ABSTRACT
The rise of 3D printing allows unprecedented customization of rehabilitation devices, and with an ever-expanding library of 3D printable (3DP) materials, the spectrum of attenable rehabilitation devices is likewise expanding. The current pilot study explores feasibility of using 3DP elastic materials to create dynamic hand orthoses for stroke survivors. A dynamic orthosis featuring a replaceable finger component was fabricated using 3DP elastic materials. Duplicates of the finger component were printed using different materials ranging from low stiffness (low elastic modulus) to relatively high stiffness (high elastic modulus). Five stroke survivors with predominantly moderate hand impairment were recruited to evaluate usability and impact of orthoses on upper extremity function and biomechanics. No significant differences in usability were found between 3D-printed orthoses and a commercial orthosis. Increases in stiffness of the 3DP material reduced pincer force (p = .0041) and the BBT score (p = .043). In comparison, the commercial orthosis did not reduce pincer force but may reduce BBT score to a degree that is clinically significant (p = .0002). While preliminary, these findings suggest that a dynamic orthosis is a feasible clinical application of 3DP elastic materials, and future study is warranted.
Introduction
Among nearly 800,000 new strokes which occur in the USA annually, approximately 85% of cases involve debility in the hand (Benjamin et al., Citation2017; Lawrence et al., Citation2001; Parker et al., Citation1986). This debility is consequent to common post-stroke impairments (e.g., paralysis of the limb, diminished sensation, and abnormal muscle tone) (Raghavan, Citation2015). Chronic debility remains in nearly half of cases, and this is directly associated with reduction in quality of life (Broeks et al., Citation1999; Desrosiers et al., Citation2006; Morris et al., Citation2013).
After a stroke, hand rehabilitation paradigm relies increasingly on technology as evidenced by recent guidelines (Billinger et al., Citation2014; Dobkin, Citation2005; Winstein et al., Citation2016). This is further demonstrated by numerous device-based research studies exploring wearable sensors, exoskeletons, and soft robotics (Duret et al., Citation2019; Held et al., Citation2018; Miller et al., Citation2009; Nuckols et al., Citation2020; Oliveira et al., Citation2019; Polygerinos et al., Citation2015). This technology reliance is likely due to stroke survivors living longer, growth of the at-risk population, limited reimbursement of in-person therapies, and growing evidence that more therapy is needed (Rinere O’brien, Citation2010; United Nations, Citation2019; Q. Yang et al., Citation2017).
Dynamic hand orthoses are a rehabilitation technology that may offer many benefits to stroke survivors, and recent reviews emphasize a need for future large-scale studies on this technology (Alexander et al., Citation2022). However, the research on and the clinical uptake of rehabilitation technology are complicated by mismatches between the device design and the user needs – mismatches that play a central role to device abandonment (van Ommeren et al., Citation2018). Among all assistive devices, abandonment rates of 30% exist (Riemer-Reiss & Wacker, Citation2000). For upper extremity devices in stroke rehabilitation, the rate is approximately doubled (Safaz et al., Citation2015).
Iterative design of technology is recognized for reducing mismatched designs (Meyer et al., Citation2022; van Ommeren et al., Citation2018). Iterations, however, contribute cost to product development (Money et al., Citation2011; Shah & Robinson, Citation2007). Iterative design at reduced cost is motivation for additive manufacturing of rehabilitation technology.
Additive manufacturing with 3D printable (3DP) materials offers rapid fabrication of complex geometries, cost-effective part customization, reduction of part counts, and decentralized manufacturing (Barrios-Muriel et al., Citation2020; S. Yang et al., Citation2015). Research on the clinical feasibility of 3DP hand devices has largely concentrated on rigid devices (Blaya et al., Citation2018; Fernandez-Vicente et al., Citation2017; Gabriele et al., Citation2016; Kim & Jeong, Citation2015; Paterson et al., Citation2015; Portnoy et al., Citation2020). Despite evidence in stroke literature supporting movement-based therapy (Kwakkel et al., Citation2015; Sawaki et al., Citation2008; Winstein et al., Citation2016; S. L. Wolf et al., Citation2008), the translation of 3DP techniques beyond rigid hand devices has been limited. Elastic materials can stretch and deform when subject to external forces, can passively store energy, and can return to their original shape when those forces are removed. The rise of 3DP elastic materials is a promising link to innovative 3DP devices that foster movement-based rehabilitation.
Objectives
In this pilot study, we examined the clinical feasibility of a rehabilitation device developed using 3DP elastic materials. This was a dynamic wrist–hand–finger orthosis (WHFO) with finger-extension assist – a prototype device developed in-house by our team for individuals with a history of stroke. We considered five different 3DP elastic materials for the finger-extension-assist feature. The primary objective of the study was to assess usability of this prototype dynamic WHFO among persons with a history of stroke and compare with a commercially available dynamic WHFO. As a secondary objective, we examined how each 3DP elastic material, when incorporated to the prototype dynamic WHFO, impacted hand biomechanics and upper extremity function of the users. We seek to build a foundation for innovative orthotic designs that promote movement-based hand therapies for stroke survivors.
