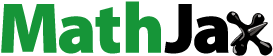
Abstract
Matrix high-throughput screening (HTS) methods are increasingly employed to rapidly define potential therapeutic drug combinations. We used combination HTS to identify compounds showing synergistic anti-proliferative activity with ibrutinib, an irreversible, small-molecule inhibitor of Bruton’s tyrosine kinase. The goal was to identify ibrutinib combinations with maximum synergistic effects in heme malignancy lines, particularly in non-Hodgkin lymphoma including diffuse large B-cell lymphoma (DLBCL). Growth inhibition (GI) was used to measure cell viability; synergy scores characterized strength of synergistic interaction. Single-agent ibrutinib demonstrated varying degrees of activity across 30 cell lines evaluated. In DLBCL lines, TMD8 was the most sensitive to ibrutinib (GI50 = 0.001); combinations with BCL-2 inhibitor ABT-199, and PI3K inhibitors IPI-145 and GDC-0941 showed the strongest synergistic activity. Anti-proliferative synergies were also observed with BET bromodomain inhibitor (+)-JQ1, XPO1 inhibitor selinexor, and IRAK4 inhibitor, and confirmed using apoptosis assay. These findings are intended to inform and advance treatment of B-cell malignancies.
Introduction
Multi-targeted drug combinations are evaluated in vitro and pre-clinically in order to establish anti-cancer efficacy and potential standard of care for different types of malignancy. These regimens can comprise various combinations of standard of care with a novel agent for a particular indication. In B-cell malignancies, Bruton’s tyrosine kinase (BTK) has emerged as one of the central targets for therapy [Citation1]. BTK is required for B-cell receptor (BCR) signaling, plays a key role in B-cell maturation, and is overexpressed in a number of B-cell malignancies [Citation2]. Specifically, constitutive B-cell activation via the BTK-BCR, resulting in a cascade of effects impacting the nuclear factor NF-κB pathway, has been implicated in various hematological malignancies such as chronic lymphocytic leukemia (CLL), mantle cell lymphoma (MCL), and the activated B-cell (ABC)-like subtype of diffuse large B-cell lymphoma (DLBCL) [Citation3].
DLBCL is a highly heterogeneous disease, with ABC and germinal center B-cell (GCB) subtypes representing molecularly distinct classes, and is defined by somatic mutations, translocations, and genomic copy number changes [Citation4–6]. Constitutive activation of the NF-κB pathway emanating from either the BCR pathway or the signaling adapter MyD88 appears to be a universal feature of the ABC subtype of DLBCL [Citation7]. Accounting for 30–35% of non-Hodgkin lymphoma (NHL) [Citation8], DLBCL is a current focus of research into optimal ibrutinib combinations.
Ibrutinib is a first-in-class, once-daily, oral, covalent inhibitor of BTK [Citation2,Citation9]. In vitro, ibrutinib inhibited the growth of DLBCL cell lines that overexpress BTK [Citation7], and it has also shown activity in animal models of B-cell malignancies [Citation2]. A phase 1 study that established the dose, safety profile, pharmacokinetics, pharmacodynamics, and tumor response in patients with relapsed/refractory NHL and CLL demonstrated complete or near-complete BTK occupancy with a clinically active dose of ibrutinib [Citation9]. Subsequent phase 2 and 3 single-agent and combination studies have shown the benefits of ibrutinib across multiple B-cell malignancies [Citation10–16]. Ibrutinib has been approved in many regions for indications covering the treatment of patients with MCL who have received at least one prior therapy, patients with CLL or small lymphocytic lymphoma (SLL), CLL or SLL patients with the presence of deletion in the short arm of chromosome 17p13.1 (del17p) or TP53 mutation, and patients with Waldenstrom’s macroglobulinemia (WM).
The further clinical development of ibrutinib in B-cell malignancies will be aided by establishing ibrutinib combination treatments with maximum synergistic effects. Combination high-throughput screening (cHTS) platforms enable numerous drug–drug pairs to be explored rapidly to identify actionable drug combinations. We report on the use of one such platform which uses conditioned media from a human bone marrow stromal cell line in order to better mimic the type(s) of growth stimuli that would typically be present in the tumor microenvironment [Citation17]. This platform is used to systematically screen for combinations of ibrutinib with approved therapeutics, emerging drugs, and well-characterized molecular probes targeting different aspects of the BCR-signaling pathway known to be relevant in the pathogenesis of cancer. The goal of this project was to identify ibrutinib combinations, their dose, and a sequence of administration that had maximum synergistic effects in cell lines from different B-cell malignancies, with a focus on DLBCL.
