Abstract
The inhalation of dust containing certain nickel compounds has been associated with an increased risk of lung and nasal cancers in occupational studies of workers who process or refine sulfidic nickel ores and are exposed to relatively high levels of mixtures of water-soluble, sulfidic, oxidic, and/or metallic forms of nickel. We conducted a systematic review of the potential carcinogenicity of metallic nickel, focusing on cancers of the respiratory tract. We evaluated the quality and risk of bias (RoB) of the relevant epidemiology, experimental animal, and in vitro mechanistic studies using the National Toxicology Program's Office of Health Assessment and Translation (OHAT) RoB Rating Tool. We then used a systematic review protocol based on the OHAT approach to critically assess whether metallic nickel should be considered a human respiratory carcinogen. Our evaluation of the epidemiology studies indicates that there is no substantive evidence of increased respiratory cancer risk in workers exposed predominantly to metallic nickel. Animal evidence indicates that metallic nickel does not increase the incidence of respiratory tumors in rodents exposed by inhalation. The in vitro studies are limited in value, as they bypass normal clearance mechanisms. Nevertheless, the mechanistic evidence indicates that metallic nickel is not mutagenic but can induce DNA strand breaks under certain conditions. Based on a standard framework for assessing causality, we conclude that the evidence does not support a causal relationship between metallic nickel exposure and respiratory cancer in humans.
Introduction
Nickel is a metal that is naturally found in soils, sediments, water, and air. Various forms of nickel can be extracted from sulfidic and lateritic nickel ores through nickel smelting and refining processes. These processes contribute sulfidic and oxidic nickel compounds, as well as water-soluble nickel compounds (i.e. nickel salts such as nickel sulfate hexahydrate and nickel chloride hexahydrate) and nickel metal (Ni) to the air.
The inhalation of dust containing certain nickel compounds has been associated with an increased risk of lung and nasal cancers in occupational studies of workers who processed or refined sulfidic (but not lateritic) nickel ores (ATSDR Citation2005; Goodman et al. Citation2009; IARC Citation2012; Buxton et al. Citation2019). Many of these workers were exposed to high concentrations of mixtures of sulfidic, oxidic, water-soluble, and metallic forms of nickel, primarily by inhalation, with some potential for dermal contact or ingestion of dusts (ATSDR Citation2005; NTP Citation2016). By contrast, the general population is exposed to nickel primarily through the diet, with a typical intake of 70–250 μg Ni/day, as nickel is naturally present in air, soil, water, plants, and foods (predominantly as complex oxidic forms in soil, an ionic form of Ni in water, and complex organic molecules containing Ni in plants and food) (ATSDR Citation2005; IARC Citation2012; De Brouwere et al. Citation2012; NTP Citation2016; Buxton et al. Citation2019). Inhalation of nickel from the ambient air, which is typically present in the ng/m3 range (Buekers et al. Citation2015) and includes primarily oxidic and soluble forms of nickel, contributes less than 1% to aggregated nickel exposure from all sources, and, thus, is considered a minor route of exposure for the general population (De Brouwere et al. Citation2012; IARC Citation2012), whose overall exposure to nickel from all potential sources is generally low (ATSDR Citation2005; De Brouwere et al. Citation2012; NTP Citation2016).
Metallic nickel includes pure nickel metal as well as nickel alloys, such as stainless steel. Metallic nickel is used in electroplating, batteries, coins, welding products, electrical components, catalysts, magnets, machinery parts, and medical devices (ATSDR Citation2005; IARC Citation2012; NTP Citation2016). The general population is exposed to metallic nickel primarily through dermal contact with stainless steel, coins, and jewelry (NTP Citation2016). The most comprehensive epidemiology study of cancer risks associated with occupational exposure to metallic nickel and nickel compounds, conducted by the International Committee on Nickel Carcinogenesis in Man (ICNCM) over 30 years ago, concluded that metallic nickel was not associated with an increased risk of lung and nasal cancers (ICNCM Citation1990). Cross-classification analyses of some of the nickel refinery studies have suggested that the increased cancer risks in the refinery cohorts were associated with exposure to sulfidic, and possibly oxidic, nickel, with an indication that water-soluble nickel exposure accentuated these risks but that there was no evidence to suggest that metallic nickel exposure increased lung cancer risk (ICNCM Citation1990). In addition, inhalation bioassays in rodents reported increased incidence of respiratory tract cancers with oxidic and sulfidic nickel compounds, but not with metallic nickel (as reviewed by Goodman et al. Citation2009, Citation2011; IARC Citation2012). Cancer bioassays in rodents with inhalation or oral exposure to water-soluble nickel compounds have also been negative (Goodman et al. Citation2009, Citation2011; IARC Citation2012).
The carcinogenic hazard of metallic nickel and nickel compounds has been assessed by several scientific agencies. The International Agency for Research on Cancer (IARC) classified metallic nickel as possibly carcinogenic to humans (Group 2B), based on inadequate evidence in humans for the carcinogenicity of nickel metal and nickel alloys, and sufficient evidence in experimental animals for the carcinogenicity of metallic nickel (IARC Citation1990). It is noteworthy that all of the metallic nickel studies in experimental animals that form the basis of this classification used exposure routes that are not relevant to human exposures (i.e. intratracheal instillation; subcutaneous implantation; and intrapleural, intramuscular, intraperitoneal, intravenous, intrarenal, subperiosteal, and intramedullary injection) and reported local tumors at the administration site. IARC classified nickel compounds as carcinogenic to humans (Group 1), based on sufficient evidence in humans for cancers of the lung, nasal cavity, and paranasal sinuses with exposure to mixtures that include nickel compounds and nickel metal, as well as sufficient evidence for carcinogenicity in experimental animals for nickel compounds and nickel metal (IARC Citation2012).
The National Toxicology Program has classified nickel compounds as “known to be human carcinogens” and metallic nickel as “reasonably anticipated to be a human carcinogen,” with the latter based on what it considered to be sufficient evidence from studies in experimental animals (NTP Citation2002). The experimental animal studies relied on for the classification of metallic nickel include the same studies that used non-relevant exposure routes that were relied on for the IARC classification, as this evaluation was conducted prior to the publication of an inhalation bioassay for metallic nickel (Oller et al. Citation2008).
The American Conference of Governmental Industrial Hygienists (ACGIH) has classified insoluble nickel compounds as “A1: Confirmed human carcinogens,” water-soluble nickel compounds as “A4: Not classifiable as human carcinogens,” and metallic nickel as "A5: Not suspected as a human carcinogen" (ACGIH Citation2001). The United States Environmental Protection Agency (US EPA) has not evaluated the potential carcinogenicity of metallic nickel or water-soluble nickel compounds, but has classified both nickel subsulfide and nickel refinery dust as "A; human carcinogen" (US EPA Citation2002, Citation2006).
In the European Union, under the Classification, Labeling and Packaging (CLP) Regulation, nickel compounds are classified as Category 1; H350i (known human carcinogens via inhalation), as the tumors that serve as the basis for their classification occurred only in the respiratory tract and only after inhalation exposure (ECHA Citation2018). Nickel metal carries a classification as Category 2; H351, indicating it is a suspect carcinogen (ECHA Citation2019). The opinion of the Committee for Risk Assessment (RAC) of the European Chemicals Agency on nickel and nickel compounds indicates that neither the epidemiology nor experimental animal data provide support for carcinogenicity of metallic nickel (ECHA Citation2018).
To our knowledge, there has been no comprehensive review of the potential carcinogenicity of metallic nickel published in the peer-reviewed literature since 2005 (Sivulka Citation2005). Below, we conduct a systematic review of metallic nickel carcinogenicity. We focus on cancers of the respiratory tract, as these are the basis for most of the carcinogenicity classifications discussed above. In addition, there is tumor site concordance in the respiratory tract between humans and experimental animals for carcinogenic nickel compounds (Krewski et al. Citation2019). First, we briefly describe the physical and chemical properties, toxicokinetics, and bioavailability of metallic nickel, followed by a systematic review of the epidemiology, experimental animal, and in vitro mechanistic evidence to assess whether metallic nickel can be considered a human respiratory carcinogen.
Methods
Literature searches and study selection
The principal question of our evaluation is whether the available evidence supports metallic nickel as a human respiratory carcinogen. We conducted separate literature searches for epidemiology, experimental animal, and mechanistic/in vitro studies published through 1 June 2020, using the PubMed and Scopus databases. The search terms we used for identifying studies from each database are provided in the Supplemental materials. We also reviewed the reference lists of relevant reviews to identify additional studies.
We included studies in the English language that evaluated carcinogenic, mutagenic, genotoxic, or cellular transformation effects of metallic nickel in humans, experimental animals, or in vitro test systems. For completeness, we included experimental animal studies that used an exposure route with administration into the respiratory tract, such as inhalation, intratracheal instillation, or injection into the lungs, even though the latter two routes are of limited relevance to human exposure scenarios. We excluded studies that were not published in English; studies that evaluated other forms of nickel but did not include any exposure to metallic nickel; human or experimental animal studies that did not evaluate respiratory cancers (such as cancers of the lung, bronchus, larynx, nose, and sinuses); review articles that did not include new analyses or unpublished evidence; in vivo studies that did not use an exposure route with administration into the respiratory tract; and mechanistic studies that evaluated metallic nickel nanoparticles but not micron-sized particles or nanoparticles that agglomerated into particles in the micron range (as discussed below).
Study quality criteria
We developed criteria to evaluate study quality and risk of bias (RoB) consistently across each realm of evidence (epidemiology, experimental animal, and in vitro), based on the NTP RoB Rating Tool (NTP Citation2015). This tool was created to aid in the assessment of an individual study's internal validity (i.e. whether the design and conduct of the study compromised the credibility of any reported link between exposure and outcome). This tool does not take into consideration the potential for random error in the study results. Instead, random error is captured in the sample size and 95% confidence interval (CI) of each study, as presented in our tabular summaries of the study results. The RoB Rating Tool uses a set of questions with detailed criteria that define the aspects of study design, conduct, and reporting that are used to assign a study quality/RoB rating for each question. We modified the questions and criteria to be relevant to the evaluation of studies of respiratory cancers. The questions and criteria are specific enough that if different investigators applied them to the studies reviewed here, it is expected that they would assign the same study quality/RoB ratings as we did to each study.
Epidemiology studies
The study quality/RoB questions for epidemiology studies were divided into three key domains (exposure characterization, outcome assessment, and confounding), as well as four other RoB domains (attrition bias, statistical methods, temporality, and selective reporting bias). The specific questions and criteria for each of the seven domains for epidemiology studies are presented in Supplemental Table S1.
Although the study quality/RoB criteria are based on the criteria listed in the NTP RoB Rating Tool, a few details regarding our specific criteria are worth noting. We considered smoking, other occupational co-exposures, and nonmetallic forms of nickel as important confounders, in addition to sociodemographic characteristics. Smoking and some occupational co-exposures (e.g. asbestos, chromium, wood dust) are known risk factors for respiratory cancer that also tend to have a different distribution between comparison groups (e.g. workers versus general population, lung cancer patients versus general population) (Brackbill et al. Citation1988; Cataldo et al. Citation2010; Malhotra et al. Citation2016). The use of local (such as county) cancer rates instead of national rates for comparison to workers better reflects the socioeconomic/lifestyle choices (e.g. smoking rates) of worker populations. The difference in distribution of these factors could explain observed differences in the outcomes even without nickel exposure; therefore, these risk factors should be accounted for when examining the association of nickel exposure with respiratory cancers. When studies stratified their analyses (or we restricted our analysis of the study) to workers within specific departments with metallic nickel exposure, we considered this as controlling for confounding by occupational co-exposures.
In many studies, nonmetallic forms of nickel (e.g. water-soluble, sulfidic, oxidic forms of nickel), whether measured or not, coexisted with metallic nickel and thus may confound the association between metallic nickel and respiratory cancer. Although the specific evaluation of metallic nickel exposure may not have been the focus of all the studies included in this review, we evaluated the adjustment of nonmetallic forms of nickel for the purpose of understanding the specific association between metallic nickel and respiratory cancer. We considered analyses of cohorts predominantly exposed to metallic nickel compared to other forms of nickel as being adjusted for nonmetallic forms of nickel.
In addition, for temporality, we considered a longer lag time (>10 years) to be more informative than a shorter (e.g. <2-year) or no lag time. Given that respiratory cancer is associated with a long latency period, exposure immediately before cancer incidence or mortality is reported is not etiologically relevant. There could also be a delay in cancer detection, in which case exposure within a certain time period before diagnosis actually occurred after the true cancer onset. In other words, a reasonable, longer lag time helps ensure temporality between exposure and cancer outcomes.
Experimental animal studies
The study quality/RoB criteria for experimental animal carcinogenicity studies include the key domains of exposure characterization, outcome assessment, and adequate number of animals, as well as five other study quality/RoB domains (randomization, experimental conditions, blinding, attrition bias, and statistical methods). Although these criteria are based on the RoB questions and domains for experimental animal studies in the NTP RoB Rating Tool (NTP Citation2015), we added a criterion for adequate number of animals based on Organization for Economic Co-operation and Development (OECD) guidelines for carcinogenicity bioassays (OECD Citation2018). We also removed the criteria for concealed allocation to study groups, as we included this in our criteria for blinding, and we removed the criteria regarding whether all measured outcomes were reported, as this was included in our evaluation of whether outcome data were complete. The specific questions and criteria for each of the eight domains for experimental animal studies are presented in Supplemental Table S2.
In vitro studies
NTP does not specify RoB criteria for in vitro studies in its RoB Rating Tool, but the NTP Handbook for Conducting a Literature-based Health Assessment Using OHAT Approach for Systematic Review and Evidence Integration (herein, the "OHAT Handbook"; NTP Citation2019) lists key data elements to extract from in vitro studies for systematic reviews, including elements that can be used to assess internal validity/RoB. We based our study quality/RoB criteria for in vitro genotoxicity/mutagenicity studies on these elements, as well as the study quality criteria for in vitro studies presented by Lynch et al. (Citation2016), the SciRAP Tool (Stockholm University, Karolinska Institutet Citation2019), and US EPA in its Application of Systematic Review in TSCA Risk Evaluations (US EPA Citation2018). The criteria for in vitro studies include the key domains of exposure characterization, outcome assessment, and control groups, as well as five other study quality/RoB domains (replicates, experimental conditions, blinding, complete data, and statistical methods). The specific questions and criteria for each of the eight domains for in vitro studies are presented in Supplemental Table S3.
Study quality/risk of bias ratings
We used the criteria discussed above to assign study quality/RoB ratings to each of the epidemiology, experimental animal, and in vitro studies for each of their respective domains; study relevance is assessed during the evidence integration process (Goodman et al. Citation2020). We then used the guidance from the OHAT Handbook (NTP Citation2019) for dividing studies into three tiers of study quality based on their study quality/RoB ratings across domains. In this approach, studies are divided into tiers of decreasing quality/increasing RoB as follows:
Tier 1 – A study must be rated as "definitely low" or "probably low" RoB for all key domains for that study type AND have most (i.e. at least half) other RoB domains as "definitely low" or "probably low."
Tier 2 – Study does not meet the criteria for Tier 1 or Tier 3.
Tier 3 – A study must be rated as "definitely high" or "probably high" RoB for all key domains for that study type AND have most (i.e. at least half) other RoB domains as "definitely high" or "probably high."
Although the overall tiers are only a crude measure of quality/RoB (as a study may have low RoB based on some criteria but high RoB based on others), studies categorized into Tier 3 have a high concern for bias and therefore are of low quality and reliability (NTP Citation2019). Consistent with the guidance in the OHAT Handbook (NTP Citation2019), we did not consider the results of Tier 3 studies in the integration of evidence.
Evidence integration
We assessed the results of the studies within each realm in the context of their methodological strengths and limitations, as determined from the study quality/RoB analysis. We then integrated the evidence both within and across the epidemiology and experimental realms. lists the criteria we considered for evaluating whether the results of experimental studies (in vivo and in vitro) support causation, and for evaluating their human relevance. These criteria are based on the International Program on Chemical Safety (IPCS) Mode-of-Action/Human Relevance framework (Boobis et al. Citation2008; Meek et al. Citation2014) and the NTP OHAT framework for evaluating confidence in a body of literature (NTP Citation2019). Although the IPCS framework was intended to evaluate key events from mechanistic studies, we modified the framework so it can be used to evaluate toxicity studies that assess apical effects, such as cancer, and whether the reported effects (or a lack thereof) are consistent and coherent with key events identified in mechanistic studies (Goodman et al. Citation2020). Our modified criteria are similar to those that NTP OHAT uses to evaluate confidence in the evidence presented in a body of literature (i.e. RoB, unexplained inconsistency, indirectness, imprecision, magnitude, dose-response, and consistency across models/species/related outcomes). We incorporated all of these criteria, although RoB was assessed in the individual studies during the initial evaluation of study quality.
Table 1. Criteria for evaluating causation and the human relevance of experimental studies.
If all the criteria listed in for assessing the body of experimental studies for causation were met, it would be difficult to imagine a situation in which there is not causation, so we concluded that these experimental studies demonstrate causation (though not necessarily relevance) . If not all of the criteria were met, then we determined whether it is more likely that the evidence from these studies supports causation, supports no causation, or is inadequate to determine causation. We also evaluated the human relevance of results of experimental studies in a similar manner, depending on the extent to which the criteria for human relevance in were met.