Methods
Study design and participants
This pilot study featured a mixed-methods, repeated-measures study design. Five individuals were recruited based on a history of stroke leading to impairment of an upper extremity, and participant demographics are shown in . Inclusion criteria were history of subacute or chronic stroke, age 18–80 years, ability to actively extend wrist on affected upper extremity to maintain neutral position, and ability to create pincer grasp using thumb and index finger of affected upper extremity. Exclusion criteria were prior disease significantly impacting hand dexterity (e.g., osteoarthritis and trauma), inability to read/understand instruction in English, prior history of substance or alcohol use disorder, or cognitive deficits as determined be the Mini-Cog test (Borson et al., Citation2005). Prior to data collection, all participants gave informed consent according to the procedures reviewed and approved by the University of Kentucky Institutional Review Board. Additionally, all participants completed the Stroke Impact Scale (SIS) prior to data collection, which is a standardized questionnaire that elicits participant perceptions on their recovery in several broad categories including hand function (SISHand).
Table 1. Participant demographics.
Dynamic wrist–hand–finger orthoses
Our study examined a prototype dynamic WHFO as well as a comparable off-the-shelf orthosis. Fabricated using 3DP materials, the prototype dynamic WHFO comprised a simple two-component assembly to achieve a finger-extension-assist feature as shown in . Assistance to finger extension is a common feature for such dynamic orthoses as the literature on post-stroke muscle activation has shown more profound deficits in the extensor versus flexor muscles of affected hand (Hoffmann et al., Citation2015). Prior devices achieve this feature using a variety of wrist-worn apparatuses and tension systems (Chang & Lai, Citation2015; Hoffman et al., Citation2011). In this study, a commercially available device was selected (SaeboGlove, Saebo Inc., Charlotte, NC, USA) as a comparator to the prototype.
Figure 1. Dynamic wrist–hand–finger orthosis. Rendering of prototype orthosis on the left and fabrications of orthosis on the right with five different finger-extension components fabricated using 3DP elastic materials from commercial vendors: (i) thermoplastic polyurethane by Shapeways Holdings, Inc.; (ii) elastomeric polyurethane by Shapeways Holdings, inc.; (iii) thermoplastic polyurethane by Sculpteo; (iv) elastomeric polyurethane by Sculpteo; and (v) thermoplastic polyurethane by i.Materialize N.V.
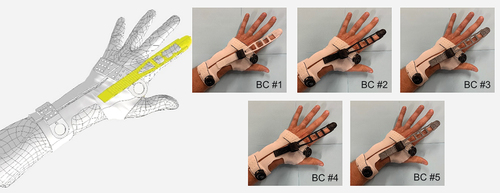
For the prototype, the base wrist component was fabricated by selective laser sintering of a thermoplastic polyurethane (TPU) powder (Pan et al., Citation2020). The finger-extension-assist feature relied on an elastic element applied to the dorsal surface of the index finger – an element referred to as the finger-extension component. For the fully assembled orthosis, the finger-extension component length was set to minimize perceived tension and strain when hand placed palm-down on a flat table with all fingers fully extended to the degree possible without discomfort. This finger-extension component was physically duplicated to create five versions (BC #1 through BC #5) with identical geometries but unique 3DP material composition. The materials were selected based on commercial availability from prominent 3D printing bureaus and are summarized in . The selected materials represented an elastic modulus ranging between 3.2 (low stiffness) and 45.8 MPa (relatively high stiffness).
Table 2. Commercial 3DP elastic materials for the dynamic WHFO.
The elastic modulus was measured using a tensile testing machine (Instron 5kN, Illinois Tool Works Inc., Norwood, MA, USA). This was done because the mechanical properties of plastics are known to change during processing (Zhu & Yang, Citation2020). For in-house tensile testing, 3DP material specimens were ordered from the manufacturers based on the dogbone specimen design, which is standardized for elastic materials (American Society for Testing and Materials, Citation2021). Of note, the 3DP dogbone specimens were ordered intentionally at the same time as the 3DP orthosis parts to promote consistency between the material undergoing tensile testing and the material assessed in the pilot study. For each 3DP material, five dogbone specimens were ordered and tested, and the average value served as the in-house measurement of elastic modulus ().
Data collection
This study comprised data from a usability survey, biomechanical tests, and a clinical assessment of hand function, and all data acquisition occurred at the University of Kentucky Stroke and Spinal Cord Rehabilitation Research Laboratory, which is housed inside a free-standing inpatient rehabilitation facility.
The usability survey featured a total of 10 Likert-scale questions patterned after the Technology Acceptance Model (Davis & Davis, Citation1989). With regard to the commercial brace and with regard to the orthotic prototypes collectively, participants were asked to rate the following items on a 10-point scale with lower scores reflecting more positive perception: intention-to-use, ease-of-use, intuitiveness, comfort, and esthetics. Additionally, the participants were prompted with open-response questions to identify and provide supporting commentary regarding the orthosis they liked least and most.
A battery of tests was used to evaluate the participant’s hand biomechanics and upper extremity functions. Included tests were the pincer force test, pincer aperture test, and the box and block test (BBT) (Bae et al., Citation2015; Paterson et al., Citation2015; Platz et al., Citation2005). Each participant completed the test battery for six different orthosis conditions: once without the orthosis (representing a baseline), once for each configuration of the prototype orthosis (each configuration defined by a unique elastic material for the finger-extension component), and once with an off-the-shelf brace. While the test battery was executed in a fixed sequence, the order of the orthosis conditions was randomized for each participant.
