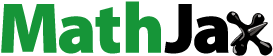
Abstract
Inorganic chloramines are not commercially available, but monochloramine is produced in situ for disinfection or for use in chemical synthesis. Inorganic chloramines are also formed when free chlorine reacts with nitrogen containing substances, e.g. ammonia and urea, present in chlorinated water sources. Occupational exposure may, therefore, occur in e.g. swimming pool facilities and the food processing industry. Monochloramine is soluble and stable in water and the dominating inorganic chloramine in chlorinated water sources. No clinical effects were seen in healthy volunteers given monochloramine in drinking water during 4 or 12 weeks in doses of 0.043 or 0.034 mg/kg bw/day, respectively. Limited data indicate that monochloramine is weakly mutagenic in vitro but not genotoxic in vivo. One drinking water study indicated equivocal evidence of carcinogenicity in female rats but not in male rats and mice. No reproductive or developmental effects were shown in rodents in the few studies located. Dichloramine is soluble but unstable in water. In the only study located, mild histological effects in kidneys, thyroid and gastric cardia were observed in rats administered dichloramine in drinking water for 13 weeks. Trichloramine is immiscible with water and evaporates easily from water into air. Therefore, the primary exposure route of concern in the occupational setting is inhalation. Occupational exposure to trichloramine has been demonstrated in indoor swimming pool facilities and in the food processing industry where chlorinated water is used for disinfection. Exposure-response relationships between airborne levels and self-reported ocular and upper airway irritation have been shown in several studies. Exposure to trichloramine may aggravate asthma symptoms in individuals with existing asthma. The risk of developing asthma following long-term exposure to trichloramine cannot be evaluated at present. No data on genotoxic, carcinogenic, reproductive or developmental effects were located. The toxicological data for mono- and dichloramine are insufficient to recommend health-based occupational exposure limits (OELs).
As regard trichloramine, the critical effect is judged to be irritation observed in several studies on pool workers, starting at approximately 0.4 mg/m3 (stationary sampling). Based on these data, a health-based OEL of 0.1 mg/m3 (8-h time-weighted average) is recommended. This corresponds to 0.2 mg/m3 for stationary measurements in swimming pool facilities. No short-term exposure limit (STEL) is recommended.
1. Introduction
Inorganic chloramines, i.e. monochloramine (NH2Cl), dichloramine (NHCl2) and trichloramine (NCl3), are formed when free1 chlorine reacts with nitrogen-containing substances present in e.g. chlorinated (disinfection) water sources. In the occupational setting, this may occur in swimming pool facilities (WHO Citation2006) and in the food processing industry (Héry et al. Citation1998, Citation1999; King et al. Citation2006; Massin et al. Citation2007). Inorganic chloramines may also be formed in industrial processes when liquid waste containing ammonium ions is mixed with a sodium hypochlorite solution (Okada et al. Citation2014). Monochloramine, dichloramine and trichloramine are not known to be commercial products but monochloramine is generated in situ as needed to disinfect drinking water and waste water (US EPA Citation1994; IARC Citation2004).
Monochloramine and dichloramine are water soluble of which the former is the dominating inorganic chloramine in the chlorinated water sources mentioned above. Trichloramine is immiscible with water, has a relatively high vapor pressure at room temperature and thus evaporates relatively fast into the air compartment (Holzwarth et al. Citation1984). Trichloramine is, therefore, the dominating inorganic chloramine in the indoor air of swimming pools (Héry et al. Citation1995; Carbonnelle et al. Citation2008). In the food processing industry, the fraction of trichloramine in air is considerably lower (Héry et al. Citation1998, Citation1999; King et al. Citation2006; Massin et al. Citation2007).
In recent years, there has been an increased reporting of health problems such as irritation and pulmonary effects among staff in indoor chlorinated swimming pool facilities and in the food processing industry where chlorinated water is used. Chlorination of water gives rise to a number of disinfection by-products also in air, mainly inorganic chloramines (WHO Citation2006; ANSES Citation2010; Richardson et al. Citation2010; Westerlund et al. Citation2015; Manasfi et al. Citation2017). The aim of this document is to evaluate health effects associated with occupational exposure to inorganic chloramines, and if possible, to recommend health-based occupational exposure limits (OELs).
2. Substance identification
Substance identification data for the inorganic chloramines are presented in .
Table 1. Substance identification data for the inorganic chloramines (NLM Citation2018).
3. Physical and chemical properties
Physical and chemical properties for the inorganic chloramines are presented in . Chloramines are powerful oxidants whose high redox potentials can oxidize many compounds including iodide ions, the latter being of interest for analytical purposes (Section 5.1.2) (Cimetière Citation2009; Kinani et al. Citation2012).
Table 2. Physical and chemical properties of the inorganic chloramines (Hand and Margerum Citation1983; Holzwarth et al. Citation1984; Budavari Citation1989; US EPA Citation1994; Suffet et al. Citation1995; Holleman et al. Citation2007).
3.1. Monochloramine
Monochloramine (NH2Cl) is a colorless to yellow liquid with a melting point of −66 °C. It is soluble in ethanol and ethyl ether, and slightly soluble in benzene and carbon tetrachloride. Pure monochloramine liquid and monochloramine vapors are unstable at room temperature, but the substance is readily soluble and stable in aqueous solution (Weast and Astle Citation1980; US EPA Citation1994; IARC. Citation2004).
A threshold odor level of 0.65 mg/l as chlorine gas (Cl2) in aqueous solution has been reported, but the substance rarely causes taste and odor problems in drinking water below 5 mg/l as Cl2 (Suffet et al. Citation1995).
Monochloramine oxidizes sulphhydryls and disulfides in the same manner as hypochlorous acid (Jacangelo et al. Citation1987).
3.2. Dichloramine
Dichloramine (NHCl2) is a yellow gas (no boiling point data were located) at room temperature. The gas is unstable and reacts with many materials (Holleman et al. Citation2007). Because of its instability it has been prepared only in aqueous solution (Hand and Margerum Citation1983).
The odor threshold is 0.15 mg/l as Cl2 in aqueous solution, which is much lower than that of monochloramine. Most people perceive the unpleasant chlorinous smell when the concentration is above 0.5 mg/l (Suffet et al. Citation1995).
3.3. Trichloramine
Trichloramine (NCl3) is an oily yellowish liquid at room temperature. It is immiscible with water and is soluble in benzene, chloroform, carbon tetrachloride, carbon disulfide and phosphorus trichloride. Trichloramine has a relatively high vapor pressure at ambient temperature and explodes when heated above 93 °C or when the liquid is exposed to sunlight (Budavari Citation1989). It evaporates approximately 300 times faster than monochloramine and 100 times faster than dichloramine from water into the air compartment (Holzwarth et al. Citation1984).
Trichloramine has been said to produce a geranium-like and chlorinous odor. The odor threshold is low compared to the other inorganic chloramines, 0.02 mg/l in water as Cl2 (Suffet et al. Citation1995).
4. Occurrence, production and use
4.1. Occurrence
4.1.1. General
Inorganic chloramines are formed when free chlorine reacts with nitrogen containing substances such as urea and ammonia (WHO Citation2006) present in chlorinated water sources such as drinking water and waste water, swimming pools and in the food processing industry where disinfecting or cleaning water is used (US EPA Citation1994; Héry et al. Citation1995, Citation1998, Citation1999; King et al. Citation2006; WHO. Citation2006; Massin et al. Citation2007). Inorganic chloramines may also be formed in industrial processes when liquid waste containing ammonium ions (NH4+) is mixed with a sodium hypochlorite solution (Okada et al. Citation2014).
The formation of inorganic chloramines is illustrated below exemplified with ammonia (NH3) as a nitrogen-containing substance and hypochlorous acid (HClO) as a chlorinated disinfectant (Héry et al. Citation1995).
The formation of inorganic chloramines in water is dependent on the chlorine-to-nitrogen ratio and pH. In general, the optimal pH for the formation of monochloramine lies in the range 7.5–9.0. Under the conditions of water and waste water chlorination, monochloramine is the principle inorganic chloramine encountered. Dichloramine has a maximum of formation at pH 4–6, and a pH <4.4 favors the formation of trichloramine (US EPA Citation1994; Cimetière Citation2009; Kinani et al. Citation2012).
National recommendations for pH values in chlorinated swimming pools vary slightly. All Nordic countries have a recommended upper pH limit of 7.6, with Norway and Sweden having the narrowest recommended range of 7.2–7.6 (Helse Citation1996; Naturstyrelsen Citation2013; Folkhälsomyndigheten Citation2014; Valvira Citation2017). At a chlorine-to-nitrogen ratio of <5:1 and a pH >7, almost exclusively monochloramine is formed. At a chlorine-to-nitrogen ratio of >5:1 and a pH <7, dichloramine is formed. At pH <7.2, an increased formation of trichloramine has been shown. When the chlorine-to-nitrogen molar ratio decreases, the formation of trichloramine decreases (US EPA Citation1994; Schmalz et al. Citation2011; Hansen et al. Citation2013).
An increased number of swimmers in a swimming pool increases the air concentration of trichloramine (Jacobs et al. Citation2007; Bessonneau et al. Citation2011; Weng et al. Citation2011; Chu et al. Citation2013; Fantuzzi et al. Citation2013; Afifi and Blatchley Citation2015).
4.1.2. Monochloramine and dichloramine
Monochloramine and dichloramine are water soluble and thus present in chlorine disinfected drinking water, chlorine disinfected swimming pool water and chlorinated water used for cleaning or disinfection purposes in the food processing industry. In the latter, mono- and dichloramine may account for a substantial part of the inorganic chloramines in air (Héry et al. Citation1998, Citation1999; King et al. Citation2006).
4.1.3. Trichloramine
Trichloramine is immiscible with water and has a relatively high vapor pressure at room temperature and evaporates relatively fast from water. Trichloramine is the dominating inorganic chloramine in the air of indoor swimming pool facilities (Carbonnelle et al. Citation2008). In the food processing industry, trichloramine constitutes approximately 30–70% of the chlorine species in air (Héry et al. Citation1995, Citation1998; King et al. Citation2006; Massin et al. Citation2007).
4.2. Production
4.2.1. Monochloramine
Monochloramine is not known to be a commercial product but is generated in situ as needed by the action of hypochlorous acid or chlorine gas on ammonia (IARC. Citation2004).
4.2.2. Dichloramine and trichloramine
No data were located.
4.3. Use
4.3.1. Monochloramine
Monochloramine is used as an alternative to chlorine as waste water and drinking water disinfectant and is formed in situ when free chlorine and ammonia is added to the water. This disinfection method results in less formation of trihalomethanes. Monochloramine is less effective as disinfectant than free chlorine, but is persistent and is therefore primarily used as secondary disinfectant to maintain a stable residual in water distribution systems (US EPA Citation1994; IARC. Citation2004). Monochloramine has also been used in the synthesis of a wide range of chemicals including vicinal organic chloramines, N-chlorimines and hydrazine. In 1990, it was estimated that 55,000 tons of monochloramine was consumed worldwide in the production of hydrazine (Wojtowicz Citation1993).
There has been no registered use of monochloramine in the SPIN (Substances in Preparations in Nordic Countries) database (SPIN database (GINA) Citation2018).
4.3.2. Dichloramine
No data were located.
4.3.3. Trichloramine
The agene process was formerly used for bleaching flour with trichloramine. The practice was discontinued in 1950 once it became known that agene-treated flour may cause severe neurological symptoms in dogs (canine hysteria) and other species. The denaturated protein (methionine sulphoximime) in the treated flour used for production of dog biscuits was identified as the toxic compound, and there were also concerns for potential adverse effects on human health (Shaw and Bains Citation1998).
There has been no registered use of trichloramine in the SPIN database (GINA) (Citation2018).
5. Measurements and analysis of workplace exposure
5.1. Air monitoring
The method most often used today to determine inorganic chloramines in air was developed by Héry and coworkers (Héry et al. Citation1995, Citation1998). It is an indirect method as chloride ions are determined.
5.1.1. Monochloramine and dichloramine (soluble chlorine)
To determine soluble chlorine2 by the method of Héry et al. (Citation1995, Citation1998), air is sampled through a tube with silica gel coated with sulfamic acid. Following sampling the tube is desorbed in a sulfamic acid solution and the concentration of soluble chlorine is determined by potentiometry with a chlorine electrode and a pH/mV meter (Héry et al. Citation1998; INRS Citation2017) or by colorimetry (Chu et al. Citation2013).
5.1.2. Trichloramine
When determining the trichloramine concentration in air according to Héry et al., interference from soluble chlorine is avoided by the tube with silica gel impregnated with sulfamic acid (Section 5.1.1) in the front of a filter cassette which traps these substances. A Teflon filter prevents any chlorides contained in airborne water droplets from being included in the analysis of trichloramine and is discarded after sampling. For sampling of trichloramine, a quartz filter in the cassette is impregnated with a solution of sodium carbonate and diarsenic(III) trichloride. On the filter, trichloramine is reduced to chloride ions. Following desorption of the filter with deionized water, chloride is determined by ion chromatography with conductivity or capillary electrophoresis detection to estimate the air concentration of trichloramine (Héry et al. Citation1995, Citation1998; INRS Citation2017).
The limit of detection (LOD) for trichloramine using the method developed by Héry et al. (Citation1995, Citation1998) depends on the air volume sampled and the ion chromatographic method used but is generally in the range of 0.0017–0.01 mg/m3 (Héry et al. Citation1995; Bessonneau et al. Citation2011; Catto et al. Citation2012; Nordberg et al. Citation2012; Parrat et al. Citation2012; Chu et al. Citation2013; Afifi and Blatchley Citation2015).
Predieri and Giacobazzi (Citation2012) developed an impinger method for determination of trichloramine in the workplace atmosphere. In the impinger flask, trichloramine reacts with potassium iodide in water solution. The released iodine reacts with diethyl-p-phenylenediamine and produces a pink coloration. The coloration is proportional to the amount of trichloramine in sampled air and is determined by spectroscopy. The LOD was 0.0036 mg/m3 at an air sampling volume of 100 liters. Other chlorinated inorganic or organic compounds such as dichloroacetonitrile, cyanogen chloride, dichloroacetic acid, trichloroacetic acid or dichloromethylamine present in the air (Cardador and Gallego Citation2011; Weng et al. Citation2011; Afifi and Blatchley Citation2015) are suspected to interfere with the analysis leading to an overestimation of the trichloramine exposure. The percentage of interference was, however, not determined.
There is also a portable analysis tool that allows regular monitoring of trichloramine in air. The analyte is retained on quartz fiber filters, released in deionized water and subsequently analyzed by colorimetry. The minimum required sampling time is 45 min (INRS. Citation2011; Syclope Electronique Citation2019).
Air monitoring has mostly been performed as stationary sampling. The results may deviate from those obtained by personal sampling. Linear regression analysis based on data from 12 parallel 8-h samplings of trichloramine in pool air suggested a relation between personal and stationary sampling of 1:1.6 (Westerlund et al. Citation2015). In a similar study by the same group, a linear regression based on 8 personal and 14 stationary 8-h samplings showed a corresponding relation of 1:2.2 between personal and stationary sampling (Westerlund et al. Citation2019). Only data from personnel who spent >50% of their workday in the pool area were included in the analyses.
5.2. Biological monitoring
For saliva and gastric fluid samples, membrane introduction mass spectrometry and tandem mass spectrometry has been used (US EPA Citation1994).
Monochloramine, dichloramine and monobromamine were determined in real-time in single human breaths by selected ion flow tube mass spectrometry. Preliminary measurements showed concentrations of 10–150 ppb of these substances in the breath of volunteers (healthy or with chronic obstructive pulmonary disease). Limits of detection approached 10 ppb (Senthilmohan et al. Citation2008). For trichloramine, 10 ppb corresponds to 0.05 mg/m3.
6. Occupational exposure data
Trichloramine is the dominating inorganic chloramine in the indoor air of swimming pools. The remaining fraction as sampled by the method of Héry et al. (Citation1995, Citation1998) is soluble chlorine (monochloramine, dichloramine, hypochlorite/hypochlorous acid and chlorine). Carbonnelle et al. (Citation2008) reported that trichloramine accounted for about 90% of the chlorine species in swimming pool air. In the food processing industry, the corresponding figure is 30–70%. These lower percentages are due to intense stirring and spray washing of the chlorinated water, which may cause transfer of mono- and dichloramine into the atmosphere as vapors and aerosols (Héry et al. Citation1995, Citation1998; King et al. Citation2006; Massin et al. Citation2007). Massin et al. (Citation2007) carried out personal exposure measurements during different work tasks such as foaming and rinsing in the food industry. The exposure to the sum of soluble chlorine and trichloramine was 0.01–5.46 mg/m3 (unclear if expressed as trichloramine, or chlorine, equivalents).
6.1. Monochloramine and dichloramine (soluble chlorine)
Héry et al. (Citation1998) performed air sampling of soluble chlorine in a green salad production plant. Personal air sampling was done during a half work shift and showed concentrations of <0.1–3.7 mg/m3. Stationary sampling for 1–8 h showed air concentrations of 0.1–10.9 mg/m3.
Personal exposures to soluble chlorine were 0.02–0.16 mg/m3 during fish curing, 0.03–0.85 mg/m3 in slaughter houses, 0.04–1.33 mg/m3 in poultry processing, 0.03–0.17 mg/m3 in pet food production and <0.01–0.30 mg/m3 in delicatessen trade (Héry et al. Citation1999).
King et al. (Citation2006) performed personal sampling of soluble chlorine among poultry processing workers with exposures of 0.010–0.13 mg/m3.
Chu et al. (Citation2013) reported mean (± standard deviation; SD) air concentrations of soluble chlorine (stationary sampling) of 0.072 ± 0.052 mg/m3 in 10 indoor swimming pools and 0.085 ± 0.056 mg/m3 in 6 spa pools.
Air concentrations of soluble chlorine in an indoor waterpark resort ranged from non-detectable to 0.25 mg/m3 (Dang et al. Citation2010).
6.2. Trichloramine
Air measurements of trichloramine have predominantly been performed in indoor swimming pool facilities (during pool work and swimming) and in the food processing industry (during cleaning and disinfection) (). The air measurement data presented in this section are retrieved from studies in which health effects of trichloramine were also studied.
Table 3. Air concentrations of trichloramine in indoor swimming pool facilities and in the food processing industry in different countries.
Most stationary air measurements during pool work were made at spots where the personnel are likely to be located during a work day. The sampling times were typically 1.5–3 h. The exposure levels (arithmetic mean; AM) were usually 0.15–0.65 mg/m3 for public and school baths and 0.2–1.25 mg/m3 for adventure baths (Héry et al. Citation1995, Massin et al. Citation1998; Thickett et al. Citation2002; Jacobs et al. Citation2007; Valkeinen et al. Citation2007; Dang et al. Citation2010; Bessonneau et al. Citation2011; Weng et al. Citation2011; Catto et al. Citation2012; Nordberg et al. Citation2012; Parrat et al. Citation2012; Chu et al. Citation2013; Fantuzzi et al. Citation2013; Fornander et al. Citation2013; Afifi and Blatchley Citation2015; Lévesque et al. Citation2015; Westerlund et al. Citation2015). In two studies in indoor swimming pool facilities, personal sampling was used in parallel with stationary sampling. Overall, the personal exposure was approximately 50% of the levels obtained by stationary sampling (Section 5.1.2) (Westerlund et al. Citation2015, Citation2019).
Air measurements of trichloramine during competitive and recreational swimming have been performed as stationary sampling at spots close to the swimming pool. Exposure levels during swimming were similar to those measured during pool work, i.e. 0.2–1.30 mg/m3 (Carbonnelle et al. Citation2002; Bernard et al. Citation2003, Citation2006; Lévesque et al. Citation2006; Bernard et al. Citation2007; Carbonnelle et al. Citation2008; Bernard et al. Citation2009; Font-Ribera et al. Citation2009; Jacobs et al. Citation2012; Seys et al. Citation2015; Llana-Belloch et al. Citation2016; Gomà et al. Citation2017).
In a green salad processing plant, the personal exposure was <0.1–2.3 mg/m3 of trichloramine during a half work shift. Stationary sampling showed air concentrations of 0.1–5.9 mg/m3. Sampling times were 1–8 h (Héry et al. Citation1998).
In another study, personal exposures were <0.05–1.31 mg/m3 in slaughter houses, <0.05–0.24 mg/m3 during fish curing, 0.01–2.0 mg/m3 in poultry production, <0.01–0.11 mg/m3 in pet food production and 0.03–0.59 mg/m3 in delicatessen trade (Héry et al. Citation1999). Sampling times were 10–160 min and may thus not represent full-shift exposures. Short-time sampling is however useful to evaluate the exposure during specific work tasks.
King et al. (Citation2006) performed full-shift personal sampling of trichloramine in the poultry industry. The geometric mean (GM) ranged from non-detectable to 0.16 mg/m3.
7. Toxicokinetics
7.1. Human data
No human data were located, but inhalation is the dominating route of exposure to inorganic chloramines in the occupational setting. Exposure to monochloramine may also occur orally, via drinking water and via aspiration of water in swimming pools.
7.2. Animal data
7.2.1. Monochloramine
No inhalation or dermal data were located.
In the only study located, Abdel-Rahman et al. administered a single oral gavage total dose of 1.1 mg (∼5 mg/kg bw) radiolabelled monochloramine (NH236Cl) to each of 4 male Sprague Dawley rats. Blood samples were collected at 15, 30 and 60 min and 2, 4, 8, 16, 24, 48, 72, 96 and 120 h following administration. In a parallel experiment, a similar amount of radiolabelled monochloramine was administered to another 4 rats. Expired air samples and fecal and urine samples were collected at 8, 16, 24, 48, 72, 96 and 120 h.
A peak 36Cl plasma level was reached 8 h after administration and the absorption rate constant was 0.278 mg/hour with an absorption half-time of 2.5 h. The 36Cl plasma level remained at a plateau 8–48 h after administration.
At 24 h following administration, the 36Cl plasma level was 0.87% of the administered dose. At 120 h, the highest 36Cl activity was measured in plasma and whole blood and the lowest in the liver, ileum and adipose tissue. After 48 h, the radiolabel was eliminated from the plasma with a half-time of 38.8 h. Most of the total 36Cl was identified as 36Cl− which according to the authors indicated that the chlorine moiety was eliminated primarily in this form. Of the administered dose, 25% was excreted in urine and 1.98% in feces within 120 h. 36Cl was not detected in expired air throughout the experiment (Abdel-Rahman et al. Citation1983).
There is a lack of information on the rate of chloramine-chloride exchange (NH236Cl + Cl− ⇄ NH2Cl + 36Cl) in solutions of high chloride concentrations. If the exchange rate is significant, the toxicokinetics of NH236Cl could appear to resemble that of chloride, when, in fact, the compound has lost its radiolabel through chloramine-chloride exchange.
7.2.2. Dichloramine and trichloramine
No data were located.
7.3. In vitro data
The persistence of monochloramine in saliva and gastric fluid was examined. Pooled samples of human saliva or gastric fluid samples were exposed to 1.0–20 mg/l of monochloramine. The samples were continuously monitored using membrane introduction mass spectrometry and tandem mass spectrometry in a multiple monitoring procedure. Monochloramine in saliva was completely depleted in approximately 5 min at the 1-mg/l level. The 5-mg/l solution was incompletely depleted in 2 h and the higher concentration solutions (not specified) were unaffected. Monochloramine in gastric fluid disappeared completely in <30 s at all concentrations. Dichloramine, trichloramine and molecular chlorine were not observed in saliva or gastric fluid during depletion (US EPA Citation1994).
8. Biological monitoring
As there are no human toxicokinetic data for inorganic chloramines, there is no basis for biological exposure monitoring of the substances at present.
Several effect markers have been suggested, none of which are specific for the inorganic chloramines.
Pneumoproteins, serum proteins especially produced in the respiratory tract, such as club cell protein 16 kDa (CC16) and surfactant-associated proteins (SP-A, SP-B and SP-D), have been suggested as biomarkers of the hyperpermeability that occurs in the deep lung (Bernard et al. Citation2005). CC16 is an anti-inflammatory protein secreted by club cells (formerly called Clara cells) in the airways and predominantly in terminal bronchioles from where it leaks into serum (Bernard and Lauwerys Citation1995). SP-A is the major surfactant-associated protein mainly secreted by alveolar type II cells and is considered to collaborate with SP-B and SP-C to spread and stabilize the phospholipid layer at the alveolar surface and thus reduce the surface tension (Hermans and Bernard Citation1999). CC16 and SP-A are largely, and SP-B exclusively, confined to the lungs, whereas SP-D is expressed by a number of tissues (Hermans et al. Citation2003). Small amounts of CC16, SP-A, SP-B and SP-D occur in blood, and these pneumoproteins have been validated as blood markers of lung hyperpermeability in a variety of lung disorders caused by different lung toxicants (Hermans and Bernard Citation1998). CC16 can also be used as a peripheral marker of the integrity of club cells (Hermans et al. Citation1999).
