Abstract
When the human health risk assessment/risk management paradigm was developed, it did not explicitly include a “problem formulation” phase. The concept of problem formulation was first introduced in the context of ecological risk assessment (ERA) for the pragmatic reason to constrain and focus ERAs on the key questions. However, this need also exists for human health risk assessment, particularly for cumulative risk assessment (CRA), because of its complexity. CRA encompasses the combined threats to health from exposure via all relevant routes to multiple stressors, including biological, chemical, physical and psychosocial stressors. As part of the HESI Risk Assessment in the 21st Century (RISK21) Project, a framework for CRA was developed in which problem formulation plays a critical role. The focus of this effort is primarily on a chemical CRA (i.e., two or more chemicals) with subsequent consideration of non-chemical stressors, defined as “modulating factors” (ModFs). Problem formulation is a systematic approach that identifies all factors critical to a specific risk assessment and considers the purpose of the assessment, scope and depth of the necessary analysis, analytical approach, available resources and outcomes, and overall risk management goal. There are numerous considerations that are specific to multiple stressors, and proper problem formulation can help to focus a CRA to the key factors in order to optimize resources. As part of the problem formulation, conceptual models for exposures and responses can be developed that address these factors, such as temporal relationships between stressors and consideration of the appropriate ModFs.
Introduction
A primary aim of risk assessment is informing decision makers about the risks posed to public health by “real-world” environmental exposures to facilitate the weighing of these risks against the benefits that accrue from the activities that generate the risk. Over time and with increased analytical sophistication, the complexity and diversity of real-world environmental exposures to all types of stressors have become increasingly apparent, necessitating a more holistic approach to risk assessment; one that accounts for the combined effects from exposure to both chemical and nonchemical stressors (Callahan & Sexton Citation2007; NRC Citation2009).
Cumulative risk assessment (CRA) is the term commonly used for this process, the assessment of risks from combined exposures. CRA has been described and developed in numerous frameworks and reports (e.g., USEPA Citation2007; EFSA Citation2008, Citation2013; NRC Citation2009; Price et al. Citation2009, Citation2012; Meek et al. Citation2011). Some of the existing approaches are discussed in some detail in Moretto et al. (Citation2016). The problem formulation (PF) phase is a shared activity between risk assessors and risk managers as well as other stakeholders and, as such, is a prerequisite before embarking on a risk assessment (USEPA Citation2014). The present paper examines the key issues involved in undertaking PF in the context of CRA, and identifies analytical frameworks and conceptual models that can help simplify the process and make the underlying assumptions more explicit. The final product of the PF phase should be a formal analytical plan that provides the foundation for the subsequent assessment of cumulative risks.
RISK21 approach to cumulative risk
The ILSI Health and Environmental Sciences Institute (HESI) created the Risk Assessment in the 21st Century (RISK21) project to address issues and catalyze improvements in human health risk assessment. RISK21 has developed a conceptual framework that is a PF-based, exposure-driven paradigm that takes maximum advantage of existing information and aids in identifying when additional data are needed to make a decision. The overarching principles of the RISK21 approach are introduced by Pastoor et al. (Citation2014) and explained in detail in Embry et al. (Citation2014).
The overall objective of the RISK21 CRA approach is to provide a process for assessing the possible adverse health effects from combined exposure to multiple chemical and non-chemical stressors. The companion paper (Moretto et al. Citation2016) describes the framework and approach in detail, following the PF phase; however, it is important to note that cumulative risk framework is not prescriptive. Rather the emphasis is on documenting and supporting a logical rationale for why a CRA is needed and under what restrictions, if any, the CRA is being conducted. The scope of RISK21 CRA is limited to multiple chemical stressors (i.e., two or more chemicals) with subsequent consideration of non-chemical stressors, defined as “modulating factors” (ModFs). Therefore, evaluations focused only on non-chemical stressors or single chemicals plus ModFs were considered out of scope.
The term ModFs was introduced in the RISK21 paper describing a quantitative key events/dose–response framework (Simon et al. Citation2014). The various factors that can modulate the effect of, or exposure to, a chemical (or both) were separated into simpler elementary constituents. ModFs represent biological, environmental and lifestyle factors, including host factors that can modulate the response to chemical stressors, thus altering the probability or magnitude of the adverse outcome. ModFs also include factors influencing exposure, such as processing of food or weather patterns. Their consideration in the PF step is further described in the section “Consideration of modulating factors” and the methodology for their inclusion in the RISK21 CRA framework is discussed in detail in Moretto et al. (Citation2016).
Background on PF
PF is a systematic approach that identifies all factors critical to a risk assessment and considers the following elements:
Purpose of the risk assessment;
Overall risk management goal & options;
Scope and depth of the analysis;
Analytical approach and
Available resources.
When the human health risk assessment/risk management paradigm was first presented in 1983 (NAS Citation1983) it did not explicitly include a PF phase. The concept of PF was first introduced in the context of ecological risk assessment (ERA) and in 1992, a PF step was incorporated in the framework for ERAs (USEPA Citation1992) (). More recently, the importance of an explicit PF phase in risk assessment related to human health and safety of food has also become more widely recognized (Renwick et al. Citation2003; NRC Citation2009; WHO Citation2009; WHO IPCS Citation2009; Sheldon Citation2010) and this was a point stressed in EPA’s recent Framework for Human Health Risk Assessment to Inform Decision Making (USEPA Citation2014).
Figure 1. Diagrammatic representation of the processes of risk assessment and its relationship to risk management. Communication between the assessors and managers (dashed arrows) and the iterative nature of the process is also illustrated (modified from USEPA Citation1992).