During the pincer force test, the participants were instructed to apply a pincer grasp to a calibrated piezoelectric gauge (Model 13 Subminiature Load Cell, Honeywell Inc, Charlotte, NC, USA) with a goal to create maximum force during an allotted 2-s duration. The pincer force test was repeated for five total trials for each participant; between trials, the participant was provided a 30-s rest period during which a tare function was applied to the piezoelectric gauge. Force data were collected at a sampling rate of 200 hertz (Hz) and was subsequently low-pass filtered at 5 Hz cutoff frequency using a fourth-order bidirectional Butterworth filter (Gao et al., Citation2007). During data collection, both the force magnitude as measured in Newtons and the time elapsed were monitored using a custom Labview program with real-time data displayed as visual feedback to participants. If non-pincer grasp observed (e.g., lateral pinch or tripod pinch), the investigator provided instruction and measurements were repeated with corrected grasp.
For pincer aperture, quantified in centimeters (cm), the participants were asked to use a pincer grasp to pick up a series of progressively larger plastic blocks (1 cm to 10 cm wide cubic blocks in increments of 0.5 cm). No limitation on time was instituted. To limit compensatory maneuvers, an observer evaluated the participant’s grasp for inadvertent tripod grasp or lateral pinch and provided verbal feedback. The size of the largest block, in cm, that was successfully picked up by the patient was recorded as the pincer aperture outcome. Participant repeated this assessment for a total of five repetitions for each orthosis condition with rest breaks between.
For the BBT, an individual must transfer wooden blocks from one box container, across a partition, to another box container. The BBT is a validated clinical tool used to assess upper extremity function and hand dexterity (J. Desrosiers et al., Citation1994; Lin et al., Citation2010). The test duration is 60 s, and the outcome score equals the number of times the participant successfully moves the hand across the partition to transfer a wooden block. The setups used for pincer force measurement, the pincer aperture test, and the BBT are depicted in .
Statistical approach
Ordinal data from the usability survey were analyzed using non-parametric signed-rank test. For open-response questions, the responses from participants were reviewed to identify shared elements and to highlight perspectives relevant to usability. For each outcome of hand biomechanics and upper extremity function, the data were analyzed using two separate repeated-measures analysis of covariance models: (i) one model comparing braces as a categorical variable and (ii) one model comparing braces according to a continuous variable – the measured modulus of elasticity associated with the brace material. For the first model, when a significant effect was seen over all braces, then the effect of each individual brace was compared with respect to the no-brace condition (BC). Additionally, for the first model, subgroup analyses were performed which examined the 3DP BCs grouped by prototype (e.g., prototypes as a collective group), by manufacturer, by the material type, and by specific fabrication method. Of note, while the first model included all BCs as factors, the second model excluded the commercial brace as a factor as its multi-material composition could not readily be reduced to a singular elastic modulus value. Conversely, the baseline condition (no brace) was included in the second model by defining its modulus of elasticity as 0 mPA. For all models, the participant demographics, including age, time since stroke, SISHand, and a dichotomous variable whether stroke-affected side was dominant side, were included as factors to determine potential effects on the measured outcomes. All models use a compound symmetry correlation structure. Significance was indicated in all models if p < .05 and no correction for multiple comparisons was performed given the preliminary nature of this pilot study. Due to non-normality of the force outcome, a square root transformation was used for the models. For aperture, a transformation was used to convert the score to a value between 0 and 1, and a beta generalized linear mixed model was used.
Results
Usability
There were no significant differences between the 3DP prototype braces and the commercial brace for any of the usability categories as shown in . Based on the open-response comments, two of the participants indicated a preference for the commercial brace, two indicated a preference for the prototype brace, and one participant indicated that the prototype was both the most and least preferred.
Table 3. Usability survey results for the dynamic WHFO.
Hand biomechanics and function
Mean (SD) of the maximum pincer force for all participants and orthosis conditions is summarized in . Overall, when considering BC as a categorical factor, the difference in the maximum pincer force measured across braces was significant (p = .0486). More specifically, BC #1 (TPU via SLS by Shapeways), BC #3 (TPU via MJP by Sculpteo), and BC #5 (TPU via MJP by i.Materialize) (p = .0091, p = .0363, and p = .0090, respectively) were found to cause a significant decrease in the maximum pincer force as compared to no brace. Subgroup analyses in this model revealed a significant negative effect when comparing the prototypes as a collective group to the baseline condition (p = .0118); otherwise, the subgroup analyses were without significant findings. Furthermore, when considering the quantifiable elasticity of the orthoses as a continuous factor, increases in modulus of elasticity (increased stiffness) resulted in a decrease in pincer force (p = .0041). The same two participant demographics were found to be significant factors in all models. The participant’s self-reported baseline hand function as determined by SISHand contributed a significant effect (p ≤ .0005)—that is, higher self-reported hand function predicted higher pincer force. Regarding hand dominance, if the participant’s non-dominant hand was affected by the stroke, then a significant inverse effect on pincer force was found (p ≤ .02).
Figure 4. Maximum pincer force for each participant and orthosis condition. Note baseline on the left and commercial brace on the right and 3DP prototype brace listed in order of increasing stiffness.