We then used the evaluation of causation and human relevance in experimental studies to assess whether there is high, moderate, or inadequate confidence in the biological plausibility of metallic nickel exposure causing respiratory cancer in humans, or high or moderate confidence in a lack of biological plausibility, using the scheme outlined in . If the body of experimental evidence demonstrated causation and human relevance, then we concluded that there is high confidence that metallic nickel exposure causing respiratory cancer in humans is biologically plausible. If there was inadequate evidence regarding human relevance, but the experimental study results supported causation, we concluded that there is moderate confidence that exposure to metallic nickel causing respiratory cancer in humans is biologically plausible. If the experimental study results indicate that the relationship between metallic nickel exposure and respiratory cancer is not causal and there is either evidence in support of human relevance or inadequate evidence to determine human relevance, we concluded that there is either high or moderate confidence (respectively) that metallic nickel exposure causing respiratory cancer in humans is not biologically plausible. In all other cases, we concluded that there is inadequate evidence to assess biological plausibility.
Table 2. Confidence in the biological plausibility of a health outcome in humans based on experimental studies.
We integrated the results of the biological plausibility assessment with those of the epidemiology evidence evaluation using modified Bradford Hill aspects, as described by Goodman et al. (Citation2020) (). We used the experimental evidence to provide information on biological plausibility in humans, as described above, and on coherence (i.e. whether all the evidence fits together). Similar to the framework for assessing causation and human relevance of experimental studies, if all the Bradford Hill aspects were met, we concluded that the evidence as a whole supports causation. If not all of the aspects were met, we determined whether it is more likely that the evidence as a whole supports causation (i.e. provided likely explanations for any aspect that was not met), is suggestive of causation, supports no causation, or is inadequate to determine causation.
Table 3. Modified Bradford Hill aspects for evidence integration.
Causal conclusion
shows how evidence from different realms were integrated to evaluate causation. We used a four-tiered framework for causality that is consistent with other causal frameworks, such as that defined in the Institute of Medicine (IOM) report Improving the Presumptive Disability Decision-Making Process for Veterans (IOM Citation2008). As shown in , if all the modified Bradford Hill aspects were met, or most were met and there is a likely explanation for each that was not met, we concluded that the relationship between metallic nickel exposure and respiratory cancer in humans is causal. If an assessment of the overall evidence indicated that a causal relationship is more likely than not, but there was inadequate information to assess some of the modified Bradford Hill aspects and all other aspects were met or there is a likely explanation for each that was not met, we concluded that the relationship between metallic nickel exposure and respiratory cancer in humans is suggestive. If there was inadequate information to assess most or all of the modified Bradford Hill aspects or most/all of the aspects were not met and there is no likely explanation for why they were not, we concluded that the evidence for a causal relationship is inadequate. Finally, if the overall evidence indicated there is no causal relationship, based on the modified Bradford Hill aspects (e.g. there was a consistent lack of an association in robust epidemiology studies and the experimental evidence indicated a lack of biological plausibility), we concluded that the relationship between metallic nickel exposure and respiratory cancer in humans is not causal.
Figure 1. The experimental and epidemiology evidence are integrated together, using modified Bradford Hill aspects to aid in judgment of causality, with experimental evidence informing the aspects of coherence and biological plausibility. A four-tiered framework for causality is used to reach a causal conclusion. Adapted from Goodman et al. (Citation2020).
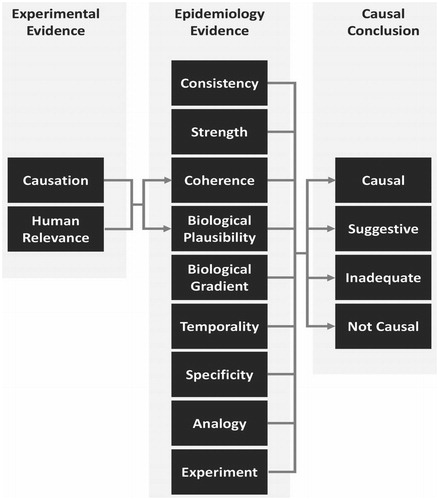
Table 4. Criteria for reaching conclusions about causality.
Results
Metallic nickel properties and toxicokinetics
Physical and chemical properties
Nickel metal can exist as a hard, lustrous, silvery-white metal or a gray powder. Nickel is the 24th most abundant element in the earth's crust (IARC Citation1990; ATSDR Citation2005). It is soluble in dilute nitric acid, slightly soluble in hydrochloric acid and sulfuric acids, and insoluble in water at any temperature (IARC Citation1990). Nickel substances can exist in five oxidation or valence states (−1, 0, +2, +3, and +4), but the most common under normal environmental conditions is the +2 valence state, from which many species of nickel compounds are formed (ATSDR Citation2005).
Metallic nickel encompasses nickel substances in the zero valence state, and these substances require oxidation to release the Ni2+ ion. At standard temperatures, the silvery metal form is not reactive in air or water, but the powder form is reactive in air, depending on its size distribution (ATSDR Citation2005). Upon prolonged storage, finely divided nickel metal powder can undergo surface oxidation in air, resulting in the conversion of a large fraction to oxide form (IARC Citation1990), but this very thin layer of surface oxides does not impart the properties of nickel oxides to nickel metal. Pure nickel metal is used to produce alloys, which can be categorized as nickel–copper, nickel–chromium, nickel–iron–chromium, nickel–chromium–cobalt, nickel–aluminum, nickel–molybdenum, and nickel-containing steels; these alloys contain 3.5–66.5% nickel by weight (IARC Citation1990).
Nickel refinery workers are generally exposed to mixtures of several different nickel species when nickel metal is extracted from sulfidic nickel ores and lateritic ores through nickel smelting and refining processes. Metallic nickel is produced as nickel powder or pellets using different processes, such as the Mond carbonyl process, or as electrolytic cathodes or small "rounds" using the electro-refining process (IARC Citation1990).
As with other airborne particles, the size of metallic nickel particles affects their respirability and, thus, their toxicity. Airborne particles can be classified into different aerosol fractions based on their penetration into the various regions of the respiratory tract (Oller and Oberdorster Citation2010). The inhalable aerosol fraction consists of particles with aerodynamic diameter ≤100 μm, and these particles can enter the body through the nose and mouth during breathing. The thoracic aerosol fraction is the subfraction of inhalable particles with aerodynamic diameter <30 μm that can penetrate beyond the larynx. The respirable (or alveolar) aerosol fraction is the subfraction of inhalable particles with aerodynamic diameter <10 μm that can penetrate into the alveolar region of the lung, including the respiratory bronchioles and the alveolar ducts and sacs. While experimental animal studies of metallic nickel used particles that were generally in the range of 2–3 μm in aerodynamic diameter, typical occupational exposures to the various forms of nickel in refineries were to a mixture of particle sizes, with the majority being coarser aerosols up to 100 μm in aerodynamic diameter and generally only 10–20% of the total nickel mass comprising the respirable (<10 μm in aerodynamic diameter) fraction (Oller and Oberdorster Citation2010).
Some nickel metal and other airborne particles are classified as nanoparticles, which are particles that have at least one of their dimensions in the range of 1–100 nm (Magaye and Zhao Citation2012). Nickel nanoparticles have certain characteristics that are not present in nickel particles of larger diameter, including a high surface area, high surface energy, and high magnetism, as well as low melting and burning points (Magaye and Zhao Citation2012; Ahamed and Alhadlaq Citation2014). These characteristics have led to nickel nanoparticles being used in small volumes for a number of industrial applications in recent years, including as sensors, electrode materials in multilayer ceramic capacitors, nanorings in memory cells, and in controlled magnetic hyperthermia applications (Magaye and Zhao Citation2012; Ahamed and Alhadlaq Citation2014). The physical and chemical characteristics, as well as the toxicity, of nanoparticles cannot be predicted from larger particles of the same substance, and several in vitro and in vivo studies have shown that nanoparticles (including nickel metal nanoparticles) are more toxic than their higher-diameter counterparts, with surface chemistry playing a role in nanoparticle toxicity (Oberdorster et al. Citation1994; Oberdorster Citation2001; Zhang et al. Citation2003; Zhao et al. Citation2009; Magaye et al. Citation2016).
There are no epidemiology studies or cancer bioassays evaluating the potential carcinogenicity of nickel nanoparticles available in the current literature, though there are some studies of the potential mutagenicity and genotoxicity of nickel-containing nanoparticles, including metallic nickel. Exposures in the studies of workers in the nickel refining and processing industry reviewed in this paper were to micron-sized particles of metallic nickel and nickel compounds, with a very small percentage of incidental nanoparticles expected to have been present with very high temperature processes and primarily as nickel oxides. In addition, exposures to experimental animals in the available cancer bioassays with metallic nickel and nickel compounds were also to micron-sized particles. Thus, it is unclear whether the mutagenicity and genotoxicity studies of metallic nickel nanoparticles are informative to the evaluation of the potential carcinogenicity of micron-sized metallic nickel particles, and for this reason, we did not include the mechanistic studies of metallic nickel nanoparticles in our evaluation.
Toxicokinetics and bioavailability
According to ATSDR (Citation2005), approximately 20–35% of nickel deposited in the lungs after inhalation exposure is absorbed into the systemic circulation, depending on the solubility of the nickel compound. Higher urinary levels of nickel have been observed in workers exposed to water-soluble nickel compounds compared to insoluble nickel compounds. Once inhaled, water-soluble nickel compounds are more likely to undergo dissolution and absorption, compared to insoluble nickel compounds which are more likely to be cleared via alveolar macrophages and/or the mucociliary escalator. Regardless of the exposure route, absorbed nickel is excreted in the urine, whereas nickel not absorbed from the gastrointestinal tract after macrophage-mediated clearance is excreted in the feces (ATSDR Citation2005).
The lung clearance kinetics of metallic nickel are not well studied. WIL Research Laboratories, Inc (Citation2004) examined lung burdens in rats exposed to nickel metal powder at 1–8 mg/m3 for 6 h per day, 5 days per week, for 90 days. The authors found that depending on the exposure concentration, retention half-times ranged from 26.5 to 110 days. In a chronic study in rats exposed to 1 mg/m3 nickel metal powder (Oller et al. Citation2008), the retention half-time was 26.5 days in male rats and 53.3 days in female rats (Goodman et al. Citation2011). Similar retention half-times (28–39 days) were reported in rats after a 5-h inhalation exposure to 0.15, 1.14, or 2.54 mg/m3 nickel metal nanoparticles that formed agglomerates in the exposure aerosol with a mass median aerodynamic diameter (MMAD) of 1.3 μm (Serita et al. Citation1999).
The processes that follow lung deposition and retention in the respiratory tract can modify the intracellular and intranuclear bioavailability of nickel ions in target cells in the respiratory tract. Several studies have shown that endocytosis by lung epithelial cells is the primary mechanism of uptake of nickel ions from insoluble nickel compounds in vitro (Benson et al. Citation1992; Goodman et al. Citation2011). Metallic nickel is taken up by endocytosis to a lesser extent than sulfidic nickel particles, however, though this has not been examined in lung epithelial cells. For example, Costa et al. (Citation1981b) reported uptake indices of 0–1.2% for metallic nickel and 6.1–23% for sulfidic nickel compounds in Chinese hamster ovary (CHO) cells (an epithelial-like cell line) exposed to similar concentrations of each nickel substance. Similarly, Costa and Heck (Citation1982) reported uptake indices of 4% for metallic nickel and 22–27% for crystalline α-nickel sulfide in CHO cells. Other studies have also shown that sulfidic forms of nickel are more readily taken up by endocytosis in CHO cells or rodent embryo cell lines than other nickel compounds, including metallic nickel (Costa and Mollenhauer Citation1980a, Citation1980b; Abbracchio et al. Citation1981, Citation1982; Costa et al. Citation1981a; Miura et al. Citation1989). A factor that may contribute to the poor intracellular uptake of metallic nickel particles is their ability to passivate, which involves the formation of a non-equilibrium film on their surface that blocks oxidizing agents from reacting with the metal to form divalent nickel ions, thus decreasing their surface reactivity (Revie and Uhlig Citation2008). It has been shown that surface charge influences the potential for endocytosis of nickel-containing particles, as negatively charged particles are more readily endocytosed than neutral or positively charged particles (Abbracchio et al. Citation1981, Citation1982; Heck and Costa Citation1982).
Metallic nickel particles are expected to have low extracellular dissolution, given their low predicted solubility in lung fluids (Oller et al. Citation2009). If taken up into cells by endocytosis, nickel particles are retained within cytoplasmic vacuoles where they undergo slow dissolution, or the vacuoles can fuse with lysosomes and expose the nickel particles to the acidic content of the lysosome, which may enhance the dissolution rate and release of nickel ions (Costa and Mollenhauer Citation1980a, Citation1980b; Abbracchio et al. Citation1981; Costa et al. Citation1981b, Citation1982; Evans et al. Citation1982). Nickel metal does not react rapidly with diluted, non-oxidizing acids or with organic acids, however, but under oxidizing conditions with low pH, increased nickel ion release over time is expected (Oller et al. Citation2009). This may vary based on the specific substance, as Latvala et al. (Citation2016) reported 100% particle dissolution of a sample of nickel metal particles 24 h after being dispersed in artificial lysosomal fluid, whereas a different sample underwent only 68% dissolution. After uptake into A549 cells, Latvala et al. (Citation2016) reported that both samples of particles were largely intact after 24 h, indicating slow intracellular release. Based on the poor endocytotic uptake and relatively slow intracellular dissolution (based on corrosion via oxidation), it is expected that the yield of intracellular nickel ions from metallic nickel particles would be low (Sivulka Citation2005; Oller et al. Citation2009).
Epidemiology studies
Literature selection
Our literature search for epidemiology studies evaluating respiratory cancer risks among participants who were potentially exposed to metallic nickel yielded 505 articles in PubMed and 569 articles in Scopus. After a review of titles and abstracts, as well as reference lists from relevant review articles, we identified 88 articles for full text review. After full text review, we identified 28 studies that included results for lung cancer, nasal/sinonasal cancer, laryngeal cancer, or respiratory cancers combined. The majority of these studies were conducted in US, Canadian, or European populations. Among the 28 studies, 13 included both males and females and 15 included only males. With regard to study design, 20 were cohort studies (with study characteristics summarized in Supplemental Table S4) and 8 were case–control studies (with study characteristics summarized in Supplemental Table S5).
Table 5. Study quality/risk of bias ratings for cohort studies evaluating metallic nickel exposure and respiratory cancer outcomes.
The 20 cohort studies evaluated respiratory cancer risks of 18 cohorts in particular industries (as discussed further below) that were generally followed for decades between the 1950s and the 2010s. Some of these cohorts were also included in the comprehensive evaluation of nickel carcinogenesis conducted by ICNCM (Citation1990). Workers in these industries were typically exposed to different combinations of several forms of nickel (e.g. metallic, oxidic, sulfidic, and water-soluble nickel compounds) and other non-nickel substances (e.g. chromium, cobalt, tungsten), where any health effects attributable to metallic nickel alone cannot be determined. Only one cohort study directly assessed the potential carcinogenicity of exposure to metallic nickel alone (Cragle et al. Citation1984), whereas several other cohort studies evaluated workers with exposure to predominantly metallic nickel (either nickel metal or nickel alloys) who may or may not have had some co-exposure to oxidic nickel.
The case–control studies each focused on patients with respiratory cancers who were generally identified from the population over several years (mostly between the 1970s and 2000s) and had occupational histories in a wide variety of industries. The particular forms of nickel exposures were not specified in any of the case–control studies, but potentially include metallic nickel.
Cohorts of workers exposed to metallic nickel
We identified 18 cohorts of workers involved primarily in four types of operations where exposure to metallic nickel (and often together with other forms of nickel) occurred, including barrier manufacturing, alloy manufacturing/grinding, nickel refining, and hardmetal production.
One cohort with workers in barrier manufacturing was identified. This cohort was made up of workers at the Oak Ridge Gaseous Diffusion Plant in Tennessee, US, which produced barrier material for the enrichment of uranium using finely divided, high-purity, metallic nickel powder (exposure ranging between 0.1 and 1.0 mg/m3) (Cragle et al. Citation1984; ICNCM Citation1990). Nickel exposure in this cohort was only to metallic nickel.
Seven cohorts were identified in the alloy manufacturing/grinding industry. These include the Kemi mine and Tornio production units of Outokumpu Group, Finland, where in the stainless steel melting shop, nickel was added as an alloying metal (together with molybdenum) with median ambient air concentrations of 1.8–3.1 μg/m3 (Huvinen and Pukkala Citation2013); the Henry Wiggin Alloy Company in Hereford, England, where low levels of oxidic and metallic nickel were used, together with iron, cobalt, copper, and chromium (total mean nickel exposure of 0.04–0.84 mg/m3) (Sorahan Citation2004); a French factory producing stainless and alloyed steel, where nickel was used simultaneously with chromium in most workplaces (Moulin et al. Citation2000); the International Nickel Company (INCO) Huntington Alloys plant operations in West Virginia, US, where nickel-containing alloys were produced and no nickel refining was conducted after 1946 (Enterline and Marsh Citation1982; ICNCM Citation1990); and a cohort from 13 high nickel alloy plants located throughout the US (including the INCO Huntington Alloys plant), where metallic and/or oxidic nickel were likely used and mean total nickel exposures for various work areas are estimated to have ranged from 0.13 to 2.22 mg/m3 (average 0.73 mg/m3) (Arena et al. Citation1998; Sivulka and Seilkop Citation2009). Nickel alloy grinding workers are exposed to similar nickel forms as alloy workers (although exposure levels are likely much lower), so we also evaluated a cohort from two Swedish plants where stainless steel (18% nickel, 8% chromium, 74% iron) grinding was conducted for the production of sinks and saucepans (Jakobsson et al. Citation1997) and a subcohort of workers who performed stainless steel grinding from 79 Danish welding companies where nickel and hexavalent chromium were used in stainless steel (but not mild steel) welding (Hansen et al. Citation1996).
