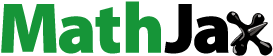
Abstract
Thrombocytopenia remains a challenge in myeloid malignancies, needing safer and more effective therapies. JNJ-26366821, a pegylated synthetic peptide thrombopoietin (TPO) mimetic not homologous to endogenous TPO, has an in-vitro EC50 of 0.2 ng/mL for the TPO receptor and dose dependently elevates platelets in volunteers. We demonstrate that JNJ-26366821 increases megakaryocytic differentiation and megakaryocytic colony formation in healthy controls and samples from myelodysplastic syndrome (MDS) and acute myeloid leukemia (AML). JNJ-26366821 had no effect on proliferation of malignant myeloid cell lines at doses up to 1000 ng/mL and malignant patient-derived mononuclear cells showed no increased cell growth or leukemic colony formation capacity at concentrations between 0.2 ng/mL and 10 ng/mL. Furthermore, JNJ-26366821 did not enhance in-vivo engraftment of leukemic cells in an AML xenotransplantation murine model. Our results show that JNJ-26366821 stimulates megakaryopoiesis without causing proliferation of the malignant myeloid clones in MDS/AML and provides the rationale for clinical testing of JNJ-26366821 in myeloid malignancies.
Introduction
Thrombocytopenia in patients with myelodysplastic syndromes (MDS) and acute myeloid leukemia (AML) is a result of ineffective hematopoiesis due to expansion of malignant clones in the bone marrow [Citation1–3]. Alleviation of thrombocytopenia is a desired clinical outcome, but the current standard of care mostly relies on platelet transfusions and while platelet components are widely available, recurrent transfusions have significant limitations, including potential transfusion-related complications and platelet transfusion refractoriness [Citation4,Citation5]. This population therefore, would benefit from safer and more effective treatment options. Furthermore, the most common indications among hematologic malignancies for a hematopoietic stem cell transplant (HSCT), includes high risk acute leukemias like AML or pre-leukemic conditions such as MDS. Among patients requiring a HSCT, including those with MDS and AML, thrombocytopenia and poor recovery of platelet counts at or following engraftment is a risk factor for morbidity and mortality [Citation6–9].
Thrombopoietin (TPO) receptor agonists have demonstrated clinical efficacy as targets for thrombocytopenia in patients with myeloid malignancies [Citation10,Citation11], particularly in low-risk MDS [Citation12–14]. However, clinical studies of higher-risk MDS and AML with the currently available agents, romiplastin and eltrombopag, had conflicting results with regards to efficacy [Citation15,Citation16], and strong data supporting their widespread use is lacking [Citation17]. Additionally, concerns regarding stimulation of malignant hematopoiesis hampered clinical development of these agents [Citation18]. Recently clinical studies with longer follow-up [Citation19,Citation20] and systematic reviews of large patient pools from clinical trials [Citation21,Citation22] have shown no significant safety concerns with these agents in myeloid malignancies. Nevertheless, before conducting clinical studies of new TPO mimetics in patients with MDS and AML who may proceed to HSCT, it is important to evaluate if the agent has potential stimulatory effect on malignant hematopoiesis.
JNJ-26366821 is a synthetic peptide polyethylene glycol (PEG)-conjugated TPO mimetic that has no sequence homology to endogenous TPO. It is a development stage compound that stimulates platelet production and has been shown to protect against thrombocytopenia in preclinical animal models [Citation23] and raise platelets in a dose dependent manner in healthy human volunteers [Citation24]. In addition to stimulating platelet production, JNJ-26366821 has also demonstrated protective effects against radiation-induced vascular injury and mortality in animal models of radiation toxicity [Citation25–27].
Results with JNJ-26366821 support its potential use as a new treatment option to target thrombocytopenia and vascular protection in patients with hematologic malignancies, such as MDS or AML, undergoing chemotherapy or myeloablative HSCT. Data evaluating the potential for JNJ-26366821 to cause proliferation of malignant myeloid clones is currently limited, therefore the objective of the experiments reported in this study is to evaluate its effects on proliferation of malignant clones from patients with MDS or AML. To address these questions, we evaluated the effects of JNJ-26366821 on myeloid malignant cells, including mononuclear cells (MNCs) from patients with MDS and AML, using cell proliferation and colony forming assays, differentiation cultures and a xenograft mouse model.
Materials and methods
Reagents
Stocks of JNJ-26366821 at concentrations of 60 µg/mL and 100 µg/mL were provided by Janssen Pharmaceuticals. The stock solutions were stored at –20 C, then allowed to come to room temperature and diluted as needed.
Cell lines
Cell lines MDS-L (myelodysplastic syndrome with del[5q]), THP-1 (acute monocytic leukemia), MOLM-13 (acute myeloid leukemia), MV-4-11 (biphenotypic B myelomonocytic leukemia) and CMK (acute megakaryocytic leukemia) were cultured in Roswell Park Memorial Institute 1640 medium (RPMI) containing 2.05 mM L-glutamine, supplemented with 10% fetal bovine serum (FBS) and 1% penicillin/streptomycin (PS). Culture was supplemented with 100 U/mL IL-3 for MDS-L and 50 μM 2-mercaptoethanol for THP-1. Cell line UT-7 (acute megakaryoblastic leukemia) was cultured in Iscove’s modified Dulvecco’s medium (IMDM) supplemented with 10% FBS, 1% PS and 1 ng/mL of granulocyte-macrophage colony-stimulating factor. Cell growth was monitored by a manual count of living cells with the 0.4% trypan blue viability exclusion test. Cell lines were obtained from the American Type Culture Collection (ATCC).
Proliferation assays
MDS-L, THP-1, MOLM-13, MV-4-11, UT-7 and CMK cells were plated at densities of 1 × 103 cells/mL and 5 × 103 cells/mL into 96-well plates with 100 µL of medium containing JNJ-26366821 in logarithmic increments from 0.01 ng/mL to 1000 ng/mL. After 72 h, cells were incubated with 20 µL of CellTiter-Blue reagent (CellTiter-Blue viability assay, Promega) and fluorescence was recorded with a microplate reader (FLUOstar Omega, BMG LabTech). Fluorescence versus JNJ-26366821 concentration was plotted after subtracting the average medium background from the raw values.