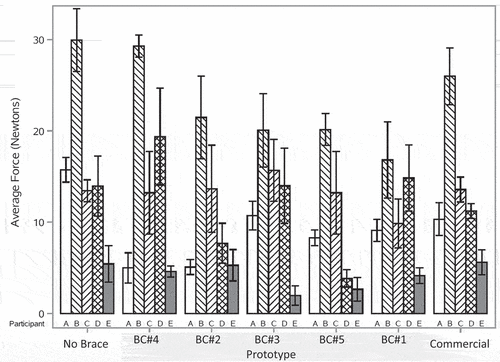
For pincer aperture, when treating BC as a categorical factor, a significant effect was found (p = .03). When treating elasticity of the brace as a continuous factor, there was no significant effect. Regarding participant demographics, the time since stroke occurrence was found to be significant factor to the pincer aperture (p < .0001); other demographic factors did not reach significance. Additional analysis was not possible due to lack of convergence of the model, which is a topic of discussion.
The BBT results for all participants and BCs are shown in . When treating BC as a categorical factor, a significant effect was found (p = .0129), and in subsequent analysis comparing each individual brace to the baseline (no brace), a significant negative effect was found for BC #1 (TPU via SLS by Shapeways), BC #3 (TPU via MJP by Sculpteo), BC #5 (TPU via MJP by i.Materialize), and commercial brace (p = .0164, p = .0255, p = .0480, and p = .0002, respectively). Subgroup analyses revealed significant negative effect when comparing prototype versus baseline condition (p = .0107) and significant positive effect when comparing prototype versus commercial (p = .0062); otherwise, no significant effects were seen when comparing across manufacturers, across materials types, or across 3D printing methods. When treating elasticity of the brace as a continuous factor, increases in modulus of elasticity (increased stiffness) resulted in a significant decrease in the BBT score (p = .043). None of the participant demographics were found to be significant factors to the BBT score.
Discussion
This preliminary study investigated different 3DP elastic materials used to fabricate a dynamic finger-extension-assist WHFO for stroke survivors. Our primary objective was to assess the feasibility of applying these materials to this orthotic device, and our secondary objective was to understand the impact of elastic material selection on the end user’s biomechanics (pincer aperture/force) and hand function (BBT). Based on usability compared to a commercial orthosis, a dynamic WHFO created with 3DP elastic materials appears feasible, which was expected. For 3DP materials with higher elastic modulus, a greater inhibition to pincer force was seen (decreased pincer force), which was expected. However, with higher elastic modulus, a lack of effect on pincer aperture was observed, which differed from our expectation. We additionally expected hand function to improve (increased BBT score) as elastic modulus increased, but the opposite effect was observed. Interestingly, use of the commercial brace reduced hand function more profoundly compared to the prototype dynamic WHFO. While these findings should be considered preliminary, they provide motivation for confirmatory studies and impetus for future exploratory studies of 3DP elastic materials.
In reviewing the participants’ commentary to open-response questions on the usability survey, a common drawback of the prototype was its omission of features compared to the commercial brace. Given the intentional simplicity of its initial design, a lack of perceived features is not surprising. For future prototype iterations, the addition of features may improve the end users perceptions, and more specifically, an ability to customize features may improve perceptions and long-term acceptance according to user-centered design literature (Meyer et al., Citation2022; van Ommeren et al., Citation2018). Perhaps reassuring, the primary design feature of the prototype – assistance of index finger extension – garnered few comments except for the tactile sensory loss that one participant attributed to the gloved fingertip. This was not unique to the prototype, however, as another participant commented that the material thickness of the commercial brace fingertips was a hindrance to picking up objects. Given the known importance of sensory afferent signals to motor control and recovery, addressing this concern in future prototype iterations would be prudent (Augurelle et al., Citation2003; Ridding et al., Citation2005; Shim et al., Citation2012).
Compared to baseline (no brace), a significant inverse effect on pincer force was found for the orthosis conditions BC #1, BC #3, and BC #5. That is, these braces predicted a significant reduction in the maximum pincer force achieved by the participant. Surprisingly, the commercial brace was found to have no significant effect on pincer grasp force. The similar effects among the three prototype orthosis conditions may be directly linked to an obvious similarity – all these conditions involve TPU materials.
An explanation behind the uniformity of TPU’s impact on pincer force may be revealed by considering material properties. In this study, measurable increases in stiffness (increased elastic modulus) of the 3DP materials were found to have an inverse effect on pincer force. This is reassuring given the design intent of the prototype to assist index finger extension through an elastic apparatus along the dorsal finger surface. Conceivably, this counteracts the finger flexors and ultimately reduces the net metacarpophalangeal and interphalangeal joint torques contributing to pincer force. Given the relatively high stiffness of TPU among tested materials in this study, a simple but important connection surfaces – the reduced pincer force observed for TPU is due in part to the relatively high stiffness (high elastic modulus) of TPU.
An unexpected finding from this study regarded the effects of orthosis conditions on the BBT score. When compared to baseline, a significant decrease in BBT score was found for the BC #1, BC #3, and BC #5 orthosis conditions (all comprising TPU) as well as for the commercial brace. In fact, for the latter, this effect appeared to be far more profound as evidenced by beta coefficients. That is, keeping all other factors equal, the use of the commercial brace predicted nine fewer blocks transferred as compared to no brace. This exceeds the minimal clinically important difference for the BBT (seven blocks) (Liang et al., Citation2021). In contrast, the use of a prototype brace predicted roughly four fewer blocks transferred as compared to no brace, which does not reach clinical significance. The explanation for this finding is not clear and should be interpreted cautiously given the preliminary nature of this pilot study. The adverse impact of the braces on the BBT may be secondary to the participants’ unfamiliarity with using the braces.