Six cohorts were identified in the nickel refining industry. These cohorts include workers who potentially worked in refinery operations that ended in 1946 at the INCO Huntington Alloys plant in West Virginia, US (Enterline and Marsh Citation1982; ICNCM Citation1990); nearly all of the workers in the Mond/INCO refinery in Clydach, Wales (Easton et al. Citation1992; Sorahan and Williams Citation2005); the entire cohort working at the Falconbridge refinery in Kristiansand, Norway (Grimsrud et al. Citation2003); the INCO smelter and refinery workers in Ontario, Canada (Seilkop et al. Citation2017); and the Outokumpu Oy smelter and refinery workers in Harjavalta, Finland (Pavela et al. Citation2017). These cohorts were made up of workers who refined and processed sulfidic nickel ores, resulting in varying amounts of sulfidic, oxidic, water-soluble, and metallic nickel in workplace air. A subgroup of workers in nickel pellet and powder production at the Mond/INCO refinery in Wales is assumed to have been exposed predominantly to nickel metal, however (Sorahan and Williams Citation2005). In addition, the Sherritt hydrometallurgical nickel refinery in Fort Saskatchewan, Alberta, Canada (Egedahl and Collins Citation2009) was made up of workers who recovered nickel from solution by adding hydrogen to produce nickel powder, which predominantly involves the use of nickel concentrate and high levels of metallic nickel (mean exposure 2–4 mg/m3) (Egedahl et al. Citation2001). We focused our analysis of the nickel refining industry on these latter two groups of workers, as their nickel exposures were predominantly to metallic nickel.
Four cohorts were identified in the hardmetal production industry. These cohorts included multiple sites in the US (Marsh et al. Citation2017a), UK (McElvenny et al. Citation2017), Sweden (Svartengren et al. Citation2017; Westberg et al. Citation2017), and Germany (Morfeld et al. Citation2017), where nickel was added as a trace element to hardmetal mixtures of tungsten carbide to impart specific properties and may also have been used as a binding agent, alone or in conjunction with cobalt. The ratio mean (i.e. sum of cumulative exposure/sum of duration) intensity of nickel exposure ranged from 0.004 to 0.006 mg/m3 across the countries (Marsh et al. Citation2017b). Although the nickel exposures in this industry were low and there were co-exposures to other metals, we included these cohorts in our evaluation for completeness.
Overall, our evaluation of epidemiology studies focuses on the 16 cohort studies where nickel exposures were to predominantly (or only) metallic nickel, with some possible co-exposure to oxidic nickel. This includes the studies of barrier manufacturing, alloy manufacturing/grinding, pellet and powder production at a refinery, hydrometallurgical nickel refining, and hardmetal production. Results for the other identified cohort studies of refinery workers and the case-control studies are presented in the Supplemental materials (Tables S6 and S7, respectively).
Table 6. Results of metallic nickel and respiratory cancer cohort studies.
Table 7. Study quality/risk of bias ratings for experimental animal studies evaluating metallic nickel exposure and respiratory cancer outcomes.
Study quality evaluation
We applied our study quality/RoB criteria for epidemiology studies (Table S1) to the 16 identified cohort studies that evaluated exposures to predominantly metallic nickel (). Of these, eight were categorized into Tier 1 (Cragle et al. Citation1984; Sorahan Citation2004; Sorahan and Williams Citation2005; Egedahl and Collins Citation2009; Huvinen and Pukkala Citation2013; Morfeld et al. Citation2017; Marsh et al. Citation2017a, Citation2017b) and the remaining eight studies were categorized into Tier 2. There are a number of common methodological limitations of the epidemiology studies, particularly with regard to the three key RoB criteria (exposure characterization, outcome assessment, and confounding), that affect the causal interpretation of study results. As none of the studies were categorized into Tier 3, however, all 16 epidemiology studies are included in our evaluation and integration of the evidence.
The retrospective assessment of nickel exposure in the 16 cohort studies is subject to information bias. Nickel exposure was most commonly reconstructed based on a combination of historical measurement data and job history, though two studies (Hansen et al. Citation1996; Moulin et al. Citation2000) did not use any exposure measurements. The historical measurement data were also usually very limited. For example, area air measurements or personal measurements tended to be collected in select departments over select portions of the study period, and among a small group of workers; therefore, even if measurement data were used, they may not have represented the actual exposure levels of the participants given their particular job histories (e.g. department and time) and personal characteristics (e.g. job location and associated activities), introducing potential measurement error.
The assessment of respiratory cancer outcomes is subject to outcome misclassification in all but one of the cohort studies (Jakobsson et al. Citation1997). These studies did not incorporate histological or cytological examination in addition to relying on official records (e.g. medical records, registries, death certificates). While the use of official records ensures objectiveness of outcome assessment and good capture rates of cancer patients, it may include misdiagnosed cases without histological confirmation (i.e. the gold standard). It is also worth noting that most (12 out of 16) of the cohort studies only measured cancer mortality, which is affected by both cancer development and survival, although lung cancer survival tends to be of a relatively short duration (i.e. less than 5 years) (Allemani et al. Citation2018). By contrast, measurement of incident cases directly captures cancer development and is more informative for evaluating cancers with a high survival rate, such as laryngeal cancer (Jayakrishnan et al. Citation2020).
Confounding likely has substantially impacted most of the study results and was the main driver for categorization of studies into Tier 1 or Tier 2. As noted above, smoking, other occupational exposures, and nonmetallic forms of nickel are important confounders for the association between metallic nickel exposure and respiratory cancer risk that should be adjusted for in the analysis, as they tend to be more prevalent among metallic nickel-exposed workers compared to comparison groups (i.e. national or local populations), leading to overestimation of respiratory cancer risk associated with metallic nickel exposure. For example, prevalence rates for current smoking are higher among blue collar workers than the general population (Brackbill et al. Citation1988), and Marsh et al. (Citation2017a) reported a higher smoking prevalence in the US cohort of hardmetal production workers (87.5% of lung cancer cases and 64.1% of workers that did not develop lung cancer) compared to the average smoking prevalence of the general US population (50%) from a 1995 survey of behavioral risk factors. Similarly, Sorahan and Williams (Citation2005) reported that 67% of workers in the Mond/INCO refinery in Wales with known smoking status were current or past smokers, though they did not report smoking prevalence for the general population of Wales at that time.
None of the epidemiology studies took into consideration all three important confounders (smoking, other occupational exposures, and nonmetallic forms of nickel), which is not surprising given that the primary objectives of many of the 16 selected cohort studies were not to evaluate metallic nickel specifically. Seven of the cohort studies only adjusted for sociodemographic characteristics, but none of the three important confounders; the remaining studies adjusted for some, but not all, of these important confounders. In addition, none of the studies evaluated whether individuals with respiratory cancer had a history of chronic obstructive pulmonary disease, bronchitis, or diffuse pulmonary inflammation and fibrosis to assess other potential etiologies.
Other common characteristics of the epidemiology studies include low potential attrition bias and selective reporting bias, as well as a lack of sensitivity analyses to assess the robustness of study results. With regard to temporality of exposure and outcome, while six studies considered a clear and reasonable lag time (i.e. at least 10 years) between nickel exposure and respiratory cancer outcomes by design, seven other studies only examined lag time in stratified analyses, and three studies did not consider any lag time. Overall, the majority of studies were rated as having definitely or probably low RoB for each of the study quality/RoB criteria.
Evaluation of study results
The results of the cohort studies are summarized in and are discussed below for each type of respiratory cancer.
Lung cancer
The association between lung cancer and nickel exposure was examined in all of the cohort studies except for that by Hansen et al. (Citation1996). The study of 813 barrier manufacturing workers at the Oak Ridge Gaseous Diffusion Plant, who were exposed only to the metallic form of nickel, reported no excess lung cancer mortality compared to the US population (standardized mortality ratio [SMR] = 0.54, 95% CI: 0.25–1.03). Workers with at least 15 years since first exposure also had no increased risk of lung cancer (SMR = 0.60, 95% CI: 0.17–1.13), nor did workers with at least 15 years of employment in this group (SMR = 1.09, 95% CI: 0.35–2.54) (Cragle et al. Citation1984; ICNCM Citation1990). None of these results were adjusted for smoking or occupational co-exposures. Similarly, the study of 718 Canadian hydrometallurgical refinery workers exposed primarily to metallic nickel by Egedahl and Collins (Citation2009) reported no association with death rate for lung cancer compared to the Canadian population (SMR = 0.74, 95% CI: 0.38–1.29). This study also reported a statistically significant decrease in mortality from all causes and all neoplasms compared to the national population. While some have attributed this to the healthy worker effect, this is not a valid explanation for cancer endpoints because of the long latency for cancer and lack of factors to predict future cancers at the time individuals enter the workforce (Li and Sung Citation1999; Fornalski and Dobrzyński Citation2010).
Sorahan and Williams (Citation2005) reported that the 219 workers in pellet and powder production (exposed primarily to metallic nickel) at the Mond/INCO refinery in Wales had no statistically significant elevation in lung cancer mortality compared to the national population (SMR = 1.71, 95% CI: 0.78–3.25), though these results were not adjusted for smoking. Similarly, in the alloy production industry, the study by Sorahan (Citation2004) reported no excess lung cancer mortality among 1999 workers compared to the general population, even when stratified by years since they were hired (e.g. SMR = 1.1, 95% CI: 0.75–1.56, for the subgroup of workers with at least 30 years since hire) or cumulative duration of employment after adjustment for smoking (e.g. relative risk [RR] = 1.3, 95% CI: 0.61–2.74, for ≥20 years employment duration compared to 5–9 years duration). Another study of alloy production workers reported no association with lung cancer incidence in the Finnish cohort of 703 workers evaluated by Huvinen and Pukkala (Citation2013), after controlling for occupational co-exposures but not for smoking or nonmetallic nickel (standardized incidence ratio [SIR] = 0.76, 95% CI: 0.09–2.76).
Other studies of alloy production workers were categorized into Tier 2, largely because of a lack of adjustment for important potential confounders, especially smoking and nonmetallic forms of nickel (e.g. oxidic nickel). Nevertheless, these studies reported no statistically significant excess lung cancer mortality in a cohort of 4897 French workers (SMR = 1.19, 95% CI: 0.89–1.55), even when examined by increasing exposure levels in a nested case–control study of this cohort with adjustment for smoking (Moulin et al. Citation2000), or the cohort of 1353 alloy production workers in the INCO Huntington Alloys plant (Enterline and Marsh Citation1982; ICNCM Citation1990), even in workers with at least 20 years of employment and 15 years since first exposure (SMR = 0.48, 95% CI: 0.10–1.41). Although exposures to metallic nickel during stainless steel grinding are much lower than exposures during alloy manufacturing, the study of 719 Swedish stainless steel grinders by Jakobsson et al. (Citation1997) also reported no increase in the incidence of lung cancers compared to the general population (SIR = 0.6, 95% CI: 0.2–1.2).
The largest alloy production cohort of approximately 31 000 workers across 13 high nickel alloy plants in the US was studied by Arena et al. (Citation1998). Nickel exposures for various work areas across the plants ranged from 0.13 to 2.22 mg Ni/m3 (Sivulka and Seilkop Citation2009). The entire cohort contributed 800 373 person-years, with 24 202 person-years distributed among workers with at least 20 years of cumulative employment. Arena et al. (Citation1998) reported no excess risk of lung cancer mortality in the total cohort compared to the local population (SMR = 1.01, 95% CI: 0.95–1.08), and a small increased risk compared to the US population (SMR = 1.13, 95% CI: 1.06–1.21). Neither of these effect estimates were adjusted for smoking or other important potential confounders. There was no increasing exposure–response association with duration of exposure or time since first employment for either comparison, and the authors provided evidence to suggest that the small increase in lung cancer mortality in the comparison to the US population was attributable to non-occupational factors associated with geographic areas of residence rather than to occupational exposure. In addition, the authors noted that while use of local mortality rates from urban areas can sometimes overestimate the expected number of deaths for lung and some other cancers, the plants themselves (and, thus, the local populations in this study) were in urban industrial areas, providing some control for non-occupational lung cancer risk factors associated with urban residence. The study by Arena et al. (Citation1998) does not provide substantive evidence that exposure to metallic nickel increases the risk of lung cancer, and this is supported by a lack of excess lung cancer risk for 216 workers in the powder metallurgy department of the plants (RR = 0.67, 95% CI: 0.24–1.46), where average exposures to metallic nickel were much higher (approximately 3 mg Ni/m3) compared to other departments (Sivulka Citation2005).
In the hardmetal production industry, studies of cohorts in the US, Germany, and the UK reported no excess mortality from lung cancer compared to local or national populations, nor when examining cumulative nickel exposure for the US and German cohorts. For example, Marsh et al. (Citation2017a) reported an SMR of 0.97 (95% CI: 0.79–1.18) for US workers compared to the local population, which decreased to 0.7 (95% CI not reported) after adjustment for smoking. The authors also evaluated RRs across levels of cumulative exposure with adjustment for occupational co-exposures, reporting no increase in lung cancer risk for the group of workers with the highest cumulative exposure (RR = 0.78, 95% CI: 0.26–2.4). Morfeld et al. (Citation2017) reported an SMR of 0.93 (95% CI: 0.68–1.25) for German workers compared to the national population and a hazard ratio (HR) of 1.09 (95% CI: 0.01–18.81) for a 1-unit increment in cumulative nickel exposure, with adjustment for occupational co-exposures. McElvenny et al. (Citation2017) reported an SMR of 0.91 (95% CI: 0.15–1.92) for workers in the UK with at least 20 years of employment, with no adjustment for important potential confounders.
Studies of the hardmetal production cohort in Sweden reported an increased incidence of lung cancer (SIR = 1.38, 95% CI: 1.01–1.85) and excess lung cancer mortality (SMR = 1.68, 95% CI: 1.50–1.89) compared to the general Swedish population (Svartengren et al. Citation2017; Westberg et al. Citation2017); however, these increases were not adjusted for smoking or other important potential confounders and an examination of exposure duration showed an inverse relationship with lung cancer. The effect estimates for lung cancer were attenuated and no longer statistically significant when workers with at least 20 years of employment and a latency period of at least 20 years were analyzed (SIR = 1.17, 95% CI: 0.59–2.10 for the study by Svartengren et al. Citation2017; SMR = 1.3, 95% CI: 0.9–1.8 for the study by Westberg et al. Citation2017). As these workers were co-exposed to cobalt and tungsten, internal comparisons indicated that the increased risks of lung cancer showed no correlation to cobalt exposure and therefore could be attributable to other factors besides occupation. Exposure data for metallic nickel were available for only one process (hot rolls production) in one of the three hardmetal production companies studied and indicated that nickel levels were low (i.e. most measurements were less than 0.1 mg/m3). When 18 cases of lung cancer were analyzed based on dichotomized nickel exposure (≤0.01 and >0.01 mg/m3), no statistically significant excess in lung cancer mortality was reported, though both of the SMRs exceeded 1 (Westberg et al. Citation2017).
When the lung cancer mortality data from each of the four hardmetal production cohorts (plus workers from an Austrian cohort exposed to cobalt without co-exposure to nickel) were pooled together in an analysis of more than 32 000 workers (with the Swedish cohort contributing the most workers, at 15 633), a statistically significant excess in lung cancer mortality was reported for the entire pooled cohort (SMR = 1.2, 95% CI: 1.09–1.31) compared to the local population (Marsh et al., Citation2017b). There was no excess lung cancer mortality in long-term workers (i.e. those employed for at least one year) compared to the local population (SMR = 1.1, 95% CI; 0.97–1.23), however, indicating that the overall elevated lung cancer mortality was driven by short-term workers (i.e. those with a shorter duration of exposure), making it less likely that nickel exposure is associated with excess lung cancer mortality. Neither of these results were adjusted for important potential confounders. Analyses of lung cancer RRs based on increasing duration, cumulative exposure, and average intensity of exposure to nickel showed no exposure–response relationship in both univariate and multivariate models adjusted for country (see for results of multivariate models), and lung cancer risk was not elevated in long-term workers specifically exposed to nickel after adjustment for confounding by smoking, indicating that there is no evidence of an association between metallic nickel exposure and lung cancer mortality across the cohorts of hardmetal production workers.
Effect estimates (SMRs or SIRs) for lung cancer from each study reviewed above are presented in . When more than one SMR or SIR was reported in a study, the estimate with comparison to the local population versus the national population, or for the longest exposure duration or latency, or the highest cumulative exposure is presented in the figure. If a study also reported relative risks (RRs) or hazard ratios (HRs) with adjustment for important potential confounders, those are presented below the SMR/SIR for that study. We did not calculate a summary estimate, as very few studies evaluated exposures to predominantly metallic nickel.
Figure 2. Effect estimates are SMRs or SIRs for each study (filled squares). If a study also reported an RR or HR with adjustment for important potential confounders, those are presented directly below the SMR or SIR for that study (unfilled squares). (a) Workers with ≥15 years since first exposure and ≥15 years of employment. (b) 95% confidence interval of effect estimate was calculated from the number of observed and expected deaths based on Fisher's exact test, as it was not reported by the study authors. (c) >5 years of employment, stainless steel melting. (d) ≥30 years since hire. (e) Adjusted for smoking. (f) ≥20 years of employment. (g) Hired in 1947 or later, with ≥15 years since first exposure. (h) Pellet and powder production. (i) Latency ≥20 years and >20 years of employment. (j) Long-term (≥1 year) workers. (k) Cumulative exposure ≥0.085 mg/m3-years. (l) Adjusted for occupational co-exposures. (m) Cumulative exposure per 1 mg/m3-years increment. HR: hazard ratio; RR: relative risk; SIR: standardized incidence ratio; SMR: standardized mortality ratio.