Patient samples
Consent was obtained from an IRB approved protocol to acquire primary bone marrow (BM) and/or peripheral blood (PB) samples during routine diagnostic procedures from patients with de novo AML (n = 14) and primary MDS (n = 10) treated at Montefiore Medical Center (). To guarantee sufficient number of mononuclear cells (MNCs), patients with known excess of CD34+ blasts were selected. Cytomorphology was reviewed; blast counts ranged between 32% and 91% in AML, and between 5% and 18% in MDS patients. Healthy controls included PB from volunteers (n = 2) and commercial CD34+ enriched stem cells (StemCell Technologies).
Table 1. Clinical characteristics of patients.
Isolation of mononuclear cells
MNCs from anticoagulated (ethylenediaminetetraacetic acid) BM aspirates or PB samples were isolated by density centrifugation using Ficoll-Hypaque (GE Healthcare). Cells were collected, washed with phosphate-buffered saline (PBS), evaluated for viability with trypan blue, reconstituted in IMDM with 10% FBS and immediately cultured for colony assays or fluorescent-activated cell sorting (FACS) analysis.
In vitro colony formation assays
For characterization of granulocytic-monocytic, erythrocytic and leukemic colony-forming units (CFUs), 2 × 103 CD34+ stem cells or 2 × 105 isolated MNCs per 1 mL of human MethoCult H4434 (StemCell Technologies) were plated in duplicates and cultured following the manufacturer’s recommendations. Cultures were untreated or contained JNJ-26366821 at concentrations of 0.2 ng/mL, 1 ng/mL, and 10 ng/mL. Colonies were scored using an inverted microscope after 14 days of incubation.
For characterization of megakaryocytic colonies (CFU-Mk), the MegaCult-C assay kit (StemCell Technologies) was utilized. In duplicates, 2 × 103 cells CD34+ stem cells or 2 × 105 MNCs per 1 mL of MegaCult-C were incubated in double-chamber slides for 12 days, following the manufacturer’s recommendations. Cultures were untreated or contained 0.2 ng/mL, 1 ng/mL, and 10 ng/mL of JNJ-26366821. Slides were fixed with methanol-acetone solution and immunocytochemically stained with anti–human GPIIb/IIIa (CD41a) antibody, biotin-conjugated goat anti–mouse IgG, avidin-alkaline phosphatase conjugate, and alkaline phosphatase substrate. CFU-Mk, defined as clusters of 3 or more CD41a + cells, and mixed colonies containing both CD41a + and CD41a − cells were scored using an inverted microscope.
Flow cytometric analysis
To measure expression of differentiation markers, patients’ MNCs were cultured for 12 days in semisolid methylcellulose medium containing hrIL-3, hrSCF, hrIL-6, insulin, and transferrin, supplemented with 0.2 ng/mL, 1 ng/mL or 10 ng/mL of JNJ-26366821. The medium was washed with PBS and cells were stained with the following fluorescent surface antibodies: anti–human CD45 (allophycocyanin [APC]; eBioscience), anti–human CD34 (fluorescein [FITC]; BioLegend), anti–human CD38 (BUV496; BDBioscience), anti–human CD41a (R-phycoerythrin [PE]; BDBioscience), anti–glycophorin A (PE-Cyanine 5; Invitrogen) and anti–human CD11b (Pacific Blue, Biolegend). Viability was assessed using 4.6-Diamidine-2-Phenylindole (DAPI) staining. Immunostained cells were analyzed using an LSRII FACS analyzer (BD Biosciences).
Western blot analysis
Phosphorylation of signal transducer and activator of transcription (STAT) 3 and STAT5 was assessed in lysates of CMK and UT-7 cells stimulated with JNJ-26366821 for 1–4 h. Proteins were loaded at 20 ug, fractionated by sodium dodecyl sulfate polyacrylamine gel electrophoresis and transferred to a polyvinylidene difluoride membrane. Membranes were incubated at 4 °C for 12 h with anti-human rabbit antibodies against phosphorylated(p)-STAT5, STAT5, p-STAT3, and STAT3, then washed and incubated for 2 h with a horseradish peroxidase-conjugated anti-rabbit antibody (Cell Signaling Technologies). Detection was performed with an enhanced chemiluminescence blotting analysis system (Amersham Biosciences). Actin was used as loading control.
Xenograft model
Non-obese diabetic-severe combined immunodeficiency-IL-2Rγ null (NSG) mice (The Jackson Laboratory) were irradiated (200 rads) and cells were transplanted by injecting 2 × 106 patient MNCs in 200 uL of PBS into the tail vein 24 h after irradiation. After 3 weeks, engraftment was assessed by determining the percentage of human cells by FACS analysis of BM aspirates from transplanted mice using an APC fluorescent anti-human CD45 antibody (eBioscience). Mice were considered successfully engrafted if they showed 1% or higher human derived CD45+ cells. Successfully transplanted mice were divided into two groups, the treatment group received 2 doses of 300 ug/Kg JNJ-26366821, administered 1 week apart via subcutaneous injection, and the control group was injected with PBS. BM aspiration was repeated 3 weeks after the first treatment dose and aspirates were analyzed by FACS to identify the percentage of CD45+ human cells. All animal experiments were performed in compliance with guidelines from the Institute for Animal Studies and approved by the Animal Institute Committee of the Albert Einstein College of Medicine.
Statistical analysis
Data are presented as the mean plus or minus standard deviation (SD), unless otherwise noted. Student t-test or one-way analysis of variance (ANOVA) tests were used to examine statistical significance between the means of two or more unrelated groups, respectively. Statistical significance was considered when p-value was < .05.