Materials and methods
Cell lines
A total of 30 cell lines were examined from a range of B-cell malignancies (acute myeloid leukemia [AML], acute B-lymphoblastic leukemia [B-ALL], Burkitt’s lymphoma [BL], follicular lymphoma [FL], MCL, multiple myeloma [MM], and DLBCL). The DLBCL cell lines examined were HBL-1, TMD8, OCI-Ly3, OCI-Ly10, and SU-DHL-6-epst. Of the five DLBCL cell lines used, all were ABC-DLBCL except for SU-DHL-6-epst, which was GCB-DLBCL. All cell lines were provided by the Horizon Discovery Group (formerly Zalicus) (Cambridge, UK). HBL-1, OCI-Ly3, and OCI-Ly10 cell lines were cultured in Iscove Modified Dulbecco Medium (IMDM), with 10% fetal bovine serum (FBS; HBL-1), 20% FBS (OCI-Ly3), or 20% human serum (OCI-Ly10). TMD8 cell lines were grown in minimal essential medium (MEM) with 10% FBS, and SU-DHL-6-epst cell lines were cultured in Roswell Park Memorial Institute (RPMI) medium with 10% FBS. Details of the culture conditions for all cell lines included in the screen are shown in Supplemental Table 1.
Cell lines were screened in the presence of conditioned media from a human bone marrow stromal cell line in order to encourage the type(s) of growth stimuli that would typically be present in the tumor microenvironment. Bone marrow stromal cells were provided by Asterand Bioscience (Royston, UK).
Compounds and antibodies
Media were supplemented with goat anti-human IgG (catalog # 2040-01, 10 μg/mL final in assay) and goat anti-human IgM (catalog # 2020-01, 10 μg/mL final in assay), which are antibodies that bind to the BCR. Both antibodies were provided by Southern Biotech (Birmingham, AL).
Ibrutinib was combined with inhibitors of the BCR pathway and regulators of apoptosis. Targets (agents) included BCL-2 (ABT-199; venetoclax), isoforms of PI3K (GDC-0941, IPI-145; duvelisib), XPO1 (selinexor), BET bromodomain (JQ1), IRAK4 (aminopyrimidine-1) [Citation18], and MCL-1 (A-1210477). All compounds except for JQ1 were sourced by the Horizon Discovery Group. JQ1 was obtained from Selleck Chemicals (Houston, TX).
To identify anti-proliferative combination effects at on-target concentrations, the single-agent dose axis for ibrutinib was limited to a concentration of 200 nM.
Anti-proliferation assay
Cells were thawed from a liquid nitrogen preserved state. Screening began once the cells had been expanded and were dividing at their expected doubling times. They were seeded in growth media in black 384-well tissue culture-treated plates at 1500 cells per well. Cells were equilibrated in assay plates via centrifugation and placed in incubators attached to the dosing modules at 37 °C for 24 hours before treatment.
The endpoint readout of the anti-proliferation assay was based on quantitation of adenosine triphosphate (ATP) as an indicator of viable cells. At the time of treatment, a set of assay plates (which did not receive treatment, referred to as T-zero [T0]), were collected and ATP levels were measured by adding ATPlite (PerkinElmer Inc., Boston, MA). The T0 plates were read using ultra-sensitive luminescence on Envision Plate Readers (PerkinElmer, Boston, MA) and served as untreated controls. Treated assay plates were incubated with compound for 72 hours. After 72 hours, plates were developed for endpoint analysis using ATPlite. All data points were collected via automated processes, quality controlled, and analyzed using Horizon Discovery proprietary software (Cambridge, UK). Assay plates were accepted if they passed the following quality control standards: consistent relative luciferase values throughout the entire experiment, Z-factor scores >0.6, and consistent behavior of untreated/vehicle controls on the plate.