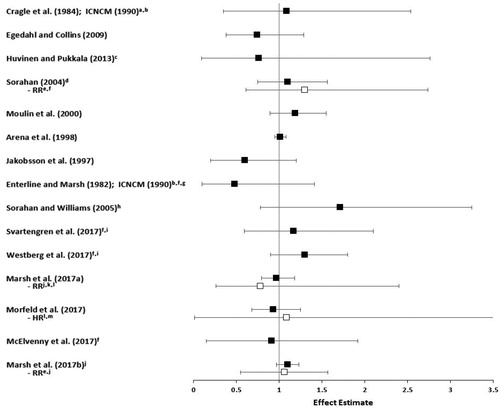
Nasal/sinonasal cancer
The association between metallic nickel exposure and nasal/sinonasal cancer was examined in five of the cohort studies (Marsh Citation1982; Cragle et al. Citation1984; Enterline and Huvinen and Pukkala Citation2013; Sorahan Citation2004; Jakobsson et al. Citation1997). None of these studies reported any cases of these cancers or deaths from these cancers, providing no evidence for an association between metallic nickel exposure and nasal/sinonasal cancers.
Laryngeal cancer
The association between metallic nickel exposure and laryngeal cancer was examined in nine of the cohort studies (Enterline and Marsh Citation1982; Cragle et al. Citation1984; Jakobsson et al. Citation1997; Arena et al. Citation1998; Sorahan Citation2004; Svartengren et al. Citation2017; Westberg et al. Citation2017; Marsh et al. Citation2017a, Citation2017b). The study of barrier manufacturing workers by Cragle et al. (Citation1984) and the study of alloy production workers by Enterline and Marsh (Citation1982) reported no deaths from laryngeal cancer (ICNCM Citation1990). Sorahan (Citation2004) reported no excess mortality from laryngeal cancer in the total cohort of 1,999 alloy production workers (SMR = 0.51, 95% CI: 0.01–2.85), based on only one case. Similarly, Jakobsson et al. (Citation1997) reported no increase in laryngeal cancer incidence in stainless steel grinders based on one case (SIR = 0.7, 95% CI: 0.0–3.9) and Arena et al. (Citation1998) reported no excess laryngeal cancer mortality in white, male alloy production workers compared to the local population (SMR = 0.67, 95% CI: 0.45–1.01).
Marsh et al. (Citation2017a) reported no excess mortality from laryngeal cancer compared to the local population, based on three cases in a cohort of 7304 hardmetal production workers in the US (SMR = 0.99, 95% CI: 0.20–2.88). In studies of Swedish hardmetal production workers, Svartengren et al. (Citation2017) did not report a statistically significant increased incidence of laryngeal cancer (SIR = 1.9, 95% CI: 0.62–4.44), nor did Westberg et al. (Citation2017) (SIR = 1.51, 95% CI: 0.55–3.28). Neither of these estimates accounted for important potential confounders. Marsh et al. (Citation2017b) reported no excess mortality from laryngeal cancer compared to the local population in a pooled analysis of hardmetal production workers from five countries, in both the total cohort (SMR = 0.85, 95% CI: 0.41–1.57) and subcohort of long-term workers only (SMR = 0.48, 95% CI: 0.13–1.24).
Effect estimates (SMRs or SIRs) for laryngeal cancer from each study are presented in . When more than one SMR or SIR was reported, the estimate with comparison to the local population versus the national population, or for the longest exposure duration, or for the white male population versus nonwhite males or females is presented in the figure.
Respiratory cancers combined
The association between metallic nickel exposure and respiratory cancers combined (which is dominated by lung cancer) was examined in six studies (Hansen et al. Citation1996; Arena et al. Citation1998; Egedahl and Collins Citation2009; McElvenny et al. Citation2017; Marsh et al. Citation2017a, Citation2017b). Egedahl and Collins (Citation2009) reported no excess mortality from all respiratory cancers combined in hydrometallurgy workers exposed to predominantly metallic nickel (SMR = 0.75, 95% CI: 0.40–1.29). Hansen et al. (Citation1996) reported no statistically significant increase in the incidence of all respiratory system cancers combined among 521 Danish stainless steel welders after adjusting for occupational co-exposures but not smoking (SIR = 1.57, 95% CI: 0.78–2.81). The large study of alloy production workers in 13 high nickel alloy plants in the US by Arena et al. (Citation1998) reported no excess risk of respiratory cancers combined in white males compared to the local population (SMR = 1.00, 95% CI: 0.94–1.07) and a small increased risk compared to the US population (SMR = 1.11, 95% CI: 1.03–1.18). This appears to be driven by the increase in lung cancer mortality when this cohort was compared to the US population. As discussed above, the lack of an exposure–response association and evidence that the reported increase in lung cancer mortality was likely attributable to confounding by non-occupational factors associated with metropolitan lifestyle indicates that this study does not provide evidence that nickel exposure in this cohort is associated with an increased risk of respiratory cancers.
Marsh et al. (Citation2017a) reported no excess mortality from all respiratory cancers combined among 7304 hardmetal production workers in the US compared to the local population (SMR = 0.96, 95% CI: 0.79–1.17). Similarly, McElvenny et al. (Citation2017) reported no excess mortality from all respiratory cancers combined compared to the local population in a cohort of 1538 hardmetal production workers from the UK (SMR = 0.81, 95% CI: 0.51–1.26). Marsh et al. (Citation2017b) reported a small but statistically significant excess in mortality from all respiratory cancers combined for a pooled cohort of hardmetal production workers from five countries compared to the local population (SMR = 1.19, 95% CI: 1.08–1.30). This association appears to be driven by short-term workers (SMR = 1.42, 95% CI: 1.22–1.64), as there was no association in an analysis of long-term workers only (SMR = 1.08, 95% CI: 0.96–1.21), making it less likely that nickel exposure is associated with excess respiratory cancer mortality. Neither estimate accounted for important potential confounders, however. The positive association in the total cohort is driven by the results for lung cancer alone, which, as discussed above, were not likely related to metallic nickel exposure.
Effect estimates (SMRs or SIRs) from each study are presented in . When more than one SMR or SIR was reported, the estimate with comparison to the local population versus the national population, or for the longest exposure duration, or for the white male population versus nonwhite males or females is presented in the figure.
Summary
The epidemiology literature discussed above indicates that for cohorts of workers with exposure to predominantly (or only) the metallic form of nickel, with some co-exposure to oxidic nickel or other metals in certain cohorts, there is no evidence of increased risks of lung, laryngeal, or nasal/sinonasal cancers, nor of all respiratory cancers combined. Taken together, these studies do not provide evidence of an association between exposure to metallic nickel and respiratory cancers, particularly in light of methodological limitations with respect to potential information bias, outcome misclassification, and confounding by smoking, other occupational exposures, and nonmetallic forms of nickel, which limit the reliability of the results and their causal interpretation.
Experimental animal studies
Literature selection
Our literature search for experimental animal studies of metallic nickel carcinogenesis yielded 849 articles in PubMed and 351 articles in Scopus. After a review of titles and abstracts, as well as reference lists from relevant review articles, we identified 16 articles for full text review. After full text review, we identified six studies that evaluated the potential carcinogenicity of metallic nickel in experimental animals using an exposure route with administration into the respiratory tract (with study characteristics summarized in Supplemental Table S8). Two of these studies evaluated more than one animal species and/or exposure route in separate bioassays. Three studies used inhalation as the exposure route, with exposure durations up to two years. Three studies used intratracheal administration, either as a single exposure or weekly/biweekly for up to 24 weeks, and one study used injection into the lungs, as two administrations one year apart.
Table 8. Results of experimental animal studies of metallic nickel respiratory carcinogenicity.
Study quality evaluation
We applied our study quality/RoB criteria for experimental animal studies (Table S2) to each bioassay conducted among the six identified studies. Of the 11 bioassays, one was categorized into Tier 1, four were categorized into Tier 2, and six were categorized into Tier 3 based on their study quality/RoB ratings across domains (). The majority of studies evaluated tumors only when the animals died rather than during a specific time period after exposure, and did not include statistical analyses of the results. Some of the studies had poor characterization of the metallic nickel exposures, did not have an adequate number of animals, and did not adequately address attrition, which led to their categorization into Tier 2 or Tier 3. None of the studies reported that researchers were blinded to the exposure status of the animals; this can lead to false positive results, raising questions about the reproducibility of the study results (Begley and Ioannidis Citation2015; Macleod and Mohan Citation2019). The study by Oller et al. (Citation2008) included an independent pathology peer review of the findings, however, decreasing the likelihood of effects on reproducibility.
Evaluation of study results
The results of the experimental animal studies are summarized in . The only Tier 1 experimental animal study is the inhalation carcinogenicity study by Oller et al. (Citation2008) that was conducted according to OECD and US EPA guidelines for carcinogenicity studies and in compliance with Good Laboratory Practice (GLP) regulations. In this study, 50 rats of each sex were exposed to 0, 0.1, 0.4, or 1 mg/m3 nickel metal powder for 6 h per day, 5 days per week, for up to 24 months, followed by a 6-month observation period. The nickel metal powder was aerosolized in a generation system that was optimized to produce an aerosol of particles with an MMAD of 1.5–3 μm (geometric standard deviation ∼2.0 μm), as characterized by cascade impactors. The pathology findings were peer reviewed and a panel of academic, regulatory, and industrial experts oversaw the study. High mortality rates among rats in the highest dose group led to an early termination of exposures in that group and to the establishment of 0.4 mg/m3 as the maximum tolerated dose (MTD). There were no exposure-related increases in tumors in the respiratory tract (including in the nose) with either the 0.1 or 0.4 mg/m3 exposure, although there were statistically significant increases in the incidence of adrenal gland pheochromocytomas (benign and malignant) in males and combined adrenal cortical adenomas and carcinomas in females exposed to 0.4 mg/m3 nickel metal. Adrenal pheochromocytomas were also observed in rats chronically exposed to insoluble nickel compounds (NTP Citation1996a, Citation1996b) as well as other particulates (Ozaki et al. Citation2002; Greim et al. Citation2009) and are of questionable relevance to human occupational exposures to particulates (Greim et al. Citation2009). Oller et al. (Citation2008) considered these tumors to be secondary to the lung toxicity and hypoxemia caused by the nickel metal exposure rather than a direct effect of nickel on the adrenal gland. The increased incidence of combined cortical adenomas and carcinomas in females fell within the historical control range and was considered by the authors to be of uncertain relationship to the nickel metal exposure.
Two other inhalation carcinogenicity studies of metallic nickel included bioassays that were categorized as Tier 2 or 3. Hueper and Payne (Citation1962) exposed rats (Tier 2) or hamsters (Tier 3) to a mixture of powdered nickel (the majority of which had a particle diameter of 1–3 μm after grinding in a ball mill), limestone, and sulfur dioxide, though no exposure concentration or duration were reported. The sulfur dioxide was included to induce chemical trauma to the bronchial mucosa and serve as a co-carcinogen, and the limestone was added to prevent nickel particles from forming conglomerates. There were no tumors observed in the respiratory tract of rats or hamsters. As the exposure duration was not reported, it is unknown whether the animals were exposed long enough for any tumors to develop. Rats had chronic inflammatory changes, fibrosis, and squamous cell metaplasia of the epithelial lining in the lungs, whereas there were no such chronic respiratory effects reported in hamsters. Hueper (Citation1958) exposed Wistar rats (Tier 2), as well as Bethesda rats, guinea pigs, and mice (all Tier 3), to 15 mg/m3 metallic nickel powder with a diameter of 4 μm or less, prepared by precipitating nickel metal from nickel carbonyl, for 6 h per day, 4–5 days per week, for up to 21 months. Some benign and malignant tumors were observed in some of the guinea pigs and rats, but none were in the respiratory tract. There were no tumors or other abnormalities observed in mice. This study was compromised by high toxicity, as all animals had died before the end of the planned exposure period, and both this study and that by Hueper and Payne (Citation1962) did not include control groups except for the evaluation of guinea pigs by Hueper (Citation1958). We did not rely on the bioassays categorized as Tier 3 for evidence regarding the potential carcinogenicity of metallic nickel.
Three studies evaluated the carcinogenicity of metallic nickel after intratracheal administration. Although this exposure route bypasses the normal clearance mechanisms that occur with inhalation exposure, limiting its relevance to humans, such studies may be useful for understanding the carcinogenic potential of metallic nickel in the respiratory tract if the doses are not so high as to completely overwhelm clearance and induce particle overload. In the study by Ivankovic et al. (Citation1988), hamsters were administered a single dose of nickel metal powder (0, 10, 20, or 40 mg/animal), prepared by water atomization processes and ground in a ball mill to a diameter of 3–8 μm, as evaluated by electron microscopy. Although there was some evidence of a statistically significant increase in nickel-related malignant tumors in the highest dose group, these tumors were sarcomas located in the mediastinum, pleura, and cervix, and not carcinomas at alveolar sites as observed in inhalation bioassays with insoluble nickel compounds and in nickel refinery workers exposed to insoluble nickel compounds. There was also high toxicity in this dose group, though the deaths did not appear to be tumor related.
Muhle et al. (Citation1992) exposed hamsters intratracheally to a cumulative dose of 9.6 mg (with 12 administrations at 14-day intervals) nickel metal powder received from the INCO refinery in Clydach, Wales, with a median diameter of 3.1 μm, as evaluated by electron microscopy. The authors reported no statistically significant increase in tumor rates, though the statistical analyses were not described. The authors noted that several particulates, including nickel subsulfide and metallic nickel (in the intratracheal study by Pott et al. (Citation1987) described below) have induced lung tumors in rats but not in hamsters, suggesting that the hamster is not a good model for lung tumors from exposures to particulates. This is consistent with the lack of lung carcinomas in hamsters in the studies by Ivankovic et al. (Citation1988) and Hueper and Payne (Citation1962) discussed above. Although there is a lack of adequate historical control data for hamsters in carcinogenicity bioassays (as the vast majority of well-conducted carcinogenicity bioassays used rats or mice), this is not an issue for studies of nickel-containing substances, to which hamsters are not susceptible.
The Tier 3 study by Pott et al. (Citation1987) exposed rats to cumulative doses of 6 or 9 mg nickel metal powder that was noted to have been received from INCO in Ontario, Canada (with no information regarding preparation or particle size provided by the authors), over 20 or 10 weeks, respectively, via intratracheal administration. The authors reported adenocarcinomas or squamous cell carcinomas in 9 of 39 rats examined in the 6 mg dose group and in 7 of 32 rats examined in the 9 mg dose group, though the starting number of animals in each dose group was not reported. No analyses were performed to address the statistical significance of these findings and no comparison to historical controls was made, though no lung tumors were reported in the vehicle control group. In addition, the total lung burden of 6 mg in this study is more than 200-fold higher than the lung burden attained after 1 or 2 years of exposure to metallic nickel at the MTD of 0.4 mg/m3 (i.e. approximately 25 μg Ni/right lung) in the inhalation bioassay by Oller et al. (Citation2008), indicating that Pott et al. (Citation1987) used doses that cannot be achieved via a physiological exposure route. As the study by Pott et al. (Citation1987) was categorized as Tier 3 due to its methodological shortcomings and high RoB, its results should not be relied on as evidence for metallic nickel carcinogenicity. Similarly, the Tier 3 analysis of rats exposed to nickel metal powder by injection into the lungs by Hueper and Payne (Citation1962) reported one spindle cell carcinoma in the lungs in 1 out of 34 rats and no other respiratory tumors, with no statistical analyses, no control groups, and no comparison to historical controls. Thus, this study should not be relied on as evidence regarding the potential carcinogenicity of metallic nickel.
Overall, the Tier 1 and Tier 2 experimental animal studies of metallic nickel carcinogenicity indicate that there is generally no evidence of respiratory carcinogenicity with inhalation exposures to metallic nickel, and no evidence of respiratory tumors relevant to human exposures in studies that used intratracheal administration as the exposure route. Several studies have been conducted that used various parenteral exposure routes (e.g. intramuscular, intraperitoneal, intravenous, intrarenal, or subcutaneous injection) to administer metallic nickel to rodents, and these reported tumor responses that were mainly sarcomas localized at the injection site (as reviewed by IARC Citation1990 and Sivulka Citation2005). We did not include these studies in our evaluation because of their lack of relevance to human exposures to metallic nickel and nickel compounds in the respiratory tract.
Mechanistic studies
We evaluated the potential genotoxicity of metallic nickel in both in vitro and in vivo studies. Genotoxicity refers to the ability of substances to cause DNA damage, and genotoxicity tests can be categorized into mutagenicity tests and indicator tests. Mutagenicity tests evaluate whether a substance can cause permanent and heritable changes in DNA, such as gene mutations or alterations in the number or structure of chromosomes (though chromosomal changes may only induce mutations if they disrupt the coding sequence of genes), whereas indicator tests evaluate whether a substance can interact with and damage DNA without necessarily causing mutations (Eastmond et al. Citation2009). While indicator tests can provide suggestive, but not definitive, evidence that a substance is mutagenic, mutagenicity tests provide more clear evidence that a substance can cause the type of DNA damage that can potentially lead to cancer. Because of this, the determination of whether a substance is mutagenic is most relevant to evaluating its mode of action for carcinogenicity, and mutagenicity tests should be given preference over indicator tests for risk assessments of potential carcinogens (Eastmond et al. Citation2009). We also evaluated the ability of metallic nickel to induce cellular transformation in in vitro studies.
Various nickel compounds have been shown to be nonmutagenic in bacterial or yeast test systems, with inconsistent results across in vitro and in vivo mutagenicity tests (including gene mutations, micronucleus formation, or chromosome aberrations) (ATSDR Citation2005; Beyersmann and Hartwig Citation2008; Das et al. Citation2008; Goodman et al. Citation2009; Buxton et al. Citation2019). Nickel compounds have also been positive for genotoxicity in certain in vitro indicator tests, such as DNA strand breaks and sister chromatid exchanges (SCEs), as well as in studies of in vitro cellular transformation (ATSDR Citation2005; Goodman et al. Citation2009; Buxton et al. Citation2019). It should be noted that evaluation of SCEs is no longer considered an acceptable genotoxicity test by OECD, based on a lack of understanding of the mechanistic basis of the effect detected by the test, which is not reflective of genetic damage (OECD Citation2017; Moore et al. Citation2019).