Elevated levels of exhaled nitric oxide are associated with increased release from epithelial cells of the bronchial wall and reflect chronic eosinophilic airway inflammation (Kharitonov and Barnes Citation2000). Measurement of the fraction of exhaled nitric oxide (FENO) is a noninvasive and standardized test used in clinical practice for diagnosis and management of asthma (Sandrini et al. Citation2010). FENO has been suggested as a possible marker of airway inflammation due to exposure to irritant agents and has been associated with airway responsiveness in lifeguards (Demange et al. Citation2009).
Analysis of protein changes in nasal lavage fluid was applied in a study on pool workers by Fornander et al. (Citation2013) Nasal lavage fluid was collected from 9 pool workers and 4 control subjects. Protein profiling of nasal lavage fluid showed altered distribution of three innate immunity proteins; the levels of α-1-antitrypsin and lactoferrin were significantly higher and that of S100 calcium binding protein A8 (S100-A8, also called calgranulin A) was significantly lower in the pool workers than in the control subjects. These effects were most pronounced in subjects with airway irritation.
Evaluation of the predominating cell types within the nasal mucosa can indicate inflammation (rhinitis) due to occupational irritants. Nasal smear is collected for cytology and assessed using light microscopy. Erkul et al. (Citation2014) used nasal cytology and found that pool workers had significantly more eosinophils in nasal smear than non-exposed workers, indicating allergic inflammation in the nasal mucosa.
9. Mechanisms of toxicity
Inorganic chloramines are potent sensory irritants that cause eye and upper respiratory tract irritation by interaction with neuronal sensors located in the mucous membranes of the respiratory tract and the eyes (shown for mono- and trichloramine) (Eichelsdörfer et al. Citation1975; Gagnaire et al. Citation1994).
Chloramines are membrane penetrating oxidants and react rapidly with sulphhydryl groups of proteins in the cytoskeleton and the extracellular matrix causing disruption of tight junctions and an almost immediate increase in epithelial permeability, as shown in in vitro studies [(Tatsumi and Fliss Citation1994; Nakamura et al. Citation1995; Musch et al. Citation1999), as cited by Carbonnelle et al. (Citation2002)].
9.1. Monochloramine
Adverse health effects (methemoglobinaemia resulting in hemolytic anemia) have been shown in long-term hemodialyzed patients exposed to monochloramine through chloraminated dialysis water (Eaton et al. Citation1973; Kjellstrand et al. Citation1974; Tipple et al. Citation1991). Monochloramine induces these effects through oxidation of hemoglobin and inhibition of the hexose monophosphate shunt which protect the red blood cells from oxidant damage through generation of reduced nicotinamide adenine dinucleotide phosphate (Eaton et al. Citation1973; Kjellstrand et al. Citation1974). However, these effects were reported following a specific route of exposure (dialysis) and are not considered relevant for workplace exposure.
Piva et al. (Citation1991) investigated the effect of monochloramine on the glutamine and glucose transport systems in HeLa cells (human cervical cancer cell line) and rat mesenteric lymphocytes. Slight inhibition of both glutamine and glucose transport systems was initially observed in both the HeLa cells and lymphocytes. When the HeLa cells were pre-exposed to monochloramine, its inhibitory action increased. Similar results were obtained in the lymphocytes, suggesting that the effects of monochloramine are not cell specific. Only the sodium ion-independent (system L) component of the glutamine transport activity in HeLa cells was inhibited by monochloramine, and neither inhibition of cell metabolism nor enhanced cell lysis was observed suggesting that monochloramine inhibits cellular transport activity by binding to thiols (sulphhydryl groups) on the membrane.
9.2. Dichloramine
No data were located.
9.3. Trichloramine
Besides the established irritant potency of trichloramine, it has been hypothesized that exposure to chlorination products in indoor pools may promote the development of asthma in children and swimmers (Bernard et al. Citation2003). A basic mechanism underlying the associations could be a repeated or chronic disruption of the lung epithelial barrier facilitating the penetration of allergens in the lung, and resulting in a loss of pneumoproteins (Section 8). In contrast to mono- and dichloramine, trichloramine is almost completely immiscible with water and cannot easily penetrate the ciliated surface and epithelial lining fluid of airways. Thus, trichloramine may exert its toxic action in the deep lung where the cells are non-ciliated and the tight junctions more accessible. This hypothesis is supported by the observation that allergic sensitization is facilitated in the case of allergens having proteolytic activity, a characteristic which allows them to degrade extracellular matrix proteins and cross the airway epithelium more readily (Bernard et al. Citation2003). The low water solubility of trichloramine also explains why it mainly increases the serum levels of pneumoproteins associated with the deep lung (SP-A, SP-B, SP-D), and much less that of CC16 predominantly produced in the terminal bronchioles (Bernard et al. Citation2005).
10. Effects in animals and in vitro studies
Important studies are summarized in .
Table 4. Effects in animals after exposure to monochloramine.
Table 5. Effects in animals after exposure to dichloramine.
Table 6. Effects in animals after exposure to trichloramine.
10.1. Irritation and sensitization
10.1.1. Monochloramine
Clear conjunctival irritation was observed when the eyes of rabbits (5–6 animals/group) were constantly wetted with a monochloramine solution corresponding to 4 mg/l of Cl2 for 1 h, whereas a 2-mg/l solution did not produce eye irritation. Monochloramine was considerably more irritating than free chlorine (Eichelsdörfer et al. Citation1975). Female SENCAR mice submerged (except head) in monochloramine solutions in concentrations up to 1000 mg/l for 10 min for 4 consecutive days did not display epidermal hyperplasia like mice exposed to e.g. hypochlorous acid did (Robinson et al. Citation1986).
10.1.2. Dichloramine
No data were located.
10.1.3. Trichloramine
Bradypnoea (slow breathing), indicative of upper airway irritation in mice, was evaluated during a 60-min oronasal exposure to increasing concentrations of chlorine (5.1–44 mg/m3) or trichloramine (4.5–25 mg/m3). The airborne concentration causing a 50% decrease in respiratory frequency (RD50) in mice was calculated for each chemical. Chlorine and trichloramine showed different time-courses in their responses. While the maximal response of trichloramine was reached in 10 min, the maximal response of chlorine was reached after 45–60 min of exposure. The RD50 values of chlorine and trichloramine were 17.5 and 12.5 mg/m3, respectively. The authors concluded that trichloramine appeared to be a strong sensory irritant (Gagnaire et al. Citation1994).
10.2. Effects of single exposure
10.2.1. Monochloramine
No inhalation data were found.
Male Sprague Dawley rats (4/group) were given a single dose of 3 ml of water with a monochloramine concentration of 0, 10, 20 or 40 mg/l by gavage (corresponding to ∼0.19, 0.38 and 0.75 mg/kg bw). Blood was sampled 15, 30, 60 and 120 min after the administration for analysis of glutathione (GSH) and osmotic fragility. The GSH level was significantly increased 15 min after administration of 20 or 40 mg/l and after 30 and 60 min in all dose groups. After 2 h, the GSH level returned to normal. The authors suggested that the increase of GSH is explained by an increase in the activity of glutathione reductase to compensate for the oxidative stress of monochloramine during the first hour after exposure. Osmotic fragility was without any change in all dose groups (Abdel-Rahman et al. Citation1984).
10.2.2. Dichloramine
No data were located.
10.2.3. Trichloramine
Sprague Dawley rats (5/sex/group) were exposed to clean air or average air concentrations of 290, 560, 570, 620 or 785 mg/m3 of trichloramine in a glass exposure chamber during 1 h. Of the animals exposed to 785 mg/m3, 8 out of 10 died during the exposure and the remaining 2 within 23 min after termination of exposure. In the groups exposed to 560–620 mg/m3, the mortality rate was 40–80%. All animals survived exposure at 290 mg/m3. During the 4-h post-exposure period, labored breathing and yellowish stains of the anogenital region were frequently noted. Additional observations were gasping, rapid breathing, mucoid or red nasal discharge, excessive salivation and lacrimation, shedding of red tears, reduced activity and convulsive movements. The surviving animals were observed during 14 d after exposure, and dry rales, red or mucoid nasal discharge, rapid or labored breathing and soft stool were noted. All animals that died exhibited red mottling of the lungs and clear fluid in the trachea or the lungs. Many showed distension of the gastrointestinal tract at and below the level of the stomach. According to the authors the respiratory tract appears to be a primary site of damage in rats exposed to trichloramine. All animals that died showed pulmonary edema. The lethal concentration for 50% of the exposed animals at single inhalation exposure (LC50) was estimated to be 560 mg/m3, 95% confidence interval (CI) 535–585 mg/m3 (Barbee et al. Citation1983).
In an experiment performed by Carbonelle et al., 2-month-old female C57Bl/6 mice were exposed to trichloramine in an inhalation exposure chamber. In a first experiment, groups of 9 mice were exposed to clean air or to 11.9 mg/m3 of trichloramine during 1, 2, 4 or 8 h and sacrificed immediately after exposure. Blood was collected by cardiac puncture and bronchoalveolar lavage (BAL) was performed. Latex immunoassay techniques were used to determine albumin levels in BAL fluid (BALF) and CC16 levels in both serum and BALF. Total protein and lactate dehydrogenase (LDH) levels were determined in BALF. Serum CC16 levels increased significantly during exposure, peaking after 4 h at levels on average 2.5 times the pre-exposure level. CC16 levels in BALF decreased with a maximal reduction of about 80% after 8 h of exposure. Statistically significant elevations of both albumin and total protein levels in BALF after 8 h were observed. According to the authors, the lung epithelium hyperpermeability caused by trichloramine was not associated with cell cytotoxicity, since the LDH levels in BALF remained normal throughout the experiment. No evidence of lung toxicity was seen at light microscopy. In a parallel experiment, groups of 10 mice were exposed for 4 h either to filtered air or to 0.53, 0.8, 3.45 and 13.1 mg/m3 of trichloramine. The observations in the previous experiment were reproduced when mice were exposed to 13.1 mg/m3 but were not found at 0.53–3.45 mg/m3 (Carbonnelle et al. Citation2002).
To determine the in vitro toxicity of trichloramine, human alveolar cancer cells (A-549) were exposed to 0.1–40 mg/m3 of the substance by passing test atmospheres over the cells in a continuous-flow-module during 1 h. Nitrogen dioxide and synthetic or clean air were used as positive and negative control, respectively. Cell viability and inflammatory response manifested as interleukin (IL)-6 or IL-8 release were investigated 2, 24 and 48 h post-exposure. A decreasing cell viability with increasing trichloramine concentration was observed. Increased IL-8 and IL-6 levels were demonstrated >10 mg/m3 and at 20–30 mg/m3, respectively. Exposure >30 mg/m3 inhibited the release of both IL-6 and IL-8 due to cytotoxicity. Corresponding investigations with indoor swimming pool air showed similar inflammatory effects in the lung cells at doses <0.2 mg/m3 trichloramine. The authors concluded that there are additional substances present in the indoor pool air that contribute to the inflammatory response (Schmalz et al. Citation2011).
10.3. Effects of short-term exposure (up to 90 d)
10.3.1. Monochloramine
No inhalation data were found.
Adult African Green monkeys (5 males and 7 females) were administered drinking water containing 100 mg/l of monochloramine daily during 6 weeks giving a total dose of 10 mg/kg bw/day. Blood samples were analyzed for different hematological and clinicochemical parameters (red cell count, cell indices, reticulocytes, methemoglobin and hemoglobin, white cell and differential counts, red cell GSH content, creatinine and blood urea nitrogen (BUN), total bilirubin, total protein, albumin, alkaline phosphatase, LDH and aspartate and alanine aminotransferases) and serum thyroxine (T4). No effects were seen in any of these parameters or on body weight. Each animal served as its own control (Bercz et al. Citation1982).
Monochloramine was given in drinking water at 2.5–200 mg/l (∼0.44–35 mg/kg bw/day) to A/J mice (12 males/group) for 30 days. With the exception of body weight loss and increased hematocrit at doses ≥50 mg/l, all indicators of prehemolytic or hemolytic stress (i.e. 10 hematological parameters including osmotic fragility and GSH levels) failed to show any significant exposure-related changes. In the case of hematocrit, which indicates anemia if it is lowered, values actually increased (Moore et al. Citation1980).
Body weight gain and hematological parameters in rats exposed 45 d to drinking water containing 10, 50 or 100 mg/ml (∼1.2, 6.0, 12 mg/kg bw/day) of monochloramine did not differ from those in control animals. The only significant finding was a decrease in methemoglobin in blood, the opposite of what was expected (Bull Citation1980).
Young male Sprague Dawley rats (12/group) were daily given drinking water containing 0, 9, 19 or 38 mg/l (∼0.8, 1.7, 3.4 mg/kg bw/day) of monochloramine ad libitum during 9 weeks. The water consumption and estimated daily doses were not given. Parameters of immunity measured were spleen and thymus weights, antibody production, delayed-type hypersensitivity reactions, natural killer cell cytotoxicity, oxidative metabolism response and phagocytosis by macrophages, and production of two immunoregulatory cytokines (IL-2 and prostaglandin E2). Rats had reduced relative spleen weight (38 mg/l), decreased serum immunoglobulin G (IgG) antibody production (9 and 19 mg/l) and augmented prostaglandin E2 production by adherent resident peritoneal cells (19 and 38 mg/l). The authors concluded that monochloramine is not a particularly strong immunodepressant as the effects were observed at relatively high doses (Exon et al. Citation1987).
Immunotoxic effects were examined in groups of 8 female B6C3F1 mice administered monochloramine via drinking water at 2–200 mg/l (∼0.38–38 mg/kg bw/day) for 28 d. No significant differences in drinking water consumption, body weight, body weight gain, organ weights or hematological parameters were noted and some minimal immunological effects (mixed-leukocyte response and activity of natural killer cells) were judged to be biologically insignificant (Guo et al. Citation2011).
Miller et al. (Citation1986) studied drinking water collected at a pilot-scale drinking water plant using monochloramine for disinfection. The residual monochloramine level was 2.1 mg/l. The samples were concentrated by reverse osmosis (100 and 400 times) before administered to CD-1 mice (10/sex) as drinking water for 30 days. There were some significant differences in relative organ weights but no consistent pattern was observed.
10.3.2. Dichloramine and trichloramine
No data were found.
10.4. Genotoxicity
10.4.1. Monochloramine
10.4.1.1. In vitro studies
Monochloramine mutagenesis was tested using different strains of Bacillus subtilis. Prepared early stationary phase cells were diluted 10 times into monochloramine solutions (0, 18, 37, 56, 74 and 92 µM) and treated for 30 min at 37 °C. The reaction was stopped by adding one volume of 0.02 M sodium thiosulfate. Viable cell counts were scored by averaging the colonies at two plates (amino acid media) after incubation at 37 °C for 2 d. The minimum number of colonies per plate was 161 ± 8%. The authors concluded that monochloramine is a weak mutagen in the trpC locus, when reversion of trpC to trp+ in B. subtilis is used as an assay (Shih and Lederberg Citation1976).
Thomas et al. (Citation1987) tested the mutagenic activity of monochloramine in Salmonella typhimurium TA100. Bacteria were incubated with 40 µM monochloramine for 1 h at 37 °C. Duplicate samples were added to top-agar with histidine and biotin. Negative control (no mutagen) values were 140 ± 40 colonies and positive control values with sodium azide was 2 800 ± 700 colonies. Monochloramine showed a low or no mutagenic activity. The authors concluded that monochloramine is highly reactive thus unlikely to penetrate deeply into body tissues when in contact with skin and mucous membranes.
A study was designed to examine whether monochloramine could damage the DNA of gastric cells. Rabbit gastric mucosal cells (RGMC) or human gastric carcinoma cells (KATO III) were cultured and suspended. Cell suspensions were exposed to 0.1 mM hypochlorite, ammonia or monochloramine for 15 min, respectively. Monochloramine significantly induced DNA double strand breaks as well as chromatin condensation evoked by DNA fragmentation in the RGMC and KATO III cells (p < 0.05). Ammonia or hypochlorite had no such effects on these cell types. Monochloramine, but not ammonia or hypochlorite enhanced the levels of DNA injury (Suzuki et al. Citation1997).
Shibata et al. (Citation1999) treated plasmid pUC18 DNA with 3 mM monochloramine, which induced double strand DNA breaks.
The genotoxicity tests used in the cited studies above are all indicator tests. A full evaluation of a chemical’s ability to induce the possible types of genetic damage involved in adverse human health outcomes (cancer, non-cancer diseases involving somatic cell mutation, and heritable diseases) includes tests that can detect gene mutations, chromosomal damage and aneuploidy. To adequately cover all the genetic endpoints, one must use multiple tests (i.e. a test battery); as no individual test can provide information on all endpoints. The results from the cited studies cannot be used to evaluate effects on genotoxicity in humans.
10.4.1.2. In vivo studies
Meier et al. (Citation1985) performed a series of assays evaluating the induction of chromosomal aberrations (4/sex/group) and micronuclei (5/sex/group) in the bone marrow of Swiss CD-1 mice. The animals were dosed with 1 ml of a test solution containing 0, 40, 100 or 200 mg/l (1.6, 4 or 8 mg/kg bw) of monochloramine by oral gavage for 5 consecutive days and were sacrificed after the final exposure. An acute exposure test administration was also done for the bone marrow aberration test by giving the animals 1 ml of each test solution. The animals were killed 6, 24 and 48 h after dosing. The authors concluded that there was no evidence of any effect caused by exposure to monochloramine in any of the test performed. Monochloramine was also negative in the sperm-head abnormality assay (described further in Section 10.6.1).
Gauthier and coworkers evaluated the clastogenicity of water treated with sodium hypochlorite or monochloramine (0.05, 0.1 or 0.15 µg/ml) using a micronucleus test in newt larvae (Pleurodeles waltl) reared for 12 d. Frequencies of micronucleated red blood cells were evaluated per 1000 cells. The level of micronuclei increased with increasing concentration of monochloramine. Only the 0.15-µg/ml concentration gave a statistically significant response. The authors concluded that monochloramine is responsible for the clastogenic effect in newt larvae (Gauthier et al. Citation1989). The use of newt larvae to evaluate the clastogenicity of a chemical is not accepted by the OECD Guidelines.
In addition, there is only one in vivo study regarding genotoxicity testing in mammals located in our literature search. Thus, it is not possible to evaluate a possible genotoxic effect following exposure to monochloramine in humans.
10.4.1.3. Conclusion on monochloramine
Monochloramine was a weak mutagen in B. subtilis and S. typhimurium, and induced double-strand breaks in plasmid DNA, as well as DNA fragmentation, double strand DNA breakage and chromatin condensation in gastric mucosa cells. Monochloramine did not induce chromosomal aberrations, micronuclei or sperm abnormality in mice but induced micronuclei in newt larvae. Thus, limited data indicate that monochloramine is weakly mutagenic in vitro but not genotoxic in vivo.
10.4.2. Dichloramine and trichloramine
No data were located.
10.4.3. Drinking water and swimming pool water
More than 600 disinfection by-products have been identified in drinking water, many of which are carcinogenic, and some of them are also genotoxic. A large number of studies have examined the mutagenicity of drinking water extracts or concentrates disinfected by chlorination. The few studies available on drinking water prepared by alternative disinfection methods (including chloramination) all showed that such drinking water was considerably less mutagenic than chlorinated drinking water. Although the chloramination reduced the formation of trihalomethanes and haloacetic acids, it may cause increased levels of other disinfection by-products, e.g. nitrosamines, several of which are carcinogenic (Richardson et al. Citation2007).
Swimming pools constitute environments with high levels of disinfectant by-products in water and air due to continuous disinfection and constant organic load from bathers. Only a few studies have investigated the mutagenicity of swimming pool water (Richardson et al. Citation2010). In a recent comprehensive study, water and air samples were collected from a public chlorinated swimming pool and the concentrations of free chlorine, inorganic chloramines and trihalomethanes were determined. Monochloramine and dichloramine, but not trichloramine, could be detected in the pool water. However, a mean air level of 0.29 mg/m3 indicated that trichloramine had evaporated from the pool water. In addition, approximately one hundred other disinfection by-products were identified in the pool water. Two water samples extracted using a XAD resin process were subjected to a standard plate incorporation Ames Salmonella mutagenicity assay (without metabolic activation) in the base-substitution strain TA100 or in the strain RSJ100, which expresses the rat glutathione S-transferase theta 1 (GSTT1) gene, and its control strain TPT100. Extracts were tested up to 100 µl/plate over a dose range of 0.01–0.3 liter-equivalents (L-eq) based on doses used for mutagenicity tests for drinking water. One sample was mutagenic in TA100, whereas the other sample showed cytotoxicity based on a reduction of revertants/plate in TA100 at the highest doses. It was suggested that the 30% higher concentration of chloroform in the latter sample may have masked the mutagenic activity due to cytotoxicity. The limited data indicated that the dose range over which the pool waters were mutagenic was approximately 0.1–0.3 L-eq/plate. In comparison, the typical dose range for drinking water mutagenicity is 0.3–1.5 L-eq/plate (Richardson et al. Citation2010).
A large number of identified disinfection by-products demonstrate the chemical complexity of the chlorinated/chloraminated water and the difficulty to identify individual mutagenic substances in the water.
10.5. Effects of long-term exposure and carcinogenicity
No inhalation studies were located.
10.5.1. Monochloramine
Male Sprague Dawley rats (4/group) were exposed via drinking water containing 0, 1, 10 or 100 mg/l (∼0.05, 0.5, 5.2 mg/kg bw/day) of monochloramine daily for 12 months. Blood samples were collected for analysis of blood GSH and osmotic fragility every 2nd month and for hematological parameters every month. [3H]Thymidine incorporation (a measure of cell proliferation) into the nuclei of the liver, kidney, testes, small-intestinal mucosa and spleen was determined after 3 months only. The GSH content in blood was significantly decreased at some time-points during exposure in all dose groups, which according to the authors could be related to the oxidative stress of monochloramine and the protective role of GSH against damage caused by oxidants. Increased osmotic fragility was observed in the 10- and 100-mg/l groups, and significantly at a few time-points during exposure. Significant changes in a few hematological parameters were observed at 10- and 100 mg/l at 3 and 10 months, but were not observed at any other time-points. Monochloramine administered for 3 months increased [3H]thymidine incorporation in the kidney, spleen, liver and testes in all dose groups, but without a clear dose–response pattern. The authors concluded that this indicates that exposure to monochloramine increases the DNA synthesis in these organs. The body weight decrease in the 100-mg/l group at 3 months persisted throughout the dosing period (Abdel-Rahman et al. Citation1984). However, the lack of dose-response suggests that this is not a treatment related finding.
In a subchronic study, monochloramine was administered in drinking water at concentrations of 0, 25, 50, 100 and 200 mg/l for 90 days to groups of Sprague Dawley rats (10/sex). Reductions in final body weight (at 200 mg/l both sexes) and body weight gain (≥50 mg/l in males; 200 mg/l in females) along with a dose-related decrease in water consumption (in both sexes at all concentrations) were noted. At 200 mg/ml, reduction in organ weights (absolute, relative or both) was observed in both males and females, liver and spleen weights decreased in both sexes. In addition, decreased liver and lung weights were noted in males at 100 and 50 mg/ml, respectively. Subsequent histopathological examination did not reveal any exposure-related changes. A few alterations in parameters of hematology and clinical chemistry were noted but were considered not biologically significant, not dosage-related, or within the normal range for rats of this age and strain. The 100-mg/ml concentration (5.8–7.7 mg/kg bw/day) was considered to be a no observed adverse effect level (NOAEL) (Daniel et al. Citation1990).
In a similar study by the same team, B6C3F1 mice (10/sex/group) were given 0, 12.5, 25, 50, 100 and 200 mg/l of monochloramine in drinking water for 90 d. Final body weight was significantly decreased at doses ≥100 mg/l in both sexes and body weight gain at doses ≥50 mg/l in males and ≥100 mg/l in females. In parallel, dose-related decreases in water and food consumption were observed in both sexes. A variety of changes in parameters of hematology and clinical chemistry were noted with no consistent exposure-related pattern. Numerous significant reductions in absolute and/or relative organ weights were evident at doses ≥100 mg/l but no exposure-related histopathology was observed. Decreased liver, heart and lung weights (males) and liver, heart and spleen weights (females) were seen at 100 mg/l, along with decreased body weight gain, reduced water and food consumption. The 50 mg/l concentration (8.6–9.2 mg/kg bw/day) was considered to be the NOAEL (Daniel et al. Citation1991).
Sprague Dawley rats (10 males) were exposed to 200 mg/l (21.6 mg/kg/day) of monochloramine in drinking water for 13 weeks to resolve whether such exposure could cause reduced body weight gain and other changes observed in earlier studies. Two control groups were included; one group was given water ad libitum and the other was given the same volume of water as that consumed by the exposed animals. The authors concluded that reduced body weight gain and the minor biochemical, hematological, immunological and histopathological changes in exposed rats were largely related to reduced water and food consumption and not a direct effect of monochloramine (Poon et al. Citation1997).