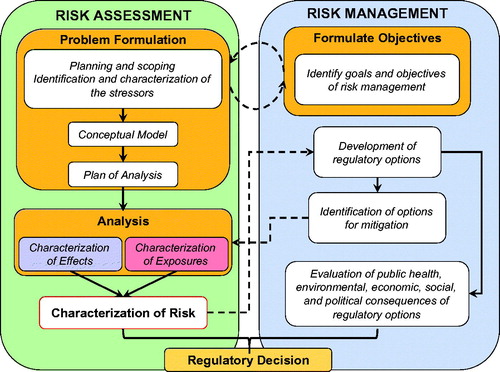
The first step in PF is defining the purpose or scope of the assessment. This will set the boundaries for the assessment, with the goal of making it “fit for purpose”. For example, it may be that a regulatory decision on a specific chemical within a specific legislative category is needed and therefore, the assessment should be limited to that chemical plus other chemicals in that category. It could be that there is concern about exposures from a single source, comprising a limited but diverse range of chemicals. Or perhaps the concern is about the totality of exposure to chemicals affecting a specific biological process/target, such as the estrogen hormonal axis.
It is important to note that PF should be an iterative process during which uncertainties (data gaps) are identified and plans are made to address these. As key knowledge is refined during the risk assessment, this informs reformulation of the problem such that the risk assessment can proceed with the degree of certainty and precision necessary to enable a decision to be made. This iteration may take place early or later in the process when data on exposures and effects are better understood but, in all cases, this should lead to a refinement of the focus of the PF. In this context, it should be noted that exposures to some stressors may be more easily mitigated than others and that, for some, mitigation may not be practical or possible. For this reason, an additional refinement of the PF would be to consider options for risk management early in the process and to focus on stressors that can, in fact, be practically managed (see Table 7.1 of NRC Citation2009).
All PFs use existing information to characterize the stressor(s) to be assessed and the population(s) or subgroup(s) exposed to the stressor(s). For each stressor, this requires knowledge of the determinants of the routes of exposure, the frequency of exposures and the magnitude of exposure.
“Gatekeeper” step
A prerequisite to PF in the CRA approach proposed by RISK21 is a “gatekeeper” step, which determines whether CRA is warranted. In reality, not all risk assessments will necessitate consideration of multiple stressors. In many instances, completing a full PF for CRA without first determining whether there is sufficient evidence to support such an assessment would be a waste of time and resources. Asking and answering the initial question, “In which circumstances and under what conditions is a CRA necessary or even appropriate?” is extremely important.
A CRA is deemed not necessary or prudent if the totality of information is such that it does not indicate evidence for both co-exposure and common mode of toxic action or target system. This step establishes a minimum set of conditions that trigger the need for a CRA and requires that sufficient evidence is available to justify moving into the full PF phase. illustrates the proposed PF process for CRA, including the addition of this initial “gatekeeper” step. A more detailed description of the proposed RISK21 CRA Framework can be found in Moretto et al. (Citation2016).
Figure 2. Schematic representation and description of the steps involved in PF for CRA, including the “gatekeeper” step.
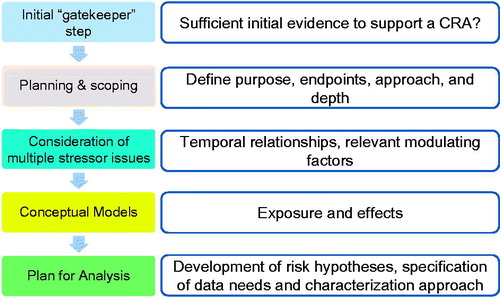
Specifically, two key questions are proposed as part of the gatekeeper step, to help guide the determination for a CRA.
If the currently available data demonstrate co-exposure, is there a rationale for considering any of the compounds in a common chemical assessment group (CCAG) based on knowledge of toxicity?
CCAG is discussed in Moretto et al. (Citation2016) and is defined as a group of chemicals sharing both evidence for likelihood of co-exposure within a relevant timeframe AND evidence for dose-additive response at relevant human exposure levels.
If the currently available data suggest or demonstrate common toxicity, or any other reason to consider dose-additivity is likely to occur, is there a rationale for considering any of the compounds in a CCAG based on knowledge of exposure?
The data used to complete this gatekeeper step should be carefully documented and integrated into the overall PF. Scientific evidence should therefore be assembled such that a transparent determination can be made concerning the likelihood of co-exposure AND the likelihood of common toxicity for all chemicals being considered. Both pieces of information are requisite to justify the need to assess cumulative risk and are emphasized as part of this step, which establishes a minimum set of conditions that trigger the need for a CRA and requires that sufficient evidence is available to justify moving into the full PF phase.
It is stressed that this gatekeeper step does not involve a complete assessment but rather represents an initial collection and summary of the data in-hand for the purpose of formulating the problem of CRA. Given a sufficient rationale can be established via available evidence on likelihood of co-exposure AND common toxicity, a full PF should ensue. There is no need for tiers at the gatekeeper stage, they will be applied during the CRA procedure following the PF.
PF for CRA
PF for CRA is a stepwise process () and is described in detail in the following sections.
Many risk assessments are conducted within a specific context that involves regulators and a number of stakeholders who have an interest in the issue in question. The USEPA Framework for CRA (USEPA Citation2003) and the WHO IPCS (Meek et al. Citation2011) recommend that planning and scoping of the risk assessment () should begin with a dialog among these individuals or groups. This dialog should be two-way and may be iterative as the PF is refined, the plan of analysis is developed, and the resources required for the assessment are identified.
Planning and scoping
In the planning and scoping stage, it is important to carefully define the terms of reference (ToR) of the CRA. These will comprise the focus of the risk assessment and the key questions to be addressed. The ToR should be such that the CRA will provide relevant, science-based evidence to answer the concerns of decision makers and other stakeholders, in an appropriate time-frame and within the available resources. This should be a formal and transparent process and the approaches used to identify these key questions and concerns need to be documented. In this sense, the PF is the refinement and translation of questions from decision makers and various stakeholders into scientifically testable hypotheses.