Unfortunately, the aperture outcome measure faced an obvious ceiling effect, which limited analysis. Of the five participants, only one exhibited substantial variability in the aperture test, and this same participant may be considered the outlier given their prolonged time since stroke (greater than 120 months) and the severity of their hand function (SISHand of 20), both of which were extremes for the group. In fact, if this participant removed, insufficient variance remained for the analysis.
During the course of this study, none of the participants reported any limitations to study participation due to pain, and no significant adverse events were observed in this study. For all the 3DP elastic materials, the availability of certifications of biocompatibility was confirmed, and no instances of skin irritation were observed during this pilot study (D Printing Solution, Citation2020; D, Citation2021; Electro Optical Systems, Citation2021).
Limitations and opportunities
Several important limitations are worth noting for this pilot study. The small sample size prevents high-powered findings, and the lack of longitudinal data hinders further analysis on device acceptance, safety, and clinical effects. While participant perceptions were captured, additional perceptions from other devices’ stakeholders would be desirable including clinicians, caregivers, and insurers. Regarding the prototype, efforts to maintain geometric equivalence among the duplicates were likely impacted by variations in vendor quality control, which could impact material properties (e.g., elastic modulus and Poisson’s ratio). Additionally, more material properties could be explored. Material surface texture and thermal conduction may impart unique sensory afferent information to the user – a scenario that could impact motor control leading to facilitation or inhibition of the user’s pincer force (Augurelle et al., Citation2003; Ridding et al., Citation2005; Shim et al., Citation2012). Regarding data collection, visual monitoring of the participant’s grasp was challenging, and there is a possibility that alternative grasps were used but not seen by the monitor (e.g., lateral pinch and tripod pinch). Future studies would benefit from alternative monitoring techniques (e.g., pressure mapping of the participant’s fingertips). With regard to the pincer aperture data, a ceiling effect was obvious and future efforts might benefit by modifying the assessment (e.g., larger block sizes) or by kinematic assessment with motion capture. Additionally, while the test battery includes validated assessments in stroke population, additional assessments may be helpful. For example, other assessments of upper extremity function include a wider variety of task-based activities, which may better portray a subject’s true function (Lang et al., Citation2006; S. Wolf et al., Citation2001).
Conclusions
Based on preliminary evidence of this pilot study, a dynamic WHFO for stroke survivors may be feasible using 3DP elastic materials. Such devices may present advantages compared to the current commercially available competitors, and further study is needed to confirm and explore such advantages.
Author contributions
Study design and planning was led by JH. Data acquisition and manuscript preparation were conducted by JH. Data analysis was performed by SS, and data interpretation was led by JH with review by BB. Manuscript revisions were performed by JH, BB, and SS. All authors approved the final manuscript.
Consent for publication
Consent authorizing release of information was obtained from each participant and secured.
Ethical approval and informed consent
Research has been performed in accordance with the Declaration of Helsinki and has been approved by the University of Kentucky Institutional Review Board (IRB# 77375). Informed consent authorizing participation was obtained from each participant and secured.
Acknowledgments
We thank the anonymous reviewers for their constructive feedback during the course of this manuscript creation.
Disclosure statement
No potential conflict of interest was reported by the author(s).
Data availability statement
The datasets generated and analyzed during current study are available from the corresponding author upon reasonable request.
Additional information
Funding
References
- Alexander, J., Dawson, J., & Langhorne, P. (2022). Dynamic hand orthoses for the recovery of hand and arm function in adults after stroke: A systematic review and meta-analysis of randomised controlled trials. Topics in Stroke Rehabilitation, 29(2), 114–124. https://doi.org/10.1080/10749357.2021.1878669
- American Society for Testing and Materials. (2021). Standard test methods for vulcanized rubber and thermoplastic elastomers–Tension. ASTM International. https://doi.org/10.1520/D0412-16R21. www.astm.org
- Augurelle, A. S., Smith, A. M., Lejeune, T., & Thonnard, J. L. (2003). Importance of cutaneous feedback in maintaining a secure grip during manipulation of hand-held objects. Journal of Neurophysiology, 89(2), 665–671. https://doi.org/10.1152/jn.00249.2002