Growth inhibition
Horizon Discovery utilized growth inhibition (GI) as a measure of cell viability. The cell viability of vehicle was measured at the time of dosing (T0) and after 72 hours (T72). A GI reading of 0% represented no GI (i.e. cells treated with compound and T72 vehicle signals were matched). A GI of 100% represented complete GI (cell numbers do not increase during the treatment period), potentially suggesting a cytostatic effect for compounds reaching a plateau at this effect level. A GI of 200% represented complete death of all cells in the culture well. Compounds reaching an activity plateau of 200% GI are considered cytotoxic. Horizon Discovery calculated GI by applying the following test and equation:
If
If
where T is the signal measure for a test article, V is the vehicle-treated control measure, and V0 is the vehicle control measure at time zero. This formula was derived from the GI calculation used in the National Cancer Institute’s NCI-60 HTS [Citation19].
Analysis of combination activity
Dose–response matrix screening (9 × 9 optimized matrix, four replicates) was used to measure combination effects, which manifest as potency shifts or efficacy boosts (). Combination activity was then characterized by comparing each data point with a combination reference model derived from single-agent curves using the Loewe additivity model [Citation20], where synergy was determined relative to Loewe additivity. Matrices with moderate-to-high scores were reviewed to select a cutoff using the histogram analysis feature of the Chalice Analyzer [Citation17], enabling potentially valid moderate synergies to be identified.
Figure 1. Schematic illustrating potency shifts (light gray line) and efficacy boosts (dark gray line) used in the identification of combination activity in dose–response matrix screening.

A scalar measure known as the synergy score was devised by Horizon Discovery to characterize the strength of the synergistic interaction, and is calculated as follows:
The fractional inhibition for each component agent and combination point in the matrix was calculated relative to the median of all vehicle-treated control wells.
Potency shifting was evaluated using an isobologram, which demonstrated how much less drug was required in combination to achieve a desired effect level, compared with the single-agent doses needed to reach that same effect.
Apoptosis assay
Apoptosis was evaluated by DNA-flow cytometry and analyzed by Annexin-V kit (# 556547, Becton Dickinson, Waltham, MA). During the early stages of apoptosis, phosphatidylserine residues translocated from the inner to the outer leaflet of the plasma membrane to bind Annexin-V-FITC. For detection of apoptosis, cells were harvested during the logarithmic growth period and counted with a cell counter. Cell concentrations were adjusted; 200 μL cell suspensions were added to 96-well round bottom plates and gently mixed for even cell distribution. Plates were incubated at 37 °C in 5% CO2 overnight. Cell lines were then treated with appropriate compounds and incubated for 72 hours. After treatment, cells were collected and incubated with Annexin-V-FITC in a binding buffer (provided by the manufacturer) for 30 min at 4 °C. Analysis of apoptotic cells was performed by flow cytometry (FACS Aria C6, Becton Dickinson, Franklin Lakes, NJ) using Annexin V and PI double staining. Experiments were conducted in triplicate and mean standard deviations were calculated (Supplemental Table 3).
Results
Single-agent dose–response analysis
Single-agent ibrutinib demonstrated varying activity and potency across the 30 hematological malignancy cell lines evaluated within the combination screen. No single-agent activity was observed in MM. Single-agent activity was shown in 4/5 MCL, 3/4 FL, 3/5 AML, 2/2 BL, 1/4 B-ALL, and 2/5 DLBCL cell lines (). The median GI50 across the entire cell line panel was 0.59 μM; two DLBCL, one FL, two MCL, one B-ALL, and two AML lines had GI50 values that were below this level. Fifteen cell lines did not achieve a GI50 level with single-agent ibrutinib and these data are not shown in this figure. Among the DLBCL cell lines screened, TMD8 was the most sensitive to ibrutinib, with a GI50 value in the picomolar range (, Supplemental Table 2), and the two DLBCL cell lines with single-agent activity (TMD8 and HBL-1) were both ABC subtype. The MCL cell line REC-1 also showed a GI50 value in the picomolar range.
Figure 2. Ibrutinib single-agent dose–response analysis showing GI50 values by type of hematological malignancy (15 cell lines did not achieve a GI50 level with single-agent ibrutinib; data are not shown). Ibrutinib GI50 data were extracted from the combination screen and values derived from a fitted curve. GI: growth inhibition; AML: acute myeloid leukemia; B-ALL: acute B-lymphoblastic leukemia; BL: Burkitt’s lymphoma; DLBCL: diffuse large B-cell lymphoma; FL: follicular lymphoma; MCL: mantle cell lymphoma.