Results
Synthetic TPO mimetic, JNJ-26366821, stimulates megakaryopoiesis in normal hematopoietic precursors
We established the effect of JNJ-26366821 on megakaryocytic differentiation using CD34+ stem cells and MNCs from healthy volunteers. To examine the megakaryocytic clonogenic capacity, cells were plated in collagen-based semisolid medium, supplemented with JNJ-26366821 at doses of 0.2 ng/mL, 1 ng/mL and 10 ng/mL, and CFU-Mk were counted after 12 days. JNJ-26366821 significantly increased CFU-Mk formation at all JNJ-26366821 doses from both CD34+ cells and MNCs from healthy controls (). The effect was dose dependent, resulting in a mean increase of 1.7-fold among healthy controls for the half maximal effective concentration (EC50) dose of 0.2 ng/mL (p = .002) and up to a 2.2-fold increase for the highest dose (p < .001). Following previously described methods [Citation28], CFU-Mk were classified based on their morphology into small (arising from mature megakaryocytic precursors), medium or large (arising from immature precursors) and mixed (arising from immature megakaryocytic/erythrocytic precursors) (). A representative picture of the megakaryopoietic effect on a control sample is shown (). In MNCs from healthy volunteers and CD34+ stem cells, the megakaryopoietic effect from baseline to the 10 ng/mL dose, was seen across all subtypes and JNJ-26366821 enhanced the formation of mixed CFU-Mk up to 3-fold, medium/large CFU-Mk up to 2.5-fold and small CFU-Mk up to 2-fold compared to untreated cells (). To characterize the effect on granulocytic-monocytic and erythrocytic CFU formation in normal controls, CD34+ cells and healthy volunteers’ MNCs were plated in cytokine-enriched methylcellulose medium supplemented with JNJ-26366821 at the above-mentioned concentrations and CFUs were counted after 14 days. Treatment with increasing concentrations of JNJ-26366821 did not cause a significant change in the morphology () or the number and subtype distribution of granulocytic-monocytic and erythrocytic CFUs (). Together, these results demonstrate that at doses up to 10 ng/mL, JNJ-26366821 preferentially increased megakaryocytic potential in primary human stem and progenitor cells from healthy volunteers.
Figure 1. JNJ-26366821 stimulates megakaryopoiesis from normal hematopoietic progenitor cells. (A) CD34+ enriched human stem cells and MNCs obtained from normal volunteers (n = 2) were seeded in collagen-based semisolid medium supplemented with cytokines optimized for human megakaryocytic colony formation. Cultures were untreated or supplemented with JNJ-26366821 at concentrations of 0.2 ng/mL, 1 ng/mL and 10 ng/mL. Total number of CFU-Mk were counted after 12 days of incubation (*p < .05 by t-test when compared to untreated). (B) Based on morphology, CFU-Mk were classified as derived from most immature bipotent (mixed), immature (medium and large) and mature (small) megakaryocytic progenitors. (C) Representative low-power pictures of increased number of CFU-Mk with JNJ-26366821 treatment compared to untreated culture in a sample from a healthy control are shown. (D) Relative numbers of all different CFU-Mk subtypes are presented (*p < .05 by one-way ANOVA). (E) Non megakaryocytic colony formation was assessed by seeding control CD34+ enriched cells and MNCs in cytokine supplemented methycellulose-based medium and scoring the CFUs after 14 days of incubation. RWJ-800088 did not change CFU morphology as manually assessed on microscopy. Characteristic pictures of a CFUs from a healthy volunteer are shown. (F) JNJ-26366821 did not alter the formation or distribution of granulocytic-monocytic or erythrocytic CFUs. CFU-GEMM: Colony-forming unit-granulocyte, erythroid, macrophage and megakaryocyte. CFU-GM: Colony-forming unit-granulocyte-macrophage. BFU-E Burst-forming unit-erythroid. CFU-E: Colony-forming unit-erythroid. Results are presented as means of 2 independent experiments + SD.

JNJ-26366821 stimulates megakaryopoiesis in primary MDS/AML patient samples
MDS and AML are associated with dysplastic maturation of megakaryocytes with resulting thrombocytopenia. We tested whether JNJ-26366821 can stimulate megakaryopoiesis in MNCs derived from MDS and AML patients. MNCs were cultured in cytokine-enriched methylcellulose-based medium and differentiation surface markers were analyzed by FACS after 12 days of incubation. Treatment with JNJ-26366821 at doses of 0.2 ng/mL, 1 ng/mL and 10 ng/mL dose dependently increased surface expression of CD41a (). The relative number of CD41a-expressing megakaryocytic cells was significantly increased from untreated at all JNJ-26366821 concentrations in 3 of 4 MDS patients () and 4 of 6 AML patients (), indicating a significant effect on megakaryocytic differentiation. The mean increase in CD41a-expression for cells treated with 1 ng/mL was 2.2-fold (SD 0.4-fold) in MDS samples and 2.4-fold (SD 0.2-fold) in AML samples, compared to untreated cultures (). Treatment with JNJ-26366821 did not result in significant changes in differentiation surface marker expression of the granulocytic/monocytic or erythrocytic lineages at any of the tested concentrations (data not shown).
Figure 2. JNJ-26366821 stimulates megakaryopoiesis in MNCs derived from patients with MDS and AML. Patient derived MNCs were grown in cytokine-supplemented medium and treated with JNJ-26366821 at concentrations of 0.2 ng/mL, 1 ng/mL and 10 ng/mL for 12 days. Medium was washed with PBS and differentiation cell surface marker expression was determined by FACS. The relative number of CD41a-expressing megakaryocytic cells was calculated the effect of JNJ-26366821 was determined in comparison to untreated cultures. (A) A representative FACS histogram plot of cells from an AML patient sample cultured with JNJ-26366821 at different concentrations and stained with fluorescent CD41a antibody is shown. (B) In samples from patients with MDS, JNJ-26366821 numerically increased the CD41a-expressing cells in all cases (*p < .05 by one-way ANOVA). (C) In samples from patients with AML, the effect was also noted in most cases (*p < .05 by one-way ANOVA). (D) Average increase in CD41a-expressing cells is presented for all analyzed MDS and AML samples, showing a dose-dependent response (*p < .05 by t-test when compared to untreated, 1 MDS and 2 AML samples were not analyzed [N/A]). All results are presented as means of 2 independent experiments + SD.