- Bae, J. H., Kang, S. H., Seo, K. M., Kim, D. K., Shin, H. I., & Shin, H. E. (2015). Relationship between grip and pinch strength and activities of daily living in stroke patients. Annals of Rehabilitation Medicine, 39(5), 752–762. https://doi.org/10.5535/arm.2015.39.5.752
- Barrios-Muriel, J., Romero-Sánchez, F., Alonso-Sánchez, F. J., & Rodríguez Salgado, D. (2020). Advances in ORthotic and prosthetic manufacturing: A technology review. Materials (Basel , Switzerland), 13(2), 295. https://doi.org/10.3390/ma13020295
- BASF 3D Printing Solution. (2020). Ultrasint TPU01 product Information. Retrieved August 17, 2022, from https://am3d.pl/wp-content/certyfikaty/tpu01-certyfikat-biozgodnosci.pdf
- Benjamin, J. E., Blaha, J. M., Chiuve, E. S., Cushman, R. M., Das, D. S., Deo, R. R., De Ferranti, C. S., Floyd, C. J., Fornage, E. M., Gillespie, H. C., Isasi, T. C., Jiménez, H. M., Jordan, E. L., Judd, W. S., Lackland, K. D., Lichtman, R. J., Lisabeth, J. L., Liu, J. S., Longenecker, A. C. … Muntner, S. P. (2017). Heart disease and stroke statistics—2017 update: A report from the american heart association. Circulation, 135(10), e146–603. https://doi.org/10.1161/CIR.0000000000000485
- Billinger, S. A., Arena, R., Bernhardt, J., Eng, J. J., Franklin, B. A., Johnson, C. M., MacKay-Lyons, M., Macko, R. F., Mead, G. E., Roth, E. J., Shaughnessy, M., & Tang, A. (2014). Physical activity and exercise recommendations for stroke survivors: A statement for healthcare professionals from the American heart association/American stroke association. Stroke, 45(8), 2532–2553. https://doi.org/10.1161/str.0000000000000022
- Blaya, F., Pedro, P. S., Silva, J. L., D’amato, R., Heras, E. S., & Juanes, J. A. (2018). Design of an orthopedic product by using additive manufacturing technology: The arm splint. Journal of Medical Systems, 42(3), 54. https://doi.org/10.1007/s10916-018-0909-6
- Borson, S., Scanlan, J. M., Watanabe, J., Tu, S. P., & Lessig, M. (2005). Simplifying detection of cognitive impairment: Comparison of the mini-cog and mini-mental state examination in a multiethnic sample. Journal of the American Geriatrics Society, 53(5), 871–874. https://doi.org/10.1111/j.1532-5415.2005.53269.x
- Broeks, J. G., Lankhorst, G. J., Rumping, K., & Prevo, A. J. (1999). The long-term outcome of arm function after stroke: Results of a follow-up study. Disability and Rehabilitation, 21(8), 357–364. https://doi.org/10.1080/096382899297459
- Carbon3D. (2021). EPU 40 technical data sheet, document #103208-00 rev F. Retrieved August 18, 2022, from https://docs.carbon3d.com/files/technical-data-sheets/tds_carbon_epu-40.pdf
- Chang, W. D., & Lai, P. T. (2015). New design of home-based dynamic hand splint for hemiplegic hands: A preliminary study. Journal of Physical Therapy Science, 27(3), 829–831. https://doi.org/10.1589/jpts.27.829
- Davis, F., & Davis, F. (1989). Perceived usefulness, perceived ease of use, and user acceptance of information technology. MIS Quarterly, 13(3), 319. https://doi.org/10.2307/249008
- Desrosiers, J., Bravo, G., Hébert, R., Dutil, E., & Mercier, L. (1994). Validation of the box and block test as a measure of dexterity of elderly people: Reliability, validity, and norms studies. Archives of Physical Medicine and Rehabilitation, 75(7), 751–755. https://doi.org/10.1016/0003-9993(94)90130-9
- Desrosiers, J., Noreau, L., Rochette, A., Bourbonnais, D., Bravo, G., & Bourget, A. (2006). Predictors of long-term participation after stroke. Disability and Rehabilitation, 28(4), 221–230. https://doi.org/10.1080/09638280500158372
- Dobkin, B. H. (2005). Rehabilitation after stroke [Article]. The New England Journal of Medicine, 352(16), 1677–1684. https://doi.org/10.1056/NEJMcp043511
- Duret, C., Grosmaire, A. -G., & Krebs, H. I. (2019). Robot-assisted therapy in upper extremity hemiparesis: Overview of an evidence-based approach. Frontiers in Neurology, 10. 412–412. https://doi.org/10.3389/fneur.2019.00412
- Electro Optical Systems. (2021). EOS TPU 1301 declaration of compliance regarding biocompatibility tests. Retrieved August 17, 2022, from https://www.hasenauer-hesser.de/fileadmin/dokumente_infos/datebblaetter/werkstoffe/Declaration_of_Compliance_biocomp_EOS_TPU_1301__V1_final.pdf
- Fernandez-Vicente, M., Escario Chust, A., & Conejero, A. (2017). Low cost digital fabrication approach for thumb orthoses. Rapid Prototyping Journal, 23(6), 1020–1031. https://doi.org/10.1108/RPJ-12-2015-0187
- Gabriele, B., Sami, H., & Alberto, S. (2016). A critical analysis of a hand orthosis reverse engineering and 3D printing process. Applied Bionics and Biomechanics, 2016. https://doi.org/10.1155/2016/8347478
- Gao, F., Latash, M. L., & Zatsiorsky, V. M. Similar motion of a hand-held object may trigger nonsimilar grip force adjustments. (2007). Journal of Hand Therapy, 20(4), 300–307. quiz 308; discussion 309. https://doi.org/10.1197/j.jht.2007.06.002