Combination activity based on synergy score analysis
Global analysis
Cell line-specific synergistic activity was demonstrated when ibrutinib was combined with compounds from several molecular classes targeting the BCR pathway, at clinically achievable concentrations. summarizes synergy scores with all ibrutinib combinations tested across the DLBCL, FL, MCL, AML, and BL cell lines included in the cHTS. Ibrutinib combinations with BCL-2 and PI3K inhibitors were the most active in the DLBCL cell lines and in one FL cell line (DOHH-2). Ibrutinib combinations with BCL-2, along with the BET bromodomain XPO1, and IRAK were identified as the most active in one MCL cell line (REC-1). The highest activity, with a synergy score of 63.9, was seen in the DLBCL cell line TMD8 with the PI3K inhibitor IPI-145. Synergy score values for AML, ALL, BL, and MM cell lines were generally below the experimentally determined threshold value (9.59) for synergistic activity, with a few exceptions of moderate synergy such as with ABT-199 in AML.
Table 1. Synergy scores with ibrutinib drug combinations, showing breadth of activity across the DLBCL, FL, MCL, AML, and BL cell lines tested in the HTS. Synergy score values for AML are included to show some near-threshold synergy scores.
BCL-2 inhibitors
Combination activity was observed with ibrutinib and the BCL-2 inhibitor ABT-199 below clinically achievable concentrations (∼3–4 μM) [Citation21] in select DLBCL cell lines ( and ). Strong synergy scores of 40.0 and 21.6 were calculated for the ibrutinib/ABT-199 combination in DLBCL cell lines HBL-1 and TMD8, respectively. This combination was also synergistic in several FL and MCL lines as well, and interestingly, exhibited moderate synergy (score >9.59 but <19.2) in three AML lines.
Figure 3. Synergy scores for DLBCL cell lines by ibrutinib drug combination. Ibrutinib concentration gating at 200 nM. Scores in the range 9.59–19.2 indicate moderate synergy, and scores >19.2 indicate strong synergy.

Figure 4. Dose matrices (9 × 9) for the ibrutinib/BCL-2 inhibitor (ABT-199) combination in DLBCL cell lines HBL-1 and TMD8. The dose-matrix format allows identification of combination activity at multiple ratios across a wide range of concentrations of each compound. Each point from the observed combination data (left matrix) is compared with single-agent responses in the Loewe dose-additivity model to calculate data showing activity in excess of the dose-additivity model (right matrix). The blue line denotes drug levels below clinically achievable concentrations.

PI3K pathway inhibitors
Synergistic combination activity was also observed with ibrutinib and PI3K inhibitors (of varying isoform specificity) in select DLBCL cell lines at concentrations below levels that have been reported to be achievable in vivo (GDC-0941 [PI3Kα/δ] ∼ 0.3 μM; IPI-145 [PI3Kδ/γ] ∼ 2 μM) [Citation22,Citation23] ( and ). Ibrutinib combination with the PI3K inhibitors IPI-145 and GDC-0941 resulted in strong synergy scores of 63.9 and 23.9, respectively, for the DLBCL cell line TMD8.
Other molecule synergies
Strong synergistic anti-proliferative activity was also observed with ibrutinib in combination with the potent BET bromodomain inhibitor (+)-JQ1 and the XPO1 inhibitor selinexor (selective inhibitor of nuclear export) at concentrations below those known to be achievable in vivo in select DLBCL cell lines (JQ1 > 20 μM; selinexor∼650 nM) [Citation24,Citation25], and with the IRAK4 inhibitor at ≤1 μM (0.042 μM in TMD8 cell line) (). There was no observed antagonism with any of the ibrutinib combinations screened.
Apoptosis assay
In order to verify if proliferation results could be extended to a different assay, apoptosis induced by ibrutinib and its combinations was tested in TMD8, DOHH2, OCI-LY3, and HBL-1 cell lines. The results of the apoptosis assay paralleled the results demonstrating the synergistic effect of ibrutinib combinations on GI. In the TMD-8, HBL-1, and DOHH2 cell lines, which are sensitive to ibrutinib alone as well as to ABT-199 alone, there was an increase in apoptotic cells when treated with the combination (; ; Supplemental Figure 2). The combination of ibrutinib and IPI145 showed synergy in TMD-8 and DOHH2 lines. Similar results were also seen with other synergistic combinations, such as with IRAK4 and PI3K inhibitors. However, this increased apoptosis was not seen in the ibrutinib-resistant OCI-LY3 cell line, or in a GCB-DLBCL line SU-DHL2 (Supplemental Figure 2).