The reported mutagenic and genotoxic effects in the in vitro studies of nickel compounds were most often weak and occurred at doses that were cytotoxic (Beyersmann and Hartwig Citation2008; Das et al. Citation2008; Goodman et al. Citation2009). Excessive cytotoxicity is an important consideration, because cells with precancerous changes must survive and undergo division for cancer to develop. Below, we evaluate the literature for the mutagenicity and genotoxicity of metallic nickel specifically.
Literature selection
Our literature search for mechanistic studies of metallic nickel yielded 549 articles in PubMed and 256 articles in Scopus. After a review of titles and abstracts, as well as reference lists from relevant review articles, we identified 51 articles for full text review. After full text review, we identified nine in vitro studies that evaluated the potential genotoxicity or cellular transformation ability of exposure to metallic nickel and one in vivo genotoxicity study (with study characteristics summarized in Supplemental Table S9). The mutagenicity studies specifically evaluated the endpoints of gene mutations, micronucleus formation, and chromosome aberrations, whereas the only genotoxicity indicator tests were the evaluation of DNA strand breaks in the in vitro studies and the evaluation of SCEs in the in vivo study.
Table 9. Study quality/risk of bias ratings for in vitro studies evaluating metallic nickel genotoxicity.
Study quality evaluation
We applied our study quality/RoB criteria for in vitro mechanistic studies (Table S3) to each assay conducted among the nine identified in vitro studies. Of the 10 in vitro assays, 4 were categorized into Tier 1, 5 were categorized into Tier 2, and 1 was categorized into Tier 3 based on their study quality/RoB ratings across domains (). The vast majority of studies used well-established assays to assess the outcomes, ensured that experimental conditions were identical across study groups, reported all data for the assays they included, and used appropriate statistical methods. Some studies did not adequately characterize the metallic nickel exposure, did not include appropriate controls (usually a lack of a positive control), and did not include an adequate number of replicates per study group, which led to their categorization into Tier 2 or Tier 3. None of the studies indicated whether research personnel were blinded to study group, evaluated results with metabolic activation, or compared results to historical control data, except for the study by Buxton et al. (Citation2020).
The majority of the studies did not report how the metallic nickel particles were prepared in the micron size range used in the genotoxicity experiments, though Costa et al. (Citation1981a, Citation1981b) reported that the particles in their studies were ground in mixing mills using vials with stainless steel or tungsten carbide grinding surfaces, and sized using light and electron microscopy. Other studies reported assessing particle size by electron microscopy or low angle laser light scattering (Magaye et al. Citation2014, Citation2016; Latvala et al. Citation2016; Buxton et al. Citation2020).
Some studies addressed the insolubility of metallic nickel in water (and thus aqueous cell culture medium) by vortexing or sonicating particles prior to their dilution into stock solutions or the cell culture medium to ensure the accuracy of particle concentrations (Costa et al. Citation1981b; Magaye et al. Citation2014, Citation2016; Latvala et al. Citation2016; Buxton et al. Citation2020), and Buxton et al. (Citation2020) conducted a solubility test with various solvents and vehicles, identifying dimethyl sulfoxide as the best solvent for stock solutions of the metallic nickel particles before their administration to the cell culture.
Evaluation of study results
The results of the in vitro mechanistic studies are summarized in . One study evaluated the ability of metallic nickel to induce gene mutations in vitro. This study evaluated gene mutations at the HPRT locus and was conducted in accordance with OECD guidelines (OECD Citation2016a). The results of this study were negative for HPRT mutations with exposure of hamster lung fibroblasts or mouse embryonic stem cells to metallic nickel particles (Buxton et al. Citation2020), providing evidence that metallic nickel does not induce point mutations in vitro.
Table 10. Results of in vitro mechanistic studies evaluating metallic nickel genotoxicity.
Buxton et al. (Citation2020) also evaluated micronucleus formation, and another study (Paton and Allison Citation1972) evaluated chromosome aberrations after exposure to metallic nickel. The study by Buxton et al. (Citation2020) was conducted in accordance with OECD guidelines (OECD Citation2016b) and reported no increase in micronucleus formation in hamster lung fibroblasts treated with metallic nickel particles at doses ranging from 3 to 18 μg/cm2. Paton and Allison (Citation1972) reported a lack of chromosome aberrations in human leukocytes exposed to nickel powder, but did not provide information on the particle size or concentration, the number of replicates, the statistical methods used, or the inclusion of a positive control. Based on these methodological shortcomings and high RoB, the results of the Tier 3 study by Paton and Allison (Citation1972) should not be relied on as evidence regarding the potential for metallic nickel to induce chromosome aberrations.
Three studies examined the ability of metallic nickel to induce DNA strand breaks. Two of these (Magaye et al. Citation2016; Assad et al. Citation1999) reported positive results in human lung alveolar epithelial adenocarcinoma cells (a malignant cell line with a high spontaneous mutation rate) and human peripheral blood lymphocytes, respectively, but also reported high cytotoxicity (≥50%) at the metallic nickel concentrations that induced statistically significant increases in DNA strand breaks. It has been reported that because DNA damage is associated with cell death, cytotoxicity in excess of 30% can induce false positive results in assays for DNA strand breaks (Tice et al. Citation2000; OECD Citation2014). Thus, it is likely that the positive results in these studies were confounded by high cytotoxicity. The other study, by Latvala et al. (Citation2016), did not report excess cytotoxicity. These authors exposed human lung alveolar epithelial adenocarcinoma cells to the same dose (20 μg/cm2) of two different samples of metallic nickel particles and found that one sample (Ni-m2) did not induce DNA strand breaks after 4 or 24 h of exposure, whereas the other sample (Ni-m1) induced a statistically significant increase in DNA strand breaks after 24 h of exposure but not after 4 h. Overall, these studies indicate that metallic nickel can induce DNA damage in certain cell types in vitro, but often as a result of high cytotoxicity.
Four studies examined cellular transformation of metallic nickel. Costa et al. (Citation1981a, Citation1981b) conducted cell colony transformation assays in primary hamster embryo cells exposed to metallic nickel (0.6–8 μg/cm2) and reported no transformation. Crystalline nickel subsulfide particles were used as a positive control and amorphous nickel sulfide was used as a negative control. Hansen and Stern (Citation1984) reported positive results for anchorage-independent transformation in soft agar for hamster kidney cells exposed to 32 or 64 μg/cm2 metallic nickel, but not at a lower dose of 16 μg/cm2. There were no positive controls, and the results for the negative controls were not reported. Magaye et al. (Citation2014) reported that metallic nickel particles were weakly positive for transformation of mouse epidermal cells in soft agar with exposure to a single dose (1 μg/cm2). Overall, these studies indicate mixed results for metallic nickel to induce cellular transformation, with some evidence for weak transforming ability in certain cell types.
One in vivo study evaluated the potential mutagenicity and genotoxicity of metallic nickel in rats (Zhong et al. Citation1990). We did not evaluate the study quality/RoB for this study as it was very poorly reported. The authors reported no effect on the frequency of SCEs in peripheral lymphocytes, and increases in chromosome aberrations and micronucleus formation in bone marrow cells of rats 1.5 months after they were exposed to a single dose of 2.5 mg metallic nickel (99.9% purity; 95% of particles <5 μm diameter) by intratracheal instillation. This study did not provide information on the animals, their housing conditions, or the methods for the endpoints assessed; used only one dose and one time point of questionable relevance to the endpoints; used an exposure route that is not relevant to human inhalation exposures; and did not include any statistical analysis of the results. In addition, the positive results in bone marrow after intratracheal exposure are questionable, given that a study assessing bone marrow mutagenicity after oral exposure to nickel sulfate hexahydrate (a soluble form of nickel with greater absorption than metallic nickel) at doses up to 112 mg Ni/kg-day was negative for micronuclei, even with elevated levels of nickel measured in the bone marrow and blood (Oller and Erexson Citation2007). Thus, this study does not provide reliable evidence for whether metallic nickel can induce genotoxicity or mutagenicity in vivo, and it is not considered further.
Because nickel ions are only weakly genotoxic, other mechanisms for nickel carcinogenicity that do not require nickel ions to interact directly with DNA have been proposed. A contributing mechanism for the respiratory carcinogenicity of many metals that has also been proposed for nickel is inflammatory responses triggered by cytotoxicity from high exposures, leading to increased regenerative cell proliferation in the respiratory tract (Oller et al. Citation1997; Goodman et al. Citation2011). Several studies have suggested that nickel ions induce indirect DNA damage by producing reactive oxygen species (ROS), leading to oxidative stress (Beyersmann and Hartwig Citation2008; Das et al. Citation2008; Goodman et al. Citation2009; Koedrith and Seo Citation2011; Magaye et al. Citation2012). Beyersmann and Hartwig (Citation2008) noted that there is often a discrepancy between the high, often cytotoxic doses of metals required to generate ROS and the comparatively low doses of those metals that potentially induce tumors in vivo, however, suggesting that metal-induced oxidative stress is not the sole mechanism for potential metal carcinogenesis.
Another potential mechanism is activation of signal transduction pathways and alterations in gene expression that can promote cell survival and proliferation through interference with iron homeostasis or other mechanisms (Costa et al. Citation2005; Beyersmann and Hartwig Citation2008; Koedrith and Seo Citation2011). Several studies have reported the transactivation or upregulation of expression of proteins involved in cell signaling pathways associated with tumor development and progression in in vitro studies with metallic nickel specifically, but only in dermal cells. For example, Magaye et al. (Citation2014) reported increased transactivation of AP-1 and NF-KB, as well as upregulation of R-Ras, C-myc, C-Jun, p65, and p50, in mouse epidermal cells exposed to nickel metal particles. Zhao et al. (Citation2009) reported increased expression of the apoptosis inhibitors Bcl-2 and phosphorylated Akt in mouse epidermal cells exposed to nickel metal particles. It should be noted that skin tumors have not been associated with dermal exposure to metallic nickel, which is widespread in human populations through contact with jewelry (ATSDR Citation2005; NTP Citation2016). Inhibition of DNA repair and interference with DNA methylation and histone acetylation have also been proposed, but would likely require nickel to be present in the cell nucleus (Beyersmann and Hartwig Citation2008; Koedrith and Seo Citation2011), and these mechanisms have not been evaluated for metallic nickel specifically.
Evidence integration for experimental studies
We integrated the evidence from the experimental animal and in vitro mechanistic studies for causation and human relevance, using the criteria in .
Causation
Consistency
Of the few experimental animal studies of metallic nickel that used inhalation as the exposure route, the most reliable (Tier 1 or Tier 2) analyses consistently showed a lack of increased tumor induction in the respiratory tract. The two Tier 2 studies that used intratracheal administration also were consistent in showing that there is no evidence of an increase in respiratory tumors relevant to human exposures. These results are consistent with the poor intracellular uptake of nickel metal particles (e.g. see Costa et al. Citation1981a, Citation1981b). There were a few Tier 3 studies with either inhalation or intratracheal administration, but their results are too unreliable to consider for evaluating causation. The results from mutagenicity tests indicate that metallic nickel particles did not induce gene mutations or micronuclei in vitro (Buxton et al. Citation2020). A Tier 3 study also evaluated chromosome aberrations but the results are not reliable. The results for induction of DNA strand breaks were positive in studies that were confounded by high cytotoxicity, based on guidelines for the extent of cytotoxicity in the evaluation of this endpoint (Tice et al. Citation2000; OECD Citation2014), with one study reporting mostly negative results in the absence of cytotoxicity (Latvala et al. Citation2016), though none of the studies of this endpoint included a comparison to historical controls. Studies of cellular transformation reported mixed results, with two studies reporting no transformation and two studies reporting weakly positive results. Overall, the only genotoxicity outcome with some consistency across in vitro studies is DNA strand breaks, with these results being positive primarily in the presence of cytotoxicity; however, in vitro studies do not take DNA repair into account, which could explain the lack of increased tumor induction in the respiratory tract in the experimental animal studies.
Magnitude
An evaluation of the magnitude of effects in the experimental animal studies is not warranted, given their consistency in showing a lack of increased respiratory tumor induction with metallic nickel exposure. The magnitude of the effects from in vitro genotoxicity studies are difficult to evaluate beyond reporting whether a result was statistically significant compared to the negative controls (and none of the studies reporting positive results included a comparison to historical controls), though comparison of test outcomes with a positive control can also provide an indication of magnitude. Some of the studies reporting positive, statistically significant effects did not report results for a positive control, however. For example, the statistically significant increase in DNA strand breaks reported by Assad et al. (Citation1999) was not compared to a positive control; however, results of evaluations of DNA strand breaks that were compared to a positive control were generally of a similar magnitude to the positive controls (e.g. Latvala et al. Citation2016; Magaye et al. Citation2016). By contrast, the study with positive results for cellular transformation that included positive controls reported that metallic nickel particles induced very weak transformation compared to these controls (Magaye et al. Citation2014). Overall, the magnitude of the in vitro effects is difficult to discern, though there is some evidence that the cellular transformation effects were weak, but this is not consistent with the lack of increased respiratory tumor induction with metallic nickel exposure in the experimental animal studies.
Exposure–response
An evaluation of exposure–response relationships in the experimental animal studies is not justified, given their consistency in showing a lack of increased respiratory tumor induction with metallic nickel exposure. Doses from the in vitro studies cannot be compared to those used in the experimental animal studies because the in vitro studies did not provide any analysis of the comparative dosimetry between the in vitro and in vivo studies. There are no reliable studies of metallic nickel genotoxicity in vivo to compare to the in vivo carcinogenicity studies, either. A comparison of the doses used in the in vitro genotoxicity studies to those used in the cellular transformation studies indicates that genotoxicity is observed in some cell types only at doses higher than those associated with weak transformation in other cell types. Some of the in vitro studies evaluated only a single dose of metallic nickel. One study that reported positive results across multiple doses showed a clear dose–response relationship but did not conduct any statistical analysis of the results (Hansen and Stern Citation1984).
Biological concordance
The evaluation of mutagenicity and genotoxicity of metallic nickel, whether a result of nickel ions directly interacting with DNA or an indirect effect resulting from nickel cytotoxicity, is consistent with the biological knowledge of carcinogenesis. It is not clear from the available mechanistic studies whether indirect damage to DNA from metallic nickel exposures can lead to carcinogenesis, though several plausible mechanisms for this have been hypothesized for nickel-containing substances. Inhalation of metallic nickel induces cytotoxicity and inflammation in the respiratory tract, which could conceivably lead to indirect genotoxicity; however, cytotoxicity and inflammation alone are not sufficient to induce lung tumors after metallic nickel exposure, as evidenced by the high degree of lung toxicity and inflammation but lack of an increased incidence of respiratory tumors observed in rats in the inhalation carcinogenicity study by Oller et al. (Citation2008).
Essentiality and specificity
The criteria of essentiality and specificity can be considered together when the apical effect is tumor development. None of the experimental studies of metallic nickel assessed the reversibility of effects if exposure was stopped, but it is known that cancer cells require multiple mutations in oncogenes and tumor suppressor genes to develop, and chemical exposures can also promote proliferation of these cells into tumors. If the exposure ceases such that the induction of mutations or the promotion of proliferation does not occur, it is possible that a tumor will not develop. Although not all substances that induce mutations in in vitro tests are carcinogens, the ability to cause mutations increases the likelihood for carcinogenicity. As discussed above, mutagenicity tests are more relevant to the evaluation of a mode of action for carcinogenicity than genotoxicity indicator tests. With regard to temporality, mutagenicity is known to be a key event that occurs prior to tumor development, though it can continue to occur in tumor cells at a high rate because of their inherent genomic instability.
Analogy
For this criterion, we compared what is known for other nickel compounds that have been shown to be carcinogenic in the respiratory tract in humans and experimental animals to our evaluation of metallic nickel. Insoluble nickel compounds, such as nickel subsulfide and nickel oxide, induce respiratory tumors in rodents and have been implicated as carcinogens in epidemiology studies of nickel refinery workers (Goodman et al. Citation2009, Citation2011). Nickel ions from these substances are more bioavailable at the nucleus of respiratory tract cells compared to metallic nickel, however, increasing the likelihood that they can interact with chromatin and induce mutations in DNA (Goodman et al. Citation2011). Although nickel ions from these and other nickel compounds have been shown to be only weakly genotoxic, as discussed above, the higher bioavailability of nickel ions from insoluble nickel compounds compared to the relatively lower bioavailability of nickel ions from metallic nickel is consistent with the lack of an increase in respiratory tumor induction in the experimental animal studies of metallic nickel.
Overall, the experimental animal and mechanistic studies indicate that the criterion of consistency is met in support of no causation, as there was a consistent lack of increased tumor induction in the experimental animal inhalation studies and a lack of mutagenicity in in vitro studies, even if indirect DNA damage could be induced. The lack of tumor induction with inhalation exposures does not allow for a true evaluation of magnitude and dose-response, and provides support that these criteria are not met for a positive tumor response and therefore support no causation. Although indirect, but not direct, effects of nickel ions from metallic nickel on DNA are possible, the lack of carcinogenicity in the inhalation studies and the lack of mutagenicity are supportive of no causation when considering biological concordance. Essentiality, specificity, and temporality would be met if there was consistent evidence of tumor induction in the inhalation studies, but the lack of such evidence does not inform these criteria. Based on this evaluation, not all of the criteria in for assessing the body of experimental studies for causation were met, and it is more likely that the experimental evidence supports a lack of causation.