In a carcinogenicity study by the National Toxicology Program (NTP), drinking water containing 0, 50, 100 or 200 mg/l of monochloramine was provided to male and female F344/N rats or B6C3F1 mice (70 animals in each group) daily for up to 2 years. Doses were calculated to be 0, 5.0, 8.9 and 15.9 mg/kg bw/day for male mice and 0, 4.8, 9.0 and 17.2 mg/kg bw/day for female mice. The corresponding figures for male rats were 0, 2.1, 4.8 and 8.7 mg/kg bw/day and 0, 2.8, 5.3 and 9.5 mg/kg bw/day for female rats. The animals were evaluated at 14, 66 or 155 weeks. Mean body weights of high-dosed rats and dosed mice were lower than those of their respective controls. Decreases in absolute and/or relative organ weights were observed in the high-dose groups of rats and mice at some time-points. There was a dose-related decrease in water consumption in both species. Mononuclear cell leukemia occurred with marginally increased incidence in the mid- and high-dose female rats receiving chloraminated water (control 8/50, low dose 11/50, mid-dose 15/50 and high dose 16/50). The marginal increase in leukemia incidence in female rats was considered equivocal evidence of carcinogenic activity. There were no neoplasms or non-neoplastic lesions in male rats or in male and female mice that were associated with the consumption of chloraminated water (NTP Citation1992). There was a relatively low survival in dosed (12-33%) and control (28%) male rats in comparison with female rats where 55−70% of the dosed animals and 62% of the controls survived throughout the study. This might have had an impact on the results for the male rats. In addition, there was a high and variable incidence of leukemias in the female control rats, and the incidence in the treated group was within the range of the controls, and there was no dose-response relationship as well. Moreover, using a statistical significance of <0.05 for comparison is less appropriate for common tumors such as mononuclear cell leukemia. We suggest that this is a negative finding.
In a study carried out by Herren-Freund and Pereira (Citation1986), 9 male rats (strain not given) were administered 14.75 mg/kg bw of monochloramine 24 h after 2/3 partial hepatectomy. Seven days after the initiation, promotion by 500 mg/l of phenobarbital in the drinking water was begun. After 10 weeks, the exposure to the promoter was ceased and 1 week later the rats were killed. Samples of the liver tissue were stained for the incidence of γ-glutamyltranspeptidase-positive foci (GGT foci) as an indicator of carcinogenicity. Diethylnitrosamine (DENA) was used as a positive initiator control. Exposure to monochloramine did not initiate GGT foci and the authors concluded that monochloramine is not capable of initiating carcinogenesis (Herren-Freund and Pereira Citation1986). The positive control (DENA) induced a high incidence of GGT foci, indicating that the test was functioning properly, which supported the author’s conclusions.
In a rat liver foci study, the carcinogenic activity of drinking water treated with monochloramine and concentrated up to 4000 times by the XAD resin procedure was evaluated. Around 10 rats per group were used for the test samples, vehicle control (2% Emulphor) and positive control (50 mg/kg bw of DENA). All rats were hepatectomized on day 0 and dosed orally 24 h later with the test solutions or the control solutions. A week later, the rats including controls received 500 mg/l of sodium phenobarbital in drinking water for 56 d. Liver samples were tested for histochemical detection and quantification of GGT foci. No statistically significant differences between monochloramine exposed rats and vehicle control animals were detected. The authors concluded that there was no effect on the rat liver assay following exposure to monochloramine (Miller et al. Citation1986). The positive control (DENA) induced a high incidence of GGT foci, indicating that the test was functioning properly, thus supporting the authors’ conclusions.
10.5.2. Dichloramine
Male and female Sprague Dawley rats were each randomly divided into 6 groups each containing 10 animals. The dichloramine solution was prepared in 0.1 M acetate buffer and 2 control groups were used (reagent-grade water and 0.1 M acetate buffer). The rats were exposed to dichloramine in drinking water for 13 weeks at doses of 0.025, 0.26, 2.5 or 24 mg/kg bw/day for females and 0.019, 0.19, 1.9 or 18 mg/kg bw/day for males. No significant changes were detected in xenobiotic metabolizing enzyme activities or in hematological and biochemical parameters. Dichloramine induced minimal to mild adaptive histopathological changes in thyroid and kidneys in both sexes (no statistical analysis for individual dose levels). In the thyroid, reduced follicle size, colloid density and vesiculation of nuclei were seen. Dichloramine was associated with minimal histological changes in the gastric cardia (epithelial hyperplasia) at concentrations ≥0.19 mg/kg bw/day in males and ≥2.5 mg/kg bw/day in females. Body weight gains were not significantly different from controls (Nakai et al. Citation2000). The minimal histologic changes in gastric cardia (epithelial hyperplasia), most certainly represents the fore stomach and is secondary to irritation, which is not relevant to human risk.
No carcinogenicity studies were located.
10.5.3. Trichloramine
In an experiment parallel to the one described above for dichloramine, Sprague Dawley rats were exposed to trichloramine in drinking water for 13 weeks at doses of 0.020, 0.23, 1.1 or 9.6 mg/kg bw/day for males and 0.028, 0.29, 1.3 or 13 mg/kg bw/day for females. The trichloramine solution was prepared in 0.1 M phosphate buffer and contained an average of 20 mg/l residual free chlorine, therefore the following control groups were used: reagent-grade water and 0.1 M phosphate buffer containing 19 mg/l chlorine. Increases in hepatic glutathione S-transferase and uridine diphosphate glucuronosyltransferase activities were detected in females receiving 13 mg/kg bw/day. No significant changes were detected in other xenobiotic metabolizing enzyme activities or hematological and biochemical parameters. Trichloramine induced minimal to mild adaptive histopathological changes in thyroid and kidneys in both sexes. The histopathological changes described in the thyroid were reduced follicle size, colloid density and vesiculation of nuclei. Relative kidney weights were increased in both sexes at the highest dose. Males in the high-dose group had minimal changes in kidneys consisting of glomerular adhesions and protein casts in the tubules of the inner cortex. Females in all dose groups displayed minimal tubular mineralization in the outer cortex of the kidneys but with no clear dose–response over the range examined. The authors concluded that trichloramine produced mild histological effects at doses >0.23 mg/kg bw/day in males and >0.29 mg/kg bw/day in females (Nakai et al. Citation2000). It is unclear how these effect levels were derived from the results presented in the paper. However, the finding of renal changes of glomerular adhesions and protein casts in the tubules most likely represents chronic progressive nephropathy, and is not relevant to human assessment. In addition, the finding of minimal tubular mineralization in the kidneys of the rats is a common finding in many strains, including Sprague Dawley rats, and is not relevant to human assessment.
No carcinogenicity studies were found.
10.6. Reproductive and developmental effects
10.6.1. Monochloramine
No inhalation data were found.
Reproductive effects were studied in female (24/group) and male (12/group) Long-Evans rats administered 0, 2.5, 5.0 or 10.0 mg/kg bw of monochloramine by oral gavage. Males were dosed 56 d prior to breeding and throughout the 10-d breeding period. Following breeding, the males were necropsied and evaluated for sperm parameters and reproductive tract histopathology. Females were dosed 14 d prior to breeding and throughout the 10-day breeding period, during gestation and lactation until the pups were weaned at day 21 after birth. Adult females and some pups were necropsied at weaning on postnatal day 21. Other pups were dosed post-weaning until 28 or 40 d of age. These pups were evaluated for the day of vaginal patency and thyroid hormone levels. No differences were observed between control rats and rats exposed up to 10 mg/kg/day of monochloramine when fertility, viability, litter size, day of eye opening or day of vaginal patency were evaluated. No alterations in sperm count, sperm direct progressive movement, percent motility or sperm morphology were observed among adult males. In addition, male and female reproductive organ weights were comparable to their respective control groups, and no significant histopathological changes were observed in the reproductive tract of exposed males and females (Carlton et al. Citation1986).
Meier et al. (Citation1985) administered B6C3F1 mice (10 males/group) 1.6, 4 or 8 mg/kg bw of monochloramine by oral gavage for 5 consecutive days and evaluated sperm-head abnormality at 1, 3 and 5 weeks after the last dose. Monochloramine was judged to be negative in the assay.
In a developmental study, mature virgin Sprague Dawley rats (6 females/group) were given 0, 1, 10 or 100 mg/l (∼0.09, 0.9, 9.3 mg/kg bw/day) of monochloramine in drinking water ad libitum daily for 2.5 months prior to and throughout gestation. On day 20 of gestation, the rats were killed and the fetuses (34 controls, 26–28 exposed) were examined. Monochloramine did not produce any significant changes regarding fetal weight, type of skeletal anomaly, skeletal or soft-tissue defects at any dose level. The authors concluded that monochloramine in drinking water at the concentration noted is relatively harmless when fed to pregnant rats (Abdel-Rahman et al. Citation1982).
To conclude, limited data indicate that monochloramine does not induce reproductive or developmental effects.
10.6.2. Dichloramine and trichloramine
No data were located
11. Observations in man
11.1. Irritation and sensitization
Several studies have investigated eye and respiratory tract irritation among workers occupationally exposed to inorganic chloramines in indoor swimming pool facilities and in cleaning and disinfection in the food processing industry as well as among competitive and recreational swimmers, as described below and summarized in (effects predominantly evaluated against measurements of trichloramine levels).
Table 7. Irritation in humans exposed to airborne trichloramine (exposure levels refer to stationary sampling if not stated otherwise).
Data on irritation from accidental exposure and on asthma-related symptoms and effects are presented in Sections 11.2.1 and 11.3.1, respectively. No studies on dermal sensitization were found.
11.1.1. Swimming Pool workers
Chu et al. (Citation2013) investigated the prevalence of work-related irritation in eyes and airways among pool workers in 10 indoor swimming pools in Taiwan of which 6 had spa pools located in the same buildings. The exposed group consisted of 61 lifeguards and swimming instructors (36 men, 25 women). The reference group consisted of 43 employees (15 men, 28 women) working at the same swimming facilities, but their exposure was considered negligible. Information regarding previous diagnosis of asthma, atopy or a family history of allergy among the participants was not presented. Exposure classification on an individual basis was not performed. Airborne levels of trichloramine were determined using stationary sampling, performed from April through October 2010. The overall average concentration of trichloramine was 0.035 (0.017–0.15) mg/m3 in swimming pools and 0.059 ± 0.042 (SD) mg/m3 in spa pools. Corresponding soluble chlorine concentrations were 0.072 (0.005–0.21) mg/m3 and 0.085 ± 0.056 mg/m3. Questionnaires were given to the pool workers during the same time as the air samplings were performed and comprised questions about ocular and respiratory symptoms. Symptoms were considered work-related if present during or after a work shift. The prevalence of various symptoms was compared on a group level; in general, exposed workers reported more symptoms from eyes and airways than non-exposed subjects did. Exposed workers had significantly more [odds ratio (OR), 95% CI] throat irritation (11.3, 1.4–88] and phlegm (4.2, 1.2–15) than non-exposed subjects, but there were no significant differences for ocular and nose irritation.
Dang et al. (Citation2010) investigated work-related symptoms via questionnaire among 143 exposed and unexposed pool workers at an indoor waterpark in the United States (US). The prevalence of hay fever or other allergies was 34% in exposed and 39% in unexposed, and 17% versus 12% reported asthma before working at the waterpark. Stationary measurements of airborne trichloramine were performed during two high-occupancy days and one low-occupancy day. The average concentration for the first high-occupancy day was 0.44 mg/m3. Only 20% of all samples collected during the second high-occupancy day were quantifiable, but the highest concentration (1.06 mg/m3) was found this day. Lifeguards (37 men, 32 women) reported significantly more work-related irritation [prevalence ratio (PR), 95% CI] in throat (12, 2.9–48), nose (3.5, 1.9–6.6) and eyes (9.0, 4.1–20) than unexposed employees working outside the pool area (24 men, 50 women). In addition, the lifeguards completed a daily symptom questionnaire during the high-occupancy days (n = 43) and low-occupancy day (n = 27). Work-related symptoms were significantly more common during the high-occupancy days than the low-occupancy day (PR, 95% CI), such as eye irritation (2.0, 1.2–3.2) and cough (2.2, 1.1–4.5) (adjusted for smoking habits, subjects with asthma not included).
The prevalence of ocular and respiratory symptoms was assessed via questionnaire in 128 pool workers in an Italian study. Information regarding previous asthma, atopy, or a family history of allergy was not presented. Lifeguards and swimming instructors (40 men, 41 women) comprised the exposed group and the other employees were considered non-exposed (21 men, 26 women). Measurements of airborne trichloramine using stationary sampling showed an average concentration of 0.65 mg/m3. Exposed pool workers reported ocular and upper respiratory symptoms (sometimes or often) more frequently than the unexposed. Five levels of exposure to airborne trichloramine were defined: ≤0.5, >0.5, >0.6, >0.7 and >0.8 mg/m3. A strong dose–response relationship between trichloramine air levels and self-reported irritation in eyes and upper airways was demonstrated. Pool workers exposed to airborne trichloramine levels >0.5 mg/m3 had significantly higher risk (OR, 95% CI) for experiencing runny nose (2.9, 1.2–6.9), voice loss (3.6, 1.6–7.9), red eyes (3.2, 1.5–6.8) and itchy eyes (2.2, 1.04–4.8) than non-exposed subjects (Fantuzzi et al. Citation2013).
Work-related respiratory symptoms were investigated via interview of 146 pool workers (58 men, 88 women) employed at 46 swimming pool facilities in Sweden. Subjects diagnosed with asthma or atopy were not included in the study. In total, 17% of the pool workers reported work-related respiratory symptoms; there were no significant gender differences. The prevalence was relatively high among workers employed 1–3 year (31%) and lower among those employed 4–7 years (6%), indicating a possible healthy worker effect (in this case a selection bias meaning that employees who experience health problems at work tend to quit, and that those who continue to work have better health). However, the prevalence of symptoms was 22% in those employed >7 years. Most pool workers with airway symptoms (64%) came from 6/46 swimming pool facilities. Five indoor swimming pools with high prevalence of reported airway irritation and four swimming pools without reported airway irritation among the employees were selected for measurements of airborne trichloramine via stationary sampling. The average concentration of airborne trichloramine was 0.20 mg/m3. The 39 pool workers were evaluated by questionnaire. No association between self-reported work-related airway symptoms and levels of airborne trichloramine was found. Protein profiling of nasal lavage fluid in 9 pool workers and 4 control subjects showed altered distribution of three innate immunity proteins (α-1-antitrypsin, lactoferrin and S100-A8) in pool workers, and more pronounced in subjects with airway irritation (Fornander et al. Citation2013).
Héry et al. (Citation1995) measured indoor airborne levels of trichloramine in 7 standard swimming pools and 5 recreational swimming pools in France using stationary sampling. The aim was to assess the exposure of swimming instructors who had complained of irritative symptoms during work. The sampling devices were placed at different spots that were chosen to be as representative as possible of the swimming instructors’ exposure. Trichloramine concentrations as ranges of AMs were 0.15–0.39 mg/m3 at the swimming pools and 0.23–1.25 mg/m3 at the recreational centers. Swimming instructors were requested to report at the onset of irritative symptoms during sampling. The onset of irritation in eyes and upper respiratory tract began at concentrations around 0.5 mg/m3 and all questioned complained of irritation when the concentration reached 0.7 mg/m3. Information regarding number, age and gender of participating subjects, and how they were selected for the study was lacking.
A questionnaire was sent to 1066 pool workers from 38 swimming pool facilities in the Netherlands. The response rate was 59% and thus questionnaires from 624 exposed and unexposed pool workers (240 men, 384 women) remained for analysis. Work-related and general respiratory symptoms as well as symptoms indicative of allergy were asked for. Measurements of airborne trichloramine were performed using stationary sampling in 6 different swimming facilities; the overall mean was 0.56 mg/m3. Swimming instructors (n = 121) reported more work-related symptoms (OR, 95% CI), mainly from upper airways such as sinusitis (2.4, 1.2–4.9), chronic cold (3.4, 1.2–10.1), sore throat (2.4, 1.2–4.5), blocked nose (2.0, >1.0–3.8) and sneezing (2.0, 1.1–3.6) than 282 reception, catering and management employees. ORs (95% CIs) for runny nose and irritation in eyes were 1.2 (0.8–2) and 1.5 (0.8–2.6), respectively. The 142 employees who combined the jobs of pool attendant and swimming instructor also reported more work-related symptoms in the upper airways (OR, 95% CI) such as chronic cold (3.5, 1.2–10), sore throat (3.2, 1.7–5.9), blocked nose (3.7, 1.9–7.3), runny nose (2.2, 1.3–3.5), sneezing (2.3, 1.2–4.4) and irritation in the eyes (3.0, 1.7–5.3). The OR for sinusitis was 2.3 (1.0–5.6). No association between estimated long-term levels of airborne trichloramine (AM 0.66 mg/m3) and symptoms was found, but 1-week cumulative exposures (trichloramine levels of each pool multiplied by the quantity of working hours per week for each individual) were significantly associated with upper respiratory symptoms (Jacobs et al. Citation2007).
Löfstedt et al. (Citation2016) investigated the prevalence of ocular and respiratory symptoms among 52 pool workers (16 men, 36 women) from 8 indoor swimming pool facilities in Sweden. The reference group consisted of 50 office workers (15 men, 35 women). The prevalence of ocular and respiratory symptoms during the last week, as well as during the last 3 months was assessed via questionnaire. Asthma at any time or allergic symptoms during childhood was asked for. Phadiatop was positive in 24 (46%) exposed subjects and 12 (24%) referents. The concentration of airborne trichloramine was measured by personal sampling, and the AM was 0.071 mg/m3. Stationary sampling was performed in parallel to personal sampling and showed an AM of 0.18 mg/m3 (Westerlund et al. Citation2015). Exposed workers reported more often itchy eyes (36% versus 14%), at least one ocular symptom (62% versus 37%) and blocked nose (55% versus 32%) than referents during the last week. After adjusting for smoking, asthma and sensitization to inhaled allergens, exposed workers reported more often (OR, 95% CI) at least one ocular symptom such as itchy, smarting, dry, running and red eyes, gravel sensation or swollen eyelids (2.4, 1.0–5.7) and one nasal symptom such as blocked, dripping or itchy nose, and sneezing (2.3, 1.0–5.6) during the last week compared with the referents. The OR (95% CI) for respiratory (tracheobronchial) symptoms was 1.6 (0.7–3.9). Exposed workers reported more often ocular, nasal and/or respiratory symptoms during the last 3 months than referents (data not shown in paper). No associations were shown between exposure levels and studied health effects.
Massin et al. (Citation1998) assessed irritant eye and upper airway symptoms at work by questionnaire in 334 lifeguards (256 men, 78 women) recruited from 46 public swimming pools and 17 adventure swimming pools in France. In addition, information regarding personal and family history of respiratory diseases and symptoms was collected. There was no reference group. Stationary measurements showed that the average airborne concentrations of trichloramine were 0.24 mg/m3 in the swimming pools and 0.67 mg/m3 in the adventure swimming pools. The lifeguards were divided in four subgroups due to measured trichloramine concentrations in air: <0.14, 0.14–0.22, 0.22–0.50 and >0.50 mg/m3. The prevalence rates of eye irritation in the groups were 50%, 56%, 63% and 86%, respectively. Corresponding numbers for nose irritation were 12%, 20%, 28% and 61%. For sore throat and dry cough, the rates were 16%, 15%, 27% and 29%, and 9%, 12%, 21% and 42%, respectively. These concentration–response relationships were all significant. In addition, a cumulative exposure index was calculated for each lifeguard by multiplying the average trichloramine level by the number of working years (mg/m3-years). The lifeguards were divided in four subgroups: <0.58, 0.58–1.6, 1.6–3.12 and >3.12 mg/m3-years. The prevalence rates of eye irritation in the groups were 51%, 67%, 64% and 75%, respectively. The corresponding numbers for nose irritation were 22%, 27%, 35% and 39%. For sore throat and dry cough, the rates were 15%, 24%, 23% and 25%, and 15%, 26%, 24% and 22%, respectively. Significant concentration–response relationships were found between cumulative exposure index and irritant eye and nose symptoms. No such relations were shown between cumulative exposure and sore throat and dry cough. ORs were not reported.
Parrat et al. (Citation2012) investigated the occurrence of ocular and respiratory symptoms including current asthma, and symptoms of allergy in 178 swimming pool workers (117 men, 61 women) in a Swiss study. Self-reported symptoms that had been present during and before the last 12 months, respectively, were collected by questionnaire. The reference group consisted of 71 office workers (38 men, 33 women). Stationary sampling was used to determine airborne trichloramine levels; the average concentration was 0.11 mg/m3. Irritative symptoms (sum of symptoms that appeared before, and during the last 12 months, hereafter referred to as “ever”) in eyes (53% versus 22%), throat (79% versus 7%), nose (38% versus 7%) and skin (61% versus 8%) due to the working conditions were significantly more common among pool workers than among referents. Pool workers exposed to airborne trichloramine levels >0.29 mg/m3 (AM 0.36 mg/m3, range 0.30–0.52 mg/m3) had significantly higher prevalences of irritation (OR, 95% CI) in eyes (5.6, 1.3–23.7) and nose (4.2, 1.4–13) during the last 12 months, and “ever” in nose (4.3, 1.5–13) than referents. No increased symptom prevalences were found in pool workers exposed to 0.1–0.29 (AM 0.15) mg/m3. Employees were divided in five groups according to a 1-week cumulative exposure index (combining the ratio of professional activity, the time spent working in potentially trichloramine-contaminated areas and the measured trichloramine concentration). The group with highest exposure index had increased prevalences of eye and nose irritation, and the second highest index group had increased eye irritation.
Westerlund et al. (Citation2019) investigated irritant symptoms among 23 pool workers (3 men, 20 women) from 10 habilitation and rehabilitation swimming pool facilities in Sweden. The reference group consisted of 50 office workers (15 men, 35 women). Concentrations of airborne trichloramine measured by personal and stationary sampling were 0.001–0.076 (AM 0.019) mg/m3 and 0.001–0.140 (AM 0.023) mg/m3, respectively. Data concerning ocular, nasal and respiratory symptoms during the last week, as well as during the last 3 and 12 months were collected via questionnaire. Asthma at any time or allergic symptoms during childhood was asked for. Five exposed workers (22%) and 12 referents (24%) had positive ImmunoCap tests. There was no significant difference in prevalences of irritation between exposed and referents at any time-point. The only significant finding was a higher prevalence of having at least 1 out of 6 ocular symptoms (itchy, smarting, dry, running and red eyes, and swollen eyelids) during the last week among those exposed to trichloramine above the median of 0.020 mg/m3 than in those exposed below the median (relative risk 1.2, 95% CI 1.1–1.4) (adjustment for age and smoking). The finding of eye irritation from exposure to trichloramine levels as low as 0.020–0.076 mg/m3, seems unlikely, in view of the lack of significant differences between exposed and referents and the results from other studies (described above) suggesting effects only at higher levels.
Demange and coworkers investigated 39 French lifeguards (29 men, 10 women) and reported a high prevalence of work-related symptoms, such as irritation in eyes (72% in men, 70% in women), nose (52%, 50%) and throat (48%, 30%). Two men (7%) and 3 women (30%) had a personal history of allergy, and two subjects with current astma were included (Demange et al. Citation2009). There was no reference group for comparison and no measurements of airborne trichloramine were performed.
The occurrence of allergic rhinitis was evaluated in 27 pool workers (13 men, 14 women) in Turkey by clinical examination, a skin prick test and a nasal smear. The prevalence of positive skin prick test was 30% compared to 18% in the reference group consisting of 49 office workers (22 men, 27 women); the difference was not statistically significant. Pool workers had significantly more eosinophils in nasal smear than non-exposed workers, indicating allergic inflammation, likely due to exposure to chlorine derivatives in the indoor air, according to the authors (Erkul et al. Citation2014). No measurements of airborne trichloramine were performed.
A questionnaire was sent to 1741 individuals who indicated in the Swedish Census of Population and Housing 1990 that they worked at indoor swimming pools. Ocular and respiratory symptoms related to work were asked for, as well as number of years hired as a pool worker and time spent in the pool area. The response rate was 63% and 1102 respondents (513 men, 589 women) remained for analysis. Measurements of airborne trichloramine were not performed, but exposure was classified in 3 different categories based on the average time during a workday each participant spent in the pool area. Exposure category 0 consisted of those who did not spend any time in the pool area, category 1 occasionally spent some time and category 2 spent most of the workday in the pool area. Irritative symptoms were common among all pool workers, i.e. irritation in eyes (37%), nose (29%), throat (24%) and coughing (23%). A statistically significant association was found between the average number of hours per day spent in the pool environment and the percentage of workers reporting acute work-related irritant symptoms (p < 0.01 logistic regression) (Nordberg et al. Citation2012).