Discussion
We report experimental and technical details of a cHTS in matrix form that enabled in vitro investigation of multiple ibrutinib combinations in DLBCL and other B-cell malignancies. Compelling ibrutinib synergies have been identified that are likely to have future therapeutic utility; ibrutinib shows cell line-specific combination activity with several mechanistically distinct classes of compounds that target the BCR signaling pathway at clinically achievable concentrations, providing good rationale for future clinical testing. In specific DLBCL cell lines (TMD8 and HBL-1), maximum activity appeared to be achieved when ibrutinib was combined with inhibitors of BCL-2 and PI3K. Strong synergy was also observed with inhibitors of XPO1, bromodomain, and IRAK4 in DLBCL. These ibrutinib combinations showed further synergistic anti-proliferative activity in MCL and/or FL cell lines.
The synergistic activity observed with ibrutinib and the BCL-2 inhibitor ABT-199 in this study supports previous findings. Favorable ibrutinib synergy with the BCL-2 inhibitor ABT-737 was observed in a different combination screen, and the two compounds appeared to show combination activity in a number of the same cell lines, including DLBCL lines TMD8, HBL-1, and SU-DHL-6-epst (data not shown). Synergies between ibrutinib and both ABT-199 and ABT-737 have also been reported in MCL and CLL cells [Citation26,Citation27]. In all MCL cell lines tested and in primary cells from recurrent MCL patients, strong synergistic effects on proliferation inhibition and apoptosis were demonstrated with an ibrutinib/ABT-199 combination [Citation27]. Further data from this study provide insights into the molecular mechanisms driving the interaction in MCL cells, including the down-regulation of BCL2 family proteins (BCL-2, BCL2L1, and MCL1), which regulate apoptosis, are often overexpressed in lymphoma, and have been implicated in the development of resistance to many treatments. In the MCL cell line CCMCL1, ibrutinib alone or in combination with ABT-199 down-regulated MCL1, leading to induction of apoptosis [Citation28]. Alone, each agent had relatively little effect on BCL-2 or BCL2L1, but in combination, the agents down-regulated both proteins. While it is known that ibrutinib and ABT-199 act on different targets, rather than simply producing additive effects when combined, each compound appeared to enhance the activity of the other on its known targets. Similar findings were presented from ex vivo and in vitro studies in CLL with ABT-199 and ABT-737 [26]. In addition, while lymphocytosed CLL cells from patients receiving ibrutinib exhibited some degree of resistance to ibrutinib after ibrutinib therapy, the cells appeared similarly sensitive to ABT therapy pre- and post-ibrutinib [Citation26]. The moderate synergy of ibrutinib with ABT-199 seen in 3/5 AML lines in this study was notable in the light of minimal activity otherwise seen in this histology.
The synergistic anti-proliferative activity observed with ibrutinib and PI3K pathway inhibitors is also consistent with previous reports and with the current understanding of DLBCL pathogenesis. Normal BCR signaling also results in activation of PI3K in addition to BTK, and the PI3Kh pathway is thought to play a role in the pathogenesis of DLBCL, perhaps through regulation of NF-κB [Citation29]. Studies have reported favorable interactions with ibrutinib and PI3K inhibitors in DLBCL cell lines, including combinations with GDC-0941, IPI-145, and CAL-101 (idelalisib) [Citation30,Citation31]. A combination effect of ibrutinib/IPI-145 was observed in the DLBCL cell lines SU-DHL-4 and OCI-Ly8, supporting the mechanistic rationale for combining PI3K-δ,γ and BTK inhibition in this disease setting [Citation30]. Synergies with the PI3K-δ inhibitor CAL-101 were noted in another combination screen, and marked synergistic effects of CAL-101 and GDC-0941, among other PI3K pathway inhibitors, were previously reported with ibrutinib in an HTS of ABC DLBCL cell lines [Citation31].