Human relevance
In risk assessment, rodents are generally considered good models for carcinogenicity in humans, though not all carcinogenic substances induce tumors in both rodents and humans, either at all or at the same sites. There are significant differences between rodent and human respiratory tract physiology and pathogenesis of respiratory tumors, limiting the relevance of rodents as models for human respiratory cancers (Pandiri Citation2015). The positive results for respiratory tumors in cancer bioassays in rats with certain insoluble nickel compounds support the use of rat models for assessing nickel carcinogenicity in humans, however, as there is tumor site concordance in the respiratory tract between humans and rats after inhalation exposure to these compounds (e.g. see Goodman et al. Citation2009, Citation2011). As discussed above, hamsters may not be a good model for exposures to particulates, and they appeared to be less sensitive to the toxicity of metallic nickel in the studies reviewed here. Other evidence supports that hamsters, as well as mice, are less sensitive than rats to the inhalation carcinogenicity of metals, including nickel (Dunnick et al. Citation1995; Oller et al. Citation1997; Kasprzak et al. Citation2003).
The experimental animal studies with intratracheal administration, by themselves, are not relevant to humans, as this exposure route bypasses normal clearance mechanisms that occur with inhalation exposure, resulting in much higher deposition at the site of administration. We included these studies in our evaluation for completeness; however, it is important to note that these studies include exposure levels in the respiratory tract that cannot be achieved via physiological routes.
The exposures in the experimental animal studies were to pure nickel metal particles that were small in size, with most particles measured as having an MMAD of 2–3 μm. By contrast, exposures to nickel in occupational settings are usually to mixtures of nickel and nickel compounds, with particles of 2–3 μm MMAD comprising less than 5% of the measured inhalable aerosol (Hsieh et al. Citation1999). Based on modeling studies conducted with water-soluble and insoluble nickel compounds, human occupational exposures would need to be approximately 10 times higher than those tested in the inhalation studies in experimental animals to obtain an equivalent deposition of respirable nickel particles (Hsieh et al. Citation1999). The no observable adverse effect concentration (NOAEC) of 0.4 mg/m3 nickel metal in the chronic inhalation rat study by Oller et al. (Citation2008) was converted to a human equivalent concentration (HEC) range of 1.2–4.4 mg/m3, as described by ECHA (Citation2017). Occupational exposures to metallic nickel in the cohort studies reviewed here were estimated to be in the range of 0–5.25 mg/m3 (as discussed by Goodman et al. Citation2011), which encompasses the range of exposures in the rat study. This indicates that the workers were exposed to metallic nickel concentrations high enough to obtain an equivalent deposition of respirable particles as the exposed rats, yet neither the rats nor the workers had an increased risk of respiratory tumors.
The inhalation study in experimental animals by Oller et al. (Citation2008) reported a statistically significant increase in adrenal pheochromocytomas in male rats exposed to 0.4 mg/m3 nickel metal. These tumors were not considered to be a direct effect of nickel on the adrenal gland, however, as they were considered to be secondary to lung toxicity and hypoxemia caused by the particulate nickel exposure, and they were not observed in a cancer bioassay with oral exposure to nickel sulfate hexahydrate that resulted in higher measured internal exposure to nickel ions due to greater systemic absorption of water-soluble nickel compounds compared to less soluble forms (Heim et al. Citation2007). Adrenal pheochromocytomas are frequently observed in male rats after inhalation exposures to particulates (Ozaki et al. Citation2002) and are not considered to be relevant to human occupational exposures, as there is no indication that substances inducing adrenal pheochromocytomas in rats also induce these tumors in humans (Greim et al. Citation2009). None of the epidemiology studies of workers exposed to metallic nickel evaluated incidence or mortality from adrenal tumors specifically, though two cohort studies evaluated cancers of the endocrine glands combined (not including the thyroid). These studies reported no cases of cancers of the endocrine glands among workers that manufactured nickel alloys (Sorahan Citation2004) and workers in a nickel carbonyl refinery (Sorahan and Williams Citation2005), providing some evidence of a lack of site concordance for adrenal tumors from metallic nickel exposure across rats and humans.
The in vitro studies of metallic nickel genotoxicity, mutagenicity, and transformation are of very limited relevance to humans. The cell lines used in these studies were from a number of different species and tissue types, and most were conducted in nonepithelial cells, limiting their relevance to the induction of respiratory tumors, which occur in epithelial cells. Some were conducted in human lung alveolar epithelial adenocarcinoma cells, which are malignant cells with a high spontaneous mutation rate and, therefore, are not relevant to an evaluation of genotoxicity in nonmalignant cells.
Cell culture conditions allow for high exposure concentrations with no clearance mechanisms, increasing cellular uptake of nickel particles and allowing nickel ions to reach the cell nucleus, when in vivo this would be precluded by particle clearance. To achieve such high concentrations of nickel ions in respiratory tract cells in vivo would require exposure to high concentrations of nickel particles, above the MTD, that would likely result in severe toxicity. None of the in vitro studies discussed the relevance of the doses used to human inhalation exposures in the workplace.
Overall, the experimental animal studies that used inhalation as the exposure route are relevant to humans in that they used a relevant exposure route and exposure concentrations in an animal model that is sensitive to carcinogenicity from particulate exposures. The in vitro mechanistic studies are less relevant to humans, as they were conducted in artificial conditions that cannot replicate the conditions of particle clearance and uptake in the respiratory tract of humans exposed by inhalation, and there are no reliable in vivo studies of genotoxicity. Thus, we consider the available evidence from the experimental studies to be inadequate to determine whether all of the criteria listed in for assessing human relevance can be met.
Confidence in biological plausibility
We applied our conclusions regarding causation and human relevance of the experimental studies to assess confidence in the biological plausibility of respiratory cancers as a consequence of metallic nickel exposure in humans. Our conclusion is that the experimental evidence supports a lack of causation, but it is unclear how relevant the in vitro studies are to humans, so we can only categorize the experimental evidence as inadequate for fully assessing human relevance. Based on this conclusion for the experimental evidence and the scheme in , there is moderate overall confidence that exposure to metallic nickel as a cause of respiratory cancer in humans is not biologically plausible.
Evidence integration across realms
We evaluated the epidemiology evidence using modified Bradford Hill aspects (), and used the experimental evidence to provide information on coherence and biological plausibility.
Consistency
An association between occupational nickel exposure in refineries and elevated lung cancer risk has been established for decades (Goodman et al. Citation2009, Citation2011). However, studies evaluating cohorts of workers exposed predominantly to metallic nickel, with or without some co-exposure to oxidic nickel and other metals, consistently indicate a lack of excess incidence or mortality for lung, laryngeal, and nasal/sinonasal cancers and all respiratory cancers combined. This is true across cohorts, as well as type of industry, including the only study to evaluate exposures to metallic nickel without any co-exposures to other forms of nickel (Cragle et al. Citation1984). Overall, the aspect of consistency is not met for positive associations between metallic nickel exposure and respiratory cancers.
Strength
The epidemiology studies reported mostly null results, with several non-statistically significant decreases in risk and some non-statistically significant increases in risk reported for certain respiratory cancers. Though a few epidemiology studies reported statistically significant, positive associations with lung cancer and all respiratory cancers combined in certain analyses (Arena et al. Citation1998; Svartengren et al. Citation2017; Westberg et al. Citation2017; Marsh et al. Citation2017b), these were not adjusted for important potential confounders such as smoking or other occupational exposures, and they were determined to be unrelated to the occupational exposure. Overall, the aspect of strength is not met for positive associations between metallic nickel exposure and respiratory cancers.
Coherence
A lack of clear evidence for associations between metallic nickel exposure and respiratory cancers in the epidemiology studies reviewed here is consistent with the lack of respiratory tumors observed in chronic inhalation studies in experimental animals, and with the lack of mutagenicity and weak cellular transformation capacity of metallic nickel in vitro, even though these latter studies are of limited relevance to humans. Overall, the aspect of coherence is met for a lack of an association (but not for a positive association) between exposure to metallic nickel and respiratory cancers.
Biological plausibility
As indicated above, our evaluation of the experimental evidence supports a lack of causation, but it is unclear how relevant the in vitro studies are to humans, so we can only categorize the experimental evidence as inadequate for fully assessing human relevance. Because of this, the overall confidence for biological plausibility is moderate, but for a lack of biological plausibility, as the evidence strongly supports that respiratory carcinogenicity of metallic nickel is not plausible. Based on this conclusion, the aspect of biological plausibility is not met for positive associations between metallic nickel exposure and respiratory cancers.
Biological gradient
Exposure–response relationships were examined for lung cancer in four studies by conducting statistical testing for trend (Sorahan Citation2004; Marsh et al. Citation2017a, Citation2017b; Morfeld et al. Citation2017). None of these analyses indicated a statistically significant exposure–response relationship between duration of exposure, cumulative exposure, or exposure intensity and lung cancer mortality. Exposure-response relationships were not evaluated for laryngeal or nasal/sinonasal cancer, though most effect estimates for laryngeal cancer were below the null, and there were no reported cases of nasal/sinonasal cancer in the studied cohorts. Overall, the aspect of biological gradient is not met for positive associations between metallic nickel exposure and respiratory cancers.
Temporality
It is evident in all of the reviewed studies that the metallic nickel exposure preceded the outcome, and in 11 of the 16 cohort studies, the authors considered a clear and reasonable lag period (e.g. at least 10 years) to ensure the relevance of the outcome under examination, which is necessary for making causal inferences for respiratory cancer studies. Thus, the aspect of temporality for associations between metallic nickel exposure and respiratory cancers is partially met.
Specificity
Evidence that links a specific exposure to a specific outcome can strengthen a causal inference, but any given outcome may have multiple causes. None of the respiratory cancers examined in this analysis are specific to exposure to nickel (in any form). As discussed above, smoking and several occupational exposures, including those that can be co-exposures in workplaces with nickel exposure (e.g. asbestos, chromium, wood dust) are known risk factors for respiratory cancers. The majority of the effect estimates in the reviewed studies did not adjust for these important potential confounders, though overall the studies do not provide evidence of excess risks of respiratory cancers. Thus, the aspect of specificity for associations between metallic nickel exposure and respiratory cancers is not met.
Analogy
Other chemical forms of nickel have been implicated as respiratory carcinogens in both occupational studies of nickel refinery workers as well as in chronic carcinogenicity assays in experimental animals. These studies indicate consistent, increased respiratory cancer risks with exposure to the insoluble sulfidic and oxidic nickel compounds, indicating site concordance across experimental animals and humans. By contrast, metallic nickel has not been clearly shown to be a respiratory carcinogen in human or experimental animal studies. Overall, the aspect of analogy for associations between metallic nickel exposure and respiratory cancers is not met.
Experiment
There are no available studies reporting the results of natural experiments specifically for metallic nickel exposure, but one study addressed exposure to multiple forms of nickel in a refinery. Workers in the Mond/INCO nickel carbonyl refinery in Clydach, Wales experienced a significant reduction in exposure to occupational dust containing four nickel species since 1902, and particularly after the 1950s, as a result of technical improvements in operational processes. By contrasting workers' lung cancer and nasal cancer mortality by decades of first employment, Grimsrud and Peto (Citation2006) demonstrated that the cancer hazards reduced significantly from 1902 to the 1930s, resulting in substantial reductions of cancer risk among workers first employed in later periods. As this analysis was not specific to metallic nickel exposure, however, the aspect of natural experiments for associations between metallic nickel exposure and respiratory cancers is not met.
Causal conclusion
We used the four-tiered framework shown in to form a causal conclusion regarding the evidence for the human respiratory carcinogenicity of metallic nickel. The vast majority of the Bradford Hill aspects were not met for positive associations between metallic nickel exposure and respiratory cancers; rather, most were indicative of a lack of an association. Thus, the evidence as a whole supports no causation, and we conclude that the relationship between metallic nickel exposure and respiratory cancer in humans is not causal.
Discussion
We conducted a systematic review of the potential human respiratory carcinogenicity of metallic nickel and concluded that any relationship between metallic nickel exposure and respiratory cancer in humans is not causal. A strength of our analysis is that we used an approach that was based on NTP OHAT guidance for systematic reviews, including the use of the OHAT RoB Rating Tool to evaluate study quality and RoB across all studies prior to evaluating their results. The OHAT RoB Rating Tool was developed using recent guidance from multiple organizations, including the Agency for Healthcare Research and Quality, the CLARITY Group at McMaster University, Cochrane, and the Navigation Guide, as well as comments from technical advisors, the public, and staff at other federal agencies (NTP Citation2015). We slightly modified the RoB criteria for epidemiology and experimental animal studies to be specific to studies evaluating respiratory cancer from metallic nickel exposures, and we added RoB criteria for in vitro studies based on key data elements for in vitro studies specified by NTP OHAT (NTP Citation2019) and on study quality criteria for in vitro studies described by other organizations (Lynch et al. Citation2016; US EPA Citation2018; Stockholm University, Karolinska Institutet Citation2019). Another strength is that we evaluated and integrated the evidence from studies of metallic nickel and formed a causal conclusion in a manner that was consistent with best practices for evaluating, synthesizing, and integrating evidence across scientific disciplines and making causal determinations, as described by a number of sources (Boobis et al. Citation2008; IOM Citation2008; Rhomberg et al. Citation2013; Meek et al. Citation2014; US EPA Citation2018, Citation2019).
Several epidemiology studies have evaluated carcinogenicity in refinery workers with exposures to different combinations of several forms of nickel (e.g. metallic, oxidic, sulfidic, and water-soluble nickel compounds) (Enterline and Marsh Citation1982; Easton et al. Citation1992; Grimsrud et al. Citation2003; Seilkop et al. Citation2017; Pavela et al. Citation2017). We summarized the results of these studies in Supplemental Table S6. We also summarized the results of eight case–control studies of patients with occupational histories that were assumed to have included exposure to metallic nickel, but the particular forms of nickel exposures were not specified (Supplemental Table S7). We did not include these studies in our main analysis, as such mixed or unknown exposures are less relevant for evaluating health effects that are attributable to metallic nickel specifically. Together, these refinery and case–control studies reported no increased risks of laryngeal cancer and mixed (both positive and null) results for lung, nasal, and respiratory cancers combined. While it cannot be known whether positive results in these studies of mixed or unknown exposures to different forms of nickel can be attributable to metallic nickel specifically, the null results provide support that metallic nickel, in a mixture with other forms of nickel, did not increase the risk of respiratory cancers.
As discussed above, cross-classification analyses of some of the refinery studies have suggested that there is no evidence that metallic nickel exposure increased lung cancer risk (ICNCM Citation1990). Similarly, Easton et al. (Citation1992) modeled lung cancer risks for exposure to the different forms of nickel for refinery workers hired in 1930 or earlier, finding no statistically significant associations (p = 0.10) with water-soluble and metallic nickel. Even so, when the authors applied their model to workers hired after 1930, lung cancers were substantially overpredicted in long-term workers, leading to the conclusion that they may have overestimated risks from metallic and water-soluble nickel and underestimated risks from sulfidic and oxidic nickel. In a study investigating the relationship between exposure to different forms of nickel and lung cancer risk in nickel refinery workers in Norway, Grimsrud et al. (Citation2002) concluded that there was little (if any) evidence of an association between metallic nickel exposure and lung cancer. Overall, the results of the studies of mixed exposures to several different forms of nickel are consistent with the results of our main analysis that metallic nickel exposure is not associated with respiratory carcinogenicity in humans.
Our evaluation indicates that the epidemiology and experimental animal studies of metallic nickel exposure do not support an increased risk of respiratory cancers, and the in vitro studies show some evidence for DNA strand breaks and weak cellular transformation in various cultured cell types, but no strong evidence for other genotoxic effects, including mutagenicity. Direct genotoxicity requires nickel ions from metallic nickel exposures to enter the cell nucleus and interact with DNA. The particle size, respiratory toxicity, clearance, cellular uptake, and dissolution of nickel particles determine the bioavailability of nickel ions at the nucleus of respiratory tract cells (Goodman et al. Citation2011). Compared to insoluble nickel compounds that have been shown to induce respiratory tract tumors in nickel refinery workers and experimental animals (e.g. sulfidic and oxidic nickel compounds), metallic nickel has a relatively high toxicity and an intermediate retention half-time in the lungs (Goodman et al. Citation2011). Metallic nickel is not readily taken up by cells (Costa et al. Citation1981a, Citation1981b), though once inside cells, an increase in the release of nickel ions from metallic nickel particles is possible under acidic conditions. The lack of respiratory tumor induction in rats with inhalation exposure to metallic nickel (Oller et al. Citation2008) indicates that the overall nickel ion bioavailability must have been below the threshold for carcinogenicity, even though lung cytotoxicity and inflammation were present, indicating that they are necessary but not sufficient for induction of lung tumors. The lung burden of nickel metal powder at the MTD in this study was 6-fold higher than the minimal lung burden resulting in respiratory tumors in rats exposed chronically to nickel subsulfide (NTP Citation1996b); thus, the low cellular uptake and intracellular dissolution of metallic nickel may be the driving factors for its lack of carcinogenicity (Goodman et al. Citation2011).
Although the bioavailability of nickel ions in the nucleus of respiratory tract cells is expected to be low after exposures to metallic nickel, it is still possible that metallic nickel could exert indirect genotoxicity through mechanisms that do not require nickel ions to enter the nucleus. Several mechanisms have been proposed, with some supporting evidence from in vitro studies with various nickel compounds and metallic nickel. These studies have limited relevance to humans, however, as there is no clearance in vitro, which can increase the interaction of nickel particles with cell membranes or the uptake of nickel particles into cells. The lack of carcinogenicity of metallic nickel in vivo (even in the presence of a high degree of lung toxicity and inflammation) strongly suggests that these mechanisms are only relevant in vitro and may not be relevant in humans, making it highly unlikely that metallic nickel is genotoxic by any mechanism.