A key activity in the planning and scoping phase is to clearly identify the goals for protecting human health and/or the environment and the endpoints used to determine risk or harm. These “protection goals” are usually defined in the various statutes and regulations that relate to the protection of human health and the environment and, although these differ somewhat between jurisdictions, the main objective is the protection of the individual, a population, or an ecosystem against a specific risk or against any harm. However, the meaning of “protection” and “harm” is subject to interpretation and, whilst a laudable social and/or political objective it may be hard to define numerically or even qualitatively (e.g., is mild sensory irritation harm?) and therefore it may be difficult to determine if the level of protection is adequate or if mitigation measures have actually improved the situation. Often, implicit levels of protection are used, based on regulatory exposure limits (assessment endpoints), i.e., if exposure is below the RfD or ADI, the desired level of protection has been achieved.
Assessment endpoints are actual measures against which protection can be evaluated. They are predicated on the (implicit) protection goals but are quantitative so that they can be easily combined and/or compared in a mathematical sense. In keeping with the objectives of assessing risks to humans from exposures to multiple chemical stressors, endpoints that could be used in the CRA are those that are currently used in various regulations; however, attention must be paid to the population at risk and the predicted route of exposure. For example, if only oral ingestion is expected, endpoints of concern via the dermal route of exposure would be irrelevant. In the case of chemical stressors for which standards or other regulatory decisions exist, the endpoints will have been identified in toxicity testing of the individual chemicals and extrapolated to humans for the purposes of setting of guidelines for exposures from air, food and water. These limits may reflect a probability of risk (e.g., the often-employed 1 in 106 estimate for cancer risk) or application of a set of extrapolation factors for responses that have, or are more likely to have, a threshold and/or are readily reversible.
Endpoints for ModFs are less well defined. While it would be possible to include easily-quantified measures of the physical environment, such as temperature, humidity and intensity of noise as assessment endpoints, this would be much more difficult for the psychosocial environment, nutrition or infectious diseases. However, where these are influencing or contributing to exposure or the responses to chemical stressors, the assessment endpoints for the chemicals can be used.
As has been pointed out (Moretto et al. Citation2016), CRA should be conducted using a tiered approach. This allows stressors that truly do not present or do not significantly contribute to a cumulative risk to be eliminated from consideration early in the process and helps to simplify the assessment by reducing the number of hypotheses that need to be tested. The framework for cumulative risks from chemicals proposed by IPCS (Meek et al. Citation2011) serves as a starting point. It should be pointed out that this process is tiered and that it may not be necessary to complete all of the tiers to reach a decision. For instance, Tier 0 data may be sufficient to conduct a CRA based on worst-case assumptions of exposure and interaction which demonstrates an acceptable level of risk, as defined by the PF, eliminating the need for further consideration or assessment. However, if multiple tiers of information are required, the assessment endpoint remains the same.
Considerations specific to multiple stressors
PF differs for single and multiple stressors. PFs for single substances may involve many considerations but are manageable to carry out. However, PFs for combinations of chemicals and ModFs could become orders of magnitude more complex and, perhaps, even unworkable. Thus, what is needed is the appropriate information to identify the boundaries within which the risk of the combined exposures can be accepted and not necessarily a complete description of all the characteristics of the stressors in question. Whilst important for a single stressor, it is critical for multiple stressors that the PF efficiently narrows the focus of the assessment to the key issues and questions, and bounds the uncertainties. This should be done with a view to the desired goal of the assessment in light of actions that can be taken for risk management which will have a real impact.
For a CRA, the factors identified in the initial PF lead to a conceptual model which organizes the linkages and manages the assessment. For example, the probability of co-occurrence of exposures that would lead to cumulative effects must be considered (see below). This requires an understanding of the spatial distribution of the stressors and their temporal co-occurrence, at least within the time-frame for the causation of cumulative responses. Based on such considerations, it may be possible to eliminate some stressors from further discussion, thus reducing complexity.
For chemical stressors, information of how they may interact in the exposed population or subgroup should be identified, if possible. For this, knowledge of the mechanisms and/or mode of action of the stressors as well as their toxicodynamics (TD) and toxicokinetics (TK) might become essential if higher tiered assessment is needed. Key data on TK include distribution and (lack of) persistence of the substances in organs or tissues. This provides information on which temporal co-occurrence in potential target organs can be assessed and can help reduce the number of hypotheses on interactions that need to be considered. Key information on TD includes the time-course of recovery and the potential for joint action (additive or otherwise). Both TK and TD may be modified by other stressors.
The complexity of the assessment can vary markedly with the needs of the risk manager, and will depend on the policy context. This should be clearly identified in PF. Additional considerations that must be taken into account when dealing with multiple stressors are described below.
Temporal relationships between stressors
Timing of exposures to multiple stressors is a major determinant of risks of adverse responses to combined exposures. Traditionally, contemporaneous (co-)exposure to two or more stressors has been used as the trigger for considering additive or other interactive effects. However, consecutive, separate or partially overlapping exposures may also be important. For some responses, particularly interactive responses or those mediated via ModFs, prior exposures may be more important than simultaneous exposure. This, in turn, will be influenced by the duration of the response, any latency of response to the stressor(s) and rate of recovery from the effects of the stressor(s) (). The influence of temporality of responses and recovery is but one pathway for cumulative interactions. Recovery may be very rapid (hours), such as for carbamylated AChE, longer (days-weeks) for phosphorylated/aged AChE, or even transgenerational, such as for some genetic or epigenetic changes that modify TD or TK. This last point is important as stressors, such as famine, have resulted in epigenetic changes in humans that may affect interactive response in one generation (Koturbash et al. Citation2011; Maze et al. Citation2011; Smeester et al. Citation2011) or be transgenerational (Anway et al. Citation2005; Painter et al. Citation2006; Skinner et al. Citation2011; Vanhees et al. Citation2011).