11.1.2. Competitive and recreational swimmers
Gomà et al. (Citation2017) evaluated health complaints in 320 recreational swimmers (63% men, mean age 36 years) and 53 competitive swimmers including 34 water polo swimmers (84% men, mean age 22 years) before and 8 weeks after introducing a new disinfection method (details in ) in a swimming pool in Spain. Self-reported symptoms related to swimming, such as irritation of the eyes, respiratory tract and skin, personal history of allergy and asthma were collected by questionnaire. Symptoms were graded from 1 (no symptoms) to 4 (severe). In addition, leukotriene B4 (LTB4) and cysteinyl leukotrienes (CysLTs) (surrogate markers of neutrophilic and eosinophilic inflammation, respectively) were measured in exhaled breath condensate collected from the competitive swimmers 30 min before and 30 min after swimming. Stationary measurements were performed at a sampling point determined as the “worst” point based on levels of total oxidants and subjective information from the pool workers. The sampling point was situated 5 cm over the water surface level and 20 cm from the lateral edge, just over the perimeter drain. The new disinfection method resulted in a 75% reduction of total oxidants and a 39% reduction of trichloramine. Thus, the average airborne concentration of trichloramine fell from 0.62 mg/m3 using conventional chlorination to 0.38 mg/m3 using the new disinfection method. The symptom prevalences were higher in competitive swimmers than in recreational swimmers, the most common symptoms in both groups being eye and nose irritation. Competitive swimmers experienced decreased irritation in eyes (48% versus 19%; significant), nose (42% versus 28%; non-significant) and skin (30% versus 24%; non-significant) after shifting from the old to the new disinfection method. Also recreational swimmers had significantly lower prevalences regarding irritation in eyes (17% versus 6.7%), nose (12% versus 2.2%) and skin (11% versus 4.5%), and cough (5.8% versus 0%) after shifting methods. Participants with asthma (8% in competitive swimmers, 6% recreational swimmers) did not differ from non-asthmatics in symptom prevalences. The baseline level of CysLTs in exhaled breath condensate was significantly reduced when the new method was used, whereas the LTB4 baseline level was not affected.
Lévesque et al. compared the prevalence of respiratory symptoms in 305 competitive swimmers (101 boys, 204 girls) with that of 499 indoor soccer players (297 boys, 202 girls) in the Québec City region of Canada via questionnaire, and evaluated the relationship between trichloramine concentrations and the athletes’ respiratory symptoms. Information regarding family history of allergy and asthma, allergies and physician-diagnosed asthma was collected. The competitive swimmers reported significantly more symptoms (OR, 95% CI) from upper airways (3.7, 2.4–5.8), irritation in nose (7.2, 4–13), throat (2.1, 1.3–3.5) and eyes (11.9, 6.7–21) (ORs adjusted for gender, age and number of training sessions per week).
No significant difference between the groups was observed for skin problems. Respiratory symptoms were also assessed during five training sessions in 72 competitive swimmers, 8–22 years of age and 73 soccer players, 11–17 years of age. The concentration of trichloramine in air was measured in 7 swimming pool facilities and the AM was 0.34 mg/m3 (median 0.37 mg/m3) with a range of 0.26–0.41 mg/m3. The swimmers experienced more symptoms (OR, 95% CI) from upper airways (3.1, 1.8–5.4) and irritation in eyes (5.7, 1.1–28) during the five training sessions than the soccer players. Swimmers with high exposure to airborne levels of trichloramine (>0.37 mg/m3) had significantly more symptoms (OR, 95% CI) from upper airways (2.2, 1.0–4.8) and irritation in eyes (4.9, 1.9–12) compared to swimmers with an exposure <0.37 mg/m3 (Lévesque et al. Citation2006).
Seys et al. (Citation2015) initiated a study after complaints of respiratory symptoms among members of a swimming club when attending a municipal indoor swimming pool in Belgium. Competitive swimmers (n = 39) and coaches (n = 10) were invited to a medical evaluation. Of them, 22 swimmers aged 9–17 years (10 boys, 12 girls) and 6 coaches aged 18–60 years (3 men, 3 women) were evaluated by interview. Eleven swimmers were atopic based on a positive ImmunoCap test, and two of the 22 swimmers had a prior diagnosis of asthma and inhaled corticosteroids daily. Repeated measurements of airborne trichloramine were performed by stationary sampling and the concentration ranged from 0.20 to 1.30 mg/m3. Most commonly reported symptoms were cough (57%), dyspnea (46%), red tearing eyes (37%) and blocked or runny nose (21%). A glue containing polyamines used to repair a pipework was suspected to contribute to an excessive production of trichloramine. After removal of the glue, the concentration of airborne trichloramine decreased to a concentration below 0.5 mg/m3. At follow up one year later, including 13/28 subjects from the first evaluation, most subjects reported improvement but some symptoms remained. Some swimmers reported a recent recurrence of symptoms; the intake of outside air to the swimming pool had presently been reduced because of low outside temperature, resulting in rising air levels of trichloramine (0.66 mg/m3).
11.1.3. Food industry workers
King et al. (Citation2006) investigated eye and respiratory symptoms among workers in a US poultry processing facility and administered a screening questionnaire to 109 employees. Answers from 68 employees at the evisceration department (39 men, 29 women) and 41 workers in the dark meat department (18 men, 23 women) were available for analysis. The evisceration workers were chosen because of the extensive use of super-chlorinated water in their department, in contrast to the dark meat workers (reference group). Workers in both departments were similar regarding history of atopy (21% versus. 23%), defined as history of any of hay fever or other allergies, asthma or eczema. Work-related nose irritation, such as sneezing (OR 4.9, 95% CI 1.8–14) (but not itchy, running or stuffy nose), burning or stinging (OR 3.3, 95% CI 1.3–9.2) or watery eyes (OR 7.0, 95% CI 2.4–24) were significantly more common in evisceration workers than in dark meat workers; ORs adjusted for smoking status. The prevalence of work-related sore throat was also higher in evisceration workers, but did not reach statistical significance. A second survey was performed a year later comprising 18 evisceration workers and 16 dark meat workers. Measurements were performed by personal sampling. Levels of trichloramine were significantly higher in the evisceration area (GM 0.0051 mg/m3) than in the dark meat area (GM 0.0012 mg/m3). Soluble chlorine was also present in the indoor air of the premises; the concentration was significantly higher in the evisceration area (GM 0.0635 mg/m3) than in the dark meat area (GM 0.0094 mg/m3). Thus, soluble chlorine predominated in the indoor air. Exposure levels of trichloramine were significantly higher for employees reporting burning and stinging eyes (12/34, 35%) compared to those who did not report such symptoms. Further, exposure levels of soluble chlorine were higher for subjects reporting burning or stinging eyes (10/32, 31%), itchy or stuffy nose (9/32, 28%), frequent sneezing (5/32, 16%) and cough (8/32, 25%), compared to those who did not report such symptoms. Aerosolization of the chlorinated water resulting in high air levels of soluble chlorine makes it difficult to separate effects from exposure to trichloramine from those of soluble chlorine.
Massin et al. (Citation2007) recruited 175 cleaning and disinfecting workers from the food industry (149 men, 26 women) and 70 non-exposed workers (52 men, 18 women). Irritant symptoms as well as detailed history concerning respiratory diseases including asthma were evaluated by questionnaire. No information was presented regarding atopy in exposed workers. The personal inhalation exposure was given as the sum of trichloramine and soluble chlorine (range of AMs 0.33–0.49 mg/m3). In addition, glutaraldehyde and formaldehyde were used as disinfection agents, but air levels were not reported. A total exposure index including all irritants was calculated for each worker. Three subgroups were constituted; a non-exposed group and two groups with lower and higher exposure index. The prevalence of irritant symptoms was higher among subjects with highest exposure index than in non-exposed; irritation in eyes (48% versus 5.7%), nose (39% versus 1.4%), sore throat (27% versus 2.9%) and dry cough (27% versus 1.4%). Significant concentration-response relationships between irritant eye, nose and throat symptoms and exposure to irritants were found. In addition, the workers were divided in three subgroups taking exposure duration into account; non-exposed group, exposure duration ≤6 years and exposure duration >6 years. The prevalence rates for the groups with exposure duration >6 years and <6 years were 51% and 30% (eye irritation), 41% and 20% (nose irritation), 34% and 15% (sore throat) and 27% and 16% (dry cough), respectively. Significant concentration-response relationships between all symptoms and number of working years were found.
11.1.4. Conclusion
In summary, inorganic chloramines are strong mucous membrane irritants, and several cross-sectional studies have shown higher prevalences of self-reported ocular and/or upper airway irritation among workers occupationally exposed to inorganic chloramines and competitive swimmers compared to an unexposed reference group (King et al. Citation2006; Lévesque et al. Citation2006; Jacobs et al. Citation2007; Massin et al. Citation2007; Dang et al. Citation2010; Parrat et al. Citation2012; Chu et al. Citation2013; Fantuzzi et al. Citation2013; Löfstedt et al. Citation2016). Food industry workers (King et al. Citation2006; Massin et al. Citation2007) and competitive swimmers (Lévesque et al. Citation2006) are to a higher degree co-exposed to soluble chlorine from aerosols or the chlorinated water itself than swimming pool workers. Studies on these categories are, therefore, of limited value in the risk assessment of irritant effects of airborne trichloramine.
Several studies have demonstrated significantly more ocular and upper airway irritation in pool workers than in referents (Jacobs et al. Citation2007; Dang et al. Citation2010, Chu et al. Citation2013; Fantuzzi et al. Citation2013; Löfstedt et al. Citation2016) and exposure-response relationships have been shown in a few studies (Massin et al. Citation1998; Parrat et al. Citation2012; Fantuzzi et al. Citation2013). Stationary sampling was used in most of these studies. The temporal link between exposure and symptoms was sometimes weak.
Despite the amount of human irritation data on trichloramine, it is difficult to draw a firm conclusion regarding a no observed adverse effect concentration (NOAEC). In the study by Parrat et al., irritation symptoms were more frequent in pool workers exposed to 0.30–0.52 mg/m3 (AM 0.36 mg/m3) than in referents (Parrat et al. Citation2012). The study suggests a lowest observed adverse effect concentration (LOAEC) for irritation around 0.4 mg/m3. This value is supported by several other studies on pool workers which show irritation at approximately 0.5 mg/m3 (Héry et al. Citation1995; Massin et al. Citation1998; Fantuzzi et al. Citation2013).
Duration of exposure was taken into account in some studies, and an association between the prevalence of irritation in upper airways or eyes and cumulative trichloramine exposure was observed (Massin et al. Citation1998; Jacobs et al. Citation2007; Parrat et al. Citation2012). The cumulative exposures were calculated in different ways and are difficult to interpret in terms of exposure concentration. These data are, therefore, not suited for identifying NOAECs and LOAECs.
11.2. Effects of single and short-term exposure
11.2.1. Accidental exposure
Mixing solutions of ammonia and sodium hypochlorite results in acrid chloramine fumes (Gosselin et al. Citation1984). Gapany-Gapanavicius et al. (Citation1982) reported the case of a healthy 27-year-old woman who mixed ammonia and sodium hypochlorite in a small and poorly ventilated bathroom. The vapors from the mixture caused burning sensations in the eyes and throat, dyspnea, coughing, nausea and vomiting. Pneumonitis developed, but pulmonary function had returned to normal after 9 d.
A healthy 53-year-old woman was cleaning a walk-in-freezer using a mixture of ammonia and sodium hypochlorite which caused formation and release of inorganic chloramines into the air. The door was closed and there was no air exchange in the freezer. After approximately 30 min she noted shortness of breath. Over the next 3 h, she had increased tightness of the throat and lost her voice. The symptoms worsened. Emergency tracheostomy was performed. Radiologic evidence of pneumonitis developed over the next 4 h and she received supportive care. The tracheal tube was removed within 5 d and she was fully recovered within 7 d (Tanen et al. Citation1999).
As the vapors in these 2 cases probably contained also ammonia, which may produce similar health effects as inorganic chloramines, the responsible agent cannot be identified.
Bowen et al. (Citation2007) investigated outbreaks of acute ocular and respiratory symptoms associated with indoor swimming pool exposure among patrons of two hotels. In one of the hotels, the ventilation system malfunctioned during the outbreak. Out of 77 registered guests who stayed at the first hotel within 2 d of onset of outbreak, 47 (67%) were interviewed by telephone with a standardized questionnaire containing questions about exposure to the swimming pool area, general and respiratory and ocular symptoms. Among the 31 guests who visited the indoor pool area, 22 (71%) developed acute ocular symptoms such as burning and watery eyes, and 15 (48%) reported acute respiratory symptoms such as cough and sore throat. At the second hotel, 30 out of 77 registered guests and 59 companions completed the questionnaire. Among those 69 exposed to the indoor pool area, 41 (59%) developed acute ocular symptoms, and 28 (41%) acute respiratory symptoms. In total, all cases with ocular symptoms and 42/43 with respiratory symptoms had visited the pool area. Symptoms occurred within minutes of exposure to the indoor pool area and lasted up to 14 d, 4 persons sought medical care. Children were predominantly affected, but there was no association with gender. Environmental health investigations were performed. Appropriate water and air samples were not available for laboratory analysis. According to the authors, chloramines likely contributed to the illness; symptoms were consistent with trichloramine exposure and were sometimes severe.
Sanderson et al. (Citation1995) investigated outbreaks of work-related acute eye and upper respiratory irritation and other symptoms such as nausea and headache in 6 poultry processing plants. A questionnaire was administered to all 170 workers at one of these plants. Over 80% of the inspectors, evisceration/reprocessing and clean-up crew reported eye and nasal irritation, but only about one-third of the packers. Potential causes of the outbreaks were explored; air samples were collected at all 6 plants for chlorine gas and at 4 plants for ammonia, but concentrations of these gases were low. No measurements of airborne trichloramine were performed, but the outbreaks all had temporal association with problems or changes in the plant’s water chlorination and super-chlorination processes. The complaints were eliminated after technical changes, such as construction of a new water supply system and ventilation changes, suggesting a causal relationship between the reported complaints and exposure to trichloramine.
11.2.2. Effects on lung function and pneumoproteins
Studies investigating effects on lung function and pneumoproteins among workers and swimmers exposed to inorganic chloramines (effects predominantly evaluated against measurements of trichloramine levels) are described below and summarized in .
Table 8. Effects on pneumoproteins and lung function in humans after short-term exposure to airborne trichloramine (exposure levels refer to stationary sampling if not stated otherwise).
11.2.2.1 Swimming pool workers and swimmers
Löfstedt et al. (Citation2016) measured lung function and FENO before and after work in 52 pool workers and 50 office workers (for details, see Section 11.1.1). The average airborne trichloramine concentrations measured by stationary and personal sampling were 0.18 and 0.071 mg/m3, respectively. No changes in lung function were found over the work shift and there was no difference between the groups. Regarding FENO, there was a significant difference between the groups in that FENO decreased (as expected) over the work shift among referents but increased in pool workers, suggesting an inflammatory effect on the airways. The increase was more pronounced in nonsmoking pool workers.
A similar study by the same research group included 23 pool workers and 50 office workers (for details, see Section 11.1.1). The average airborne trichloramine concentrations measured by stationary and personal sampling were 0.023 mg/m3 and 0.019 mg/m3, respectively. No changes in lung function were found over the work shift and there was no difference between the groups. Mean FENO values differed between groups before shift (18 ppb versus 16.1 ppb in referents) and decreased over work shift in both groups (17.9 ppb versus 14.3 ppb), but significantly more in referents than in pool workers. Personal trichloramine concentrations were not associated with lung function or FENO (Westerlund et al. Citation2019).
Nordberg et al. (Citation2012) investigated lung function [forced expiratory volume in the first second (FEV1) and FEV% (FEV1/forced vital capacity × 100) as well as biomarkers of pulmonary epithelial integrity (CC16 and SP-D) in volunteers before and after exposure to indoor pool air. Two groups of volunteers were studied; 37 previously non-exposed healthy persons (20 men and 17 women, mean age 24.5 years) and 14 pool workers (5 men and 9 women, mean age 39.9 years) who performed bicycle exercise for 2 h in an indoor pool environment. Trichloramine in air was measured by personal sampling during pool exposures. All participants were also exposed to filtered air in a separate exposure chamber, which was used as control condition. In previously non-exposed volunteers, marginal but statistically significant decreases in both FEV1 and FEV% (p = 0.01 and 0.05, respectively) were found after exposure to pool air (0.23 mg/m3 of trichloramine). The individual differences in changes between exposure conditions in FEV1 and FEV% were significant with P-values of 0.01 and 0.004, respectively. In pool workers, a statistically significant decrease (marginal effect) was found, but only in FEV% (p = 0.003) after exposure to pool air (0.15 mg/m3 of trichloramine). The individual differences in changes between exposure conditions in FEV1 and FEV% were, however, non-significant. There were no statistically significant exposure-related changes in serum concentrations of CC16 and SP-D after exposure to pool air for 2 h. According to the authors, the lack of exposure-related changes in biomarker levels could be explained by the relatively short exposure duration and the low exposure level of trichloramine.
Bernard et al. (Citation2003) investigated short-term effects of trichloramine on the pulmonary epithelium of pool attendees. Three lung-specific proteins, the alveolar SP-A and SP-B and the bronchiolar CC16, were measured in serum of 16 children (mean age 9.6 years) and 13 adults (mean age 36.9 years), before and after attending an indoor swimming pool for 2 h. Children could have free activities while the adults were asked to stay at the poolside for 1 h and then could swim freely. Stationary sampling of trichloramine in air was performed and the mean concentration was 0.49 mg/m3. There was a significant increase in serum levels of SP-A and SP-B, and the SP-B/CC16 ratio in both children and adults; in adults already after 1 h without swimming. CC16 levels decreased significantly after 1 h and reversed toward normal 1 h later in adults, but did not show any distinct pattern in children.
Carbonnelle et al. (Citation2002) investigated the effect of trichloramine on the pulmonary epithelium of pool attendees. Three lung-specific proteins (SP-A, SP-B and CC16) were measured in serum of 29 recreational swimmers, among them 16 children aged 5–14 years (10 girls, 6 boys) and 13 adults aged 26–49 years (7 women, 6 men) before and after attending a chlorinated pool with a mean airborne trichloramine concentration of 0.49 mg/m3. These pneumoproteins were also measured in 14 trained swimmers aged 18–23 years (6 women, 8 men) before, immediately after, and 11 h after performing an intensive 45-min standardized swimming session in a chlorinated pool with a mean airborne trichloramine concentration of 0.36 mg/m3, and in a non-chlorinated pool sanitized by the copper/silver method. Pulmonary function was evaluated by spirometry at the same time as the blood sampling was done, both in recreational and trained swimmers. Stationary sampling of trichloramine in air was performed at the poolside 20 cm above the pool’s water surface to assess exposure during swimming. Serum levels of SP-A and SP-B were significantly increased in a time-dependent manner in recreational and trained swimmers attending the chlorinated pool, but were unaffected by strenuous exercise in the non-chlorinated pool. No significant decrements in lung function were found. Serum CC16 levels increased immediately after strenuous exercise in trained swimmers, both in the non-chlorinated and chlorinated pools, but were not increased in recreational swimmers. According to the authors, the latter finding is probably due to mechanical stress on the epithelial barrier caused by over-inflation and/or hyperventilation during intense exercise.
In a similar study, Carbonnelle et al. (Citation2008) investigated possible effects on the pulmonary epithelium and lung function in adults exposed to lower airborne concentrations of trichloramine. Serum levels of SP-A, SP-B, CC16 and Krebs von den Lungen-6 protein (KL-6) were measured in 11 healthy volunteers (7 women, 4 men) before and after performing an intensive 45-min standardized swimming session in a non-chlorinated pool. After 1 week, the subjects performed a similar swimming session in a chlorinated pool. All tests were repeated 3 h after exercise had ceased in both conditions. Measurements of airborne trichloramine were performed by stationary sampling on the poolside 20 cm above the pool’s water surface and ranged from 0.16 to 0.28 mg/m3. Lung function was evaluated by spirometry and possible airway inflammation was assessed using measurements of FENO. Serum levels of the pneumoproteins were unaffected after exercise in the chlorinated pool, and no decrements in lung function were found. FENO increased by 34% after exercise in the non-chlorinated pool but remained unchanged in the chlorinated pool. The authors concluded that exercise performed in a pool having an indoor air trichloramine concentration <0.3 mg/m3, did not induce any short-term changes in lung function or epithelial permeability.
Font-Ribera et al. (Citation2010) measured lung function, biomarkers of airway inflammation (FENO, 8 cytokines and vascular endothelial growth factor) and oxidative stress (8-isoprostane) in exhaled breath condensate in 48 healthy adult nonsmokers, before and after swimming for 40 min in a chlorinated indoor swimming pool. In addition, serum levels of the pneumoproteins SP-D and CC16, CC16 genotype, energy expenditure during swimming, and atopy were determined. Air measurements of trichloramine were done for a comparison with swimming pools in other countries but were not used for personal exposure estimates; the mean air concentration was 0.29 mg/m3. Most participants were women (65%), the mean age was 30 years, and 30% had a positive Phadiatop test (allergy screening test). Trihalomethanes in exhaled breath was measured as a marker of individual exposure to disinfection by-products. There was a slight increase in serum CC16 levels (3.3%) after swimming. According to the authors, both exercise and different markers of disinfection by-products exposure, such as change in trihalomethanes concentration in exhaled breath after swimming, explained this association. No significant changes in lung function tests or markers of airway inflammation or oxidative stress were found.
Font-Ribera et al. (Citation2019) investigated short-term changes in biomarkers of genotoxicity and respiratory damage in116 healthy adult nonsmokers (60 women, 56 men; mean age 24 years) before and after swimming for 40 min in a chlorinated indoor swimming pool. Serum CC16 was used as biomarker for lung epithelium permeability, and was measured before and 1 h after swimming. In addition, BMI, shortness of breath, exertion and energy expenditure during swimming were determined. Measurements of airborne trichloramine were performed once a day by stationary sampling within 1 m from the water and at a height of 60 cm above the floor level. The median airborne trichloramine concentration was 0.473 mg/m3, ranging from 0.249 mg/m3 to 0.858 mg/m3. There were no significant changes in serum CC16 levels after swimming. Serum levels of CC16 was negatively correlated to BMI and positively correlated to distance swam, but there was no association between the level of trichloramine in air and CC16. As the authors conclude, the results from this study suggest no effects on lung permeability at the observed exposure levels.
Llana-Belloch et al. (Citation2016) evaluated the effects on markers of lung epithelium permeability and oxidative stress in 20 healthy volunteers (all men, mean age 22 years) before and after swimming for 40 min in three chlorinated indoor pools with different characteristics. Blood samples were collected and analyzed for pneumoproteins SP-A, SP-B, markers of oxidative stress (plasma malondialdehyde and protein carbonyls, GSH and oxidized GSH) and lactate before and after exercise. Total air chlorine concentrations were measured in all swimming pools and were 0.1–1.0 mg/m3. The authors suggested that trichloramine was the major part of total chlorine in the air samples but the concentration of trichloramine in air was not reported. No increase in blood lactate was found after the swimming session, confirming that the exercise was performed at a moderate level. Concentrations of chlorine in water and air were not associated with changes in markers of lung epithelium integrity and oxidative damage. The authors concluded that short-term moderate exercise and exposure to chlorinated disinfection by-products will not affect markers of lung epithelial damage and oxidative stress.
11.2.2.2. Food industry workers
King et al. (Citation2006) investigated respiratory symptoms in 34 poultry processing workers (for details, see Section 11.1.3). The workers were evaluated with spirometry before and after shift, and 3/34 subjects had significant cross-shift declines in FEV1 (>10%). The airborne trichloramine exposure (personal sampling) of these subjects were significantly higher (GM 0.022 mg/m3) compared to those without significant cross-shift declines in FEV1 (GM 0.0021 mg/m3). The GM concentration of soluble chlorine in air was also higher in those with significant cross-shift declines in FEV1, however, not significantly (0.071 mg/m3 versus 0.022 mg/m3). Concurrent exposure to trichloramine and soluble chlorine, the latter due to aerosolization of the chlorinated water, makes it difficult to identify the responsible agent.
11.2.2.3. Conclusion
Short-term changes in pneumoproteins have been shown at mean airborne trichloramine concentrations ≥0.29 mg/m3 (Carbonnelle et al. Citation2002; Bernard et al. Citation2003; Font-Ribera et al. Citation2010) but not at lower exposure levels (Carbonnelle et al. Citation2008; Nordberg et al. Citation2012). Marginal effects on lung function were demonstrated in one study with personal trichloramine exposure levels of 0.15 mg/m3 and 0.23 mg/m3 (Nordberg et al. Citation2012). In contrast, no effects on lung function were demonstrated in studies with exposure levels of 0.023 mg/m3 (Westerlund et al. Citation2019), 0.18 mg/m3 (personal exposure 0.071 mg/m3) (Löfstedt et al. Citation2016), 0.16–0.28 mg/m3 (Carbonnelle et al. Citation2008), 0.29 mg/m3 (Font-Ribera et al. Citation2010), and 0.36 and 0.49 mg/m3 (Carbonnelle et al. Citation2002). FENO has been measured in some studies as a possible marker of airway inflammation, with mostly negative results. However, Löfstedt et al. (Citation2016) found an increase in FENO over work shift in pool workers exposed to 0.18 mg/m3 of trichloramine.