![Figure 2. JNJ-26366821 stimulates megakaryopoiesis in MNCs derived from patients with MDS and AML. Patient derived MNCs were grown in cytokine-supplemented medium and treated with JNJ-26366821 at concentrations of 0.2 ng/mL, 1 ng/mL and 10 ng/mL for 12 days. Medium was washed with PBS and differentiation cell surface marker expression was determined by FACS. The relative number of CD41a-expressing megakaryocytic cells was calculated the effect of JNJ-26366821 was determined in comparison to untreated cultures. (A) A representative FACS histogram plot of cells from an AML patient sample cultured with JNJ-26366821 at different concentrations and stained with fluorescent CD41a antibody is shown. (B) In samples from patients with MDS, JNJ-26366821 numerically increased the CD41a-expressing cells in all cases (*p < .05 by one-way ANOVA). (C) In samples from patients with AML, the effect was also noted in most cases (*p < .05 by one-way ANOVA). (D) Average increase in CD41a-expressing cells is presented for all analyzed MDS and AML samples, showing a dose-dependent response (*p < .05 by t-test when compared to untreated, 1 MDS and 2 AML samples were not analyzed [N/A]). All results are presented as means of 2 independent experiments + SD.](/cms/asset/1258e94b-a301-42bc-98cf-7f2dc9edca56/ilal_a_1775213_f0002_c.jpg)
To determine the effect on megakaryocytic clonogenic capacity, malignant MNCs were cultured in collagen-based medium supplemented with JNJ-26366821 at the above-mentioned concentrations and CFU-Mk were counted after 12 days. As determined by an increased total number of CFU-Mk compared to untreated cultures, JNJ-26366821 stimulated megakaryocytic colony formation in 7 of 10 patient samples. Compared to untreated samples, the difference was statistically significant for all JNJ-26366821 concentrations in 3 of 4 MDS samples () and was most notable with the highest dose of 10 ng/mL, which increased the number of CFU-Mk in all 4 cases (mean 76%, SD 38.6%). Similarly, 4 of 6 AML samples yielded statistically significant results for all JNJ-26366821 concentrations compared to untreated samples () and the highest effect was seen with 10 ng/mL in all but one sample (mean 74%, SD 42.2%). Average increase in CFU-Mk formation in all samples was statistically significant from baseline to each dose group and results showed a dose-dependent trend (). The morphology of CFU-Mk derived from patient samples was similar to colonies obtained from healthy volunteers, including a slight predominance of immature mixed and large/medium subtypes (). Compared to untreated controls, large or medium CFU-Mk subtypes were slightly favored with JNJ-26366821 treatment, possibly indicating higher effect on earlier, more immature megakaryocytic precursors.
Figure 3. JNJ-26366821 stimulates megakaryocytic colony formation in MNCs derived from patients with MDS and AML. MNCs obtained from patients with MDS and AML were seeded in collagen-based semisolid medium supplemented with cytokines optimized for human megakaryocytic colony formation. Cultures were untreated or supplemented with JNJ-26366821 at concentrations of 0.2 ng/mL, 1 ng/mL or 10 ng/mL and the total number of CFU-Mk were counted after 12 days of incubation. (A) The number of CFU-Mk increased in all samples from patients with MDS (*p < .05 by one-way ANOVA). (B) Similarly, most individual results of samples from patients with AML show an increase in the number of CFU-Mk (*p < .05 by one-way ANOVA). (C) Average effect of JNJ-26366821 on the number of CFU-Mk for all analyzed MDS and AML samples (*p < .05 by t-test when compared to untreated, 1 MDS and 2 AML samples were not analyzed [N/A]) Results are presented as means of 2 independent experiments + SD. (D) Representative pictures of all CFU-Mk subtypes obtained from cultures of cells derived from an AML patient are shown to demonstrate that morphology of colonies derived from patients’ samples was similar to those seen with samples of healthy volunteers.
![Figure 3. JNJ-26366821 stimulates megakaryocytic colony formation in MNCs derived from patients with MDS and AML. MNCs obtained from patients with MDS and AML were seeded in collagen-based semisolid medium supplemented with cytokines optimized for human megakaryocytic colony formation. Cultures were untreated or supplemented with JNJ-26366821 at concentrations of 0.2 ng/mL, 1 ng/mL or 10 ng/mL and the total number of CFU-Mk were counted after 12 days of incubation. (A) The number of CFU-Mk increased in all samples from patients with MDS (*p < .05 by one-way ANOVA). (B) Similarly, most individual results of samples from patients with AML show an increase in the number of CFU-Mk (*p < .05 by one-way ANOVA). (C) Average effect of JNJ-26366821 on the number of CFU-Mk for all analyzed MDS and AML samples (*p < .05 by t-test when compared to untreated, 1 MDS and 2 AML samples were not analyzed [N/A]) Results are presented as means of 2 independent experiments + SD. (D) Representative pictures of all CFU-Mk subtypes obtained from cultures of cells derived from an AML patient are shown to demonstrate that morphology of colonies derived from patients’ samples was similar to those seen with samples of healthy volunteers.](/cms/asset/b93e4e2c-83d6-4544-99ec-ed68955d5e3f/ilal_a_1775213_f0003_c.jpg)
Together, these results indicate that JNJ-26366821 prominently acts on patient derived progenitor cells harboring megakaryocytic differentiation potential and is capable of increasing megakaryocytic differentiation and colony formation in samples of MDS and AML.
JNJ-26366821 does not increase proliferation of MDS/AML cell lines
The effect of JNJ-26366821 on various malignant myeloid cell lines was examined. Treatment of the AML cell lines THP-1, MOLM-13, MV-4-11, UT-7 and the MDS cell line MDS-L did not result in any significant stimulatory effect on proliferation assays or methylcellulose-based cultures at various JNJ-26366821 concentrations from 0.01 ng/mL up to 1000 ng/mL (). Treatment of the megakaryocytic leukemia cell line CMK resulted in a small stimulation in proliferation assays (), with a mean increase in proliferation of 12% compared to the untreated control, and highest stimulatory effect of 18% with a concentration of 1000 ng/mL. Though this effect suggests malignant proliferation, these results were not replicated in semisolid cultures () and the effect was only statistically significant with concentrations equal or higher than 1 ng/mL, corresponding to high doses over 5 times the EC50. Furthermore, treatment of the megakaryoblastic leukemia cell line UT-7, which also expresses the TPO receptor, did not replicate these results and there was no noticeable stimulation of UT-7 cells, even at a concentration of 1000 ng/mL ().