Figure 3. Diagrammatic representation of temporal differences in exposures and how these may interact with persistence of the stressor in the organism and/or latency of response or length of recovery from the stressor. Examples: (A) a mixture of a carbamate and organophosphorus insecticides, (B) an organophosphorus insecticide followed by a carbamate and (C) a carbamate followed by an organophosphorus insecticide. While this representation may not apply to apical end-points, it may help understanding and visually describing the need for CRA when an intermediate KE occurs, although, when considering an individual compound, no clear apical effect is observed. In the example provided, AChE can be inhibited at subthreshold levels by each individual compound, but, depending on timing, the threshold can be reached (overcome) after combined exposure. Heavy black lines – exposure; shaded areas – response.
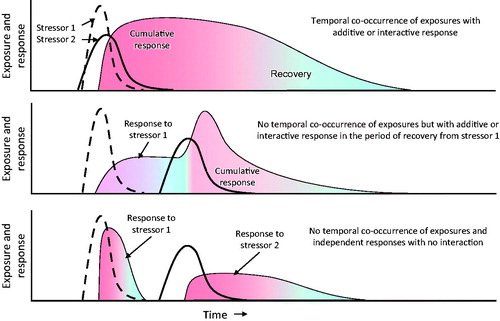
Consideration of ModFs
There is an additional level of complexity in CRA when ModFs must be considered and/or some of the chemicals do not cause effects through traditional toxicological mechanisms. In the context of initiatives on environmental justice, it has been recommended to include social, economic, cultural and community health factors, especially those involving vulnerability, into CRA frameworks (NEJAC Citation2004, Citation2010). Obviously, ModFs on their own can also interact to cause cumulative effects, but the focus of this paper is directed to situations where more than one chemical has been identified as an issue. Nevertheless, the concepts discussed here should also be useful in providing guidance for PF for combinations of stressors acting only by non-toxicological mechanisms.
ModFs fall into four main categories: host factors, lifestyle, environmental factors and other chemicals (). These need to be considered in detail during the later stages of the CRA process (Moretto et al. Citation2016), but during the PF phase, an initial appraisal should be included in the conceptual model (see section “Modulating factors”).
Table 1. Modulating factors.
Conceptual model
For PFs developed for single or multiple stressors that are known (i.e., the hazards have been identified), the outcome of the process consists of a conceptual model, a series of risk hypotheses that can then be tested with the data that have been gathered, and a plan of analysis. The conceptual model for exposure defines the routes, amounts, frequency, intensity, and, in the case of CRAs, the probability of co-occurrence of the stressors. The conceptual model for effects in the exposed (sub)groups includes the effects of the individual stressors and how these may combine to result in additive responses. Responses other than additivity, i.e., antagonistic, or synergistic, for combined chemical exposures should not be an issue since these generally occur only at biologically effective doses (Boobis et al. Citation2011; SCHER, SCCS, SCENIHR Citation2012). Possible exceptions may occur in certain instances with the “effect based” approach, when one or more components are present above their threshold (limit). Since there will be little or no information in humans on many of the potential interactions, extrapolation of effects observed in experimental systems (e.g., IVIVE) to humans may be required. The development of the model will likely be iterative, as long as any of the data used to develop the model are refined. The conceptual model provides the basis for the risk hypotheses, which may be related to different subgroups including different life-stages or (patho)physiological conditions, stressors, exposure scenarios and responses which can then be tested with the data on exposures and effects.
Exposure
The movement and fate of chemicals in the environment and how humans may be exposed to them is relatively well understood. Examples of potential sources of chemical stressors are manufacturing, combustion of fossil fuel and/or biomass, treatment of waste, agricultural activities, extraction of mineral resources, activities in the home, etc. (). If these chemicals are used, either in a process, or released to the environment they may move from the point of release to result in exposures of humans. Ultimately, these routes of exposure will be via air, soil, water, solids carried in air or water, and food.
Exposures may be via a single route (i.e., dermal, inhalation or oral) or they may be aggregated over several routes which may vary over time and space, even for a single chemical, but particularly important for cumulative assessments. For chemical stressors, their physicochemical properties will be major drivers of their movement in the environment and route of exposure.
Hazard
The key to construction of a useful and practical conceptual model of effects is to be able to quantify the relationship between exposures to the chemical stressors, relevant ModFs and response. Exposures to multiple chemical stressors may result in cumulative responses via TK and/or TD processes. The former, i.e., the absorption, distribution, metabolism and excretion of one or more xenobiotic, is a key determinant of the type and magnitude of response. This will lead to a certain combined concentration at the target site (e.g., organophosphate insecticides) (Timchalk & Poet Citation2008). Knowledge of the mode of action and ADME will provide guidance to help identify potential interactions. In the spectrum of interactions from cumulative effects of pharmaceuticals and other stressors, there is a wealth of data on TK and TD and how effects on these processes may alter responses to other pharmaceuticals, but at biologically effective doses. In the context of environmental exposure at low levels it is important to first establish whether TK and/or TD interactions are to be expected. It should also be noted that interactive effects may not necessarily be adverse in that they may stimulate detoxification of other substances or act antagonistically via TD mechanisms. In addition, components of food, such as secondary metabolites of plants, may affect TK (Alvarez et al. Citation2010; Jin & Han Citation2010) and TD of chemical stressors (Friedman Citation2006).