11.2.3. Other effects
Wones et al. (Citation1993) investigated whether a 4-week consumption of 1.5 l/day of distilled water containing monochloramine under controlled conditions would alter parameters of lipid or thyroid metabolism in healthy men. The 48 subjects drank distilled water during a 4-week dietary stabilization period. Following this period they were randomly divided into three dose groups (16/group). One group drank 1.5 l of distilled water, the second group drank 1.5 l distilled water containing 2 mg/l of monochloramine (0.043 mg/kg bw/day assuming a body weight of 70 kg) and the third group drank 1.5 l distilled water with a concentration of 15 mg/l of monochloramine (0.32 mg/kg bw/day) each day during 4 consecutive weeks. The study diet was designed individually to be isocaloric. Blood was collected in the morning in a fasted state at the beginning of the study, during 4 consecutive days at the end of the stabilization period and at the end of the monochloramine exposure. Total cholesterol, triglycerides, high-density lipoprotein (HDL) cholesterol, low-density lipoprotein (LDL) cholesterol (calculated), apolipoproteins A1, A2 and B, thyroxine (T4), triiodothyronine (T3), T3 resin uptake and thyroid stimulating hormone (TSH) were analyzed. The consumption of water containing 2 mg/l of monochloramine did not affect the parameters studied, whereas 15 mg/l was associated with an increase in the plasma level of apolipoprotein B. No information was given whether the plasma levels of apolipoprotein B were outside the normal range of the laboratory that performed the analyses. The authors did not discuss a possible toxic effect as a consequence of their finding.
Monochloramine was given in drinking water to 10 healthy male volunteers every third day at totally 6 occasions at concentrations increasing from 0.01 mg/l at day 1 to 2.4 mg/l at day 16. The 10 control subjects received untreated water. At each occasion the subjects drank two 500-ml portions, the second one administered 4 h after the first. Assuming a body weight of 70 kg, the highest dose corresponds to 0.034 mg/kg bw/day. In a second experiment, healthy male volunteers (10/group) were administered 0 or 5 mg/l monochloramine in a volume of 500 ml of water daily for 12 weeks (corresponding to 0.036 mg/kg bw/day). An extensive battery of measures including serum chemistry, blood count, urine analysis and physical examination were conducted before, during and following the experiments. The authors concluded that no definitive finding of detrimental physiological impact was identified in any of the experiments (Lubbers et al. Citation1982).
11.3. Effects of long-term exposure
11.3.1. Asthma-related symptoms
Asthma is characterized by reversible obstruction in the airways, airway hyperresponsiveness and chronic airway inflammation. Common symptoms are shortness of breath, wheezing, chest tightness and coughing (GINA Citation2018).
Spirometry is a standard test for measuring pulmonary function, and to diagnose asthma. Bronchial challenge test is used to detect airway hyperreactivity. The subject inhales nebulized methacholine or histamine to provoke bronchoconstriction and the degree of narrowing is quantified by spirometry. Chronic airway inflammation is associated with higher levels of nitric oxide and measurements of FENO can help to identify different asthma phenotypes and improving management of asthma (Ricciardolo et al. Citation2015).
Several studies have investigated chronic respiratory symptoms, indicating asthma, among workers with occupational exposure to inorganic chloramines in indoor swimming pool facilities, in cleaning and disinfection in the food industry and among swimmers, as described below and summarized in . In most studies questionnaires were used for assessment of chronic respiratory symptoms, and in several studies objective clinical tests were also used. Effects were predominantly evaluated against measurements of trichloramine levels.
Table 9. Asthma-related symptoms/effects in humans exposed to airborne trichloramine (exposure levels refer to stationary sampling if not stated otherwise).
11.3.1.1. Swimming pool workers
Chu et al. (Citation2013) investigated the prevalence of asthma-related symptoms among 61 pool workers in 10 indoor swimming pools in Taiwan of which 6 had spa pools located in the same buildings. The reference group consisted of 43 management employees (for details, see Section 11.1.1). The overall average air concentration of trichloramine was 0.035 (0.017–0.15) mg/m3 in the swimming pools and 0.059 ± 0.042 (SD) mg/m3 in the spa pools. Corresponding soluble chlorine concentrations were 0.072 (0.005–0.21) mg/m3 and 0.085 ± 0.056 mg/m3. Symptoms were considered work-related if present during or after a work shift. Exposed workers had significantly more phlegm (OR 4.2, 95% CI 1.2–15) than non-exposed subjects, but there were no significant differences for asthma-related symptoms (OR, 95% CI) such as shortness of breath (0.5, 0.1–2.5), cough (1.3, 0.5–3.5), chest tightness (2.3, 0.7–7.6) and wheezing (0.3, 0.03–4).
Dang et al. (Citation2010) investigated work-related respiratory symptoms among 143 exposed and unexposed pool workers at an indoor waterpark and performed measurements of airborne trichloramine during two high-occupancy days and one low-occupancy day (for details, see Section 11.1.1). Lifeguards (n = 69; 37 men) reported significantly more work-related respiratory symptoms (PR, 95% CI) such as coughing (10.2, 4.3–24), wheezing (9.7, 2.4–40), shortness of breath (6.7, 2.5–18) and chest tightness (6.7, 2.1–21) (adjusted for smoking habits and asthma) compared to unexposed employees working outside the pool area (n = 74; 24 men). All 6 lifeguards with existing asthma in the exposed group reported worsening of asthma symptoms at work, but none of the 7 employees with asthma in the unexposed group.
Fantuzzi et al. (Citation2013) investigated the prevalence of asthma-related respiratory symptoms in 81 lifeguards and swimming instructors (for details, see Section 11.1.1). The average air concentration of trichloramine was 0.65 mg/m3. No significant association between reported asthma symptoms and airborne trichloramine levels was shown, but the number of subjects with asthma was low (10 subjects; 3 referents, 7 exposed subjects).
In the study by Fornander et al., possible airway inflammation was evaluated in 39 pool workers by measuring FENO (for details, see Section 11.1). The average level of trichloramine was 0.20 mg/m3. In most pool workers, FENO was within the normal range (mean value 15 ± 13 ppb). Prevalence of airway irritation was not associated with FENO or air trichloramine levels (Fornander et al. Citation2013).
Jacobs et al. (Citation2007) investigated the prevalence of asthma-related symptoms, physician-diagnosed asthma and use of asthma medication in 342 exposed pool workers (for details, see Section 11.1.1). Measurements of airborne trichloramine were performed in 6 different swimming facilities and the overall mean was 0.56 mg/m3. Pool workers reported significantly more asthma-related symptoms (OR, 95% CI) such as phlegm (1.8, 1.3–2.4) and chest tightness (1.4, >1.0–1.8) during the last 12 months, physician-diagnosed asthma (2.1, 1.5–3.0), asthma attacks (2.6, 1.5–4.6) and use of asthma medication (3.6, 2.4–5.3) compared to a randomly selected reference group from the general population (n = 2711).
Massin et al. (Citation1998) used spirometry and methacholine bronchial challenge test to assess pulmonary function and airway hyperresponsiveness in 334 lifeguards recruited from 46 public swimming pools and 17 adventure swimming pools in France (for details, see Section 11.1.1). The average concentrations of trichloramine were 0.24 mg/m3 in the public swimming pools and 0.67 mg/m3 in the adventure swimming pools. Eight of the participants were diagnosed with asthma at the age of 16 or older. In general, the prevalence of chronic respiratory symptoms was low, and no association was found with current or cumulative trichloramine exposure. Measured values of pulmonary function exceeded the predicted values for all variables in both men and women. The prevalence of positive methacholine bronchial challenge test was twice as common in women as in men. No association was found between trichloramine exposure and airway hyperresponsiveness.
Thickett et al. (Citation2002) reported 3 pool workers with suspected work-related asthma following exposure to trichloramine, 2 of which had previous asthma and allergy. The investigation included spirometry, peak expiratory flow (PEF) measurements and bronchial challenge tests to methacholine, as well as trichloramine measurements. Two pool workers had PEF measurements showing significant work-related changes, as well as a positive specific challenge test (immediate asthmatic reactions) to trichloramine at 0.5 mg/m3. The third pool worker had a positive workplace challenge test, i.e. spirometry (FEV1) was first performed at baseline, and then after three 10-min exposures at the poolside. Measurements of trichloramine in the indoor swimming pool showed air levels of 0.1–0.57 mg/m3. The authors concluded that trichloramine might be a cause of occupational asthma.
In the study by Demange et al., 39 lifeguards were given a health questionnaire, and spirometry, measurement of FENO and a methacholine bronchial challenge test were performed (for details, see Section 11.1.1). Subjects with childhood or current asthma, not needing a corticosteroid treatment, and not in crisis, were included. Overall, pulmonary function values exceeded the predicted ones for FEV1, both among men and women. The prevalence of positive methacholine bronchial challenge test among lifeguards was relatively high (38%), but there was no reference group for comparison. One of the two participants with childhood asthma and two participants with adult-onset asthma were classified as having a positive methacholine bronchial challenge test. The median FENO was higher among those with positive methacholine bronchial challenge test (18.9 ppb) compared to the rest (12.5 ppb), but the values were within the upper reference range (Demange et al. Citation2009). Measurements of airborne trichloramine were not performed.
Nordberg et al. (Citation2012) investigated the prevalence of self-reported asthma in a cohort of 1102 pool workers by questionnaire (for details, see Section 11.1.1). Exposure was classified in 3 different categories based on the average time during a workday each participant spent in the pool area. Exposure category 0 was given to those who did not spend any time in the pool area, category 1 did occasionally spend some time and category 2 spent most of the workday in the pool area. “Self-reported asthma” was defined as having a positive answer to the following question: “Do you suffer from asthma or have you suffered from asthma?”. In a nested case–control study within the cohort, 44 cases of self-reported asthma that occurred after beginning as pool worker were compared with 128 age- and sex-matched controls without asthma. Subjects who spent most of the workday in the pool area (category 2) reported asthma more (non-significantly) often, after correction for heredity (OR 2.3, 95% CI 0.8–6.7). There was a tendency to a reduced risk of developing asthma in relation to the number of years of work in swimming pool environments among individuals who had worked more than 1 year, however, not significant (p = 0.07), indicating a possible healthy worker effect. Measurements of airborne trichloramine were not performed.
Rosenman et al. (Citation2015) investigated the occurrence of swimming pool workers with confirmed work-related asthma using data from the state surveillance systems in California, Michigan and New Jersey. In total, 44 confirmed cases of work-related asthma were identified; 17 cases 1994–2011 in California, 15 cases 1991–2012 in Michigan and 12 cases 1990–2011 in New Jersey. A majority (52%) of the cases were new onset; 32% secondary to an acute exposure incident and 20% to repeated exposure. Maintenance workers (35%) and lifeguards (32%) were the most common occupations. The authors concluded that the pool environment either might be a trigger of preexisting asthma or associated with new onset of work-related asthma. Airborne concentrations of trichloramine were not reported.
11.3.1.2. Food industry workers
King et al. (Citation2006) investigated asthma symptoms related to work in 109 poultry processing workers, of which 68 (39 men, 29 women) worked in the evisceration department and 41 (18 men, 23 women) in the dark meat department (for details, see Section 11.1.3). No information regarding number of subjects with a diagnosis of asthma was given. Evisceration workers reported more work-related wheezing (OR 5.9, 95% CI 1.4–40) and coughing (OR 6.2, 95% CI 2.1–21) than dark meat workers, ORs adjusted for smoking status. The prevalence of work-related asthma symptoms was also higher among evisceration workers, but did not reach statistical significance. A second survey was performed a year later comprising 34 workers (18 evisceration workers and 16 dark meat workers). Personal airborne levels of trichloramine were significantly higher in the evisceration area (GM 0.0051 mg/m3) than in the dark meat area (GM 0.0012 mg/m3). However, workers (both groups) reporting current asthma symptoms (wheezing or two of the following symptoms: chest tightness, shortness of breath and cough) did not have significantly higher exposure levels of trichloramine than those without symptoms. Instead, workers reporting current asthma symptoms had higher exposure to soluble chlorine than those without such symptoms (GMs 0.048 mg/m3 versus 0.019 mg/m3), but the relationship was no longer significant after adjustment for smoking.
Massin et al. (Citation2007) administered a symptom questionnaire to 175 cleaning and disinfecting workers in the food industry (149 men, 26 women) and 70 non-exposed workers (52 men, 18 women) (for details, see Section 11.1.3). Asthma was reported by 12 exposed subjects and 5 subjects in the non-exposed group. Lung function and bronchial responsiveness were evaluated by spirometry and an abbreviated version of the methacholine bronchial challenge test. The sum of airborne soluble chlorine and trichloramine was measured by personal samplers for each step in the production process. Glutaraldehyde and formaldehyde was also used as disinfection agents, but air levels were not reported. A total exposure index for all irritants was calculated for each exposed worker. Three subgroups were constituted; a non-exposed group and two groups with lower and higher exposure. The workers were also divided in three subgroups with respect to cumulative exposure, taking number of working years into account. Overall, the prevalence of chronic respiratory symptoms was rather low and no relation to exposure levels or exposure duration was found. Baseline levels of pulmonary function were normal and not related to exposure. No associations between bronchial responsiveness and exposure levels or exposure duration were found.
11.3.1.3. Competitive swimmers
Exercise may increase ventilation up to 200 l/min in endurance athletes such as swimmers, and exercise alone increases bronchial responsiveness to methacholine in patients with asthma. Competitive swimmers, with up to 30 h per week of training, inhale large amounts of air that floats above the water surface. In addition, they may microaspirate water in the airways. A high prevalence of asthma and use of asthma medication has been reported among competitive athletes participating in the Summer Olympic Games and particularly among endurance athletes, such as cycling, rowing and swimming (Helenius and Haahtela Citation2000).
Goodman and Hays (Citation2008) found a higher prevalence of asthma among competitive swimmers compared to other competitive athletes (OR 2.6, 95% CI 1.9–3.5) in a meta-analysis including 6 studies. Mountjoy et al. (Citation2015) reported a significantly higher prevalence of airway hyperresponsiveness/asthma in competitive swimmers compared to other aquatic disciplines.
Other studies have shown higher prevalences of asthma symptoms in competitive swimmers than in the general population (Päivinen et al. Citation2010; Romberg et al. Citation2012). However, the direction of the association is not clear and both exposure to chemicals and preference to swimming among asthmatics may be responsible for the association.
Helenius et al. (Citation1998) investigated respiratory symptoms, bronchial responsiveness and signs of airway inflammation in 29 professional swimmers (12 men and 17 women, mean age 19 years) from the Finnish national team and 19 healthy control subjects (14 men and 5 women, mean age 24.4 years). Respiratory symptoms were evaluated via questionnaire, lung function was assessed using spirometry, and bronchial responsiveness by a histamine challenge test. Induced sputum samples were also collected and a skin prick test was performed. Of the swimmers, 8 (28%), including 4 of 6 with asthma previously diagnosed by a physician, had a history of exercise-induced bronchial symptoms. Spirometry values were within normal limits for all the swimmers. Fourteen (48%) of the swimmers and three (16%) of the controls showed increased bronchial responsiveness. Fifteen (52%) of the swimmers and seven (39%) of the control subjects had positive skin prick tests. Sputum from swimmers had significantly higher differential cell counts of eosinophils (mean 2.7% versus 0.2%) and neutrophils (54.7% versus 29.9%) than sputum from control subjects (p < 0.01). Eosinophilia (sputum differential eosinophil count >4%) was observed in 6 (21%) of the swimmers and in none of the controls (p < 0.05). The 8 swimmers with a history of exercise induced bronchial symptoms had significantly higher sputum eosinophil differential cell counts than symptom-free swimmers (p < 0.01). No association was found between the sputum eosinophil counts and increased bronchial responsiveness. Atopic and non-atopic swimmers had similar amounts of sputum eosinophils and neutrophils. The authors concluded that long-term and repeated exposure to chlorine compounds in swimming pools during training and competition may contribute to the increased occurrence of bronchial hyperresponsiveness and airway inflammation in swimmers. Exposure data were not reported.
In another study, Helenius et al. (Citation2002) followed 42 professional swimmers, most of them from the Finnish national team, for 5 years. All swimmers were evaluated with spirometry, histamine challenge test, skin prick test and questionnaire at baseline and at follow-up. Sputum samples were collected from 29 swimmers at both occasions. Sixteen (38%) swimmers were still active competitive swimmers at follow-up while 26 (62%) had stopped competing. Bronchial responsiveness was increased in 7 (44%) of the 16 active swimmers at baseline and in 8 (50%) of the active swimmers at follow-up, but only in 8/26 (31%) of the past swimmers at baseline and 3/26 (12%) at follow-up. The difference in change in bronchial hyperresponsiveness between groups was significant (p = 0.023). There was also a significant difference in the change in current asthma between the groups; it occurred in 31% of active swimmers at baseline and in 44% at follow-up, the corresponding numbers in past swimmers was 31% at baseline and 12% at follow-up (p = 0.004). Mild eosinophilic airway inflammation was aggravated over time in active swimmers as reflected in the sputum eosinophil counts. The authors concluded that the athletes’ asthma is partly reversible and may develop during and decline after an active sports career. Exposure data were not reported.
Lévesque et al. (Citation2006) compared the prevalence of respiratory symptoms in 305 competitive swimmers with that of 499 indoor soccer players in the Québec City region of Canada via questionnaire, and evaluated the relationship between trichloramine concentrations and the athletes’ respiratory symptoms (for details, see Section 11.1.2). There were no differences between the groups regarding wheezing or previous or current asthma diagnosis. The competitive swimmers reported significantly more symptoms (OR, 95% CI) from the lower airways (1.5, 1.0–2.2), such as cough (1.2, 1.1–2.7) (ORs adjusted for gender, age and number of training sessions per week). In a second part of the study, respiratory symptoms were assessed during five training sessions in 72 competitive swimmers and 73 soccer players. The mean concentration of trichloramine in air was 0.34 mg/m3. The swimmers experienced significantly more symptoms from lower airways (adjusted OR 3.5, 95% CI 2.0–6.0). The swimmers were divided in two groups based on the median exposure level (0.37 mg/m3). Swimmers with high exposure did not have more symptoms from lower airways than those with lower exposure.
Pedersen et al. (Citation2008) investigated airway responsiveness and airway inflammation among 33 adolescent competitive swimmers (15 girls, 18 boys; mean age 14 years) and in two control groups consisting of 35 unselected adolescents (19 girls, 16 boys; mean age 14 years) and 32 asthmatics (13 girls, 19 boys; mean age 14 years). The swimmers and controls were evaluated via questionnaire, spirometry, methacholine challenge test, FENO and induced sputum. No differences in FENO, cellular sputum composition or prevalence of airway hyperresponsiveness were found between the groups, and the swimmers did not differ in the prevalence of respiratory symptoms from the unselected adolescents. The authors concluded that adolescent competitive swimmers that had been involved in competitive swimming for only a few years had no significant signs of airway damage.
Seys et al. (Citation2015) evaluated members of a swimming club who had complained of respiratory symptoms associated with attending a municipal indoor swimming pool in Belgium (for details, see Section 11.1.2). Competitive swimmers and coaches were invited to a medical evaluation, and of them, 22 adolescent swimmers and 6 adult coaches were investigated by clinical interview and spirometry, measurement of FENO and a methacholine bronchial challenge test. Repeated measurements of airborne trichloramine by stationary sampling showed levels of 0.20–1.30 mg/m3. Common reported symptoms from lower airways were cough (57%) and dyspnea (46%). Mean FEV1% predicted was 109.1%. Mean FENO level was 19.7 ppb; the cutoff value of 25 ppb was exceeded in 3 subjects. Airway hyperreactivity was detected in 22/28 swimmers (85%). After removal of a glue containing polyamines, used to repair a pipework, the concentration of trichloramine in air decreased to below 0.5 mg/m3. At follow-up 1 year later including 13/28 subjects from the first evaluation, the hyperreactivity was less pronounced in 6/12 subjects, more pronounced in 5/12 subjects and was unchanged in 1 subject. However, the air levels of trichloramine had presently risen due to reduced intake of outside air because of low outside temperature.
11.3.1.4. Children/adolescents
In general, children’s exposure to indoor swimming pool air during leisure time and school time is limited compared to that of pool workers and competitive swimmers. Studies conducted in Belgium have demonstrated an increased risk of asthma symptoms among children with atopy associated with cumulative indoor swimming pool attendance (Bernard et al. Citation2003, Citation2006, Citation2009).
Bernard et al. (Citation2003) investigated prevalence of asthma in relation to pool attendance among 1881 children aged 7–14 years in Belgium by retrospectively analyzing data from an earlier asthma survey conducted 1996–1999. Data on health status, respiratory symptoms and environmental and lifestyle variables were obtained via questionnaire, completed by their parents. Asthma was defined as a positive exercise induced bronchoconstriction (EIB) test, i.e. a reduction in PEF of ≥ 10% at 5 or 10 min after exercise. In addition, children with medication for asthma were included (total asthma). School directors gave information on pool attendance. Cumulative pool attendance was estimated by multiplying the time spent in pool water by the weekly frequency of attendance, and the number of years attending the school pool. The prevalence of respiratory symptoms, i.e. wheezing, was not associated with positive EIB test or total asthma. Positive EIB test and total asthma prevalence were significantly associated with cumulative attendance. The associations remained significant after adjustment for the height of the pool hall, pets and passive smoking, and were the strongest among the youngest children (p < 0.001). Measurements of airborne trichloramine were not performed.
The authors also studied effects on the lung epithelium in relation to pool attendance. Lung-specific proteins (SP-A, SP-B and CC16) and immunoglobulin E (IgE) were measured in serum of 226 children (116 boys and 110 girls), mean age 10 years. Children with asthma were not included. The school directors provided information on pool attendance; measurements of airborne trichloramine were not performed. A significant dose–effect association between cumulative pool attendance and proteins SP-A and SP-B was demonstrated (Bernard et al. Citation2003).
Bernard et al. (Citation2006) also investigated respiratory symptoms among 341 children aged 10–13 years who all visited the same indoor swimming pool facility in Belgium. The concentrations of trichloramine in air were 0.25–0.54 mg/m3. The parents completed a questionnaire concerning their children’s health status, respiratory symptoms and current and previous swimming pool attendance. Total and allergen-specific IgE were measured in serum, and an EIB test was performed. The test was defined as positive if a reduction of 20% or more in FEV1 was seen after exercise. All children performed a FENO test and it was considered elevated if nitric oxide was >30 ppb. Asthma was defined as having a positive EIB test or a physician-diagnosed asthma (total asthma). Cumulative pool attendance was associated with elevated FENO (OR 1.3, 95% CI 1.1–1.4) and total asthma but only in children with IgE >100 kIU/l (OR 1.8, 95% CI 1.1–2.7). There was a dose–response effect and the strongest effect was found with pool attendance before the age of 6–7 years.
In a later study, Bernard et al. (Citation2009) examined 847 school children, aged 13–18 years who had attended outdoor or indoor chlorinated pools, 114 of them had mainly attended a copper-silver pool (referents). Data on pool attendance and the child’s health status were collected via parental questionnaire. The students were interviewed about respiratory symptoms, and total and allergen-specific IgE were measured in serum, and an EIB test was performed. The test was defined as positive if a reduction of ≥10% in FEV1 after exercise was found. Ever asthma was defined as asthma diagnosed at any time by a physician, and current asthma was defined as physician-diagnosed asthma presently treated with medication and/or a positive EIB test. The concentration of trichloramine in air was in the range 0.3–0.5 mg/m3 in the indoor swimming pools the children attended. In those with a cumulative pool attendance >1000 h, ORs (95% CIs) for ever and current asthma was 3.7 (1.4–9.9) and 8.1 (1.7–37), respectively, compared to referents. Atopic children (i.e. those with total IgE >30 kIU/l) had a significantly higher risk for asthma symptoms and for ever or current asthma related to total numbers of hours spent in chlorinated pools; among those with cumulative pool attendance >1000 h, the ORs (95% CI) for ever and current asthma were 7.8 (2.1–29) and 15 (1.8–120), respectively, compared to atopics with cumulative pool attendance <100 h.
The information on the exposure assessments of trichloramine in these three Belgian studies (Bernard et al. Citation2003, Citation2006, Citation2009) is very sparse regarding sampling time, where measurements were performed and number of samples. This makes it difficult to relate the exposure of trichloramine to the effects reported.
Studies in other European countries such as Spain (Font-Ribera et al. Citation2009, Citation2014), the United Kingdom (Font-Ribera et al. Citation2011) and the Netherlands (Jacobs et al. Citation2012) found no association between cumulative swimming pool attendance and respiratory symptoms among children. These studies were population-based and had larger sample sizes.
In the Spanish study, Font-Ribera et al. (Citation2009) investigated the prevalence of asthma in children related to early pool attendance. A questionnaire was sent to the parents of 3223 children aged 9–12 years. Data were collected concerning the children’s pool attendance and respiratory symptoms the last 12 months. The children attended different swimming pools; most of them were chlorinated (median air trichloramine concentration 0.16 mg/m3). Swimming pool attendance during the last 12 months was associated with a slightly lower prevalence (OR, 95% CI) of current asthma (0.5, 0.2–0.8) compared to those children who never attended pools. A similar (non-significant) finding was shown for ever asthma (0.7, 0.4–1.1). Swimming pool attendance during summer was associated with less rhinitis and allergic rhinitis.