Figure 4. JNJ-26366821 does not enhance proliferation of malignant myeloid cell lines but does activate downstream targets of the TPO receptor. (A) MV-4-11, MOLM-13, THP-1, MDS-L, CMK and UT-7 cells were plated in 100 µL medium containing JNJ-26366821 in logarithmic increments from 0.01 ng/mL to 1000 ng/mL. After 72 h, viability was assessed with a fluorometric method. JNJ-26366821 at concentrations up to 1000 ng/ml had no effect on proliferation of the AML and MDS cell lines MV-4-11, MOLM-13, THP-1 and MDS-L. Treatment of CMK (a megakaryocytic cell line) but not UT-7 (a megakayoblastic cell line) resulted in a low, dose-dependent increase in proliferation only significant at doses higher than 1 ng/mL (p < .05 by t-test when compared to untreated, means of 3 independent experiments + SD, duplicated for 2 plating concentrations [not shown]). (B) Cell lines were also seeded in methylcellulose-based cultures supplemented with JNJ-26366821 at concentrations of 0.2 ng/mL, 1 ng/mL or 10 ng/mL. Total number of leukemic cells after incubation for 12 days were counted and compared to untreated controls. No stimulatory effect was seen in any of the cell lines, including CMK. (C) Proliferation curves of UT-7 cells did not replicate the small stimulation seen with CMK. (D) Suspension cultures of CMK and UT-7 cells were stimulated with 1 ng/mL of JNJ-26366821 for 1, 2, and 4 h. Total cell lysates were immunoprecipitated with anti-STAT3, anti-pSTAT3, anti-STAT5 and anti-pSTAT5 antibodies and analyzed with an enhanced chemiluminescence blotting analysis system. JNJ-26366821 induced phosphorylation of STAT5 but not STAT3 in both these cells types known to express the TPO receptor.
![Figure 4. JNJ-26366821 does not enhance proliferation of malignant myeloid cell lines but does activate downstream targets of the TPO receptor. (A) MV-4-11, MOLM-13, THP-1, MDS-L, CMK and UT-7 cells were plated in 100 µL medium containing JNJ-26366821 in logarithmic increments from 0.01 ng/mL to 1000 ng/mL. After 72 h, viability was assessed with a fluorometric method. JNJ-26366821 at concentrations up to 1000 ng/ml had no effect on proliferation of the AML and MDS cell lines MV-4-11, MOLM-13, THP-1 and MDS-L. Treatment of CMK (a megakaryocytic cell line) but not UT-7 (a megakayoblastic cell line) resulted in a low, dose-dependent increase in proliferation only significant at doses higher than 1 ng/mL (p < .05 by t-test when compared to untreated, means of 3 independent experiments + SD, duplicated for 2 plating concentrations [not shown]). (B) Cell lines were also seeded in methylcellulose-based cultures supplemented with JNJ-26366821 at concentrations of 0.2 ng/mL, 1 ng/mL or 10 ng/mL. Total number of leukemic cells after incubation for 12 days were counted and compared to untreated controls. No stimulatory effect was seen in any of the cell lines, including CMK. (C) Proliferation curves of UT-7 cells did not replicate the small stimulation seen with CMK. (D) Suspension cultures of CMK and UT-7 cells were stimulated with 1 ng/mL of JNJ-26366821 for 1, 2, and 4 h. Total cell lysates were immunoprecipitated with anti-STAT3, anti-pSTAT3, anti-STAT5 and anti-pSTAT5 antibodies and analyzed with an enhanced chemiluminescence blotting analysis system. JNJ-26366821 induced phosphorylation of STAT5 but not STAT3 in both these cells types known to express the TPO receptor.](/cms/asset/4a872487-adb0-4fb5-aaa6-3296b70e8a14/ilal_a_1775213_f0004_c.jpg)
To evaluate the effect on activation of downstream targets of the TPO receptor, we analyzed the phosphorylation of STAT3 and STAT5 after stimulation with JNJ-26366821 in 2 cell lines that express the TPO receptor. CMK and UT-7 cells were cultured in RPMI and IMDM, respectively, either untreated or stimulated with JNJ-26366821 at concentration of 1 ng/mL for up to 4 h. Phosphorylation of STAT proteins was assessed by immunoblotting of cell lysates with anti-p-STAT3 and anti-pSTAT5 antibodies. JNJ-26366821 rapidly induced phosphorylation of STAT5 in both cell lines within 1 h of exposure, and the effect was sustained up to the 4-hour time point (). By contrast, JNJ-26366821 did not induce phosphorylation of STAT3 in any of the cell lines.
JNJ-26366821 does not enhance malignant myeloid cell proliferation in vitro and in vivo
To address the concern of potential malignant hematopoiesis stimulation with JNJ-26366821 in myeloid neoplasms, MNCs from patients with MDS and AML were tested in methylcellulose-based clonogenic assays and all samples that exhibited a high-risk behavior and predominantly formed leukemic CFU in-vitro were examined. At concentrations of 0.2, 1 and 10 ng/mL, JNJ-26366821 did not cause an increase in the malignant clonogenic capacity in a statistically significant manner in any of the 14 patient samples analyzed (). Contrarily, some cultures exhibited noticeable decrease in the number of malignant colonies after treatment with JNJ-26366821. The mean inhibitory effect with 0.2 ng/mL (EC50) was 23.3%, however, due to interindividual variation, the effect was only statistically significant in 2 samples. On average, there was no stimulatory or inhibitory effect on leukemic colony formation in MDS and AML samples (). Furthermore, total cell growth was assessed in cultures of samples from MDS and AML patients and healthy controls. As determined by 12 days of incubation in methylcellulose, JNJ-26366821 did not stimulate total cell growth of patient-derived MNCs compared to untreated cultures ().
Figure 5. JNJ-26366821 does not enhance cell growth or leukemic clonogenic capacity of malignant MNCs derived from patients with MDS/AML. Patient-derived MNCs were seeded in semisolid methylcellulose-based medium containing hrIL-3, hrSCF, hrIL-6, insulin, and transferrin. Cultures were untreated or supplemented with JNJ-26366821 at concentrations of 0.2 ng/mL, 1 ng/mL and 10 ng/mL. Samples from patients with MDS (n = 6) or AML (n = 8) that showed high risk and led to formation cluster of poorly differentiated cells (malignant leukemic colonies) were analyzed after 14 days of incubation. (A) No stimulation of malignant colonies was noted on any MDS sample. Contrarily, one culture showed a significant decrease in the number of malignant colonies (*p < .05 by one-way ANOVA). (B) Similarly, malignant colonies did not significantly increase in any of the AML samples and one of them even showed a significant decrease (*p < .05 by one-way ANOVA). (C) On average, no stimulatory or inhibitory effect on leukemic colony formation was seen in MDS and AML. (D) Cumulative total cell growth in semisolid medium was also assessed after 12 days of incubation for patient samples and healthy controls (n = 2). Results are presented as means of 2 independent experiments + SD.