Combinations of ibrutinib with specific inhibitors of either IRAK1/4 or IRAK4 have shown synergistic effects in WM and DLBCL [Citation32,Citation33], and these studies provide insights into the mechanisms underlying the synergistic activity observed with ibrutinib and the IRAK4 inhibitor in the cHTS. Synergistic killing of WM cells expressing the highly prevalent somatic mutation MYD88 L265P was demonstrated through the combined suppression of BTK and IRAK using ibrutinib and dual IRAK1/4 inhibitors. The combination resulted in increased inhibition of NF-κB signaling and enhanced WM cell killing [Citation33]. Strong synergy between ibrutinib and the IRAK4 inhibitors ND-2158 and ND-2110 has also been shown in mechanistic studies of multiple ABC DLBCL cell lines [Citation32], providing support for further clinical development of IRAK4 inhibitors with simultaneous BTK inhibition in ABC DLBCL. The apoptosis assay confirmed our findings from the ATPlite combination assay demonstrating that ibrutinib combination with ABT-199 and IRAK4 inhibitors shows a synergistic increase in cell death in DLBCL cell lines. In this context, it is interesting to note that other compounds active in DLBCL may also synergize with ibrutinib through their effects on the BCR pathway, including lenalidomide, JAK inhibitors and SYK inhibitors [Citation34–36]; we also observed increased apoptosis in the combinations of these with ibrutinib (e.g. JAK1/2 inhibitor ruxolitinib, ).
While not explored in the current cHTS, there are data to indicate that ibrutinib also combines favorably with commonly used chemotherapeutic agents, including doxorubicin, gemcitabine, and docetaxel [Citation31]. It is thought that inhibition of NF-κB by ibrutinib in ABC DLBCL leads to increased apoptosis of cancer cells following treatment with DNA-damaging chemotherapeutic agents, providing a mechanistic rationale for combining ibrutinib with the current standard of care for DLBCL, R-CHOP (rituximab, cyclophosphamide, doxorubicin, vincristine, prednisone), as well as other chemotherapeutic agents. In a small phase 1 clinical trial, the addition of ibrutinib to R-CHOP chemotherapy for NHL resulted in an objective response rate of 100% [Citation16], and a randomized, double-blind, placebo-controlled phase 3 study is currently underway to compare R-CHOP plus placebo with R-CHOP plus ibrutinib in patients with newly diagnosed non-GCB subtype DLBCL (ClinicalTrials.gov identifier: NCT01855750). A phase 1 study combining ibrutinib with R-ICE (rituximab, ifosfamide, carboplatin, and etoposide) in patients with relapsed or primary refractory DLBCL is also ongoing (ClinicalTrials.gov identifier: NCT02219737). In addition, a phase 1b study of ibrutinib in combination with lenalidomide and dose-adjusted EPOCH-R (cyclophosphamide, doxorubicin, vincristine, etoposide, prednisone with rituximab) demonstrated that this combination showed acceptable safety and tolerability and promising anti-tumor activity in patients with relapsed/refractory DLBCL [Citation37].
Following the recent approval of ibrutinib for the treatment of MCL, CLL, and WM, there has been an increasing focus on the drug’s potential activity in other B-cell malignancies such as DLBCL, both as a single agent and in combination with other drugs or standard of care agents. In order to reduce the potential development of resistance and produce augmented and durable responses, it is likely that optimal treatment with ibrutinib will be achieved through combination with other agents, including small molecules with complementary mechanisms of action, and current standard of care chemotherapeutic agents. Progress on this front needs to be paralleled with in vitro testing to rapidly generate sufficient data with favorable combinations to inform further clinical investigation. In this report, we present experimental and technical details of a cHTS in matrix form that was successfully utilized to identify synergistic ibrutinib combinations with BCL-2 and PI3K inhibitors in DLBCL. HTS platforms such as that described will be instrumental for future clinical drug development, and can be further enhanced by examining additional chemical agents that affect the same targets and pathways, with longer assay times (since some activity may not be readily apparent at 72 hours), and expanded concentration sampling. Drugs may be applied using pulse dosing to limit exposure and reproduce pharmacokinetic properties, and drug sequencing explored to better understand its impact on combination activity. Colony-forming cell assays with higher resolution will enable the magnitude of the combination effect to be more accurately evaluated. Together with in vivo data to confirm synergistic activity identified by the screen, this information will help us to accelerate the discovery and clinical development of novel drug combinations and, in doing so, provide more effective treatment for patients.
Potential conflict of interest
Disclosure forms provided by the authors are available with the full text of this article online at https://doi.org/10.1080/10428194.2017.1349899
ICMJE Forms for Disclosure of Potential Conflicts of Interest
Download Zip (12.3 MB)Supplemental Material
Download MS Word (677.8 KB)Additional information
Funding
References
- Young RM, Staudt LM. Targeting pathological B cell receptor signalling in lymphoid malignancies. Nat Rev Drug Discov. 2013;12:229–243.