A prospective longitudinal study was performed in a population-based British cohort comprising 5738 children (50.7% boys) followed by questionnaires up to 10 years of age. Data on swimming were collected at 0.5–6.7 years of age, and data on respiratory symptoms, allergies, asthma and asthma medication at 7 and 10 years. Spirometry and a skin prick test were performed at 7–8 years. No increased risk of asthma or any respiratory symptoms associated to swimming pool attendance was found either in all or in atopic children. Children with high cumulative swimming pool attendance from birth to 7 years had an OR (95% CI) of 0.9 (0.6–1.4) and 0.5 (0.3–0.9), for ever and current asthma, respectively, at 7 years, and a 0.2 (95% CI 0.02–0.4) standard deviation increase in midexpiratory flow, compared to those with low cumulative exposure. Children with asthma and high cumulative swimming pool attendance had lower prevalence of current asthma at 10 years (OR 0.3, 95% CI 0.14–0.8) than those with low cumulative swimming pool attendance. The authors concluded that swimming was associated with increased lung function and a lower prevalence of asthma symptoms, especially among children with preexisting respiratory conditions (Font-Ribera et al. Citation2011). Air levels of trichloramine were not reported.
Font-Ribera et al. (Citation2014) performed a cross-sectional survey among 2758 children aged 6–12 years in Spain. Data on previous and current swimming pool attendance, health status and respiratory symptoms the last 12 months were collected via parental questionnaire. Current regular swimming in indoor pools was not associated with respiratory symptoms such as wheezing and rhinitis in the last 12 months. Regular indoor pool attendance before 2 years of age was not associated with ever had or having asthma or wheezing. Air levels of trichloramine were not reported.
The study by Jacobs et al. (Citation2012) included 2359 Dutch children aged 6–13 years, of which 419 children were tested for allergen sensitization and CC16 levels in serum. The parents were given a questionnaire concerning swimming pool attendance, upper and lower respiratory symptoms, allergy and eczema. Measurements of airborne trichloramine were performed by stationary sampling in 9 swimming pool facilities visited on a regular basis by 77% of the study population. The average concentration of airborne trichloramine was 0.21 mg/m3. The prevalence of physician-diagnosed asthma was 10%, and 6% reported current asthma symptoms. Among 419 children with available serum and questionnaire information, 29% were sensitized to at least one of five allergens tested. CC16 levels were associated with sensitization to house dust mites and common allergens but not with respiratory symptoms. Reported swimming pool attendance and trichloramine exposure were not associated with asthma, wheezing or CC16 levels.
Bernard et al. (Citation2015) measured CC16, SP-D, total and allergen-specific IgE in 835 school children (470 girls, 365 boys; mean age 15.4 years). Data on pool attendance, general and respiratory health were collected via parental questionnaire. The prevalence of physician-diagnosed asthma was 12.4% in boys, and it was 8.5% in girls. In contrast, 43% of the boys were sensitized to any aeroallergen, compared with 33% of the girls. The CC16/SP-D ratio was calculated and was believed to integrate changes in permeability (SP-D) and secretory function (CC16) of the airway epithelium. Children with the highest pool attendance (total or before the age of 7 years) had significantly lower serum levels of CC16 and CC16/SP-D ratios than other children. CC16 levels were not associated with any sensitization (pollen, house mite or pets) or prevalence of asthma, hay fever and rhinitis. Among boys, a low CC16/SP-D ratio was associated with a higher prevalence of house dust mite sensitization, of allergic rhinitis in those sensitized to house dust mite and of asthma in those sensitized to any aeroallergen (OR 3.4, 95% CI 1.2–11), house dust mite (OR 5.2, 95% CI 1.4–24) or pollen (OR 5.8, 95% CI 1.5–27). Among girls, a low CC16/SP-D ratio was associated with pet sensitization and with hay fever in those sensitized to pollen, but not with asthma. Air levels of trichloramine were not reported.
In a recent study, Andersson et al. (Citation2015) investigated the prevalence of physician-diagnosed asthma and respiratory symptoms among 1866 Swedish school children aged 11–12 years (boys 51%) by questionnaire. Of them, 1652 also participated in skin prick testing. Current swimming pool attendance was reported as ≥1/week or <1/week in the last 12 months. The prevalence of current asthma was 8.9% (boys 10%, girls 7.9%). Stratified analyses for allergic sensitization adjusted for gender, parental smoking, parental asthma and damp housing showed statistically significant associations (OR, 95% CI) between physician-diagnosed asthma (1.9, 1.1–3.3) and current asthma (1.9, 1.1–3.3) and pool attendance among atopic children. Air levels of trichloramine were not reported.
11.3.1.5 Conclusion
Exposure to trichloramine in the workplace air may aggravate asthma symptoms in individuals with existing asthma (Thickett et al. Citation2002; Dang et al. Citation2010). Some studies have shown higher prevalences of self-reported asthma-related symptoms (Jacobs et al. Citation2007; Dang et al. Citation2010), physician-diagnosed asthma, asthma attacks and use of asthma medication (Jacobs et al. Citation2007) in exposed workers compared to an unexposed reference group. The overall mean concentration of airborne trichloramine in the study by Jacobs et al. (Citation2007) was 0.56 (0.13–1.34) mg/m3. No significant associations between self-reported asthma-related symptoms and trichloramine exposure were found in other studies (King et al. Citation2006; Massin et al. Citation2007; Fornander et al. Citation2013), even at higher mean exposure levels (Fantuzzi et al. Citation2013).
Young competitive swimmers reported significantly more symptoms from lower airways in general and during training sessions (trichloramine air levels: 0.26–0.41 mg/m3) than referents, but there was no association between trichloramine levels and symptoms (Lévesque et al. Citation2006).
A high prevalence of airway hyperreactivity has been found in pool workers (Demange et al. Citation2009) as well as in competitive swimmers (Helenius et al. Citation1998; Seys et al. Citation2015). There are, however, indications that the increased hyperreactivity is partially reversible when exposure cease or decrease (Helenius et al. Citation2002; Seys et al. Citation2015). There were no associations between exposure to trichloramine and objective clinical tests of airway hyperreactivity (Massin et al. Citation1998) and airway inflammation (Fornander et al. Citation2013).
A high prevalence of work-related asthma among lifeguards (Rosenman et al. Citation2015) as well as in competitive swimmers (Goodman and Hays Citation2008; Mountjoy et al. Citation2015) has been reported, and two studies indicated that occupational exposure to trichloramine may contribute to development of asthma in individuals without previous respiratory diseases (Thickett et al. Citation2002; Nordberg et al. Citation2012). Some cross-sectional studies indicate an association between cumulative attendance in chlorinated pools and an increased risk of asthma among atopic school children (Bernard et al. Citation2003, Citation2006, Citation2009; Andersson et al. Citation2015). These findings are, however, contradicted by results from other large population-based studies in children (Font-Ribera et al. Citation2009, Citation2011; Jacobs et al. Citation2012; Font-Ribera et al. Citation2014).
11.3.2. Other effects
In a study by Zierler et al., the cancer mortality in 43 communities in Massachusetts using either chlorine or monochloramine for disinfection of drinking water was investigated. Residents exposed to chloraminated water were used as controls assuming less exposure to chlorination-by-products (trihalomethanes). In general, cancer mortality was not associated with type of disinfectant used, except a slight association for chlorine use and bladder cancer. A small increase in mortality from influenza and pneumonia was noted in chloraminated communities (Zierler et al. Citation1986). In a later case–control study, the relationship between residence in communities using chlorine for disinfection and mortality in bladder cancer remained (Zierler et al. Citation1988). However, such relationship has not consistently been shown in other studies. Again, exposure to chloraminated drinking water was used as control, and the results from these studies cannot be used to evaluate adverse health effects from exposure to inorganic chloramines.
11.4. Genotoxic effects
No data were located.
11.5. Carcinogenic effects
No data were located.
11.6. Reproductive and developmental effects
No data were located.
12. Dose–effect and dose–response relationships
12.1. Monochloramine
No inhalation data were located.
12.1.1. Human studies
Daily consumption of drinking water containing monochloramine corresponding to 0.043 mg/kg bw/day (assuming a body weight of 70 kg) for 4 weeks did not alter parameters of lipid and thyroid metabolism in 16 healthy men, whereas 0.32 mg/kg bw/day was associated with an increase in the plasma level of apolipoprotein B (Wones et al. Citation1993).
No effects were shown in 10 healthy male volunteers administered monochloramine in water daily for 12 weeks (corresponding to 0.036 mg/kg bw/day assuming a body weight of 70 kg) or every third day for 13 days at increasing concentrations corresponding to ≤0.034 mg/kg bw/day as evaluated by an extensive battery of measures including serum chemistry, blood count, urine analysis and physical examination (Lubbers et al. Citation1982).
No relevant studies on long-term exposure were located.
12.1.2. Animal studies
Animal studies on monochloramine are summarized in (Section 10). Clear conjunctival irritation was observed in rabbits after 1 h of exposure to a solution containing monochloramine (corresponding to 4 mg/l Cl2). Monochloramine was considerably more irritating than free chlorine (Eichelsdörfer et al. Citation1975).
Transiently increased GSH levels in rats were reported after single oral gavage doses of 0.19–0.75 mg/kg bw of monochloramine (Abdel-Rahman et al. Citation1984).
Some short-term drinking water studies have been performed. After 9-week exposures of rats to 0.8–3.4 mg/kg bw/day of monochloramine, some alterations in immune function were observed (Exon et al. Citation1987). In mice, administration for 28 days at dose levels of 0.38–38 mg/kg bw/day resulted in some minimal immunological effects that were judged to be biologically insignificant. No effects were observed in hematological parameters, organ weights, body weight, body weight gain or water consumption (Guo et al. Citation2011). No effects on body weight gain or hematological parameters were observed in rats exposed 45 d at 1.2–12 mg/kg bw/day, except a decrease in methemoglobin, opposite to what was expected (Bull Citation1980). In mice, reduced body weight and slightly increased hematocrit were seen at doses ≥ 8.7 mg/kg bw/day, whereas other hematological parameters (including GSH levels) were unaffected following administration during 30 d at doses of 0.44–35 mg/kg bw/day (Moore et al. Citation1980). Exposure of monkeys for 6 weeks (10 mg/kg bw/day) had no effect on hematological and clinicochemical parameters in blood, on serum thyroxine (T4) levels or body weight (Bercz et al. Citation1982).
Some long-term drinking water studies were located. Following administration of 0.05–5.2 mg/kg bw/day of monochloramine to male rats (4/group) for up to 12 months, an indication of increased DNA synthesis (thymidine incorporation) in kidney, spleen, liver and testes, and decreased blood GSH levels, were seen at all doses (Abdel-Rahman et al. Citation1984).
Male and female rats and mice were administered monochloramine in drinking water (2.1–17.2 mg/kg bw/day) for up to 2 years. No carcinogenic effects were seen in mice and male rats. A marginal, dose-related increase in mononuclear cell leukemia incidence among female rats was considered as equivocal evidence of carcinogenic activity (NTP. Citation1992).
Reduced body weight gain and minor biochemical, hematological, immunological and histopathological changes were observed in rats exposed to 21.6 mg/kg bw/day of monochloramine for 13 weeks in drinking water. The effects were largely related to reduced water and food consumption (Poon et al. Citation1997). Similar effects were reported also in other long-term studies in rats and mice at daily doses of 5–17 mg/kg bw/day (Abdel-Rahman et al. Citation1984; Daniel et al. Citation1990, Citation1991; NTP. 1992).
A few oral studies on reproductive or developmental toxicity were found. After administration of 1.6–8 mg/kg bw/day of monochloramine by oral gavage for 5 consecutive days to mice, no sperm-head abnormalities were observed 1, 3 and 5 weeks after the last dose (Meier et al. Citation1985). No histological changes or effects on reproductive organs were noted in male and female rats administered monochloramine (2.5–10 mg/kg bw/day) by oral gavage for up to 73 d. Sperm quality and fetuses were unaffected (Carlton et al. Citation1986). Neither were any effects on fetuses shown after exposure of Virgin rats to monochloramine via drinking water (0.09–9.3 mg/kg bw/day) 2.5 months prior to and throughout gestation (Abdel-Rahman et al. Citation1982).
12.2. Dichloramine
In the only study located, male and female rats were administered dichloramine in drinking water (0.019–24 mg/kg bw/day) for 13 weeks. Minimal to mild histological effects in thyroid, kidneys or gastric cardia were observed from 0.19 mg/kg bw/day in males and from 2.5 mg/kg bw/day in females, respectively ( and Section 10) (Nakai et al. Citation2000).
12.3. Trichloramine
12.3.1. Human studies
12.3.1.1 Ocular and airway irritation
Several cross-sectional studies have shown higher prevalences of self-reported ocular and/or upper airway irritation among pool workers exposed to trichloramine compared to unexposed reference groups (). Pool workers are mainly exposed to trichloramine in air, whereas food industry workers and competitive swimmers to a higher degree are exposed also to soluble chlorine, in aerosols and/or the chlorinated water itself. Only swimming pool workers are therefore included in the following compilation of irritant effects of airborne trichloramine.
Table 10. Ocular and airway irritation in swimming pool workers occupationally exposed to airborne trichloramine (exposure levels refer to stationary sampling if not stated otherwise) as reported by questionnaires.
Chu et al. (Citation2013) reported significantly more irritation in the throat in pool workers exposed to trichloramine concentrations of 0.017–0.15 mg/m3 compared to unexposed referents, however, no concentration–response data were presented. Löfstedt et al. (Citation2016) reported significantly more ocular and nasal symptoms during the last week in pool workers with stationary and personal measurements of trichloramine being in the range 0.001–0.64 mg/m3 and < 0.001–0.24 mg/m3 (AMs 0.18 and 0.071 mg/m3), respectively, compared to referents, but there was a lack of concentration–response relationships among exposed. Also some other studies did not find any concentration–response relationships between airborne trichloramine levels and self-reported irritation (Jacobs et al. Citation2007; Fornander et al. Citation2013).
In contrast, concentration–response relationships were demonstrated in some studies. Significantly higher prevalences of irritative symptoms in eyes and nose were reported in pool workers in one study at 0.36 (0.30–0.52) mg/m3 of trichloramine (Parrat et al. Citation2012) and in three other studies with pool workers from around 0.5 mg/m3 (Héry et al. Citation1995; Massin et al. Citation1998; Fantuzzi et al. Citation2013). In addition, significant concentration-response trends for eye, nose and throat irritation over the whole exposure range (4 sub-groups) were reported for lifeguards (Massin et al. Citation1998).
An association between the prevalence of irritation in upper airways or eyes and cumulative trichloramine exposure was observed in some studies (Massin et al. Citation1998; Jacobs et al. Citation2007; Parrat et al. Citation2012). However, the cumulative exposures were calculated in different ways and are difficult to interpret in terms of exposure concentration. NOAECs and LOAECs can, therefore, not be identified from the cumulative exposure data.
12.3.1.2 Effects on pneumoproteins and lung function
Respiratory effects after short-term exposure are summarized in . Effects on pneumoproteins were shown at trichloramine concentrations ≥0.29 mg/m3 (Carbonnelle et al. Citation2002; Bernard et al. Citation2003; Font-Ribera et al. Citation2010), but not at lower exposure levels (Carbonnelle et al. Citation2008; Nordberg et al. Citation2012).
Table 11. Respiratory effects in humans following short-term exposure to airborne trichloramine (exposure levels refer to stationary sampling if not stated otherwise).
Marginal but significant effects on lung function were demonstrated in one study with trichloramine concentrations of 0.15 and 0.23 mg/m3 (personal exposure) (Nordberg et al. Citation2012). In contrast, no effects on lung function were demonstrated in studies with exposure levels of 0.023 mg/m3 (Westerlund et al. Citation2019), 0.18 mg/m3 (personal exposure 0.071 mg/m3) (Löfstedt et al. Citation2016), 0.16–0.28 mg/m3 (Carbonnelle et al. Citation2008), 0.29 mg/m3 (Font-Ribera et al. Citation2010) and 0.36 and 0.49 mg/m3 (Carbonnelle et al. Citation2002).
An increase in FENO over work shift was observed in pool workers exposed to 0.18 mg/m3 of trichloramine, suggesting an inflammatory effect on the airways (Löfstedt et al. Citation2016).
12.3.1.3 Asthma
Exposure to trichloramine in the workplace air may aggravate asthma symptoms in individuals with existing asthma (Thickett et al. Citation2002; Dang et al. Citation2010). The average concentration of trichloramine reported in the study by Dang et al. (Citation2010) was 0.44 mg/m3. In the case report by Thickett et al., 2 pool workers with occupational asthma had a positive specific challenge test to trichloramine at 0.5 mg/m3, and the third pool worker had a positive workplace challenge test to indoor pool air with trichloramine levels of 0.1–0.57 mg/m3 (Thickett et al. Citation2002).
Some studies showed higher prevalences of self-reported asthma-related symptoms in exposed pool workers and competitive swimmers compared to unexposed referents (Lévesque et al. Citation2006; Jacobs et al. Citation2007; Dang et al. Citation2010). The overall mean concentration of trichloramine in the study by Jacobs et al. (Citation2007) was 0.56 (0.13–1.34) mg/m3; the air levels in the study by Lévesque et al. (Citation2006) were 0.26–0.41 mg/m3. A high prevalence of airway hyperreactivity has been found in pool workers (Demange et al. Citation2009), as well as in competitive swimmers (Helenius et al. Citation1998; Seys et al. Citation2015). No significant associations have been found between levels or duration of trichloramine exposure and self-reported asthma symptom, pulmonary function, airway hyperreactivity and airway inflammation.
12.3.2. Animal studies
Animal studies on trichloramine are summarized in (Section 10). In female mice, lung epithelium hyperpermeability (expressed as changes in concentrations of albumin and total proteins in BALF, and of CC16 in BALF and serum) was observed after single inhalation exposures at 11.9 and 13.1 mg/m3 up to 8 h. No effects were seen at 0.53–3.45 mg/m3 (Carbonnelle et al. Citation2002).
An RD50 value of 12.5 mg/m3 in mice was reported following a 1-h inhalation exposure to trichloramine (Gagnaire et al. Citation1994).
In rats, an LC50 value of 560 mg/m3 following 1 h of exposure was determined. The cause of death was lung edema (Barbee et al. Citation1983).
In the only long-term study located, male and female rats were administered trichloramine in drinking water (0.020–13 mg/kg bw/day) for 13 weeks. Minimal to mild histological effects in thyroid and kidneys were observed at doses > 0.23 mg/kg bw/day in males and > 0.29 mg/kg bw/day in females, respectively. Increased hepatic enzyme activities were observed among females only (13 mg/kg bw day) (Nakai et al. Citation2000). It is unclear how these effect levels were derived from the results presented in the paper.
13. Previous evaluations by national and international bodies
Some previous evaluations regarding inorganic chloramines have been performed with the main focus on monochloramine in drinking water. A few bodies have evaluated the health effects of trichloramine following inhalation exposure.
13.1. Chloramines/monochloramine
13.1.1. US Environmental Protection Agency (US EPA) Citation1994, Chloramines
US EPA concluded that several short-term studies on monochloramine showed no observed adverse hematological effects in mice, rats and monkeys. EPA further concluded that studies regarding mutagenicity of chloramines were inconclusive and stated that equivocal evidence of carcinogenic activity was found in female rats because of a slightly increased incidence of mononuclear cell leukemia. There was no evidence of carcinogenic activity in male rats, female or male mice. As regards human health, it was noted that no epidemiologic studies had been designed to address specifically the potential adverse health effects of exposure to chloramines. The EPA Carcinogenic Risk Assessment Verification Endeavor (CRAVE) Work group verified (12/02/92) a classification for monochloramine of group D, not classifiable as to human carcinogenicity, meaning that there is inadequate human and animal evidence of carcinogenicity (US EPA Citation1994).
13.1.2. Government of Canada. Healthy Canadians 1996, Monochloramine
Monochloramine was considered weakly mutagenic in vitro. No treatment-related developmental or reproductive effects had been observed in rats exposed to monochloramine in drinking water. Some possible immunological effects were noted, but the biological significance of these effects were considered not clear. An administered dose of 3.8 mg/kg bw had been reported to cause a reduction in spleen weight and augmented production of prostaglandin E2 in male rats. The available epidemiologic studies were regarded inadequate for the assessment of carcinogenicity of monochloramine in humans. There were some clinical evidence of neoplastic responses in rats and mice following chronic exposure to monochloramine in drinking water. The evidence for the carcinogenicity of monochloramine was considered to be limited and the compound was classified as being possibly carcinogenic to humans (inadequate evidence in humans, some evidence in animals).
A tolerable daily intake was estimated to 0.048 mg/kg bw per day and a maximum acceptable concentration (MAC) for monochloramine in drinking water of 1.8 mg/l was derived (Health Canada Citation1995).
13.1.3. International Agency for Research on Cancer (IARC.) Citation2004, Chloramines
No human carcinogenicity data specifically on chloramine were available to the Working Group. Chloraminated drinking-water (predominantly in the form of monochloramine) tested for carcinogenicity by oral administration in female and male mice and rats did not demonstrate clear evidence of carcinogenic activity. Ingestion of monochloramine produced no clinical abnormalities in male volunteers at a concentration of 15 mg/l. No reproductive or developmental effects had been associated with monochloramine. Monochloramine induced single-strand breaks and loss of DNA-transforming activity and was a week mutagen in B. subtilis. It was not mutagenic to S. typhimurium. In vitro, monochloramine caused double-strand breaks and chromatin condensation in rabbit gastric mucosal cells and human stomach cancer cells. In vivo, monochloramine did not induce micronuclei, chromosomal aberration, aneuploidy or sperm abnormality in mice, but induced the formation of micronuclei in erythrocytes of newt larvae.
IARC concluded that there was inadequate evidence in humans for carcinogenicity of chloramine and inadequate evidence in experimental animals for the carcinogenicity of monochloramine. The overall evaluation was that chloramine was not classifiable as to its carcinogenicity to humans (Group 3) (IARC Citation2004).
13.1.4. World Health Organization (WHO) Citation2011, Monochloramine
WHO concluded that although monochloramine had been shown to be mutagenic in some in vitro studies, it had not been found to be genotoxic in vivo.
A tolerable daily intake (TDI) of 94 µg/kg bw was calculated based on the highest dose administered to male rats in a 2-year drinking water study by NTP (NTP Citation1992). An additional uncertainty factor for possible carcinogenicity was not applied because equivocal cancer effects reported in the NTP study in only one species and in only one sex were within the range observed in historical controls. No other increases in tumor incidence were observed (WHO Citation2011).
13.2. Trichloramine
13.2.1. World Health Organization (WHO) 2006
WHO based its recommendations on the study by Héry et al. (Citation1995) in which onset of irritative symptoms in the eyes and throat were reported at an air level of 0.5 mg/m3 of trichloramine; at 0.7 mg/m3 all of the staff reported irritative symptoms. WHO thus recommended a maximum trichloramine level of 0.5 mg/m3 in indoor swimming pool air as a comfort value, in order to protect the visitors from possible health effects (WHO. Citation2006).
13.2.2. German Working Group on Indoor Guide Values of the Federal Environment Agency and the States’ Health Authorities Citation2011
In its risk assessment of trichloramine in the air of indoor swimming pools, the Working Group noted that few human and animal studies were available (no repeated exposure studies in animals). A valid lowest observed adverse effect level (LOAEL) could therefore not be derived from the current studies and no toxicologically based indoor air guide value established. Instead, the Swiss technical standard of 0.2 mg/m3 of trichloramine (SIA Citation2011) was adopted to protect the public against irritation from air in indoor swimming pools. As toxicological and epidemiological support, it was referred to the absence of irritation in recreational swimmers following a few hours of exposure to 0.5 mg/m3 of trichloramine (Carbonnelle et al. Citation2002), whereas irritation occurred in competitive swimmers and lifeguards after repeated exposure near 0.4 mg/m3 (Data presented by Parrat J at a lecture in Dessau 2-3.3 2009) (Working Group on Indoor Guide Values of the Federal Environment Agency and the States’ Health Authorities Citation2011).
13.2.3. Ministry of Social Affairs and Health, Finland 2012
In the evaluation of data on trichloramine, respiratory irritation was identified as the critical effect for the setting of an OEL. It was recognized that such effects had been observed at exposure levels starting from 0.5 mg/m3 (Héry et al. Citation1995; Jacobs et al. Citation2007). On the basis of these findings, an 8-h OEL of 0.5 mg/m3 was set in 2012 (Ministry of Social Affairs and Health Citation2018).
14. Evaluation of human health risks
14.1. Assessment of health risks
Inorganic chloramines are not commercially available, but monochloramine is produced in situ for disinfection or for use in chemical synthesis. Mono, di- and trichloramine are also formed when free chlorine reacts with nitrogen containing substances present in e.g. chlorinated water sources.
Monochloramine and dichloramine are water-soluble but monochloramine is the principal chloramine in chlorine disinfected water. Trichloramine is immiscible with water and evaporates rapidly into the air and is the predominant inorganic chloramine in the indoor air of swimming pool facilities. In contrast, in the food processing industry, a significant amount of the inorganic chlorine species in the air is soluble. The primary occupational exposure route for the inorganic chloramines is inhalation. Drinking water studies are, therefore, considered to be of low relevance in the occupational setting.
Air monitoring has mostly been performed as stationary sampling. The results may deviate from results obtained by personal sampling. Linear regression analyses based on data from parallel 8-h samplings of trichloramine in pool air suggested a relation between personal and stationary sampling of approximately 1:2 (Westerlund et al. Citation2015, Citation2019).