To specifically assess the immature myeloid compartment, we examined surface marker expression by FACS within the ex-vivo cultures of MNCs from MDS and AML patients and identified the relative number of CD34+ CD38- stem cells that did not express final granulocytic, erythrocytic or megakaryocytic differentiation markers. Leukemia initiating cells are known to constitute a minor subpopulation of this compartment, and the proportion is reflective of their frequency in culture [Citation29,Citation30]. Compared to untreated cultures, JNJ-26366821 did not cause an increase in the relative number of this cell compartment in any of the 10 patient samples analyzed (). A small decrease was noted in most cultures of MNCs from patients with MDS, however, the relative low numbers prevent assessment of statistical significance of this inhibitory effect. On average, there was no increase in the relative number of these immature cells at any of the tested concentrations ().
Figure 6. JNJ-26366821 does not enhance malignant myeloid growth in vitro and in vivo. MNCs derived from patients with MDS (n = 4) or AML (n = 6) were grown in cytokine-supplemented medium and incubated for 12 days with JNJ-26366821at concentrations of 0.2 ng/mL, 1 ng/mL and 10 ng/mL. Cell surface marker expression was determined by FACS and the relative abundance of immature CD34+ CD38- cells was calculated. (A) Individual results of samples from patients with MDS showed a slight decrease in most samples which was not statistically significant. (B) Treatment with JNJ-26366821 did not increase the relative abundance of these immature cells in any of the AML samples. (C) On average, there was no noticeable increase in immature CD34+ cells in cultures from patients with MDS and AML. Results are presented as means of 2 independent experiments + SD. (D) A murine xenotransplantation model was used. MNCs derived from a p53-mutant AML patient were transplanted into NSG mice after sublethal irradiation and successfully engrafted mice were treated with weekly subcutaneous injections of either 300 ug/Kg JNJ-26366821 or PBS. Bone marrow aspirates were obtained 3 weeks after the first treatment date and analyzed for human CD45-expressing by FACS. (E) Fold-increase increased in chimerism of human CD45+ cells in xenografted mice was not different with or without treatment with JNJ-26366821. (F) Relative increased percentage of human cells engrafted into xenotransplanted mice was also unchanged with JNJ-26366821 treatment (p-value for t-test, when compared to control, data presented as means for JNJ-26366821 [6 mice] and control [4 mice] + SEM).
![Figure 6. JNJ-26366821 does not enhance malignant myeloid growth in vitro and in vivo. MNCs derived from patients with MDS (n = 4) or AML (n = 6) were grown in cytokine-supplemented medium and incubated for 12 days with JNJ-26366821at concentrations of 0.2 ng/mL, 1 ng/mL and 10 ng/mL. Cell surface marker expression was determined by FACS and the relative abundance of immature CD34+ CD38- cells was calculated. (A) Individual results of samples from patients with MDS showed a slight decrease in most samples which was not statistically significant. (B) Treatment with JNJ-26366821 did not increase the relative abundance of these immature cells in any of the AML samples. (C) On average, there was no noticeable increase in immature CD34+ cells in cultures from patients with MDS and AML. Results are presented as means of 2 independent experiments + SD. (D) A murine xenotransplantation model was used. MNCs derived from a p53-mutant AML patient were transplanted into NSG mice after sublethal irradiation and successfully engrafted mice were treated with weekly subcutaneous injections of either 300 ug/Kg JNJ-26366821 or PBS. Bone marrow aspirates were obtained 3 weeks after the first treatment date and analyzed for human CD45-expressing by FACS. (E) Fold-increase increased in chimerism of human CD45+ cells in xenografted mice was not different with or without treatment with JNJ-26366821. (F) Relative increased percentage of human cells engrafted into xenotransplanted mice was also unchanged with JNJ-26366821 treatment (p-value for t-test, when compared to control, data presented as means for JNJ-26366821 [6 mice] and control [4 mice] + SEM).](/cms/asset/d0db3b65-5c7b-42b5-9c57-95425f5e955c/ilal_a_1775213_f0006_c.jpg)
The effect of JNJ-26366821 in vivo was assessed using a xenotransplantation model (). MNCs from a p53-mutant AML patient were transplanted into sub-lethally irradiated NSG mice and human cell engraftment was determined by FACS of BM aspirates 3 weeks after transplantation. Successfully engrafted mice were divided into a treatment group that received weekly JNJ-26366821 administered via subcutaneous injection at a dose of 300 ug/Kg, and a control group that received injections of PBS. BM aspirates were repeated 3 weeks after the first dose and analyzed by FACS. Compared to control mice, JNJ-26366821 did not result in increased human AML cell engraftment in NSG mice. The average increase in CD45+ human cells in murine BM was 5.6- fold for JNJ-26366821 vs. 6.1-fold for control (p = NS, ). Consistent with ex-vivo findings, JNJ-26366821 did not enhance in-vivo engraftment of malignant cells in the xenotransplantation murine model ().
Discussion
We demonstrate that the novel PEG-conjugated TPO mimetic, JNJ-26366821, is capable of increasing megakaryocytic differentiation and formation of normal megakaryocytic colonies in cells derived from healthy volunteers () and patients with MDS and AML ( and ), without stimulating cell growth or clonogenic capacity in malignant myeloid cells (). There was no evidence of a stimulatory effect on the malignant myeloid clone in multiple cell lines () as well as in the primary patient samples tested and in an AML xenograft mouse model (). Of note, we observed a small reduction in proliferation in 2 cell lines, MOLM-13 and MDS-L, a lower number of immature CD34+ CD38- cells in most MDS samples and decreased malignant clonogenic capacity in 2 MDS/AML samples. Due to the variance in the data, our overall results show no significant inhibitory effect in malignant myeloid cells, but we cannot exclude the possibility that JNJ-26366821 might have an antiproliferative effect in specific subgroups of MDS and AML through an unknown mechanism. The nonpeptide small molecule TPO mimetic eltrombopag has shown an antileukemic effect that is mediated by its ability to chelate intracellular iron and decrease the level of reactive oxygen species, which leads to cell cycle arrest and stimulates differentiation in leukemic cells [Citation31,Citation32]. Thus, further mechanistic studies with JNJ-26366821 are needed to elucidate its effect at a molecular level.