Receptors
In the case of human health risk assessments, receptors may be the population as a whole, individuals or groups of people, e.g., specific age groups such as children or the elderly; susceptible populations with preexisting disease, such as asthma; occupational groups; residents of a specific area; ethnic groups; economically disadvantaged groups, or subsets of the population with specific sensitivity mediated by genetic or epigenetic factors. Thus, in , the arrows can be modified as appropriate (e.g., bolded or dashed) to indicate that exposures may be of different intensity and some or many may not be relevant and could therefore be excluded from the assessment. The task in construction of the conceptual model is to assign appropriate quantities and/or intensity to the various potential routes of exposure and effects. This will then facilitate identification of the key questions and hypotheses of risks from exposures and responses to multiple stressors to be ranked and prioritized.
Modulating factors
ModFs can be grouped into host factors, life-style factors, environmental factors and other chemicals (). As previously discussed, these factors, if deemed necessary for inclusion, must be considered in detail in the CRA process (Moretto et al. Citation2016) but should also form part of the conceptual model in the PF phase. Given the complexity of these assessments, and the consequences of compounding multiple conservative assumptions, the number of variables to be considered should be kept to a minimum. In any case, it is important that the process is transparent and clearly communicated; an explanatory narrative should accompany the proposed table and associated graphical visualization (see below) of the effects of the ModFs.
Host factors
Some of these factors will affect the types of stressor to which humans are exposed, the intensity, and the responses. It is important to note that the vulnerability of humans to exposure and effects may differ across groups as a consequence of differences in genetic make-up, preexisting diseases, defense mechanisms and physiology.
Life-style factors
Nutritional stressors, such as obesity, starvation or deficiency of essential vitamins or micronutrients should be considered because they may interact with other stressors via alteration of physiological and biochemical processes.
Pharmaceuticals are separated from chemical and non-chemical stressors because exposures are deliberate and they are taken by humans because of their beneficial effects on health. In addition, the use of these substances is usually linked to preexisting diseases, infections, parasites and psychological stressors. As these substances are taken in doses that do alter physiological and biochemical processes, they are more likely to alter the TK and/or TD of other stressors (see below), as has been observed in the case of adverse reactions to combinations of drugs.
Environmental factors
Physical stressors include temperature (heat or cold), various forms of radiation (UV and X-rays and γ-radiation), noise and vibration. All of these have the potential to affect processes in the organism that may alter TK and/or TD of chemical stressors.
Psychological stressors and their potential interactions with human health have been summarized in an editorial (Barkin & Schlundt Citation2011) where it is proposed that a cascade of external factors such as the geophysical, economic, political, community and interpersonal environments may interact with the psychology of an individual to affect physiological and biochemical processes in the body with the end result that response to a stressor is altered. They further point out that, to identify, categorize and quantify these stressors, a multidisciplinary team will be needed with expertise in many areas.
Socioeconomic stressors may also be important and can act directly or indirectly. In the former case, for example, a lack of access to health care could result in a background of poor health that may exacerbate adverse effects from chemicals as well as biological agents. In the latter, socioeconomic stressors may exacerbate psychological, nutritional, disease stressors as well as increase exposures to chemical stressors.
For instance, an index can be created for racial/ethnic and socio-economic inequalities (Su et al. Citation2009), or for social determinants (Salinas et al. Citation2012). Matrices (WHO Citation2010) and algorithms (NEJAC Citation2010; Zartarian et al. Citation2011; USEPA Citation2016; Hastings et al. Citation2013) have been developed to help identify highest comparative risks. It is recognized that this requires additional types of scientific expertise and demands rigorous methodology for a clear delimitation of the analysis so that it is manageable and provides meaningful and applicable results.
Other chemicals
Chemical ModFs can impact the toxicity of a single chemical under consideration, or multiple chemical stressors for which the CRA is focused via TK, TD or both (Wason et al. Citation2012). For this reason, inclusion of ModFs will be highly dependent on available data and may serve to elevate the complexity of the assessment.
When determining the inclusion/exclusion of ModFs which are also chemicals, it must be stressed that the effects of the ModFs should occur and be relevant at or below the reference point/point of departure. ModFs, specifically, other chemicals that are not included in the CCAG, that are relevant at large doses (experimental doses that are several orders of magnitude higher than the human exposure levels) but irrelevant at human exposures should not be included in the CRA. There are several examples of ModFs occurring at large doses, only. These include, among others, saturation of metabolic pathways, saturation of excretory processes, consumption of buffering systems, and overload of repair systems. Examples include the hepatotoxicity of acetaminophen, which occurs only after glutathione levels in the liver are depleted (Henderson et al. Citation2000); the pulmonary toxicity of paraquat, which occurs only after renal excretion becomes saturated (Lock & Wilks Citation2010).
Plan for analysis for assessing cumulative risks
The final step in the process of PF is the development of the plan for the analysis of the risks posed by the exposures and effects identified in the conceptual models. The results of the conceptual models will identify a number of questions related to the assessment of risk of chemicals and ModFs. These include:
Which groups will be exposed and to what exposures?
What is the duration and frequency of exposures?
Within the constraints of the window of temporality that is important for CRA, what is the probability of co-occurrence of exposures?
What are the measures of effect for the mixture of stressors?
Do the stressors act additively or is there evidence for more or less than additive effects at environmentally-relevant exposures?
Based on these questions, a plan of analysis should specify which data are to be collected and how they are to be characterized. As discussed in Embry et al. (Citation2014), different amounts of data will be required for different tiers. Lower tiers based on deterministic hazard quotients require few data but are usually very conservative. Higher tiers such as probabilistic characterizations require extensive modeling or measurements or a combination of both. The hypotheses of risk are thus tested with the data on exposures and effects. These hypotheses can range from simple comparisons of deterministic values for exposures and effects via a quotient (with uncertainty factors) to full probabilistic analysis as discussed in Pastoor et al. (Citation2014).
Among the factors that need to be considered when developing a plan of analysis are the strengths of the methods of the test or model and their underlying data, their relevance to the problem that is being investigated, and uncertainties concerning the scenarios, theoretical assumptions and data obtained.