14.1.1. Monochloramine
The data base is scarce and no inhalation data were located.
No clinical effects were seen among male healthy volunteers ingesting monochloramine in drinking water daily during 4 or 12 weeks in doses corresponding to 0.043 mg/kg bw/day (Wones et al. Citation1993) or 0.034 mg/kg bw/day (Lubbers et al. Citation1982), respectively.
Monochloramine was regarded considerably more eye irritating than free chlorine in a study on rabbits (Eichelsdörfer et al. Citation1975). Minor immunological and hematological effects after short-term oral exposure (0.8–38 mg/kg bw/day) were reported in some studies in rodents (Bull Citation1980; Moore et al. Citation1980; Exon et al. Citation1987; Guo et al. Citation2011). No hematological or clinicochemical effects were seen in monkeys after 6 weeks of oral exposure to 10 mg/kg bw/day (Bercz et al. Citation1982). A transient increase in GSH production was observed in rats after single oral gavage doses of approximately 0.19–0.75 mg/kg bw (Abdel-Rahman et al. Citation1984).
In long-term drinking water studies, reduced body weight gain and minor biochemical, hematological, immunological and histopathological changes were observed in rats exposed to 21.6 mg/kg/day for 13 weeks. The effects were largely related to reduced water and food consumption (Poon et al. Citation1997). Similar effects were reported also in other long-term studies in rats and mice at daily doses of 5–17 mg/kg bw/day (Abdel-Rahman et al. Citation1984; Daniel et al. Citation1990; Daniel et al. Citation1991; NTP Citation1992).
No carcinogenic effects were seen in mice or male rats administered monochloramine in drinking water for up to 2 years. A marginal increase in mononuclear cell leukemia incidence among female rats was considered as equivocal evidence of carcinogenic activity (NTP Citation1992). Limited data indicate that monochloramine is weakly mutagenic in vitro but not genotoxic in vivo.
No reproductive or developmental effects were shown in the few animal studies located.
14.1.2. Dichloramine
No human data were located.
In the only animal study located, mild histopathological effects in kidneys, thyroid and gastric cardia were observed among male and female rats administered dichloramine in drinking water for 13 weeks (Nakai et al. Citation2000).
14.1.3. Trichloramine
The animal database is meager and comprise to our knowledge three single inhalation studies and a 13-week drinking water study. However, there were no indications of adverse systemic effects from trichloramine exposure, even after acute inhalation exposure at lethal concentrations. Human studies have mainly focused on ocular and airway irritation and pulmonary effects.
14.1.3.1. Irritation
Trichloramine is a strong sensory irritant and accidental high short-term airborne exposure may cause acute irritative symptoms in humans, mainly in the upper airways and eyes (Sanderson et al. Citation1995; Bowen et al. Citation2007). Several cross-sectional studies have shown higher prevalences of self-reported ocular and upper airway irritation among competitive swimmers and workers with occupational exposure to trichloramine compared to unexposed reference groups. In these studies, men and women as well as adolescents and children were included, but results regarding health effects were generally not presented separately for the different groups. Pool workers are mainly exposed to trichloramine in air, whereas food industry workers and competitive swimmers to a higher degree are exposed also to soluble chlorine, in aerosols and/or the chlorinated water itself. Only swimming pool workers are therefore included in the assessment of airborne trichloramine.
Irritative symptoms in eyes and upper respiratory tract are evident at airborne trichloramine levels of 0.5 mg/m3 (Héry et al. Citation1995; Massin et al. Citation1998; Fantuzzi et al. Citation2013). In another study, irritation symptoms were more frequent among pool workers exposed to 0.36 (0.30–0.52) mg/m3 than among referents (Parrat et al. Citation2012).
Irritation below 0.5 mg/m3 has been reported in some additional studies (Chu et al. Citation2013; Löfstedt et al. Citation2016). However, due to lack of exposure-response relationships, these studies were considered less suited to form the basis for a health-based OEL. Nevertheless, they provide additional support for irritation as the critical effect.
It should be noted that the measurements were performed by stationary sampling in these studies.
Duration of exposure seems to be of importance; an association between the prevalence of irritative symptoms in exposed pool workers and cumulative trichloramine exposure has been shown (Massin et al. Citation1998; Jacobs et al. Citation2007; Nordberg et al. Citation2012; Parrat et al. Citation2012). However, the data are not presented in a way that allows the cumulative exposure estimates to be used as basis for a health-based OEL.
The human irritation data are supported by animal data. Depressed breathing was shown in rats at a single exposure (60 min) to trichloramine at 4.5–25.0 mg/m3 giving an estimated RD50 value of 12.5 mg/m3 (Gagnaire et al. Citation1994).
Overall, irritation is considered to be the critical effect of trichloramine. However, despite a large amount of human data, it is difficult to draw a firm conclusion regarding a NOAEC. The study by Parrat et al. (Citation2012) suggests a LOAEC around 0.36 mg/m3, rounded to 0.4 mg/m3.
14.1.3.2. Pneumoproteins and lung function
A marginal but significant decrement in lung function was demonstrated in one study after exposure to airborne trichloramine levels of 0.15 mg/m3 and 0.23 mg/m3 (both personal sampling) (Nordberg et al. Citation2012). In contrast, no effects on lung function were demonstrated in other studies with average exposure levels of 0.02–0.49 mg/m3 (stationary sampling) (Carbonnelle et al. Citation2002; Carbonnelle et al. Citation2008; Font-Ribera et al. Citation2010; Löfstedt et al. Citation2016; Westerlund et al. Citation2019). Transient changes in serum pneumoproteins associated with changes in lung epithelium permeability were seen in some studies at airborne trichloramine concentrations ≥0.3 mg/m3 (Carbonnelle et al. Citation2002; Bernard et al. Citation2003; Font-Ribera et al. Citation2010), whereas no such effects were observed in studies at lower mean exposure levels (Carbonnelle et al. Citation2008; Nordberg et al. Citation2012).
One animal study supports an effect on pneumoproteins. Mice inhalation exposed to 12 mg/m3 of trichloramine for 1–8 h showed an increased bidirectional leakage of proteins across the pulmonary epithelium. These effects were not seen at 0.53–3.45 mg/m3 (Carbonnelle et al. Citation2002).
The results on lung function are inconsistent and the interpretation and clinical relevance of changes in pneumoproteins is uncertain at present, therefore these studies cannot be used to derive a recommendation for a health-based OEL.
14.1.3.3. Asthma
Exposure to trichloramine in the workplace air may aggravate asthma symptoms in individuals with existing asthma (Thickett et al. Citation2002; Dang et al. Citation2010).
Some studies showed higher prevalences of self-reported asthma-related symptoms in exposed pool workers (work-related) (Jacobs et al. Citation2007; Dang et al. Citation2010) and competitive swimmers (during training) (Lévesque et al. Citation2006) compared to unexposed reference groups or other competitive athletes or athletes in other aquatic disciplines (Goodman and Hays Citation2008; Mountjoy et al. Citation2015).
A high prevalence of airway hyperreactivity has been found in pool workers (Demange et al. Citation2009) as well as in competitive swimmers (Helenius et al. Citation1998; Seys et al. Citation2015).
Limited data indicate that occupational exposure to trichloramine may contribute to the development of asthma in individuals without previous respiratory diseases (Thickett et al. Citation2002; Nordberg et al. Citation2012). Some cross-sectional studies indicate an association between cumulative attendance in chlorinated pools and an increased risk of asthma among atopic school children (Bernard et al. Citation2003; Bernard et al. Citation2006, Citation2009; Andersson et al. Citation2015), but these findings are contradicted by results from other large population-based studies in children (Font-Ribera et al. Citation2009, Citation2011; Jacobs et al. Citation2012; Font-Ribera et al. Citation2014).
No significant associations have been found between levels or duration of trichloramine exposure and self-reported asthma symptom, pulmonary function, airway hyperreactivity and airway inflammation (Massin et al. Citation1998; King et al. Citation2006; Lévesque et al. Citation2006; Massin et al. Citation2007; Fantuzzi et al. Citation2013; Fornander et al. Citation2013).
In conclusion, the risk of developing asthma following long-term exposure to trichloramine cannot be evaluated at present.
14.1.3.4. Other data
Data on genotoxicity, carcinogenicity and reproductive and developmental toxicity are lacking.
14.2. Groups at extra risk
Inorganic chloramines are regarded as irritants. Asthmatics and atopic individuals who are hyperreactive to other respiratory irritants are expected to be more sensitive to these substances.
14.3. Scientific basis for an occupational exposure limit
There are insufficient toxicological data to recommend health-based OELs for monochloramine and dichloramine.
The major effect after trichloramine exposure is self-reported irritation of the eyes and upper respiratory tract. Irritation is considered to be the critical effect and has been reported in many studies on pool workers. Several of these studies reported irritation at approximately 0.5 mg/m3 of trichloramine (Héry et al. Citation1995; Massin et al. Citation1998; Fantuzzi et al. Citation2013). One study showed irritation at slightly lower trichloramine levels, 0.36 (0.30–0.52) mg/m3 (Parrat et al. Citation2012). Therefore, 0.4 mg/m3 is regarded as the LOAEC and is used to derive a recommended health-based OEL.
Considering that there are no indications of adverse systemic effects from trichloramine exposure, the sharpness of the concentration-effect curve for irritation and that a LOAEC is used as point of departure, an assessment factor of 2 is applied, to account for extrapolation from LOAEC to NOAEC (Nielsen et al. Citation2007). As the irritation data are collected from various field studies with occupationally exposed subjects, no assessment factor for interindividual variability is needed. The studies on pool workers reviewed in this document used stationary sampling to determine the trichloramine concentrations. Two studies with stationary and personal sampling carried out in parallel suggest that the stationary method may overestimate the true personal exposure in swimming pool facilities (Section 14.1) (Westerlund et al. Citation2015, Citation2019). This motivates an additional assessment factor of 2 when personal monitoring is applied.
The resulting recommended health-based OEL is 0.1 mg/m3 (0.4/2/2) as an 8-h time-weighted average. This corresponds to 0.2 mg/m3 for stationary measurements in swimming pool facilities.
No short-term exposure limit (STEL) is recommended as currently available analytical methods require longer sampling times.
No measurement difficulties are foreseen at the recommended health-based OEL.
15. Research needs
15.1. Air sampling and analysis
The air sampling method and the chemical analytical methods used today are relatively nonspecific. Methods for analysis and identification of monochloramine, dichloramine or trichloramine in air samples should be developed.
Today pumped sampling is used and the equipment is relatively heavy to wear; a diffusive sampler for the inorganic chloramines should be developed.
15.2. Health effects
Long-term animal studies are needed to establish a NOAEC for inhalation exposure.
Data on human toxicokinetics and on mechanisms of toxicity are lacking. More studies regarding short-term effects of the inorganic chloramines in humans should be performed, preferably in an exposure chamber under controlled conditions to establish human NOAECs. For future occupational studies, exposure should be assessed by personal sampling in parallel to a standardized validated questionnaire regarding human health effects. In addition to studying self-reported symptoms for evaluation of health effects following exposure to inorganic chloramines, more studies should be performed using objective measurements including effect markers such as FENO, inflammatory markers or eye and nose irritation tests. Possible gender differences in exposure and health effects should be evaluated. Larger longitudinal studies with sufficient statistical power are needed to evaluate the possible risk of developing asthma following long-term exposure to the inorganic chloramines, especially in adolescents and children with atopy. Data are lacking regarding possible persistent health effects after cessation of exposure.
16. Conclusions
The toxicological data for mono- and dichloramine are insufficient to recommend health-based occupational exposure limits (OELs).
As regard trichloramine, the critical effect is judged to be irritation observed in several studies on pool workers, starting at approximately 0.4 mg/m3 (stationary sampling). Based on these data, a health-based OEL of 0.1 mg/m3 (8-h time-weighted average) is recommended. This corresponds to 0.2 mg/m3 for stationary measurements in swimming pool facilities. No short-term exposure limit (STEL) is recommended.
All criteria documents produced by NEG may be downloaded from www.nordicexpertgroup.org,
Declaration of interest
The authors had no conflicts of interests to declare.The NEG secretariat is financially supported by the Swedish Work Environment Authority and the Norwegian Ministry of Labor and Social Affairs.
Supplemental material
Supplemental material for this article is available online here.
Data bases used in search of literature
In the search for literature the following data bases were used: Arbline, Chemical Abstracts, HSELINE, Medline EBSCO, Medline OVID, NIOSHTIC, PubMed, Toxline. Last complete search was performed in February 2017.
Abbreviations | ||
AM | = | arithmetic mean |
BALF | = | bronchoalveolar lavage fluid |
CC16 | = | club cell protein 16 kDa (formerly called Clara cell protein) |
CI | = | confidence interval |
CysLT | = | cysteinyl leukotriene |
DENA | = | diethylnitrosamine |
EIB | = | exercise-induced bronchoconstriction |
FENO | = | fraction of exhaled nitric oxide |
FEV1 | = | forced expiratory volume in the first second |
FEV% | = | FEV1/FVC × 100 |
FVC | = | forced vital capacity |
GGT | = | γ-glutamyltranspeptidase |
GM | = | geometric mean |
GSH | = | glutathione |
Ig | = | immunoglobulin |
IL | = | interleukin |
LC50 | = | lethal concentration for 50% of the exposed animals at single inhalation exposure |
LDH | = | lactate dehydrogenase |
L-eq | = | liter-equivalents |
LOAEC | = | lowest observed adverse effect concentration (at inhalation) |
LOD | = | limit of detection |
LOQ | = | limit of quantification |
LTB4 | = | leukotriene B4 |
NEG | = | the Nordic Expert Group for Criteria Documentation of Health Risks from Chemicals |
NOAEC | = | no observed adverse effect concentration (at inhalation) |
NOAEL | = | no observed adverse effect level |
NTP | = | National Toxicology Program |
OEL | = | occupational exposure limit |
OR | = | odds ratio |
PEF | = | peak expiratory flow |
PR | = | prevalence ratio |
RD50 | = | concentration causing a 50% decrease in respiratory frequency |
S100-A8 | = | S100 calcium binding protein A8 (also called calgranulin A) |
SD | = | standard deviation |
STEL | = | short-term exposure limit |
SP | = | surfactant-associated protein |
SPIN | = | Substances in Preparations in Nordic Countries |
US | = | United States |
WHO | = | World Health Organization |
Appendix. Exposure limits for trichloramine in air
Download MS Word (45.8 KB)Acknowledgements
This criteria document was prepared in cooperation with the Nordic Expert Group for Criteria Documentation of Health Risks from Chemicals (NEG). NEG’s main task is to produce criteria documents to be used by the regulatory authorities as the scientific basis for setting occupational exposure limits for chemical substances. An evaluation is made of all relevant published, peer-reviewed original literature found. The document aims at establishing dose-response/dose-effect relationships and defining a critical effect. NEG will provide, when possible, recommendations for health-based occupational exposure limits. In the derivation of such limits, the ECHA Guidance on information requirements and chemical safety assessment3 is taken into account. Whereas NEG adopts the document by consensus procedures, thereby granting the quality and conclusions, the authors are responsible for the factual content of the document. The final version was adopted at the NEG meeting 8 May 2019. Editorial work and technical editing were performed by the NEG secretariat. The following experts participated in the elaboration of the document: Gunnar Johansson (Chair, Sweden), Merete Drevvatne Bugge (Norway), Helge Johnsen (Norway), Anne Thoustrup Saber (Denmark), Piia Taxell (Finland), Mattias Öberg (Sweden), the former NEG experts Nina Landvik (Norway), Vidar Skaug (Norway), Helene Stockmann-Juvala (Finland) and Anna-Karin Alexandrie (Sweden, secretary), Jill Järnberg (Sweden, secretary).
Notes
1 Free chlorine: chlorine available to sanitise water, i.e. chlorine and hypochlorite/hypochlorous acid.
2 Soluble chlorine: in occupational settings covered in this document, this means hypochlorite, hypochlorous acid, chlorine, monochloramine and dichloramine.
3 ECHA. Guidance on information requirements and chemical safety assessment. Chapter R.8: Characterisation of dose [concentration]-response for human health. Version 2.1. Helsinki, Finland: European Chemicals Agency, 2012.
References
- Abdel-Rahman MS, Berardi MR, Bull RJ. 1982. Effect of chlorine and monochloramine in drinking water on the developing rat fetus. J Appl Toxicol. 2:156–159.
- Abdel-Rahman MS, Suh DH, Bull RJ. 1984. Toxicity of monochloramine in rat: an alternative drinking water disinfectant. J Toxicol Environ Health. 13:825–834.
- Abdel-Rahman MS, Waldron DM, Bull RJ. 1983. A comparative kinetics study of monochloramine and hypochlorous acid in rat. J Appl Toxicol. 3:175–179.
- Afifi MZ, Blatchley ER 3rd. 2015. Seasonal dynamics of water and air chemistry in an indoor chlorinated swimming pool. Water Res. 68:771–783.
- Andersson M, Hedman L, Nordberg G, Forsberg B, Eriksson K, Rönmark E. 2015. Swimming pool attendance is related to asthma among atopic school children: a population-based study. Environ Health. 14:37.
- ANSES. 2010. Risques sanitaires liés aux piscines. Évaluation des risques sanitaires liés aux piscines. Partie 1: piscines réglementées 2010. Avis de l’Afsset. Rapport d’expertise collective. Maisons-Alfort (France): Agence française de sécurité sanitaire de l’environnement et du travail (AFSSET); p. 254. [accessed March 2019]. https://www.researchgate.net/profile/Jean-Luc_Boudenne/publication/282672748_Evaluation_des_risques_sanitaires_lies_aux_piscines_Partie_1_piscines_reglementees/links/56181b8108ae78721f9a9646/Evaluation-des-risques-sanitaires-lies-aux-piscines-Partie-1-piscines-reglementees.pdf?origin=publication_detail
- Barbee SJ, Thackara JW, Rinehart WE. 1983. Acute inhalation toxicology of nitrogen trichloride. Am Ind Hyg Assoc J. 44:145–146.
- Bercz JP, Jones L, Garner L, Murray D, Ludwig DA, Boston J. 1982. Subchronic toxicity of chlorine dioxide and related compounds in drinking water in the nonhuman primate. Environ Health Perspect. 46:47–55.
- Bernard A, Carbonnelle S, de Burbure C, Michel O, Nickmilder M. 2006. Chlorinated pool attendance, atopy, and the risk of asthma during childhood. Environ Health Perspect. 114:1567–1573.
- Bernard A, Carbonnelle S, Dumont X, Nickmilder M. 2007. Infant swimming practice, pulmonary epithelium integrity, and the risk of allergic and respiratory diseases later in childhood. Pediatrics. 119:1095–1103.
- Bernard A, Carbonnelle S, Michel O, Higuet S, De BC, Buchet JP, Hermans C, Dumont X, Doyle I. 2003. Lung hyperpermeability and asthma prevalence in schoolchildren: unexpected associations with the attendance at indoor chlorinated swimming pools. Occup Environ Med. 60:385–394.
- Bernard A, Carbonnelle S, Nickmilder M, de Burbure C. 2005. Non-invasive biomarkers of pulmonary damage and inflammation: application to children exposed to ozone and trichloramine. Toxicol Appl Pharmacol. 206:185–190.
- Bernard A, Lauwerys R. 1995. Low-molecular-weight proteins as markers of organ toxicity with special reference to Clara cell protein. Toxicol Lett. 77:145–151.
- Bernard A, Nickmilder M, Dumont X. 2015. Chlorinated pool attendance, airway epithelium defects and the risks of allergic diseases in adolescents: interrelationships revealed by circulating biomarkers. Environ Res. 140:119–126.
- Bernard A, Nickmilder M, Voisin C, Sardella A. 2009. Impact of chlorinated swimming pool attendance on the respiratory health of adolescents. Pediatrics. 124:1110–1118.
- Bessonneau V, Derbez M, Clément M, Thomas O. 2011. Determinants of chlorination by-products in indoor swimming pools. Int J Hyg Environ Health. 215:76–85.
- Bowen AB, Kile JC, Otto C, Kazerouni N, Austin C, Blount BC, Wong HN, Beach MJ, Fry AM. 2007. Outbreaks of short-incubation ocular and respiratory illness following exposure to indoor swimming pools. Environ Health Perspect. 115:267–271.
- Budavari S. 1989. In Budavari S, O’Neil MJ, editors. The Merck index. An encyclopedia of chemical drugs, and biologicals. 11th ed. New Jersey: Merck, 1989.
- Bull RJ. 1980. Health effects of alternate disinfectants and their reaction products. J Am Water Works Assoc. 72:299–303.
- Carbonnelle S, Bernard A, Doyle IR, Grutters J, Francaux M. 2008. Fractional exhaled NO and serum pneumoproteins after swimming in a chlorinated pool. Med Sci Sports Exerc. 40:1472–1476.
- Carbonnelle S, Francaux M, Doyle I, Dumont X, de Burbure C, Morel G, Michel O, Bernard A. 2002. Changes in serum pneumoproteins caused by short-term exposures to nitrogen trichloride in indoor chlorinated swimming pools. Biomarkers. 7:464–478.
- Cardador MJ, Gallego M. 2011. Haloacetic acids in swimming pools: swimmer and worker exposure. Environ Sci Technol. 45:5783–5790.
- Carlton BD, Barlett P, Basaran A, Colling K, Osis I, Smith MK. 1986. Reproductive effects of alternative disinfectants. Environ Health Perspect. 69:237–241.
- Catto C, Sabrina S, Ginette CT, Manuel R, Robert T. 2012. Occurrence and spatial and temporal variations of disinfection by-products in the water and air of two indoor swimming pools. IJERPH. 9:2562–2586.
- Chu TS, Cheng SF, Wang GS, Tsai SW. 2013. Occupational exposures of airborne trichloramine at indoor swimming pools in Taipei. Sci Total Environ. 461–462:317–322.
- Cimetière N. 2009. Cimetière N. Étude de la décomposition de la monochloramine en milieu aqueux et réactivité avec des composés phénoliques (thesis). p. 165. France: Université de Poitiers.
- Dang B, Chen L, Mueller C, Dunn KH, Almaguer D, Roberts JL, Otto CS. 2010. Ocular and respiratory symptoms among lifeguards at a hotel indoor waterpark resort. J Occup Environ Med. 52:207–213.
- Daniel FB, Condie LW, Robinson M, Stober JA, York RG, Olson GR, Wang S-R. 1990. Comparative subchronic toxicity studies of three disinfectants. J Am Water Works Assoc. 82:61–69.
- Daniel FB, Ringhand HP, Robinson M, Stober JA, Olson GR, Page NP. 1991. Comparative subchronic toxicity of chlorine and monochloramine in the B6C3F1 mouse. J Am Water Works Assoc. 83:68–75.
- Demange V, Bohadana A, Massin N, Wild P. 2009. Exhaled nitric oxide and airway hyperresponsiveness in workers: a preliminary study in lifeguards. BMC Pulm Med. 9:53.
- Eaton JW, Kolpin CF, Swofford HS, Kjellstrand CM, Jacob HS. 1973. Chlorinated urban water: a cause of dialysis-induced hemolytic anemia. Science. 181:463–464.
- EFSA. 2012. Scientific opinion. Guidance on selected default values to be used by the EFSA Scientific Committee, Scientific Panels and Units in the absence of actual measured data. 32 pp. EFSA Scientific Committee, European Food Safety Authority (EFSA), Parma, Italy. Efsa J. 10:2579.
- Eichelsdörfer D, Slovak J, Dirnagl K, Schmid K. 1975. Irritant effect (conjunctivitis) of chlorine and chloramines in swimming pool water. Vom Wasser. 45:17–28.
- Erkul E, Yaz A, Cingi C, Inancli HM, San T, Bal C. 2014. Effects of indoor swimming pools on the nasal cytology of pool workers. J Laryngol Otol. 128:442–446.
- Exon JH, Koller LD, O'Reilly CA, Peter Bercz J. 1987. Immunotoxicologic evaluation of chlorine-based drinking water disinfectants, sodium hypochlorite and monochloramine. Toxicology. 44:257–269.
- Fantuzzi G, Righi E, Predieri G, Giacobazzi P, Petra B, Aggazzotti G. 2013. Airborne trichloramine (NCl3) levels and self-reported health symptoms in indoor swimming pool workers: dose-response relationships. J Expo Sci Environ Epidemiol. 23:88–93.
- Folkhälsomyndigheten. 2014. Folkhälsomyndighetens allmänna råd om bassängbad. Folkhälsomyndighetens författningssamling FOHMFS 12, p. 4. Östersund (Sweden): Folkhälsomyndigheten, 2014 (in Swedish).
- Font-Ribera L, Kogevinas M, Zock JP, Goméz FP, Barreiro E, Nieuwenhuijsen MJ, Fernandez P, Lourencetti C, Peréz-Olabarria M, Bustamante M, et al. 2010. Short-term changes in respiratory biomarkers after swimming in a chlorinated pool. Environ Health Perspect. 118:1538–1544.
- Font-Ribera L, Kogevinas M, Zock JP, Nieuwenhuijsen MJ, Heederik D, Villanueva CM. 2009. Swimming pool attendance and risk of asthma and allergic symptoms in children. Eur Respir J. 34:1304–1310.