Our study is the first to examine JNJ-26366821 within the context of hematologic malignancies and our results are evidence that it is capable of stimulating megakaryopoiesis in MNCs derived from patients with MDS and AML. The morphology of megakaryocytic colonies obtained upon treatment of patient derived MNCs with JNJ-26366821 was similar to that obtained from normal controls and suggested an expected preferential effect on early megakaryocytic progenitors, consistent with the mechanism of action of TPO mimetics. Furthermore, although these results are limited by the lack of an extracellular matrix that may affect malignant cell differentiation, there was no evidence that JNJ-26366821 stimulated malignant myeloid cellular proliferation or growth in in vitro cultures and clonogenic assays or in a xenotransplantation assay in vivo. As establishing safety was the main objective of this study, tests were conducted using samples derived from MDS and AML patients with an excess of blasts, which in the case of MDS led to selection of individuals with high-risk disease. These patients are frequently affected by clinically significant thrombocytopenia or require HSCT in the context of their anti-leukemic therapy. A limitation of this study is lack of experimental data on low-risk MDS samples, as this group of patients might benefit greatly from new thrombopoietin mimetics targeting thrombocytopenia.
Thrombocytopenia remains a clinical challenge in patients with MDS and AML [Citation33]. It is associated with delays and dose reductions of chemotherapy [Citation34], causes moderate to severe bleeding complications in 18% of MDS and 11% of AML patients [Citation1,Citation2], some of which lead to hemorrhagic death [Citation3], and constitutes an independent factor for decreased survival [Citation35,Citation36]. Platelet transfusion is the only currently available option to transiently increase platelet counts in patients with clinically significant thrombocytopenia. However, recurring transfusions are expensive and can be associated with febrile, allergic and infectious transfusion-related complications or alloimmunization, which causes platelet transfusion refractoriness [Citation4,Citation37]. Thus, enhancing platelet production constitutes an unmet need in MDS and AML, and alternative pharmacological strategies to stimulate megakaryopoiesis could greatly benefit patients suffering from thrombocytopenia [Citation35,Citation38]. Our results provide the preclinical rationale and support for the evaluation of using this novel TPO mimetic as a pharmacologic option for patients with AML and MDS, in order to improve thrombocytopenia and improve patient outcomes without promoting a relapse of the underlying malignancy. Furthermore, additional research is needed to examine if the vascular protection seen in radiation-induced vascular injury models can be beneficial for this population when administering therapeutic modalities such as HSCT.
Acknowledgements
We thank the Albert Einstein College of Medicine Flow Cytometry Core Facility and Xenotransplantation Facility for their expert technical assistance.
Mary Guilfoyle, Senior Director and Compound Development Team Leader from Johnson & Johnson provided editorial assistance to the authors during the preparation of this manuscript.
Disclosure statement
Funding for this work was provided by Janssen R&D, LLC. M.M and G.E., coauthors from Janssen R&D LLC, are employees and shareholders of Johnson and Johnson and potentially stand to benefit financially from the development and commercialization of JNJ-26366821. Montefiore Medical Center and C.G. may potentially benefit financially from the development and commercialization of JNJ-26366821 as co-inventors on one of the patents. A.V. has received funding from Janssen, GSK, Incyte, Medpacto, and Eli Lilly and is a scientific advisor for Stelexis, Novartis, Acceleron and Celgene. C.G. has received funding from Janssen.
Additional information
Funding
References
- Kantarjian H, Giles F, List A, et al. The incidence and impact of thrombocytopenia in myelodysplastic syndromes. Cancer. 2007;109(9):1705–1714.
- Webert K, Cook RJ, Sigouin CS, et al. The risk of bleeding in thrombocytopenic patients with acute myeloid leukemia. Haematologica. 2006;91(11):1530–1537.
- Neukirchen J, Blum S, Kuendgen A, et al. Platelet counts and haemorrhagic diathesis in patients with myelodysplastic syndromes. Eur J Haematol. 2009;83(5):477–482.
- Blumberg N, Heal JM, Phillips GL, et al. Platelets–to transfuse or not to transfuse. Lancet. 2012;380(9850):1287–1289.
- Comont T, Tavitian S, Bardiaux L, et al. Platelet transfusion refractoriness in patients with acute myeloid leukemia treated by intensive chemotherapy. Leuk Res. 2017;61:62–67.
- Akahoshi Y, Kanda J, Gomyo A, et al. Risk factors and impact of secondary failure of platelet recovery after allogeneic stem cell transplantation. Biol Blood Marrow Transplant. 2016;22(9):1678–1683.
- Bolwell B, Pohlman B, Sobecks R, et al. Prognostic importance of the platelet count 100 days post allogeneic bone marrow transplant. Bone Marrow Transplant. 2004;33(4):419–423.
- Bruno B, Gooley T, Sullivan KM, et al. Secondary failure of platelet recovery after hematopoietic stem cell transplantation. Biol Blood Marrow Transplant. 2001;7(3):154–162.
- Kim DH, Sohn SK, Baek JH, et al. Clinical significance of platelet count at day +60 after allogeneic peripheral blood stem cell transplantation. J Korean Med Sci. 2006;21(1):46–51.
- Shastri A, Verma AK. Eltrombopag reduces clinically relevant thrombocytopenic events in higher risk MDS and AML. Lancet Haematol. 2018;5(1):e6–e7.
- Rodeghiero F, Carli G. Beyond immune thrombocytopenia: the evolving role of thrombopoietin receptor agonists. Ann Hematol. 2017;96(9):1421–1434.
- Giagounidis A, Mufti GJ, Fenaux P, et al. Results of a randomized, double-blind study of romiplostim versus placebo in patients with low/intermediate-1-risk myelodysplastic syndrome and thrombocytopenia. Cancer. 2014;120(12):1838–1846.