Strength addresses the inherent quality of a test (or computed data) relating to the methodology of the test or model, and the way that the performance and results of the test or model are described. Testing and modeling data in hazard assessment can be realized through many different approaches and conditions, e.g., for testing data: standardized protocol or not, test design, analytical method, mixture of compounds, statistical methodology, etc.; for modeling data: homogeneity of data, outliers, modeling techniques, etc. Exposure information can also be based on different models and assumptions and the reliability of existing approaches/tools depends on factors such as definition of exposure scenarios; selection of exposure routes; etc.
Relevance covers the extent to which a data set is appropriate for a particular risk assessment. The objective is to provide a comprehensive overview of all the factors taken into account for characterizing and ranking the relevance of data respective to a given assessment context and to build a relevance consensus framework.
Uncertainty can be analyzed according to its sources in all risk assessments, i.e., variability and lack of knowledge, and the types of uncertainty. For CRA, key areas of uncertainty are:
Uncertainty in the decision-rule, e.g., choice of chemicals to include in assessment group, selection of ModFs, choice of exposure scenarios, choice of uncertainty factors for extrapolations, handling of conflicting evidence;
Uncertainty in the structure, e.g., false positive or false negative assumptions, such as the nature of the combined effect of chemicals in an assessment group, homogeneity and description of the compartment, extrapolation for other species, extrapolation from route to route, statistical models applied and
Uncertainty in the parameters, e.g., temporality of exposure, temporality of effects, number of replicates, emission measurements, measurements on partitioning and concentration in various media, physico-chemical data.
Such a classification is a prerequisite for a better mutual understanding of the confidence attributed to a given dataset.
Conclusions
PF is an iterative process in which preliminary hypotheses are developed about the possible occurrence of adverse effects as a consequence of exposure to multiple chemicals and ModFs. It provides the foundation for the technical approach to the subsequent risk assessment. The outcome of the PF process is a conceptual model, or models, that identify the stressors, exposure pathways, the population exposed and the protection goal/assessment endpoints that will be covered in the risk assessment, and which describes the relationships among them (USEPA Citation2003).
One of the key challenges of PF in a CRA is the need to adequately define the relative importance of different stressors with regard to their effect on endpoints of concern. Here, an early assessment of the temporal relationship between exposures to various stressors is a critical step, as is determining whether there is a likelihood of interaction between them, or in their effect on specific endpoints.
PF in CRA is essential to ensure that assessments undertaken are relevant to public health, and that they can be achieved in a realistic timeframe with the resources available. It is important that PF focuses the assessment on the basis of those risk mitigation strategies that are feasible and likely to have significant impact. Early identification of relevant chemicals to form the CCAG and potential ModFs during PF will ensure that the advice provided based on the CRA results will have the most utility for decision making. Through well-constructed and well-conducted PF, it should be possible to undertake CRA in a timely and informative way, so that the output is of real value to risk managers.
Declaration of interest
This publication was authored collectively by participants of the ILSI Health and Environmental Sciences Institute (HESI) Risk Assessment in the 21st Century (RISK21) Technical Committee’s Cumulative Risk Sub-Team, whose work is supported by HESI, a nonprofit institution whose mission is to collaboratively identify and help to resolve global health and environment challenges through the engagement of scientists from academia, government, industry, NGOs and other strategic partners. HESI receives funding and in-kind support from member companies and other non-industry organizations to support projects. The employment affiliation of the authors is shown on the cover page. These individuals had the sole responsibility for the writing and content of the paper, with additional input provided by other participants of the RISK21 Technical Committee’s Cumulative Risk Sub-Team (see acknowledgments). The individual authors worked as professionals in preparing the article and not as agents of their employers. Travel expenses were provided for academic and government committee participants to attend committee meetings, and they did not receive any other compensation. This work has been presented at numerous international meetings, workshops and symposia to scientists and regulators from academia, industry and government.
Abbreviations | ||
AChE | = | acetylcholinesterase |
ADI | = | acceptable daily intake |
ADME | = | absorption, distribution, metabolism, excretion |
CRA | = | cumulative risk assessment |
CCAG | = | common chemical assessment group |
EFSA | = | European Food Safety Authority |
HESI | = | Health and Environmental Sciences Institute |
ILSI | = | International Life Sciences Institute |
IVIVE | = | in vitro to in vivo extrapolation |
IPCS | = | International Program on Chemical Safety |
NAS | = | United States National Academy of Sciences |
NRC | = | National Research Council |
ModFs | = | modulating factors |
PF | = | problem formulation |
RfD | = | reference dose |
RISK21 | = | Risk Assessment in the 21st Century |
TD | = | toxicodynamics |
TK | = | toxicokinetics |
USEPA | = | United States Environmental Protection Agency |
WHO | = | World Health Organization |
Acknowledgements
The authors gratefully acknowledge the government, academic and industry scientists of the HESI RISK21 Technical Committee for their contributions to this work. A full list can be accessed at www.risk21.org. In addition, the authors gratefully acknowledge the extensive comments offered by four reviewers selected by the Editor and anonymous to the authors. The comments were helpful in revising the paper.
References
- Alvarez AI, Real R, Perez M, Mendoza G, Prieto JG, Merino G. 2010. Modulation of the activity of ABC transporters (P-glycoprotein, MRP2, BCRP) by flavonoids and drug response. J Pharm Sci. 99:598–617.
- Anway MD, Cupp AS, Uzumcu M, Skinner MK. 2005. Epigenetic transgenerational actions of endocrine disruptors and male fertility. Science. 308:1466–1469.
- Barkin S, Schlundt D. 2011. The challenge facing translation of basic science into clinical and community settings to improve health outcomes. Environ Health Perspect. 119:A418–A419.
