawa S, Kimura A, McEwen BS. Role of adrenal steroid mineralocorticoid and glucocorticoid receptors in long-term potentiation in the CA1 field of hippocampal slices. Brain Res 1996; 738: 229–235
- Paz R, Pelletier JG, Bauer EP, Pare D. Emotional enhancement of memory via amygdala-driven facilitation of rhinal interactions. Nat Neurosci 2006; 9: 1321–1329
- Peigneux P, Laureys S, Delbeuck X, Maquet P. Sleeping brain, learning brain. The role of sleep for memory systems. Neuroreport 2001; 12: A111–A124
- Peigneux P, Laureys S, Fuchs S, Destrebecqz A, Collette F, Delbeuck X, et al. Learned material content and acquisition level modulate cerebral reactivation during posttraining rapid-eye-movements sleep. Neuroimage 2003; 20: 125–134
- Peigneux P, Laureys S, Fuchs S, Collette F, Perrin F, Reggers J, et al. Are spatial memories strengthened in the human hippocampus during slow wave sleep?. Neuron 2004; 44: 535–545
- Phelps EA. Human emotion and memory: Interactions of the amygdala and hippocampal complex. Curr Opin Neurobiol 2004; 14: 198–202
- Pitman RK. Post-traumatic stress disorder, hormones, and memory. Biol Psychiatry 1989; 26: 221–223
- Plihal W, Born J. Effects of early and late nocturnal sleep on declarative and procedural memory. J Cogn Neurosci 1997; 9: 534–547
- Plihal W, Born J. Effects of early and late nocturnal sleep on priming and spatial memory. Psychophysiology 1999a; 36: 571–582
- Plihal W, Born J. Memory consolidation in human sleep depends on inhibition of glucocorticoid release. Neuroreport 1999b; 10: 2741–2747
- Plihal W, Pietrowsky R, Born J. Dexamethasone blocks sleep induced improvement of declarative memory. Psychoneuroendocrinology 1999; 24: 313–331
- Rasch B, Büchel C, Gais S, Born J. Odor cues during slow-wave sleep prompt declarative memory consolidation. Science 2007; 315: 1426–1429
- Rechtschaffen A, Kales A. A manual of standardized terminology, techniques and scoring system for sleep stages of human subjects. N.I.H, Maryland 1968, Publication No. 204
- Ribeiro S, Gervasoni D, Soares ES, Zhou Y, Lin SC, Pantoja J, et al. Long-lasting novelty-induced neuronal reverberation during slow-wave sleep in multiple forebrain areas. PLoS Biology 2004; 2: E24
- Rohleder N, Joksimovic L, Wolf JM, Kirschbaum C. Hypocortisolism and increased glucocorticoid sensitivity of pro-inflammatory cytokine production in Bosnian war refugees with posttraumatic stress disorder. Biol Psychiatry 2004; 55: 745–751
- Roozendaal B. Glucocorticoids and the regulation of memory consolidation. Psychoneuroendocrinology 2000; 25: 213–238
- Roozendaal B, Brunson KL, Holloway BL, McGaugh JL, Baram TZ. Involvement of stress-released corticotropin-releasing hormone in the basolateral amygdala in regulating memory consolidation. Proc Natl Acad Sci USA 2002; 99: 13908–13913
- Sauro MD, Jorgensen RS, Pedlow CT. Stress, glucocorticoids, and memory: A meta-analytic review. Stress 2003; 6: 235–245
- Sejnowski TJ, Destexhe A. Why do we sleep?. Brain Res 2000; 886: 208–223
- Sirota A, Csicsvari J, Buhl D, Buzsáki G. Communication between neocortex and hippocampus during sleep in rodents. Proc Natl Acad Sci USA 2003; 100: 2065–2069
- Southwick SM, Davis M, Horner B, Cahill L, Morgan CA, III, Gold PE, et al. Relationship of enhanced norepinephrine activity during memory consolidation to enhanced long-term memory in humans. Am J Psychiatry 2002; 159: 1420–1422
- Späth-Schwalbe E, Gofferje M, Kern W, Born J, Fehm HL. Sleep disruption alters nocturnal ACTH and cortisol secretory patterns. Biol Psychiatry 1991; 29: 575–584
- Späth-Schwalbe E, Uthgenannt D, Voget G, Kern W, Born J, Fehm HL. Corticotropin-releasing hormone-induced adrenocorticotropin and cortisol secretion depends on sleep and wakefulness. J Clin Endocrinol Metab 1993; 77: 1170–1173