- Font-Ribera L, Marco E, Grimalt JO, Pastor S, Marcos R, Abramsson-Zetterberg L, Pedersen M, Grummt T, Junek R, Barreiro E, et al. 2019. Exposure to disinfection by-products in swimming pools and biomarkers of genotoxicity and respiratory damage – the PISCINA2 Study. Environ Int. 131:104988.
- Font-Ribera L, Villanueva CM, Gracia-Lavedan E, Borras-Santos A, Kogevinas M, Zock JP. 2014. Indoor swimming pool attendance and respiratory and dermal health in schoolchildren-HITEA Catalonia. Respir Med. 108:1056–1059.
- Font-Ribera L, Villanueva CM, Nieuwenhuijsen MJ, Zock JP, Kogevinas M, Henderson J. 2011. Swimming pool attendance, asthma, allergies, and lung function in the Avon Longitudinal Study of Parents and Children cohort. Am J Respir Crit Care Med. 183:582–588.
- Fornander L, Ghafouri B, Lindahl M, Graff P. 2013. Airway irritation among indoor swimming pool personnel: trichloramine exposure, exhaled NO and protein profiling of nasal lavage fluids. Int Arch Occup Environ Health. 86:571–580.
- Gagnaire F, Azim S, Bonnet P, Hecht G, Héry M. 1994. Comparison of the sensory irritation response in mice to chlorine and nitrogen trichloride. J Appl Toxicol. 14:405–409.
- Gapany-Gapanavicius M, Molho M, Tirosh M. 1982. Chloramine-induced pneumonitis from mixing household cleaning agents. Br Med J (Clin Res Ed). 285:1086.
- Gauthier L, Levi Y, Jaylet A. 1989. Evaluation of the clastogenicity of water treated with sodium hypochlorite or monochloramine using a micronucleus test in newt larvae (Pleurodeles waltl). Mutagenesis. 4:170–173.
- Global Initiative for Asthma (GINA). 2018. Global strategy for asthma management and prevention; [accessed January 2019]. https://ginasthma.org/2018-gina-report-global-strategy-for-asthma-management-and-prevention/
- Gomà A, de Lluis R, Roca-Ferrer J, Lafuente J, Picado C. 2017. Respiratory, ocular and skin health in recreational and competitive swimmers: beneficial effect of a new method to reduce chlorine oxidant derivatives. Environ Res. 152:315–321.
- Goodman M, Hays S. 2008. Asthma and swimming: a meta-analysis. J Asthma. 45:639–647.
- Gosselin RE, Smith RP, Hodge HC. 1984. Clinical toxicology of commercial products. 5th ed. Baltimore (MD): Williams and Wilkins; 1984.
- Guo TL, Germolec DR, Collins BJ, Luebke RW, Auttachoat W, Smith MJ, White KL. 2011. Immunotoxicological profile of chloramine in female B6C3F1 mice when administered in the drinking water for 28 days. J Immunotoxicol. 8:381–388.
- Hand VC, Margerum DW. 1983. Kinetics and mechanisms of the decomposition of dichloramine in aqueous solution. Inorg Chem. 22:1449–1456.
- Hansen KM, Albrechtsen HJ, Andersen HR. 2013. Optimal pH in chlorinated swimming pools – balancing formation of by-products. J Water Health. 11:465–472.
- Health Canada. 1995. Chloramines. Guidelines for Canadian drinking water quality – supporting documents. October (edited 1996). Ottawa (ON): Government of Canada Publications; [accessed January 2019]. https://www.canada.ca/content/dam/canada/health-canada/migration/healthy-canadians/publications/healthy-living-vie-saine/water-chloramines-eau/alt/water-chloramines-eau-eng.pdf
- Helenius I, Haahtela T. 2000. Allergy and asthma in elite summer sport athletes. J Allergy Clin Immunol. 106:444–452.
- Helenius IJ, Rytilä P, Metso T, Haahtela T, Venge P, Tikkanen HO. 1998. Respiratory symptoms, bronchial responsiveness, and cellular characteristics of induced sputum in elite swimmers. Allergy. 53:346–352.
- Helenius I, Rytilä P, Sarna S, Lumme A, Helenius M, Remes V, Haahtela T. 2002. Effect of continuing or finishing high-level sports on airway inflammation, bronchial hyperresponsiveness, and asthma: a 5-year prospective follow-up study of 42 highly trained swimmers. J Allergy Clin Immunol. 109:962–968.
- Helse O. 1996. Helse og omsorgsdepartementet. Forskrift for badeanlegg, bassengbad og badstu m.v. Norway: Helse och omsorgsdepartementet. Norwegian; [last updtaed 2015; accessed July 2018]. https://lovdata.no/dokument/SF/forskrift/1996-06-13-592
- Hermans C, Bernard A. 1998. Pneumoproteinaemia: a new perspective in the assessment of lung disorders. Eur Respir J. 11:801–803.
- Hermans C, Bernard A. 1999. Lung epithelium-specific proteins: characteristics and potential applications as markers. Am J Respir Crit Care Med. 159:646–678.
- Hermans C, Dong P, Robin M, Jadoul M, Bernard A, Bersten AD, Doyle IR. 2003. Determinants of serum levels of surfactant proteins A and B and Clara cell protein CC16. Biomarkers. 8:461–471.
- Hermans C, Knoops B, Wiedig M, Arsalane K, Toubeau G, Falmagne P, Bernard A. 1999. Clara cell protein as a marker of Clara cell damage and bronchoalveolar blood barrier permeability. Eur Respir J. 13:1014–1021.
- Herren-Freund SL, Pereira MA. 1986. Carcinogenicity of by-products of disinfection in mouse and rat liver. Environ Health Perspect. 69:59–65.
- Héry M, Gerber JM, Hecht G, Subra I, Possoz C, Aubert S, Dieudonne M, Andre JC. 1998. Exposure to chloramines in a green salad processing plant. Ann Occup Hyg. 42:437–451.
- Héry M, Hecht G, Gerber JM, Gendre JC, Hubert G, Rebuffaud J. 1995. Exposure to chloramines in the atmosphere of indoor swimming pools. Ann Occup Hyg. 39:427–439.
- Héry M, Hecht G, Gerber JM, Hubert G, Subra IAS, Gerardin F, Dorotte M. 1999. Occupational exposure during cleaning and disinfection in the food industry. Occup Hyg. 5:131–144.
- Holleman AF, Wiberg E, Wiberg N. 2007. Lehrbuch der anorganischen Chemie, 102 ed. Berlin, Germany: De Gruyter; p. 694–701.
- Holzwarth G, Balmer RG, Soni L. 1984. The fate of chlorine and chloramines in cooling towers: Henry’s law constants for flashoff. Water Res. 18:1421–1427.
- IARC. 2004. Chloramine. In: Monographs on the evaluation of carcinogenic risks to humans Vol. 84. Some drinking-water disinfectants and contaminants, including arsenic. Lyon (France): International Agency for Research on Cancer; p. 295–315.
- INRS. 2017. Trichlorure d’azote et autres composés chlorés M-104. Métropol 11/2017. The French National Research and Safety Institute for the Prevention of Occupational Accidents and Diseases (INRS); p. 8; [accessed January 2019]. http://www.inrs.fr/publications/bdd/metropol/fiche.html?refINRS=METROPOL_104
- INRS. 2011. Triklorame, kit de mesure de la trichloramine dans l’air. Contrôle de l’air des piscines et dans l’agroalimentaire. The French National Research and Safety Institute for the Prevention of Occupational Accidents and Diseases (INRS); [accessed August 2018]. http://www.inrs.fr/services/innovation/evaluation-diagnostic/triklorame.html
- Jacangelo JG, Olivieri VP, Kawata K. 1987. Oxidation of sulfhydryl groups by monochloramine. Water Res. 21:1339–1344.
- Jacobs JH, Fuertes E, Krop EJ, Spithoven J, Tromp P, Heederik DJ. 2012. Swimming pool attendance and respiratory symptoms and allergies among Dutch children. Occup Environ Med. 69:823–830.
- Jacobs JH, Spaan S, van Rooy GB, Meliefste C, Zaat VA, Rooyackers JM, Heederik D. 2007. Exposure to trichloramine and respiratory symptoms in indoor swimming pool workers. Eur Respir J. 29:690–698.
- Kharitonov SA, Barnes PJ. 2000. Clinical aspects of exhaled nitric oxide. Eur Respir J. 16:781–792.
- Kinani S, Richard B, Souissi Y, Bouchonnet S. 2012. Analysis of inorganic chloramines in water. Trends Analyt Chem. 33:55–67.
- King BS, Page EH, Mueller CA, Dollberg DD, Gomez KE, Warren AM. 2006. Eye and respiratory symptoms in poultry processing workers exposed to chlorine by-products. Am J Ind Med. 49:119–126.
- Kjellstrand CM, Eaton JW, Yawata Y, Swofford H, Kolpin CF, Buselmeier TJ, von Hartitzsch B, Jacob HS. 1974. Hemolysis in dialized patients caused by chloramines. Nephron. 13:427–433.
- Lévesque B, Duchesne JF, Gingras S, Lavoie R, Prud’Homme D, Bernard E, Boulet LP, Ernst P. 2006. The determinants of prevalence of health complaints among young competitive swimmers. Int Arch Occup Environ Health. 80:32–39.
- Lévesque B, Vézina L, Gauvin D, Leroux P. 2015. Investigation of air quality problems in an indoor swimming pool: a case study. ANNHYG. 59:1085–1089.
- Llana-Belloch S, Quesada JIP, Pérez-Soriano P, Lucas-Cuevas ÁG, Salvador-Pascual A, Olaso-González G, Moliner-Martinez Y, Verdú-Andres J, Campins-Falco P, Gómez-Cabrera C. 2016. Disinfection by-products effect on swimmers oxidative stress and respiratory damage. Eur J Sport Sci. 16:609–617.
- Löfstedt H, Westerlund J, Graff P, Bryngelsson IL, Mölleby G, Olin AC, Eriksson K, Westberg H. 2016. Respiratory and ocular symptoms among employees at Swedish indoor swimming pools. J Occup Environ Med. 58:1190–1195.
- Lubbers JR, Chauan S, Bianchine JR. 1982. Controlled clinical evaluations of chlorine dioxide, chlorite and chlorate in man. Environ Health Perspect. 46:57–62.
- Manasfi T, Coulomb B, Boudenne JL. 2017. Occurrence, origin, and toxicity of disinfection byproducts in chlorinated swimming pools: an overview. Int J Hyg Environ Health. 220:591–603.
- Massin N, Bohadana AB, Wild P, Héry M, Toamain JP, Hubert G. 1998. Respiratory symptoms and bronchial responsiveness in lifeguards exposed to nitrogen trichloride in indoor swimming pools. Occup Environ Med. 55:258–263.
- Massin N, Hecht G, Ambroise D, Héry M, Toamain JP, Hubert G, Dorotte M, Bianchi B. 2007. Respiratory symptoms and bronchial responsiveness among cleaning and disinfecting workers in the food industry. Occup Environ Med. 64:75–81.
- Meier JR, Bull RJ, Stober JA, Cimino MC. 1985. Evaluation of chemicals used for drinking water disinfection for production of chromosomal damage and sperm-head abnormalities in mice. Environ Mutagen. 7:201–211.
- Miller RG, Kopfler FC, Condie LW, Pereira MA, Meier JR, Ringhand HP, Robinson M, Casto BC. 1986. Results of toxicological testing of Jefferson Parish pilot plant samples. Environ Health Perspect. 69:129–139.
- Ministry of Social Affairs and Health. 2018. HTP-värden 2018. Koncentrationer som befunnits skadliga. Helsinki (Finland): Social- och Hälsovårdsministeriets publikationer 10. Swedish; [accessed March 2019]. http://urn.fi/URN:ISBN:978-952-00-3946-2
- Moore GS, Calabrese EJ, McGee M. 1980. Health effects of monochloramines in drinking water. J Environ Sci Health Part A Environ Sci Eng. 15:239–258.
- Mountjoy M, Fitch K, Boulet LP, Bougault V, van Mechelen W, Verhagen E. 2015. Prevalence and characteristics of asthma in the aquatic disciplines. J Allergy Clin Immunol. 136:588–594.
- Musch MW, Sugi K, Straus D, Chang EB. 1999. Heat-shock protein 72 protects against oxidant-induced injury of barrier function of human colonic epithelial Caco2/bbe cells. Gastroenterology. 117:115–122.
- Nakai JS, Poon R, Lecavalier P, Chu I, Yagminas A, Valli VE. 2000. Effects of subchronic exposure of rats to dichloramine and trichloramine in drinking water. Regul Toxicol Pharmacol. 31:200–209.
- Nakamura TY, Yamamoto I, Nishitani H, Matozaki T, Suzuki T, Wakabayashi S, Shigekawa M, Goshima K. 1995. Detachment of cultured cells from the substratum induced by the neutrophil-derived oxidant NH2Cl: synergistic role of phosphotyrosine and intracellular Ca2+ concentration. J Cell Biol. 131:509–524.
- Naturstyrelsen. 2013. Vejledning om kontrol med svømmebade. København (Denmark): Naturstyrelsen, Miljøministeriet; p. 100. Danish.
- Nielsen GD, Wolkoff P, Alarie Y. 2007. Sensory irritation: risk assessment approaches. Regul Toxicol Pharmacol. 48:6–18.
- NIOSH. 2008. Health hazard evaluation report. Investigation of employee symptoms at an indoor water park, Mason, Ohio. HETA 2007-0163-3062. Cincinnati (OH): US Department of Health and Human Services, Public Health Service, Centers for Disease Control and Prevention, National Institute for Occupational Safety and Health; p. 53.
- NLM. 2018. PubChem Compound Database. Bethesda (MD): National Center for Biotechnology Information, US National Library of Medicine; [assessed September 2018]. https://www.ncbi.nlm.nih.gov/pccompound/
- Nordberg GF, Lundström NG, Forsberg B, Hagenbjörk-Gustafsson A, Lagerkvist BJ, Nilsson J, Svensson M, Blomberg A, Nilsson L, Bernard A, et al. 2012. Lung function in volunteers before and after exposure to trichloramine in indoor pool environments and asthma in a cohort of pool workers. BMJ Open. 2:e000973.
- NTP. 1992. Toxicology and carcinogenesis studies of chlorinated water (CAS Nos. 7782-50-5 and 7681-52-9) and chloraminated water (CAS No. 10599-90-3) (deionized and charcoal-filtered) in F344/N rats and B6C3F1 mice (drinking water studies). Natl Toxicol Program Tech Rep Ser. 392:1–466.
- Okada K, Akiyoshi M, Ishizaki K, Sato H, Matsunaga T. 2014. Analysis of an explosion accident of nitrogen trichloride in a waste liquid containing ammonium ion and platinum black. J Hazard Mater. 278:75–81.
- Päivinen MK, Keskinen KL, Tikkanen HO. 2010. Swimming and asthma: factors underlying respiratory symptoms in competitive swimmers. Clin Respir J. 4:97–103.
- Parrat J, Donzé G, Iseli C, Perret D, Tomicic C, Schenk O. 2012. Assessment of occupational and public exposure to trichloramine in Swiss indoor swimming pools: a proposal for an occupational exposure limit. Ann Occup Hyg. 56:264–277.
- Pedersen L, Lund TK, Barnes PJ, Kharitonov SA, Backer V. 2008. Airway responsiveness and inflammation in adolescent elite swimmers. J Allergy Clin Immunol. 122:322–327.
- Piva TJ, Newsholme EA, Goldstein L. 1991. Inhibition by monochloramine of the transport of glutamine and glucose in HeLa cells and lymphocytes. Int J Biochem. 23:1421–1426.
- Poon R, Lecavalier P, Tryphonas H, Bondy G, Chen M, Chu I, Yagminas A, Valli VE, D'amour M, Thomas B. 1997. Effects of subchronic exposure of monochloramine in drinking water on male rats. Regul Toxicol Pharmacol. 25:166–175.
- Predieri G, Giacobazzi P. 2012. Determination of nitrogen trichloride (NCl3) levels in the air of indoor chlorinated swimming pools: an impinger method proposal. Int J Environ Anal Chem. 92:645–654.
- Ricciardolo FL, Sorbello V, Ciprandi G. 2015. FeNO as biomarker for asthma phenotyping and management. Allergy Asthma Proc. 36:e1–e8.
- Richardson SD, DeMarini DM, Kogevinas M, Fernandez P, Marco E, Lourencetti C, Ballesté C, Heederik D, Meliefste K, McKague AB, et al. 2010. What’s in the pool? A comprehensive identification of disinfection by-products and assessment of mutagenicity of chlorinated and brominated swimming pool water. Environ Health Perspect. 118:1523–1530.
- Richardson SD, Plewa MJ, Wagner ED, Schoeny R, Demarini DM. 2007. Occurrence, genotoxicity, and carcinogenicity of regulated and emerging disinfection by-products in drinking water: a review and roadmap for research. Mutat Res. 636:178–242.
- Robinson M, Bull RJ, Schamer M, Long RE. 1986. Epidermal hyperplasia in mouse skin following treatment with alternative drinking water disinfectants. Environ Health Perspect. 69:293–300.
- Romberg K, Tufvesson E, Bjermer L. 2012. Asthma is more prevalent in elite swimming adolescents despite better mental and physical health. Scand J Med Sci Sports. 22:362–371.
- Rosenman KD, Millerick-May M, Reilly MJ, Flattery J, Weinberg J, Harrison R, Lumia M, Stephens AC, Borjan M. 2015. Swimming facilities and work-related asthma. J Asthma. 52:52–58.
- Sanderson WT, Weber A, Echt A. 1995. Case reports: epidemic eye and upper respiratory irritation in poultry processing plants. Appl Occup Environ Hyg. 10:43–49.
- Sandrini A, Taylor DR, Thomas PS, Yates DH. 2010. Fractional exhaled nitric oxide in asthma: an update. Respirology. 15:57–70.
- Schmalz C, Frimmel FH, Zwiener C. 2011. Trichloramine in swimming pools--formation and mass transfer. Water Res. 45:2681–2690.
- Schmalz C, Wunderlich HG, Heinze R, Frimmel FH, Zwiener C, Grummt T. 2011. Application of an optimized system for the well-defined exposure of human lung cells to trichloramine and indoor pool air. J Water Health. 9:586–596.
- Senthilmohan ST, Kettle AJ, McEwan MJ, Dummer J, Edwards SJ, Wilson PF, Epton MJ. 2008. Detection of monobromamine, monochloramine and dichloramine using selected ion flow tube mass spectrometry and their relevance as breath markers. Rapid Commun Mass Spectrom. 22:677–681.
- Seys SF, Feyen L, Keirsbilck S, Adams E, Dupont LJ, Nemery B. 2015. An outbreak of swimming-pool related respiratory symptoms: an elusive source of trichloramine in a municipal indoor swimming pool. Int J Hyg Environ Health. 218:386–391.
- Shaw CA, Bains JS. 1998. Did consumption of flour bleached by the agene process contribute to the incidence of neurological disease? Med Hypotheses. 51:477–481.
- Shibata H, Sakamoto Y, Oka M, Kono Y. 1999. Natural antioxidant, chlorogenic acid, protects against DNA breakage caused by monochloramine. Biosci Biotechnol Biochem. 63:1295–1297.
- Shih KL, Lederberg J. 1976. Chloramine mutagenesis in Bacillus subtilis. Science. 192:1141–1143.
- SIA. 2011. Wasser und Wasseraufbereitungsanlagen in Gemeinschaftsbädern. Anforderungen und ergänzende Bestimmungen für Bau und Betrieb. Schweizer Norm SN 546 385/9. Zürich (Switzerland): Schweizerischer Ingenieur- und Architektenverein; p. 68.
- SPIN database. 2018. Substances in Preparations in Nordic Countries; [accessed October]. http://spin2000.net/
- Suffet IH, Mallevialle J, Kawczynski E. 1995. AWWA Research Foundation, Lyonnaise des eaux. Advances in taste-and-odor treatment and control. Denver, CO: American Water Works Association Research Foundation; p. 385.
- Suzuki H, Mori M, Suzuki M, Sakurai K, Miura S, Ishii H. 1997. Extensive DNA damage induced by monochloramine in gastric cells. Cancer Lett. 115:243–248.
- Syclope Electronique. 2019. Catalogue 2019. La bonne expertise de l’eau. Equipements de contrôle portatif: trichloramine dans l’air. Sauvagnon (France): Syclope Electronique; [accessed April 2019]. https://www.syclope.fr/wp-content/uploads/2019/01/Catalogue-France-2019-r.pdf
- Tanen DA, Graeme KA, Raschke R. 1999. Severe lung injury after exposure to chloramine gas from household cleaners. N Engl J Med. 341:848–849.
- Tatsumi T, Fliss H. 1994. Hypochlorous acid and chloramines increase endothelial permeability: possible involvement of cellular zinc. Am J Physiol. 267:H1597–H1607.
- Thickett KM, McCoach JS, Gerber JM, Sadhra S, Burge PS. 2002. Occupational asthma caused by chloramines in indoor swimming-pool air. Eur Respir J. 19:827–832.
- Thomas EL, Jefferson MM, Bennet JJ, Learn DB. 1987. Mutagenic activity of chloramines. Mutat Res. 188:35–43.
- Tipple MA, Shusterman N, Bland LA, McCarthy MA, Favero MS, Arduino MJ, Reid MH, Jarvis WR. 1991. Illness in hemodialysis patients after exposure to chloramine contaminated dialysate. ASAIO Trans. 37:588–591.
- [US EPA] US Environmental Protection Agency. 1994. Drinking water criteria document for chloramines. Final draft ECAO-CIN-D002. March 1994. Washington (DC): US Environmental Protection Agency, Health and Ecological Criteria Division, Office of Science and Technology, Office of Water.
- Valkeinen R, Kalliokoski P, Päivinen M, Patovirta R-L, Putus T, Jauhiainen T, Reiman M, Rautiala S, Rantio T, Mäkinen M, et al. 2007. Uimahallin allastilojen työolosuhteet ja henkilökunnan hengityselinoireet [Working conditions and workers’ respiratory symptoms at public swimming pools]. Kuopio (Finland): University of Kuopio, Department of Environmental Sciences; [accessed May 2017]. Finnish. https://www.tsr.fi/tsarchive/files/TietokantaTutkittu/2006/106056Loppuraportti.pdf
- Valvira. 2017. Anvisning för tillämpning av bassängvattenförordningen. Anvisning 2/2017. Helsinki (Finland): Valvira, Tillstånds- och tillsynsverket för social- och hälsovården; p. 89; [accessed July 2018]. Swedish. http://www.valvira.fi/documents/18502/315832/Allasvesiasetuksen_soveltamisohje_ruotsiksi.pdf/969b36ed-cca7-aee9-6f00-043aa26babae
- Weast RC, Astle MJ, eds. 1980. CRC handbook of chemistry and physics. 60th ed. Boca Raton (FL): CRC Press.
- Weng S-C, Weaver WA, Zare Afifi M, Blatchley TN, Cramer JS, Chen J, Blatchley ER. 2011. Dynamics of gas-phase trichloramine (NCl3) in chlorinated, indoor swimming pool facilities. Indoor Air. 21:391–399.
- Westerlund J, Bryngelsson IL, Löfstedt H, Eriksson K, Westberg H, Graff P. 2019. Occupational exposure to trichloramine and trihalomethanes: adverse health effects among personnel in habilitation and rehabilitation swimming pools. J Occup Environ Hyg. 16:78–88.
- Westerlund J, Graff P, Bryngelsson IL, Westberg H, Eriksson K, Löfstedt H. 2015. Occupational exposure to trichloramine and trihalomethanes in Swedish indoor swimming pools: evaluation of personal and stationary monitoring. ANNHYG. 59:1074–1084.
- WHO. 2006. Guidelines for safe recreational water environments. Vol. 2. Swimming pools and similar environments. Geneva (Switzerland): World Health Organization. Chapter 4, Chemical hazards.
- WHO. 2011. Guidelines for drinking-water quality, 4th ed. Geneva (Switzerland): World Health Organization; p. 541.
- Wojtowicz JA. 1993. Chloramines and bromamines. In: Kroschwitz JI, Howe-Grant M, editors. Kirk-Othmer encyclopedia of chemical technology. Vol. 5, 4th ed. New York: John Wiley & Sons; p. 911–933.
- Wones RG, Deck CC, Stadler B, Roark S, Hogg E, Frohman LA. 1993. Effects of drinking water monochloramine on lipid and thyroid metabolism in healthy men. Environ Health Perspect. 99:369–374.
- Working Group on Indoor Guide Values of the Federal Environment Agency and the States’ Health Authorities. 2011. Risk assessment of trichloramine in the air of indoor swimming pools. Bundesgesundheitsblatt Gesundheitsforschung Gesundheitsschutz. 54:997–1004. German.
- Zierler S, Danley RA, Feingold L. 1986. Type of disinfectant in drinking water and patterns of mortality in Massachusetts. Environ Health Perspect. 69:275–279.
- Zierler S, Feingold L, Danley RA, Craun G. 1988. Bladder cancer in Massachusetts related to chlorinated and chloraminated drinking water: a case-control study. Arch Environ Health. 43:195–200.