- Honigberg LA, Smith AM, Sirisawad M, et al. The Bruton tyrosine kinase inhibitor PCI-32765 blocks B-cell activation and is efficacious in models of autoimmune disease and B-cell malignancy. Proc Natl Acad Sci USA. 2010;107:13075–13080.
- Novero A, Ravella PM, Chen Y, et al. Ibrutinib for B cell malignancies. Exp Hematol Oncol. 2014;3:4.
- Alizadeh AA, Eisen MB, Davis RE, et al. Distinct types of diffuse large B-cell lymphoma identified by gene expression profiling. Nature. 2000;403:503–511.
- Lenz G, Wright GW, Emre NC, et al. Molecular subtypes of diffuse large B-cell lymphoma arise by distinct genetic pathways. Proc Natl Acad Sci USA. 2008;105:13520–13525.
- Wright G, Tan B, Rosenwald A, et al. A gene expression-based method to diagnose clinically distinct subgroups of diffuse large B cell lymphoma. Proc Natl Acad Sci USA. 2003;100:9991–9996.
- Davis RE, Brown KD, Siebenlist U, et al. Constitutive nuclear factor KB activity is required for survival of activated B cell-like diffuse large B cell lymphoma cells. J Exp Med. 2001;194:1861–1874.
- Siegel R, Naishadham D, Jemal A. Cancer statistics, 2013. CA Cancer J Clin. 2013;63:11–30.
- Advani RH, Buggy JJ, Sharman JP, et al. Bruton tyrosine kinase inhibitor ibrutinib (PCI-32765) has significant activity in patients with relapsed/refractory B-cell malignancies. J Clin Oncol. 2013;31:88–94.
- Byrd JC, Furman RR, Coutre SE, et al. Targeting BTK with ibrutinib in relapsed chronic lymphocytic leukemia. N Engl J Med. 2013;369:32–42.
- Byrd JC, Brown JR, O’Brien S, et al. Ibrutinib versus ofatumumab in previously treated chronic lymphoid leukemia. N Engl J Med. 2014;371:213–223.
- Chanan-Khan A, Cramer P, Demirkan F, et al. Ibrutinib combined with bendamustine and rituximab compared with placebo, bendamustine, and rituximab for previously treated chronic lymphocytic leukaemia or small lymphocytic lymphoma (HELIOS): a randomised, double-blind, phase 3 study. Lancet Oncol. 2016;17:200–211.
- Dreyling M, Jurczak W, Jerkeman M, et al. Ibrutinib versus temsirolimus in patients with relapsed or refractory mantle-cell lymphoma: an international, randomised, open-label, phase 3 study. Lancet. 2016;387:770–778.
- Wang ML, Rule S, Martin P, et al. Targeting BTK with ibrutinib in relapsed or refractory mantle-cell lymphoma. N Engl J Med. 2013;369:507–516.
- Wilson WH, Young RM, Schmitz R, et al. Targeting B cell receptor signaling with ibrutinib in diffuse large B cell lymphoma. Nat Med. 2015;21:922–926.
- Younes A, Thieblemont C, Morschhauser F, et al. Combination of ibrutinib with rituximab, cyclophosphamide, doxorubicin, vincristine, and prednisone (R-CHOP) for treatment-naive patients with CD20-positive B-cell non-Hodgkin lymphoma: a non-randomised, phase 1b study. Lancet Oncol. 2014;15:1019–1026.
- Horizon Discovery Web site [Internet]. Horizon discovery. Horizon Chalice® Bioinformatics Software. Available from: http://chalice.horizondiscovery.com/chalice-portal/documentation/analyzer/home.jsp
- Seganish WM, McElroy WT, Herr RJ, et al. Initial optimization and series evolution of diaminopyrimidine inhibitors of interleukin-1 receptor associated kinase 4. Bioorg Med Chem Lett. 2015;25:3203–3207.
- Shoemaker RH. The NCI60 human tumour cell line anticancer drug screen. Nat Rev Cancer. 2006;6:813–823.
- Chou TC, Talalay P. Generalized equations for the analysis of inhibitions of Michaelis–Menten and higher-order kinetic systems with two or more mutually exclusive and nonexclusive inhibitors. Eur J Biochem. 1981;115:207–216.
- Pan R, Hogdal LJ, Benito JM, et al. Selective BCL-2 inhibition by ABT-199 causes on-target cell death in acute myeloid leukemia. Cancer Discov. 2014;4:362–375.