- Squire LR. Memory and the hippocampus: A synthesis from findings with rats, monkeys, and humans. Psychol Rev 1992; 99: 195–231
- Steiger A. Sleep and endocrine regulation. Front Biosci 2003; 8: s358–s376
- Sterpenich V, Albouy G, Boly G, Darsaud A, Balteau P, Peigneux P, et al. The role of sleep in the consolidation of emotional memories in humans: A fMRI study. J Sleep Res 2006; 15: 190
- Stickgold R. Sleep-dependent memory consolidation. Nature 2005; 437: 1272–1278
- Stickgold R, Scott L, Rittenhouse C, Hobson JA. Sleep-induced changes in associative memory. J Cogn Neurosci 1999; 11: 182–193
- Sutherland GR, McNaughton B. Memory trace reactivation in hippocampal and neocortical neuronal ensembles. Curr Opin Neurobiol 2000; 10: 180–186
- Tononi G, Cirelli C. Sleep function and synaptic homeostasis. Sleep Med Rev 2006; 10: 49–62
- van der Kolk BA. The body keeps the score: Memory and the evolving psychobiology of posttraumatic stress. Harv Rev Psychiatry 1994; 1: 253–265
- vanOyen WC. Traumatic intrusive imagery as an emotional memory phenomenon: A review of research and explanatory information processing theories. Clin Psychol Rev 1997; 17: 509–536
- Wagner U, Gais S, Born J. Emotional memory formation is enhanced across sleep intervals with high amounts of rapid eye movement sleep. Learn Mem 2001; 8: 112–119
- Wagner U, Fischer S, Born J. Changes in emotional responses to aversive pictures across periods rich in slow-wave sleep versus rapid eye movement sleep. Psychosom Med 2002; 64: 627–634
- Wagner U, Gais S, Haider H, Verleger R, Born J. Sleep inspires insight. Nature 2004; 427: 352–355
- Wagner U, Degirmenci M, Drosopoulos S, Perras B, Born J. Effects of cortisol suppression on sleep-associated consolidation of neutral and emotional memory. Biol Psychiatry 2005; 58: 885–893
- Wagner U, Hallschmid M, Rasch B, Born J. Brief sleep after learning keeps emotional memories alive for years. Biol Psychiatry 2006; 60: 788–790
- Walker MP, Brakefield T, Morgan A, Hobson JA, Stickgold R. Practice with sleep makes perfect: Sleep-dependent motor skill learning. Neuron 2002; 35: 205–211
- Walker MP, Brakefield T, Hobson JA, Stickgold R. Dissociable stages of human memory consolidation and reconsolidation. Nature 2003; 425: 616–620
- Wilhelm I, Born J, Kudielka BM, Schlotz W, Wüst S. Is the cortisol awakening rise a response to awakening?. Psychoneuroendocrinology 2007; 32: 358–366, (in press)
- Wilson MA, McNaughton BL. Reactivation of hippocampal ensemble memories during sleep. Science 1994; 265: 676–679
- Wixted JT. On common ground: Jost's (1897) law of forgetting and Ribot's (1881) law of retrograde amnesia. Psychol Rev 2004; 111: 864–879
- Yaroush R, Sullivan MJ, Ekstrand BR. Effect of sleep on memory. II. Differential effect of the first and second half of the night. J Exp Psychol 1971; 88: 361–366
- Yehuda R. Post-traumatic stress disorder. N Engl J Med 2002; 346: 108–114
- Yehuda R, Southwick SM, Nussbaum G, Wahby V, Giller EL, Jr., Mason JW. Low urinary cortisol excretion in patients with posttraumatic stress disorder. J Nerv Ment Dis 1990; 178: 366–369
- Yehuda R, Boisoneau D, Lowy MT, Giller EL, Jr. Dose-response changes in plasma cortisol and lymphocyte glucocorticoid receptors following dexamethasone administration in combat veterans with and without posttraumatic stress disorder. Arch Gen Psychiatry 1995; 52: 583–593
- Yehuda R, Teicher MH, Trestman RL, Levengood RA, Siever LJ. Cortisol regulation in posttraumatic stress disorder and major depression: A chronobiological analysis. Biol Psychiatry 1996; 40: 79–88