- Held, J. P. O., Klaassen, B., Eenhoorn, A., van Beijnum, B.-J. F., Buurke, J. H., Veltink, P. H., & Luft, A. R. (2018). Inertial sensor measurements of upper-limb kinematics in stroke patients in clinic and home environment. https://doi.org/10.3389/fbioe.2018.00027
- Hoffman, H. B., Blakey, G. L., & Lannin, N. (2011). New design of dynamic orthoses for neurological conditions. NeuroRehabilitation (Reading, Mass), 28(1), 55–61. https://doi.org/10.3233/NRE-2011-0632
- Hoffmann, G., Conrad, M., Qiu, D., & Kamper, D. (2015). Contributions of voluntary activation deficits to hand weakness after stroke. Topics in Stroke Rehabilitation, 23(6), 1945511915Y.1945511000. https://doi.org/10.1179/1945511915Y.0000000023
- Kim, H., & Jeong, S. (2015). Case study: Hybrid model for the customized wrist orthosis using 3D printing. Journal of Mechanical Science and Technology, 29(12), 5151–5156. https://doi.org/10.1007/s12206-015-1115-9
- Kwakkel, G., Veerbeek, J. M., van Wegen, E. E., & Wolf, S. L. (2015). Constraint-induced movement therapy after stroke. Lancet neurology, 14(2), 224–234. https://doi.org/10.1016/s1474-4422(14)70160-7
- Lang, C. E., Wagner, J. M., Dromerick, A. W., & Edwards, D. F. (2006). Measurement of upper-extremity function early after stroke: Properties of the action research arm test. Archives of Physical Medicine and Rehabilitation, 87(12), 1605–1610. https://doi.org/10.1016/j.apmr.2006.09.003
- Lawrence, S. E., Coshall, G. C., Dundas, D. A. R., Stewart, D. A. J., Rudd, D. A. A., Howard, D. A. R., & Wolfe, D. A. C. (2001). Estimates of the prevalence of acute stroke impairments and disability in a multiethnic population. Stroke: Journal of the American Heart Association, 32(6), 1279–1284. https://doi.org/10.1161/01.STR.32.6.1279
- Liang, K.-J., Chen, H.-L., Shieh, J.-Y., & Wang, T.-N. (2021). Measurement properties of the box and block test in children with unilateral cerebral palsy. Scientific Reports, 11(1), 20955. https://doi.org/10.1038/s41598-021-00379-3
- Lin, K. C., Chuang, L. L., Wu, C. Y., Hsieh, Y. W., & Chang, W. Y. (2010). Responsiveness and validity of three dexterous function measures in stroke rehabilitation. Journal of Rehabilitation Research and Development, 47(6), 563–571. https://doi.org/10.1682/jrrd.2009.09.0155
- Meyer, J. T., Weber, S., Jäger, L., Sigrist, R., Gassert, R., & Lambercy, O. (2022). A survey on the influence of CYBATHLON on the development and acceptance of advanced assistive technologies. Journal of Neuroengineering and Rehabilitation, 19(1), 38. https://doi.org/10.1186/s12984-022-01015-5
- Miller, L. C., Ruiz-Torres, R., Stienen, A. H. A., & Dewald, J. P. A. (2009). A wrist and finger force sensor module for use during movements of the upper limb in chronic hemiparetic stroke. IEEE Transactions on Biomedical Engineering, 56(9), 2312–2317. https://doi.org/10.1109/TBME.2009.2026057
- Money, A. G., Barnett, J., Kuljis, J., Craven, M. P., Martin, J. L., & Young, T. (2011). The role of the user within the medical device design and development process: Medical device manufacturers’ perspectives. BMC Medical Informatics and Decision Making, 11(1), 15. https://doi.org/10.1186/1472-6947-11-15
- Morris, J. H., van Wijck, F., Joice, S., & Donaghy, M. (2013). Predicting health related quality of life 6 months after stroke: The role of anxiety and upper limb dysfunction. Disability and Rehabilitation, 35(4), 291–299. https://doi.org/10.3109/09638288.2012.691942
- Nuckols, K., Hohimer, C. J., Glover, C., de Lucena, D. S., Moyo, W., Wagner, D., Cloutier, A., Lin, D. J., & Walsh, C. J. (2020). Effects of a soft robotic glove using a high repetition protocol in chronic stroke: A pilot study. IEEE.
- Oliveira, A. C., Rose, C. G., Warburton, K., Ogden, E. M., Whitford, B., Lee, R. K., & Deshpande, A. D. (2019). Exploring the capabilities of harmony for upper-limb stroke therapy. IEEE Int Conf Rehabil Robot, 2019 (pp. 637–643). https://doi.org/10.1109/icorr.2019.8779558
- Pan, R., Yang, L., Zheng, L., Hao, L., & Li, Y. (2020). Microscopic morphology, thermodynamic and mechanical properties of thermoplastic polyurethane fabricated by selective laser sintering. Materials Research Express, 7(5), 55301. https://doi.org/10.1088/2053-1591/ab8b87
- Parker, V. M., Wade, D. T., & Langton Hewer, R. (1986). Loss of arm function after stroke: Measurement, frequency, and recovery. International Rehabilitation Medicine, 8(2), 69–73. https://doi.org/10.3109/03790798609166178
- Paterson, A. M., Bibb, R., Campbell, R. I., & Bingham, G. (2015). Comparing additive manufacturing technologies for customised wrist splints. Rapid Prototyping Journal, 21(3), 230–243. https://doi.org/10.1108/RPJ-10-2013-0099
- Platz, T., Pinkowski, C., van Wijck, F., Kim, I. H., diBella, P., & Johnson, G. (2005). Reliability and validity of arm function assessment with standardized guidelines for the fugl-meyer test, action research arm test and box and block test: A multicentre study. Clinical Rehabilitation, 19(4), 404–411. https://doi.org/10.1191/0269215505cr832oa