- Boobis A, Budinsky R, Collie S, Crofton K, Embry M, Felter S, Hertzberg R, Kopp D, Mihlan G, Mumtaz M, et al. 2011. Critical analysis of literature on low-dose synergy for use in screening chemical mixtures for risk assessment. Crit Rev Toxicol. 41:369–383.
- Callahan MA, Sexton K. 2007. If cumulative risk assessment is the answer, what is the question? Environ Health Perspect. 115:799–806.
- ECETOC (European Centre for Ecotoxicology and Toxicology of Chemicals). 2011. Workshop on combined exposure to chemicals. 11–12 July 2011, Berlin. Workshop Report No. 22; [cited 2016 Jul 19]. Available from: http://www.ecetoc.org/wp-content/uploads/2014/08/ECETOC_WR_22._Combined_exposure_to_chemicals.pdf.
- EFSA (European Food Safety Authority). 2008. Opinion of the scientific panel on plant protection products and their residues to evaluate the suitability of existing methodologies and, if appropriate, the identification of new approaches to assess cumulative and synergistic risks from pesticides to human health with a view to set MRLs for those pesticides in the frame of Regulation (EC) 396/2005. EFSA J. 704:1–84. Available from: http://www.efsa.europa.eu/en/efsajournal/pub/705.htm.
- EFSA (European Food Safety Authority), 2013. Scientific opinion on the identification of pesticides to be included in cumulative assessment groups on the basis of their toxicological profile. EFSA J. 11:3293. Available from: http://www.efsa.europa.eu/en/efsajournal/doc/3293.pdf.
- Embry MR, Bachman AN, Bell DR, Boobis AR, Cohen SM, Dellarco M, Dewhurst IC, Doerrer NG, Hines RN, Moretto A, et al. 2014. Risk assessment in the 21st century: roadmap and matrix. Crit Rev Toxicol. 44:6–16.
- Friedman M. 2006. Potato glycoalkaloids and metabolites: roles in the plant and in the diet. J Agric Food Chem. 54:8655–8681.
- Hastings DA. 2013. The human security index: pursuing enriched characterization of development. Development. 56:66–78.
- Henderson CJ, Wolf CR, Kitteringham N, Powell H, Otto D, Park BK. 2000. Increased resistance to acetaminophen hepatotoxicity in mice lacking glutathione S-transferase Pi. Proc Natl Acad Sci USA. 97:12741–12745.
- Jin MJ, Han HK. 2010. Effect of piperine, a major component of black pepper, on the intestinal absorption of fexofenadine and its implication on food-drug interaction. J Food Sci. 75:H93–H96.
- Koturbash I, Scherhag A, Sorrentino J, Sexton K, Bodnar W, Swenberg JA, Beland FA, Pardo-Manuel Devillena F, Rusyn I, Pogribny IP. 2011. Epigenetic mechanisms of mouse interstrain variability in genotoxicity of the environmental toxicant 1,3-butadiene. Toxicol Sci. 122:448–456.
- Lock EA, Wilks MF. 2010. Paraquat. In: Krieger RI, editor. Handbook of pesticide toxicology. Vol. 1, 3rd ed. Amsterdam (NL): Elsevier. p. 1771–1827.
- Maze I, Feng J, Wilkinson MB, Sun H, Shen L, Nestler EJ. 2011. Cocaine dynamically regulates heterochromatin and repetitive element unsilencing in nucleus accumbens. Proc Natl Acad Sci USA. 108:3035–3040.
- Meek ME, Boobis AR, Crofton KM, Heinemeyer G, Raaij MV, Vickers C. 2011. Risk assessment of combined exposure to multiple chemicals: a WHO/IPCS framework. Regul Toxicol Pharmacol. 60:S1–S14.
- Moretto A, Bachman A, Boobis A, Solomon KR, Pastoor TP, Phillips R, Wilks MF, Embry M. 2016. A framework for cumulative risk assessment in the 21st century. Crit Rev Toxicol, in press.
- NAS. National Academy of Sciences. 1983. Risk assessment in the federal government: managing the process. Washington (DC): National Research Council of the National Academies. 191 p.
- NEJAC. 2004. Ensuring risk reduction in communities with multiple stressors: environmental justice and cumulative risks/impacts. Washington (DC): US Environmental Protection Agency. National Environmental Justice Advisory Council. Report 178 p; [Internet]. [cited 2016 Jul 19]. Available from: http://www.epa.gov/sites/production/files/2015-02/documents/nejac-cum-risk-rpt-122104.pdf.
- NEJAC. 2010. Nationally consistent environmental justice screening approaches. Washington (DC): National Environmental Justice Advisory Council. A Federal Advisory Committee to the U.S. Environmental Protection Agency. Report 109 p; [Internet]. [cited 2016 Jul 19]. Available from: http://epa.gov/sites/production/files/2015-02/documents/ej-screening-approaches-rpt-2010.pdf.
- NRC. National Research Council. 2009. Science and decisions: advancing risk assessment. Washington (DC): The National Academies Press.
- Painter RC, De Rooij SR, Bossuyt PM, Simmers TA, Osmond C, Barker DJ, Bleker OP, Roseboom TJ. 2006. Early onset of coronary artery disease after prenatal exposure to the Dutch famine. Am J Clin Nutr. 84:322–327.
- Pastoor TP, Bachman AN, Bell DR, Cohen SM, Dellarco M, Dewhurst IC, Doe JE, Doerrer NG, Embry MR, Hines RN, et al. 2014. A 21st century roadmap for human health risk assessment. Crit Rev Toxicol. 44:1–5.
- Price P, Dhein E, Hamer M, Han X, Heneweer M, Junghans M, Kunz P, Magyar C, Penning H, Rodriguez C. 2012. A decision tree for assessing effects from exposures to multiple substances. Environ Sci Europe. 24:26. doi:10.1186/2190-4715-24-26.