- Dong S, Guinn D, Dubovsky JA, et al. IPI-145 antagonizes intrinsic and extrinsic survival signals in chronic lymphocytic leukemia cells. Blood. 2014;124:3583–3586.
- Salphati L, Wong H, Belvin M, et al. Pharmacokinetic–pharmacodynamic modeling of tumor growth inhibition and biomarker modulation by the novel phosphatidylinositol 3-kinase inhibitor GDC-0941. Drug Metab Dispos. 2010;38:1436–1442.
- Chen CI, Gutierrez M, de Nully Brown P, et al. Antitumor activity of selinexor (kpt-330), a first-in-class oral selective inhibitor of nuclear export (SINE) XPO1/CRM1 antagonist in patients (pts) with relapsed/refractory multiple myeloma (MM) or Waldenstrom’s macroglobulinemia (WM). Paper presented at: 55th Annual Meeting of the American Society of Hematology; 2013 December 7–10; New Orleans, LA. Abstract 1942.
- Matzuk MM, McKeown MR, Filippakopoulos P, et al. Small-molecule inhibition of BRDT for male contraception. Cell. 2012;150:673–684.
- Cervantes-Gomez F, Balakrishnan K, Wierda WG, et al. Ex-vivo and in-vitro combination strategies with ibrutinib in chronic lymphocytic leukemia. Cancer Res. 2014;74(19 suppl):Abstract 4769. doi: 10.1158/1538-7445.AM2014-4769
- Zhao X, Bodo J, Sun D, et al. Combination of ibrutinib with ABT-199: synergistic effects on proliferation inhibition and apoptosis in mantle cell lymphoma cells through perturbation of BTK, AKT and BCL2 pathways. Br J Haematol. 2015;168:765–768.
- Chen S, Dai Y, Harada H, et al. Mcl-1 down-regulation potentiates ABT-737 lethality by cooperatively inducing Bak activation and Bax translocation. Cancer Res. 2007;67:782–791.
- Kloo B, Nagel D, Pfeifer M, et al. Critical role of PI3K signaling for NF-kB-dependent survival in a subset of activated B-cell-like diffuse large B-cell lymphoma cells. Proc Natl Acad Sci USA. 2011;108:272–277.
- Campbell V, Thompson R, Villegas V, et al. The potent PI3K-dg, inhibitor IPI-145 exhibits differential activity in diffuse large B-cell lymphoma (DLBCL) cell lines. Paper presented at: 55th Annual Meeting of the American Society of Hematology; 2013 December 7–10; New Orleans, LA. Abstract 1832.
- Mathews Griner LA, Guha R, Shinn P, et al. High-throughput combinatorial screening identifies drugs that cooperate with ibrutinib to kill activated B-cell-like diffuse large B-cell lymphoma cells. Proc Natl Acad Sci USA. 2014;111:2349–2354.
- Lim KH, Romero DL, Chaudhary D, et al. IRAK4 kinase as a novel therapeutic target in the ABC subtype of diffuse large B cell lymphoma. Paper presented at: 54th Annual Meeting of the American Society of Hematology; 2012 December 8–11; Atlanta, GA. Abstract 62.
- Yang G, Zhou Y, Liu X, et al. A mutation in MYD88 (L265P) supports the survival of lymphoplasmacytic cells by activation of Bruton tyrosine kinase in Waldenstrom macroglobulinemia. Blood. 2013;122:1222–1232.
- Goldstein RL, Yang SN, Taldone T, et al. Pharmacoproteomics identifies combinatorial therapy targets for diffuse large B cell lymphoma. J Clin Invest. 2015;125:4559–4571.
- Rui L, Drennan AC, Ceribelli M, et al. Epigenetic gene regulation by Janus kinase 1 in diffuse large B-cell lymphoma. Proc Natl Acad Sci USA. 2016;113:E7260–E7267.
- Yang Y, Shaffer AL, III, Emre NC, et al. Exploiting synthetic lethality for the therapy of ABC diffuse large B cell lymphoma. Cancer Cell. 2012;21:723–737.
- Wilson WH, Popplewell L, Phillips T, et al. Multicenter phase 1b dose-escalation study of ibrutinib and lenalidomide combined with dose-adjusted EPOCH-R in patients with relapsed/refractory DLBCL. Paper presented at: 57th Annual Meeting of the American Society of Hematology; 2015 December 5–8; Orlando (FL). Abstract 1527.