Our analysis of the in vitro mechanistic evidence for metallic nickel carcinogenicity highlights the importance of a systematic evaluation of the literature for mechanistic evidence that considers the quality and RoB, as well as the relevance of each study, and is integrated with the human and experimental animal evidence to support a causal conclusion. Simply noting that a substance can induce certain changes in cells that are consistent with mechanistic pathways associated with carcinogenesis or tumor progression does not provide strong evidence of carcinogenicity. An example of this is IARC's "key characteristics of carcinogens" approach to evaluating mechanistic evidence for carcinogenicity (Smith et al. Citation2016; Guyton et al. Citation2018). This approach involves a set of 10 characteristics that are common to known human carcinogens. If a study of a substance has a positive finding for one of these characteristics, one may conclude that it supports some level of evidence for that characteristic. Although metallic nickel has been shown to have some of the 10 characteristics in certain (mostly in vitro) studies (e.g. some types of genotoxicity, induction of oxidative stress), it is not apparent until one evaluates the quality and relevance of these studies, and how they inform what is known from the human and experimental animal evidence (and vice versa), that these positive results do not support metallic nickel as a human carcinogen (Goodman and Lynch Citation2017).
The evidence evaluated in this analysis was strong enough to support metallic nickel as not likely to be a human respiratory carcinogen, but there are several areas for which additional data would help improve our understanding of the carcinogenic potential of metallic nickel. Genotoxicity of metallic nickel in vivo is not well studied. Additional studies of mutagenicity and genotoxicity in experimental animals with inhalation exposures to metallic nickel could assess the relevance of the in vitro results and/or explain the lack of respiratory carcinogenicity observed in vivo with inhalation exposures. Finally, the current epidemiology data are limited, as most cohorts were exposed to several forms of nickel, making it difficult to tease out the contribution of metallic nickel to cancer risk. For cohorts exposed predominantly to metallic nickel, the sizes of the cohorts were small and the exposures were generally low, and there are no human data at higher exposures.
Conclusions
We conducted a systematic review of the potential carcinogenicity of metallic nickel, focusing on cancers of the respiratory tract. We evaluated the quality and RoB of the relevant epidemiology, experimental animal, and in vitro mechanistic studies and critically assessed whether metallic nickel should be considered a human respiratory carcinogen. Our evaluation of the epidemiology studies indicates that there is no substantive evidence of increased respiratory cancer risk in workers exposed predominantly to metallic nickel. The experimental animal evidence indicates that metallic nickel does not increase the incidence of respiratory tumors in rodents exposed by inhalation. The in vitro studies have little relevance to humans, as they bypass normal clearance mechanisms and do not consider the low bioavailability of nickel ions from metallic nickel particles in the cell nucleus. Nevertheless, the mechanistic evidence indicates that metallic nickel can induce DNA strand breaks under certain conditions in vitro, but is not mutagenic, suggesting that the concentrations of metallic nickel achieved in vivo are below the threshold for DNA damage or that any DNA damage induced by metallic nickel is repaired. After integrating the evidence from all of these study types, we conclude that there is no causal relationship between metallic nickel exposure and respiratory cancer in humans.
Supplemental Material
Download PDF (619.1 KB)Acknowledgments
The authors thank Dr Lorenz R. Rhomberg of Gradient, Mr Steven K. Seilkop of SKS Consulting Services, Dr Adriana Oller of NiPERA, Inc., and Dr Samuel Buxton of NiPERA, Inc. for their insightful comments on a draft of this manuscript. The authors also thank Ms Carla Walker of Gradient for her editorial assistance. The authors gratefully acknowledge the valuable comments and suggestions of the two individuals selected by the editor to review the manuscript, who were anonymous to the authors.
Declaration of interest
The employment affiliations of the authors are shown on the cover page. The work reported in this paper was conducted by the Gradient authors during their normal course of employment, with financial support by NiPERA, Inc. through a contract with Gradient. NiPERA, Inc. is the independently incorporated science division of the Nickel Institute (a global association of primary nickel producers) that supports scientific research for and promotes general awareness of the safe production, use, and disposal of nickel (www.nickelinstitute.org/science).
P. Boffetta was asked to contribute to the writing of this paper by the authors at Gradient. Funds were provided to P. Boffetta by NiPERA, Inc. through a subcontract with Gradient.
J.E. Goodman has previously conducted work on nickel exposure and cancer risk in the context of litigation, but that work was not specific to metallic nickel and did not influence the work presented here. J. E. Goodman and R. L. Prueitt have previously given presentations or testimony on topics related to nickel toxicology (but not specifically metallic nickel carcinogenicity) at scientific conferences and meetings with regulatory agencies, with funding provided by NiPERA, Inc. All other authors declare that they have not been involved in any legal, regulatory, or advocacy activities related to the contents of this paper.
This paper was conceived, designed, and written solely by the authors. A draft of this paper was reviewed by Dr Adriana Oller and Dr Samuel Buxton of NiPERA, Inc. and Mr Steven K. Seilkop (as a consultant on behalf of NiPERA, Inc.) for clarity, and these individuals provided helpful suggestions and edits. Their review of the paper was initiated by a request from the authors at Gradient, at the time that the project was proposed to NiPERA, Inc. This manuscript is the professional work product of the authors, and the opinions and conclusions offered within are not necessarily those of their employers or the financial sponsor of the work.
Supplemental material
Supplemental material for this article is available online here
Correction Statement
This article has been corrected with minor changes. These changes do not impact the academic content of the article.
References
- Abbracchio MP, Heck JD, Caprioli RM, Costa M. 1981. Differences in surface properties of amorphous and crystalline metal sulfides may explain their toxicological potency. Chemosphere. 10(8):897–908.
- Abbracchio MP, Heck JD, Costa M. 1982. The phagocytosis and transforming activity of crystalline metal sulfide particles are related to their negative surface charge. Carcinogenesis. 3(2):175–180.
- Agency for Toxic Substances and Disease Registry [ATSDR]. 2005. Toxicological Profile for Nickel (Final). 397p.
- Ahamed M, Alhadlaq HA. 2014. Nickel nanoparticle-induced dose-dependent cyto-genotoxicity in human breast carcinoma MCF-7 cells. Onco Targets Ther. 7:269–280.
- Allemani C, Matsuda T, Di Carlo V, Harewood R, Matz M, Niksic M, Bonaventure A, Valkov M, Johnson CJ, Esteve J, et al. 2018. Global surveillance of trends in cancer survival 2000-14 (CONCORD-3): analysis of individual records for 37,513,025 patients diagnosed with one of 18 cancers from 322 population-based registries in 71 countries. Lancet. 391(10125):1023–1075.
- American Conference of Governmental Industrial Hygienists [ACGIH]. 2001. Documentation for nickel and inorganic compounds (CAS No. 7440-02-0), including nickel subsulfide (CAS No. 12035-72-2). In: American Conference of Governmental Industrial Hygienists (ACGIH). Documentation of the Threshold Limit Values and Biological Exposure Indices (Seventh Edition).
- Arena VC, Sussman NB, Redmond CK, Costantino JP, Trauth JM. 1998. Using alternative comparison populations to assess occupation-related mortality risk. Results for the high nickel alloys workers cohort. J Occup Environ Med. 40(10):907–916.
- Assad M, Lemieux N, Rivard CH, Yahia LH. 1999. Comparative in vitro biocompatibility of nickel-titanium, pure nickel, pure titanium, and stainless steel: genotoxicity and atomic absorption evaluation. Biomed Mater Eng. 9(1):1–12.
- Begley CG, Ioannidis JPA. 2015. Reproducibility in science: improving the standard for basic and preclinical research. Circ Res. 116(1):116–126.
- Benson JM, Brooks AL, Henderson RF. 1992. Comparative in vitro cytotoxicity of nickel compounds to pulmonary alveolar macrophages and rat lung epithelial cells. In: Nieboer E, Nriagu JO, editors. Nickel and human health: current perspectives. New York: John Wiley & Sons, Inc.; p. 319–330.
- Beyersmann D, Hartwig A. 2008. Carcinogenic metal compounds: recent insight into molecular and cellular mechanisms. Arch Toxicol. 82(8):493–512.
- Boobis AR, Doe JE, Heinrich-Hirsch B, Meek ME, Munn S, Ruchirawat M, Schlatter J, Seed J, Vickers C. 2008. IPCS framework for analyzing the relevance of a noncancer mode of action for humans. Crit Rev Toxicol. 38(2):87–96.
- Brackbill R, Frazier T, Shilling S. 1988. Smoking characteristics of US workers, 1978–1980. Am J Ind Med. 13(1):5–41.
- Buekers J, De Brouwere K, Lefebvre W, Willems H, Vandenbroele M, Van Sprang P, Eliat-Eliat M, Hicks K, Schlekat CE, Oller AR. 2015. Assessment of human exposure to environmental sources of nickel in Europe: inhalation exposure. Sci Total Environ. 521–522:359–371.
- Buxton S, Garman E, Heim KE, Lyons-Darden T, Schlekat CE, Taylor MD, Oller AR. 2019. Concise review of nickel human health toxicology and ecotoxicology. Inorganics. 7(7):89.
- Buxton S, Voges Y, Donath C, Oller A. 2020. Gene (HPRT) and chromosomal (MN) mutations of nickel metal powder in V79 Chinese hamster cells. Mutat Res. 819–820:111688.
- Cataldo JK, Dubey S, Prochaska JJ. 2010. Smoking cessation: an integral part of lung cancer treatment. Oncology. 78(5–6):289–301.
- Costa M, Abbracchio MP, Simmons-Hansen J. 1981a. Factors influencing the phagocytosis, neoplastic transformation, and cytotoxicity of particulate nickel compounds in tissue culture systems. Toxicol Appl Pharmacol. 60(2):313–323.
- Costa M, Davidson TL, Chen H, Ke Q, Zhang P, Yan Y, Huang C, Kluz T. 2005. Nickel carcinogenesis: epigenetics and hypoxia signaling. Mutat Res. 592(1–2):79–88.
- Costa M, Heck JD. 1982. Specific nickel compounds as carcinogens. Trends Pharmacol Sci. 3:408–410.
- Costa M, Heck JD, Robison SH. 1982. Selective phagocytosis of crystalline metal sulfide particles and DNA strand breaks as a mechanism for the induction of cellular transformation. Cancer Res. 42(7):2757–2763.
- Costa M, Mollenhauer HH. 1980a. Carcinogenic activity of particulate nickel compounds is proportional to their cellular uptake. Science. 209(4455):515–517.
- Costa M, Mollenhauer HH. 1980b. Phagocytosis of nickel subsulfide particles during the early stages of neoplastic transformation in tissue culture. Cancer Res. 40(8 Pt 1):2688–2694.
- Costa M, Simmons-Hansen J, Bedrossian CW, Bonura J, Caprioli RM. 1981b. Phagocytosis, cellular distribution, and carcinogenic activity of particulate nickel compounds in tissue culture. Cancer Res. 41(7):2868–2876.
- Cragle DL, Hollis DR, Newport TH. 1984. A retrospective cohort mortality study among workers occupationally exposed to metallic nickel powder at the Oak Ridge Gaseous Diffusion Plant. In: Sunderman FW, editor. IARC Scientific Publication No. 53. Nickel in the Human Environment: Proceedings of a Joint Symposium held at IARC, Lyon, France, 8–11 March 1983. Lyon: International Agency for Research on Cancer (IARC); p. 57–63.
- Das KK, Das SN, Dhundasi SA. 2008. Nickel, its adverse health effects and oxidative stress. Indian J Med Res. 128(4):412–425.
- De Brouwere K, Buekers J, Cornelis C, Schlekat CE, Oller AR. 2012. Assessment of indirect human exposure to environmental sources of nickel: oral exposure and risk characterization for systemic effects. Sci Total Environ. 419:25–36.
- Dunnick JK, Elwell MR, Radovsky AE, Benson JM, Hahn FF, Nikula KJ, Barr EB, Hobbs CH. 1995. Comparative carcinogenic effects of nickel subsulfide, nickel oxide, or nickel sulfate hexahydrate chronic exposures in the lung. Cancer Res. 55(22):5251–5256.
- Eastmond DA, Hartwig A, Anderson D, Anwar WA, Cimino MC, Dobrev I, Douglas GR, Nohmi T, Phillips DH, Vickers C. 2009. Mutagenicity testing for chemical risk assessment: update of the WHO/IPCS Harmonized Scheme. Mutagenesis. 24(4):341–349.
- Easton DF, Peto J, Morgan LG, Metcalfe LP, Usher V, Doll R. 1992. Respiratory cancer mortality in Welsh nickel refiners: which nickel compounds are responsible? In: Nieboer E, Nriagu JO, editors. Nickel and human health: perspectives. New York: John Wiley & Sons, Inc.; p. 603–619.
- Egedahl R, Carpenter M, Lundell D. 2001. Mortality experience among employees at a hydrometallurgical nickel refinery and fertiliser complex in Fort Saskatchewan, Alberta (1954–95). Occup Environ Med. 58(11):711–715.
- Egedahl RD, Collins MJ. 2009. Vital status of Sherritt nickel refinery workers (1954–2003). In: Budac J, Fraser R, Mihaylov I, Papangelakis V, Robinson D, editors. Hydrometallurgy of Nickel and Cobalt: Proceedings of 39th Annual Hydrometallurgy Meeting held in in conjunction with the 48th Annual Conference of Metallurgists, Montreal, Canada. Montreal, Canada: Canadian Institute of Mining, Metallurgy and Petroleum; p. 689–699.
- European Chemicals Agency [ECHA]. 2019. Summary of classification and labeling for nickel (CAS No. 7440-02-0). [accessed 2019 October 30] https://echa.europa.eu/information-on-chemicals/cl-inventory-database/-/discli/details/133816
- Enterline PE, Marsh GM. 1982. Mortality among workers in a nickel refinery and alloy manufacturing plant in West Virginia. J Natl Cancer Inst. 68(6):925–933.
- European Chemicals Agency [ECHA]. 2017. Appendix C.2: Background Document in Support of Long-term Inhalable DNELs for Nickel Metal and Nickel Compounds. In: Reach dossier for nickel (CAS No. 7440-02-0). 57p. [accessed 2020 June 5]. https://echa.europa.eu/registration-dossier/-/registered-dossier/15544/7/1
- European Chemicals Agency [ECHA]. 2018. Annex 1 Background Document in support of the Committee for Risk Assessment (RAC) for evaluation of limit values for nickel and its compounds in the workplace. Committee for Risk Assessment (RAC), ECHA/RAC/A77-0-0000001412-86-189/F. 212p.
- Evans RM, Davies PJ, Costa M. 1982. Video time-lapse microscopy of phagocytosis and intracellular fate of crystalline nickel sulfide particles in cultured mammalian cells. Cancer Res. 42(7):2729–2735.
- Fornalski KW, Dobrzyński L. 2010. The healthy worker effect and nuclear industry workers. Dose Response. 8(2):125–147.
- Goodman J, Lynch H. 2017. Improving the international agency for research on cancer's consideration of mechanistic evidence. Toxicol Appl Pharmacol. 319:39–46.
- Goodman JE, Prueitt RL, Dodge DG, Thakali S. 2009. Carcinogenicity assessment of water-soluble nickel compounds. Crit Rev Toxicol. 39(5):365–417.
- Goodman JE, Prueitt RL, Harbison RD, Johnson GT. 2020. Systematically evaluating and integrating evidence in National Ambient Air Quality Standards (NAAQS) reviews. Glob Epidemiol. 2:1000019.
- Goodman JE, Prueitt RL, Thakali S, Oller AR. 2011. The nickel ion bioavailability model of the carcinogenic potential of nickel-containing substances in the lung. Crit Rev Toxicol. 41(2):142–174.
- Greim H, Hartwig A, Reuter U, Richter-Reichhelm HB, Thielmann HW. 2009. Chemically induced pheochromocytomas in rats: mechanisms and relevance for human risk assessment. Crit Rev Toxicol. 39(8):695–718.
- Grimsrud TK, Berge SR, Haldorsen T, Andersen A. 2002. Exposure to different forms of nickel and risk of lung cancer. Am J Epidemiol. 156(12):1123–1132.
- Grimsrud TK, Berge SR, Martinsen JI, Andersen A. 2003. Lung cancer incidence among Norwegian nickel-refinery workers 1953–2000. J Environ Monit. 5(2):190–197.
- Grimsrud TK, Peto J. 2006. Persisting risk of nickel related lung cancer and nasal cancer among Clydach refiners. Occup Environ Med. 63(5):365–366.
- Guyton KZ, Rusyn I, Chiu WA, Corpet DE, van den Berg M, Ross MK, Christiani DC, Beland F, Smith MT. 2018. Application of the key characteristics of carcinogens in cancer hazard identification. Carcinogenesis. 39(4):614–622.
- Hansen K, Stern RM. 1984. Toxicity and transformation potency of nickel compounds in BHK cells in vitro. In: Sunderman FW, editor. IARC Scientific Publication No. 53. Nickel in the Human Environment: Proceedings of a Joint Symposium held at IARC, Lyon, France, 8–11 March 1983. Lyon, France: International Agency for Research on Cancer (IARC); p. 193–200.
- Hansen KS, Lauritsen JM, Skytthe A. 1996. Cancer incidence among mild steel and stainless steel welders and other metal workers. Am J Ind Med. 30(4):373–382.
- Heck JD, Costa M. 1982. Surface reduction of amorphous NiS particles potentiates their phagocytosis and subsequent induction of morphological transformation in Syrian hamster embryo cells. Cancer Lett. 15(1):19–26.
- Heim KE, Bates HK, Rush RE, Oller AR. 2007. Oral carcinogenicity study with nickel sulfate hexahydrate in Fischer 344 rats. Toxicol Appl Pharmacol. 224(2):126–137.
- Hsieh TH, Yu CP, Oberdorster G. 1999. Modeling of deposition and clearance of inhaled Ni compounds in the human lung. Regul Toxicol Pharmacol. 30(1):18–28.
- Hueper WC. 1958. Experimental studies in metal cancerigenesis: IX. Pulmonary lesions in guinea pigs and rats exposed to prolonged inhalation of powdered metallic nickel. AMA Arch Pathol. 65(6):600–607.