- Price PS, Hollnagel HM, Zabik JM. 2009. Characterizing the noncancer toxicity of mixtures using concepts from the TTC and quantitative models of uncertainty in mixture toxicity. Risk Anal. 29:1534–1548.
- Renwick AG, Barlow SM, Hertz-Picciotto I, Boobis AR, Dybing E, Edler L, Eisenbrand G, Greig JB, Kleiner J, Lambe J, et al. 2003. Risk characterisation of chemicals in food and diet. Food Chem Toxicol. 41:1211–1271.
- Salinas JJ, Shah M, Abdelbary B, Gay JL, Sexton K. 2012. Application of a novel method for assessing cumulative risk. Int J Environ Res Public Health. 9:1820–1835.
- SCHER, SCCS, SCENIHR. 2012. Toxicity and assessment of chemical mixtures. Brussels: European Commission Directorate-General for Health & Consumers. 50 p; [Internet]. [cited 2016 Jul 19]. Available from: http://ec.europa.eu/health/scientific_committees/environmental_risks/docs/scher_o_155.pdf.
- Sheldon LS. 2010. Exposure framework. In: Krieger RI, Doull J, van Hemmen JJ, Hodgson E, Maibach HI, Ritter L, Ross J, Slikker W, editors. Handbook of pesticide toxicology. Vol. 1, 3rd ed. Burlington (MA): Elsevier. p. 971–976.
- Simon TW, Simons SS, Preston RJ, Boobis AR, Cohen SM, Doerrer NG, Fenner-Crisp PA, McMullin TS, McQueen CA, Rowlands JC. 2014. The use of mode of action information in risk assessment: quantitative key events/dose-response framework for modeling the dose-response for key events. Crit Rev Toxicol. 44:17–43.
- Skinner MK, Manikkam M, Guerrero-Bosagna C. 2011. Epigenetic transgenerational actions of endocrine disruptors. Reprod Toxicol. 31:337–343.
- Smeester L, Rager JE, Bailey KA, Guan X, Smith N, Garcia-Vargas G, Del Razo LM, Drobna Z, Kelkar H, Styblo M, Fry RC. 2011. Epigenetic changes in individuals with arsenicosis. Chem Res Toxicol. 24:165–167.
- Su JG, Morello-Frosch R, Jesdale BM, Kyle AD, Shamasunder B, Jerrett M. 2009. An index for assessing demographic inequalities in cumulative environmental hazards with application to Los Angeles, California. Environ Sci Technol. 43:7626–7634.
- Timchalk C, Poet TS. 2008. Development of a physiologically based pharmacokinetic and pharmacodynamic model to determine dosimetry and cholinesterase inhibition for a binary mixture of chlorpyrifos and diazinon in the rat. Neurotoxicology. 29:428–443.
- USEPA. 1992. Framework for ecological risk assessment. Washington (DC): United States Environmental Protection Agency. Report EPA/630/R-92/001. 41 p.
- USEPA. 2003. Framework for cumulative risk assessment. Washington (DC): Risk Assessment Forum, U.S. Environmental Protection Agency. Report EPA/630/P-02/001F. 129 p.
- USEPA. 2007. Concepts, methods and data sources for cumulative health risk assessment of multiple chemicals, exposures and effects: a resource document. Cincinnati (OH): U.S. Environmental Protection Agency, Office of Research and Development, National Center for Environmental Assessment. Report EPA/600/R-06/014F.
- USEPA. 2014. Framework for human health risk assessment to inform decision making. Washington (DC): U.S. Environmental Protection Agency Office of the Science Advisor Risk Assessment Forum. Report EPA/100/R-14/001; [Internet]. [cited 2016 Jul 19]. Available from: http://www2.epa.gov/sites/production/files/2014-12/documents/hhra-framework-final-2014.pdf.
- USEPA. 2016. EJSCREEN Environmental Justice Mapping and Screening Tool - EJSCREEN Technical Documentation, U.S. Environmental Protection Agency, Office of Policy; [Internet]. Available from: https://www.epa.gov/ejscreen/technical-documentation-ejscreen.
- Vanhees K, Godschalk RW, Sanders A, van Waalwijk van Doorn-Khosrovani SB, van Schooten FJ. 2011. Maternal quercetin intake during pregnancy results in an adapted iron homeostasis at adulthood. Toxicology. 290:350–358.
- Wason SC, Smith TJ, Perry MJ, Levy JI. 2012. Using physiologically-based pharmacokinetic models to incorporate chemical and non-chemical stressors into cumulative risk assessment: a case study of pesticide exposures. Int J Environ Res Public Health. 9:1971–1983.
- WHO (World Health Organization). 2009. A risk-based decision tree approach for the safety evaluation of residues of veterinary drugs. Geneva: WHO; [cited 2016 Jul 19]. Available from: http://www.who.int/foodsafety/chem/jecfa/decision_tree_mar_2009_final_for_web.pdf.
- WHO (World Health Organization). 2010. Urban HEART: Urban Health Equity Assessment and Response Tool. Kobe, Japan: WHO; [cited 2016 Jul 19]. Available from: http://www.who.or.jp/urbanheart.
- WHO (World Health Organization) IPCS (International Program on Chemical Safety). 2009. Environmental health criteria 240: principles and methods for risk assessment of chemicals in food. Geneva, Switzerland: World Health Organization. Report 16 p.
- Zartarian VG, Schultz BD, Barzyk TM, Smuts M, Hammond DM, Medina-Vera M, Geller AM. 2011. The Environmental Protection Agency's Community-Focused Exposure and Risk Screening Tool (C-FERST) and its potential use for environmental justice efforts. Am J Public Health. 101(Suppl 1):S286–S294.