- Polygerinos, P., Wang, Z., Galloway, K. C., Wood, R. J., & Walsh, C. J. (2015). Soft robotic glove for combined assistance and at-home rehabilitation. Robotics and Autonomous Systems, 73, 135–143. https://doi.org/10.1016/j.robot.2014.08.014
- Portnoy, S., Barmin, N., Elimelech, M., Assaly, B., Oren, S., Shanan, R., & Levanon, Y. (2020). Automated 3D-printed finger orthosis versus manual orthosis preparation by occupational therapy students: Preparation time, product weight, and user satisfaction. Journal of Hand Therapy, 33(2), 174–179. https://doi.org/10.1016/j.jht.2020.03.022
- Raghavan, P. (2015). Upper limb motor impairment after stroke. Physical Medicine and Rehabilitation Clinics of North America, 26(4), 599–610. https://doi.org/10.1016/j.pmr.2015.06.008
- Ridding, M. C., Pearce, S. L., & Flavel, S. C. (2005). Modulation of intracortical excitability in human hand motor areas. The effect of cutaneous stimulation and its topographical arrangement. Experimental Brain Research, 163(3), 335–343. https://doi.org/10.1007/s00221-004-2176-7
- Riemer-Reiss, M., & Wacker, R. (2000). Factors associated with assistive technology discontinuance among individuals with disabilities. Journal of Rehabilitation, 66(3), 44–50. https://www.nationalrehab.org/journal-of-rehabilitation
- Rinere O’brien, S. (2010). Trends in inpatient rehabilitation stroke outcomes before and after advent of the prospective payment system: A systematic review. Journal of Neurologic Physical Therapy, 34(1), 17–23. https://doi.org/10.1097/NPT.0b013e3181cfd3ac
- Safaz, I., Türk, H., Yaşar, E., Alaca, R., Tok, F., & Tuğcu, I. (2015). Use and abandonment rates of assistive devices/orthoses in patients with stroke. Gulhane Medical Journal, 57(2), 142–144. https://doi.org/10.5455/gulhane.152325
- Sawaki, L., Butler, A. J., Leng, X., Wassenaar, P. A., Mohammad, Y. M., Blanton, S., Sathian, K., Nichols Larsen, D. S., Wolf, S. L., Good, D. C., & Wittenberg, G. F. (2008). Constraint-induced movement therapy results in increased motor map area in subjects 3 to 9 months after stroke. Neurorehabilitation and Neural Repair, 22(5), 505–513. https://doi.org/10.1177/1545968308317531
- Shah, S. G., & Robinson, I. (2007). Benefits of and barriers to involving users in medical device technology development and evaluation. International Journal of Technology Assessment in Health Care, 23(1), 131–137. https://doi.org/10.1017/s0266462307051677
- Shim, J. K., Karol, S., Kim, Y. S., Seo, N. J., Kim, Y. H., Kim, Y., & Yoon, B. C. (2012). Tactile feedback plays a critical role in maximum finger force production. Journal of Biomechanics, 45(3), 415–420. https://doi.org/10.1016/j.jbiomech.2011.12.001
- United Nations. (2019). World population ageing 2019: Highlights (ST/ESA/SER.A/430).
- van Ommeren, A. L., Smulders, L. C., Prange Lasonder, G. B., Buurke, J. H., Veltink, P. H., & Rietman, J. S. (2018). Assistive technology for the upper extremities after stroke: Systematic review of users’ needs. JMIR Rehabilitation and Assistive Technologies, 5(2). e10510–e10510. https://doi.org/10.2196/10510
- Winstein, C. J., Stein, J., Arena, R., Bates, B., Cherney, L. R., Cramer, S. C., Deruyter, F., Eng, J. J., Fisher, B., Harvey, R. L., Lang, C. E., MacKay-Lyons, M., Ottenbacher, K. J., Pugh, S., Reeves, M. J., Richards, L. G., Stiers, W., & Zorowitz, R. D. (2016). Guidelines for adult stroke rehabilitation and recovery: A guideline for healthcare professionals from the American heart association/American stroke association. Stroke, 47(6), e98–169. https://doi.org/10.1161/str.0000000000000098
- Wolf, S., Catlin, P. A., Ellis, M., Archer, A. L., Morgan, B., & Piacentino, A. (2001). Assessing wolf motor function test as outcome measure for research in patients after stroke. Stroke, 32(7), 1635–1639. https://doi.org/10.1161/01.STR.32.7.1635
- Wolf, S. L., Winstein, C. J., Miller, J. P., Thompson, P. A., Taub, E., Uswatte, G., Morris, D., Blanton, S., Nichols Larsen, D., & Clark, P. C. (2008). Retention of upper limb function in stroke survivors who have received constraint-induced movement therapy: The EXCITE randomised trial. Lancet Neurology, 7(1), 33–40. https://doi.org/10.1016/S1474-4422(07)70294-6
- Yang, S., Tang, Y., & Zhao, Y. F. (2015). A new part consolidation method to embrace the design freedom of additive manufacturing. Journal of Manufacturing Processes, 20, 444–449. https://doi.org/10.1016/j.jmapro.2015.06.024
- Yang, Q., Tong, X., Schieb, L. E. A., Vaughan, A., Gillespie, C., Wiltz, J. L., King, S. C., Odom, E., Merritt, R., Hong, Y., & George, M. G. (2017). Vital signs: Recent trends in stroke death rates — United States, 2000–2015. MMWR Morbidity and Mortality Weekly Report, 66(35), 933–939. https://doi.org/10.15585/mmwr.mm6635e1External
- Zhu, X., & Yang, Q. (2020). Sintering the feasibility improvement and mechanical property of UHMWPE via selective laser sintering. Plastics, Rubber & Composites, 49(3), 116–126. https://doi.org/10.1080/14658011.2020.1718321