- Hueper WC, Payne WW. 1962. Experimental studies in metal carcinogenesis. Chromium, nickel, iron, arsenic. Arch Environ Health. 5:445–462.
- Huvinen M, Pukkala E. 2013. Cancer incidence among Finnish ferrochromium and stainless steel production workers in 1967–2011: a cohort study. BMJ Open. 3(11):e003819.
- International Agency for Research on Cancer [IARC]. 1990. IARC Monographs on the Evaluation of Carcinogenic Risks to Humans: Volume 49. Chromium, Nickel, and Welding. Lyon, France: International Agency for Research on Cancer; Geneva, Switzerland: World Health Organization (WHO). IARC Monograph No. 49. 677p.
- International Agency for Research on Cancer [IARC]. 2012. IARC Monographs on the Evaluation of Carcinogenic Risks to Humans Volume 100: A Review of Human Carcinogens. Part C: Arsenic, Metals, Fibres, and Dusts. International Agency for Research on Cancer (IARC); World Health Organization (WHO). IARC Monograph No. 100C. 524p. [accessed 2011 December 12]. http://monographs.iarc.fr/ENG/Monographs/vol100C/mono100C.pdf.
- International Committee on Nickel Carcinogenesis in Man [ICNCM]. 1990. Report of the International Committee on Nickel Carcinogenesis in Man. Scand J Work Environ Health. 16(1):1–82.
- Institute of Medicine [IOM], Committee on Evaluation of the Presumptive Disability Decision-Making Process for Veterans, Board on Military and Veterans Health. 2008. Improving the Presumptive Disability Decision-Making Process for Veterans. Washington, DC: National Academies Press, 781p. [accessed 2009 October 9]. http://books.nap.edu/openbook.php?record_id=11908.
- Ivankovic S, Zeller WJ, Komitowski D, Elder L, Lehmann E, Froehlich N. 1988. Carcinogenesis of Nickel Alloys in the Hamster Following Intratracheal Administration. [English translation]. Dortmund, Germany: Publication of the Bundesanstalt für Arbeitsschutz; p. 105.
- Jakobsson K, Mikoczy Z, Skerfving S. 1997. Deaths and tumours among workers grinding stainless steel: a follow up. Occup Environ Med. 54(11):825–829.
- Jayakrishnan TT, White RJ, Greenberg L, Colonias A, Wegner RE. 2020. Predictors of chemotherapy and its effects in early stage squamous cell carcinoma of the larynx. Laryngoscope Investig Otolaryngol. 5(3):445–452.
- Kasprzak KS, Sunderman FW, Salnikow K. 2003. Nickel carcinogenesis. Mutat Res. 533(1–2):67–97.
- Koedrith P, Seo YR. 2011. Advances in carcinogenic metal toxicity and potential molecular markers. Int J Mol Sci. 12(12):9576–9595.
- Krewski DK, Rice JM, Bird M, Milton B, Collins B, Lajoie P, Billard M, Grosse Y, Cogliano VJ, Caldwell JC. 2019. Analysis of tumour site concordance. In: Baan RA, Stewart BW, Straif K, editors. IARC Scientific Publication No. 165. Tumour Site Concordance and Mechanisms of Carcinogenesis. International Agency for Research on Cancer (IARC). Geneva: World Health Organization (WHO); p. 211–256.
- Latvala S, Hedberg J, Di Bucchianico S, Moller L, Wallinder IO, Elihn K, Karlsson HL. 2016. Nickel release, ROS generation and toxicity of Ni and NiO micro- and nanoparticles. PLoS One. 11(7):e0159684.
- Li CY, Sung FC. 1999. A review of the healthy worker effect in occupational epidemiology. Occup Med (Lond). 49(4):225–229.
- Lynch HN, Goodman JE, Tabony JA, Rhomberg LR. 2016. Systematic comparison of study quality criteria. Regul Toxicol Pharmacol. 76:187–198.
- Macleod M, Mohan S. 2019. Reproducibility and rigor in animal-based research. Ilar J. 60(1):17–23.
- Magaye R, Gu Y, Wang Y, Su H, Zhou Q, Mao G, Shi H, Yue X, Zou B, Xu J, et al. 2016. In vitro and in vivo evaluation of the toxicities induced by metallic nickel nano and fine particles. J Mol Histol. 47(3):273–286.
- Magaye R, Zhao J. 2012. Recent progress in studies of metallic nickel and nickel-based nanoparticles' genotoxicity and carcinogenicity. Environ Toxicol Pharmacol. 34(3):644–650.
- Magaye R, Zhao J, Bowman L, Ding M. 2012. Genotoxicity and carcinogenicity of cobalt-, nickel- and copper-based nanoparticles. Exp Ther Med. 4(4):551–561.
- Magaye R, Zhou Q, Bowman L, Zou B, Mao G, Xu J, Castranova V, Zhao J, Ding M. 2014. Metallic nickel nanoparticles may exhibit higher carcinogenic potential than fine particles in JB6 cells. PLoS One. 9(4):e92418.
- Malhotra J, Malvezzi M, Negri E, La Vecchia C, Boffetta P. 2016. Risk factors for lung cancer worldwide. Eur Respir J. 48(3):889–902.
- Marsh GM, Buchanich JM, Zimmerman S, Liu Y, Balmert LC, Esmen NA, Kennedy KJ. 2017a. Mortality among hardmetal production workers: US cohort and nested case–control studies. J Occup Environ Med. 59(12):e306–e326.
- Marsh GM, Buchanich JM, Zimmerman S, Liu Y, Balmert LC, Graves J, Kennedy KJ, Esmen NA, Moshammer H, Morfeld P, et al. 2017b. Mortality among hardmetal production workers: pooled analysis of cohort data from an international investigation. J Occup Environ Med. 59(12):e342–e364.
- McElvenny DM, MacCalman LA, Sleeuwenhoek A, Davis A, Miller BG, Alexander C, Cowie H, Cherrie JW, Kennedy KJ, Esmen NA, et al. 2017. Mortality among hardmetal production workers: UK cohort and nested case-control studies. J Occup Environ Med. 59(12):e275–e281.
- Meek ME, Palermo CM, Bachman AN, North CM, Lewis RJ. 2014. Mode of action human relevance (species concordance) framework: evolution of the Bradford Hill considerations and comparative analysis of weight of evidence. J Appl Toxicol. 34(6):595–606.
- Miura T, Patierno SR, Sakuramoto T, Landolph JR. 1989. Morphological and neoplastic transformation of C3H/10T1/2 Cl 8 mouse embryo cells by insoluble carcinogenic nickel compounds. Environ Mol Mutagen. 14(2):65–78.
- Moore MM, Pottenger LH, House-Knight T. 2019. Critical review of styrene genotoxicity focused on the mutagenicity/clastogenicity literature and using current organization of economic cooperation and development guidance. Environ Mol Mutagen. 60(7):624–663.
- Morfeld P, Groß JV, Erren TC, Noll B, Yong M, Kennedy KJ, Esmen NA, Zimmerman SD, Buchanich JM, Marsh GM. 2017. Mortality among hardmetal production workers: German historical cohort study. J Occup Environ Med. 59(12):e288–e296.
- Moulin JJ, Clavel T, Roy D, Dananche B, Marquis N, Fevotte J, Fontana JM. 2000. Risk of lung cancer in workers producing stainless steel and metallic alloys. Int Arch Occup Environ Health. 73(3):171–180.
- Muhle H, Bellman B, Takenaka S, Fuhst R, Mohr U, Pott F. 1992. Chronic effects of intratracheally instilled nickel-containing particles in hamsters. In: Nieboer E, Nriagu JO, editors. Nickel and human health: current perspectives. New York (NY): John Wiley and Sons, Inc.; p. 467–479.
- National Toxicology Program [NTP]. 1996a. NTP Technical Report on the Toxicology and Carcinogenesis Studies of Nickel Oxide (CAS No. 1313-99-1) in F344/N Rats and B6C3F1 Mice (Inhalation Studies). NTP TR 451. 381p.
- National Toxicology Program [NTP]. 1996b. NTP Technical Report on the Toxicology and Carcinogenesis Studies of Nickel Subsulfide (CAS No. 12035-72-2) in F344/N Rats and B6C3F1 Mice (Inhalation studies). NTP TR 453. 365p.
- National Toxicology Program [NTP]. 2002. Tenth report on carcinogens (Print copy and CD-ROM). Report to National Institute of Environmental Health Sciences (Research Triangle Park, NC). December.
- National Toxicology Program [NTP], Office of Health Assessment and Translation (OHAT). 2015. OHAT Risk of Bias Rating Tool for Human and Animal Studies. 37p. [accessed 2020 April 7]. https://ntp.niehs.nih.gov/ntp/ohat/pubs/riskofbiastool_508.pdf.
- National Toxicology Program [NTP]. 2016. Report on Carcinogens, Fourteenth Edition. Research Triangle Park, NC: U.S. Department of Health and Human Services, Public Health Service.
- National Toxicology Program [NTP], Office of Health Assessment and Translation (OHAT). 2019. Handbook for Conducting a Literature-Based Health Assessment Using OHAT Approach for Systematic Review and Evidence Integration. 101p. March 4 [accessed 2019 March 18]. https://ntp.niehs.nih.gov/ntp/ohat/pubs/handbookmarch2019_508.pdf.
- Oberdorster G. 2001. Pulmonary effects of inhaled ultrafine particles. Int Arch Occup Environ Health. 74(1):1–8.
- Oberdorster G, Ferin J, Lehnert BE. 1994. Correlation between particle size, in vivo particle persistence, and lung injury. Environ Health Perspect. 102(Suppl. 5):173–179.
- Organisation for Economic Co-operation and Development [OECD]. 2014. OECD Guideline for Testing of Chemicals: In Vivo Mammalian Alkaline Comet Assay. TG-489. 25p.
- Organisation for Economic Co-operation and Development [OECD]. 2016a. OECD Guideline for the Testing of Chemicals: In Vitro Mammalian Cell Gene Mutation Tests using the Hprt and xprt genes. OECD/OCDE 476. 18p.
- Organisation for Economic Co-operation and Development [OECD]. 2016b. OECD Guideline for the Testing of Chemicals: In Vitro Mammalian Cell Micronucleus Test. OECD/OCDE 487. 29p.
- Organisation for Economic Co-operation and Development [OECD]. 2017. Overview of the set of OECD Genetic Toxicology Test Guidelines and updates performed in 2014–2015. ENV/JM/MONO(2016)33/REV1. 70p.
- Organisation for Economic Co-operation and Development [OECD]. 2018. OECD Guideline for the Testing of Chemicals: Carcinogenicity Studies. Test No. 451. 15p.
- Oller AR, Cappellini D, Henderson R, Bates HK. 2009. Comparison of nickel release in solutions used for the identification of water-soluble nickel exposures and in synthetic lung fluids. J Environ Monit. 11(4):823–829.
- Oller AR, Costa M, Oberdorster G. 1997. Carcinogenicity assessment of selected nickel compounds. Toxicol Appl Pharmacol. 143(1):152–166.
- Oller AR, Erexson G. 2007. Lack of micronuclei formation in bone marrow of rats after repeated oral exposure to nickel sulfate hexahydrate. Mutat Res. 626(1–2):102–110.
- Oller AR, Kirkpatrick DT, Radovsky A, Bates HK. 2008. Inhalation carcinogenicity study with nickel metal powder in Wistar rats. Toxicol Appl Pharmacol. 233(2):262–275.
- Oller AR, Oberdorster G. 2010. Incorporation of particle size differences between animal studies and human workplace aerosols for deriving exposure limit values. Regul Toxicol Pharmacol. 57(2–3):181–194.
- Ozaki K, Haseman JK, Hailey JR, Maronpot RR, Nyska A. 2002. Association of adrenal pheochromocytoma and lung pathology in inhalation studies with particulate compounds in the male F344 rat – The National Toxicology Program experience. Toxicol Pathol. 30(2):263–270.
- Pandiri A. 2015. Comparative pathobiology of environmentally induced lung cancers in humans and rodents. Toxicol Pathol. 43(1):107–114.
- Paton GR, Allison AC. 1972. Chromosome damage in human cell cultures induced by metal salts. Mutat Res. 16(3):332–336.
- Pavela M, Uitti J, Pukkala E. 2017. Cancer incidence among copper smelting and nickel refining workers in Finland. Am J Ind Med. 60(1):87–95.
- Pott F, Ziem U, Reiffer FJ, Huth F, Ernst H, Mohr U. 1987. Carcinogenicity studies on fibres, metal compounds, and some other dusts in rats. Exp Pathol. 32(3):129–152.
- Revie RW, Uhlig HH. 2008. Corrosion and corrosion control. 4th ed. Hoboken (NJ): Wiley-Interscience.
- Rhomberg LR, Goodman JE, Bailey LA, Prueitt RL, Beck NB, Bevan C, Honeycutt M, Kaminski NE, Paoli G, Pottenger LH, et al. 2013. A survey of frameworks for best practices in weight-of-evidence analyses. Crit Rev Toxicol. 43(9):753–784.
- Seilkop SK, Lightfoot NE, Berriault CJ, Conard BR. 2017. Respiratory cancer mortality and incidence in an updated cohort of Canadian nickel production workers. Arch Environ Occup Health. 72(4):204–219.
- Serita F, Kyono H, Seki Y. 1999. Pulmonary clearance and lesions in rats after a single inhalation of ultrafine metallic nickel at dose levels comparable to the threshold limit value. Ind Health. 37(4):353–363.
- Sivulka DJ. 2005. Assessment of respiratory carcinogenicity associated with exposure to metallic nickel: a review. Regul Toxicol Pharmacol. 43(2):117–133.
- Sivulka DJ, Seilkop SK. 2009. Reconstruction of historical exposures in the U.S. nickel alloy industry and the implications for carcinogenic hazard and risk assessments. Regul Toxicol Pharmacol. 53(3):174–185.
- Smith MT, Guyton KZ, Gibbons CF, Fritz JM, Portier CJ, Rusyn I, DeMarini DM, Caldwell JC, Kavlock RJ, Lambert P, et al. 2016. Key characteristics of carcinogens as a basis for organizing data on mechanisms of carcinogenesis. Environ Health Perspect. 124(6):713–721.
- Sorahan T. 2004. Mortality of workers at a plant manufacturing nickel alloys, 1958–2000. Occup Med (Lond). 54(1):28–34.
- Sorahan T, Williams SP. 2005. Mortality of workers at a nickel carbonyl refinery, 1958–2000. Occup Environ Med. 62(2):80–85.
- Stockholm University, Karolinska Institutet. 2019. Science in Risk Assessment and Policy (SciRAP). [accessed 2019 March 15] http://www.scirap.org/.
- Svartengren M, Bryngelsson IL, Marsh G, Buchanich J, Zimmerman S, Kennedy K, Esmen N, Westberg H. 2017. Cancer incidence among hardmetal production workers: the Swedish cohort. J Occup Environ Med. 59(12):e365–e373.
- Tice RR, Agurell E, Anderson D, Burlinson B, Hartmann A, Kobayashi H, Miyamae Y, Rojas E, Ryu JC, Sasaki YF. 2000. Single cell gel/comet assay: guidelines for in vitro and in vivo genetic toxicology testing. Environ Mol Mutagen. 35(3):206–221.
- United States Environmental Protection Agency [US EPA]. 2002. IRIS Chemical Assessment Summary for Nickel Subsulfide (CAS No. 12035-72-2). [accessed 2017 September 13]. https://www.epa.gov/iris.
- United States Environmental Protection Agency [US EPA]. 2006. IRIS Chemical Assessment Summary for Nickel Refinery Dust (No CAS No.). [accessed 2017 September 13]. https://www.epa.gov/iris.
- United States Environmental Protection Agency [US EPA]. 2015. Preamble to the Integrated Science Assessments. EPA/600/R-15/067. [accessed 2015 December 1]. http://www.epa.gov/isa.
- United States Environmental Protection Agency [US EPA]. 2018. Office of Chemical Safety and Pollution Prevention; US EPA, Office of Pollution Prevention and Toxics. Application of Systematic Review in TSCA Risk Evaluations (Final). EPA Document # 740-P1-8001. 248p. [accessed 2018 June 15]. https://www.epa.gov/sites/production/files/2018-06/documents/final_application_of_sr_in_tsca_05-31-18.pdf.
- United States Environmental Protection Agency [US EPA]. 2019. Handbook for Developing IRIS Assessments (Version 1.0) (Draft). 271p. [accessed 2019 September 20]. https://insideepa.com/sites/insideepa.com/files/documents/2019/sep/epa2019_1559.pdf.
- Westberg H, Bryngelsson IL, Marsh G, Buchanich J, Zimmerman S, Kennedy K, Esmen N, Svartengren M. 2017. Mortality among hardmetal production workers: the Swedish cohort. J Occup Environ Med. 59(12):e263–e274.
- WIL Research Laboratories, Inc. 2004. A 13-Week Inhalation Toxicity Study (With Recovery) of Nickel Metal in Albino Rats (Final). Report to NiPERA (Durham, NC); 1779p.
- Zhang Q, Kusaka Y, Zhu X, Sato K, Mo Y, Kluz T, Donaldson K. 2003. Comparative toxicity of standard nickel and ultrafine nickel in lung after intratracheal instillation. J Occup Health. 45(1):23–30.
- Zhao J, Bowman L, Zhang X, Shi X, Jiang B, Castranova V, Ding M. 2009. Metallic nickel nano- and fine particles induce JB6 cell apoptosis through a caspase-8/AIF mediated cytochrome c-independent pathway. J Nanobiotechnol. 7:2.
- Zhong BZ, Li ZQ, Ma GY, Wang BS. 1990. Study on mutagenesis and carcinogenesis of productive nickel dust. In: Mendelsohn MJ, Albertini RJ, editors. Mutation and the environment, part E: environmental genotoxicity, risk, and modulation. New York (NY): Wiley-Liss, Inc.; p. 41–46.