- Greenberg PL, Garcia-Manero G, Moore M, et al. A randomized controlled trial of romiplostim in patients with low- or intermediate-risk myelodysplastic syndrome receiving decitabine. Leuk Lymphoma. 2013;54(2):321–328.
- Oliva EN, Alati C, Santini V, et al. Eltrombopag versus placebo for low-risk myelodysplastic syndromes with thrombocytopenia (EQoL-MDS): phase 1 results of a single-blind, randomised, controlled, phase 2 superiority trial. Lancet Haematol. 2017;4(3):e127–e136.
- Frey N, Jang JH, Szer J, et al. Eltrombopag treatment during induction chemotherapy for acute myeloid leukaemia: a randomised, double-blind, phase 2 study. Lancet Haematol. 2019;6(3):e122–e131.
- Dickinson M, Cherif H, Fenaux P, et al. Azacitidine with or without eltrombopag for first-line treatment of intermediate- or high-risk MDS with thrombocytopenia. Blood. 2018;132(25):2629–2638.
- Bussel JB. The new thrombopoietic agenda: impact on leukemias and MDS. Best Pract Res Clin Haematol. 2014;27(3–4):288–292.
- Santini V. Treatment of low-risk myelodysplastic syndrome: hematopoietic growth factors erythropoietins and thrombopoietins. Semin Hematol. 2012;49(4):295–303.
- Mittelman M, Platzbecker U, Afanasyev B, et al. Eltrombopag for advanced myelodysplastic syndromes or acute myeloid leukaemia and severe thrombocytopenia (ASPIRE): a randomised, placebo-controlled, phase 2 trial. Lancet Haematol. 2018;5(1):e34–e43.
- Fenaux P, Muus P, Kantarjian H, et al. Romiplostim monotherapy in thrombocytopenic patients with myelodysplastic syndromes: long-term safety and efficacy. Br J Haematol. 2017;178(6):906–913.
- Prica A, Sholzberg M, Buckstein R. Safety and efficacy of thrombopoietin-receptor agonists in myelodysplastic syndromes: a systematic review and meta-analysis of randomized controlled trials. Br J Haematol. 2014;167(5):626–638.
- Dodillet H, Kreuzer KA, Monsef I, et al. Thrombopoietin mimetics for patients with myelodysplastic syndromes. Cochrane Database Syst Rev. 2017;9:CD009883.
- Knight E, Eichenbaum G, Hillsamer V, et al. Nonclinical safety assessment of a synthetic peptide thrombopoietin agonist: effects on platelets, bone homeostasis, and immunogenicity and the implications for clinical safety monitoring of adverse bone effects. Int J Toxicol. 2011;30(4):385–404.
- Liem-Moolenaar M, Cerneus D, Molloy CJ, et al. Pharmacodynamics and Pharmacokinetics of the Novel Thrombopoietin Mimetic Peptide RWJ-800088 in Humans. Clin Pharmacol Ther. 2008;84(4):481–487.
- Ashcraft KA, Choudhury KR, Birer SR, et al. Application of a novel murine ear vein model to evaluate the effects of a vascular radioprotectant on radiation-induced vascular permeability and leukocyte adhesion. Radiat Res. 2018;190(1):12–21.
- Ashcraft KA, Hannan JL, Eichenbaum G, et al. Clarifying the relative impacts of vascular and nerve injury that culminate in erectile dysfunction in a pilot study using a rat model of prostate irradiation and a thrombopoietin mimetic. Int J Radiat Oncol Biol Phys. 2019;103(5):1212–1220.
- Langer HF, Stellos K, Steingen C, et al. Platelet derived bFGF mediates vascular integrative mechanisms of mesenchymal stem cells in vitro. J Mol Cell Cardiol. 2009;47(2):315–325.
- Will B, Kawahara M, Luciano JP, et al. Effect of the nonpeptide thrombopoietin receptor agonist Eltrombopag on bone marrow cells from patients with acute myeloid leukemia and myelodysplastic syndrome. Blood. 2009;114(18):3899–3908.
- Langenkamp U, Siegler U, Jorger S, et al. Human acute myeloid leukemia CD34 + CD38- stem cells are susceptible to allorecognition and lysis by single KIR-expressing natural killer cells. Haematologica. 2009;94(11):1590–1594.
- Seita J, Weissman IL. Hematopoietic stem cell: self-renewal versus differentiation. Wiley Interdiscip Rev Syst Biol Med. 2010;2(6):640–653.
- Roth M, Will B, Simkin G, et al. Eltrombopag inhibits the proliferation of leukemia cells via reduction of intracellular iron and induction of differentiation. Blood. 2012;120(2):386–394.
- Kalota A, Selak MA, Garcia-Cid LA, et al. Eltrombopag modulates reactive oxygen species and decreases acute myeloid leukemia cell survival. PLoS One. 2015;10(4):e0126691.
- Basood M, Oster HS, Mittelman M. Thrombocytopenia in patients with myelodysplastic syndromes – still an unsolved problem. Mediterr J Hematol Infect Dis. 2018;10(1):e2018046.
- Gao C, Wang J, Li Y, et al. Incidence and risk of hematologic toxicities with hypomethylating agents in the treatment of myelodysplastic syndromes and acute myeloid leukopenia: a systematic review and meta-analysis. Medicine (Baltimore). 2018;97(34):e11860.
- Li W, Morrone K, Kambhampati S, et al. Thrombocytopenia in MDS: epidemiology, mechanisms, clinical consequences and novel therapeutic strategies. Leukemia. 2016;30(3):536–544.
- Sanz GF, Sanz MA, Greenberg PL. Prognostic factors and scoring systems in myelodysplastic syndromes. Haematologica. 1998;83(4):358–368.
- Brand A. Immunological complications of blood transfusions. Presse Med. 2016;45(7–8 Pt 2):e313–e324.
- Desborough M, Estcourt LJ, Doree C, et al. Alternatives, and adjuncts, to prophylactic platelet transfusion for people with haematological malignancies undergoing intensive chemotherapy or stem cell transplantation. Cochrane Database Syst Rev. 2016;22(8):CD010982.