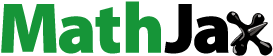
ABSTRACT
In the advanced progress of the synthesis of metal nanoparticles, the use of a bio-mimicking approach has been extensively studied and recognised as a non-toxic method. The use of Ag-NPs synthesised from inorganic compounds using plant extracts as surfactants are quite novel. Therefore, this study was carried out by synthesising and characterising Ag-NPs using a composite leave and bark extract solution of Croton macrostachyus as a capping agent. A 1:3 composite solution exhibited small nanoparticles with a rod-like shape that were active against bacteria. In addition, Ag-NPs were observed from leaf and bark extracts of a 1:1 components, as well as sand mall NPs from leaf extract, showing that leaf extract solution contained more reducing biomolecules. Furthermore, we studied the antibacterial activity of the synthesised NPs following the disc diffusion method (by preparing gram-negative and gram-positive bacteria). Thus, the synthesised NPs were active on Streptococcus pyogen compared with Pseudomonas aeruginosa bacteria.
Introduction
Nanotechnology is the engineering of functional systems on a nanoscale region. The field of nanoscience and technology has gained great importance because of their potential applications in various areas, such as chemicals, textile industries, materials industry, medical diagnostic, drug, gene delivery and electronics, artificial implants and tissues engineering [Citation1], computing, biochemical sensors [Citation2], medical imaging and so on [Citation3]. Nanoparticles, especially inorganic nanoparticles have been the subject of research for several years due to their diverse application possibilities and advantages [Citation4] Silver and gold nanoparticles have a wide range of applications, especially in the medical field. The therapeutic use of these nanoparticles is being researched. The availability, compatibility and ability of these nanoparticles to act as effective tools for medical imaging as well as treatment are currently being demonstrated [Citation5]. Nanotechnology is being used in diverse areas like chemistry, biology, catalysis, medicine, photonics, electronics, bio-labelling and information storage. Due to their unique physico-chemical properties of nanoparticles, including catalytic activity, optical and electronic properties, as well as cytotoxic and antimicrobial properties, in recent years, scientists showed gaining interest towards the development of novel methods for synthesis of nanoparticles [Citation6].
Metal nanoparticles with a high fraction of surface atoms and a large specific surface area have been extensively studied due to their unique physicochemical properties, such as catalytic activity, optical properties, electronic properties, antibacterial properties, and magnetic properties [Citation7]. The synthesis of noble nanoparticles for diverse applications including electronics, environmental science, and biotechnology is a growing field of study [Citation8], which uses electrochemical techniques [Citation9] microwave assisted process [Citation10] and now a day via green chemistry route [Citation11]. The use of plants in the synthesis of nanoparticles is new and leads to truly green chemistry that offers an advance over chemical and physical methods. Due to its positive impact, it is inexpensive, environmentally friendly, and easily scaled up for and at large-scale synthesis; method does not require high pressure, energy, temperature and toxic chemicals. Bacteria and fungi could be used for the synthesis of nanoparticles [Citation12] but use of leaf extract reduce the cost and do not require any special culture preparation and isolation techniques [Citation13]. Because of the growing need for clean, non-toxic chemicals, environmentally friendly solvents, and renewable materials, the biosynthesis of nanoparticles has received significant attention in recent years [Citation9]. Biological processes based on bacteria, fungi, bio-derived chemicals, and plant extracts are being extensively researched due to their environmentally friendly protocol and improved morphological control [Citation10].
Croton macrostachyus Hochst. ex Delile is a member of the Croton L. Euphorbiaceae family, also known as the Spurge family. It is a medium-sized, drought-bearing pioneer tree that regenerates naturally in less productive sites such as forest edges, mountain slopes, and fallow land under a variety of ecological conditions [Citation11]. Subsistence farmers in Ethiopia, Kenya, and Tanzania regard C. macrostachyus as a multipurpose tree because it is frequently grown and managed in home gardens to provide a variety of ecosystem goods and services [Citation14,Citation15].
For example, in Ethiopia, C. macrostachyus is an important tree interplant in agroecosystems to increase soil productivity in mid- and semi-arid areas [Citation14]. C. macrostachyus’ medicinal uses and pharmacological properties are also of great interest throughout its range in tropical Africa [Citation6–8]. Traditional herbal remedies are used by approximately 80% of the rural population in sub-Saharan Africa for primary health care and veterinary purposes [Citation16]. These scholars research revealed that C. macrostachyus is an important medicinal plant in tropical Africa with the potential to provide important pharmaceutical products to rural and urban communities that rely on herbal medicines for primary health care.
The world is still facing enormous problems due to number of ailments since time immemorial. Among these ailments, infectious diseases took tremendous part affecting both human and livestock throughout the world. The agents for infectious diseases are various including viruses, bacteria, fungi, protozoans, and other parasites. These agents can harbour different body parts of their hosts, which could be internal or external and can lead to more complicated situations that might end up with mortality [Citation17,Citation18].
Internally infectious agents can affect cells, tissues, organs or even body system. Skin, eye, mouth and nostrils are the most vulnerable external part of the animal body to be affected by such agents especially by bacteria, fungi and viruses [Citation19]. An animal body may respond to effects of infections to get rid of them by its immune system. However, most of the time, the effect of infection outweighs and become beyond the immune system of the animal leading to complications. As a result, different effects can be observed including irritations, rushes, inflammations, gastrointestinal disorders, and the likes. If situations reach these levels, the body has to get external supports that can help to boost and complement its immune systems so that it can get relief of infection [Citation20,Citation21].
Synthetic chemical bactericidal and fungicides are widely used in conventional agriculture to control plant and crop diseases. Environmental hazards caused by excessive use of pesticides pose health problems, as modern society is becoming more health-conscious [Citation22]. Therefore, scientists in the agricultural field are looking for an alternative, environmentally friendly and less capital-intensive approaches to combating bacterial diseases. As an alternative to chemically manufactured drugs, the use of silver nanoparticles as antimicrobial agents has become more common as technological advances make them more economical to manufacture [Citation23].
Muthukumaran et al. [Citation17] synthesised Ag-Np from Cassia roxburghii. Hence, the potential application of eco-friendly and cheapest plant (C. macrostachyus) to synthesise antibacterial agent of nanoparticles (such as Ag-Nps) trigger us to conduct further studies. UV-Visible spectrophotometer (Metash UV-visible 9000 Spectrophotometer) to confirm the formation and stability of silver nanoparticles in aqueous solution, scanning electron microscope (SEM, Hitachi S-4500) to study the morphological behaviour of the synthesised nanoparticle, energy dispersive X-ray (EDX, JEOL-MODEL 6390) for elemental mapping, Fourier transform infrared spectroscopy (FT-IR, Perkin Elmer 65) for functional group identification and X-ray diffraction (XRD) (XRD-700, Shimadzu, South Korea) are used for analysis.
Materials and Methods
Chemicals and reagents
Silver nitrate (AgNO3, Sigma Aldrich), (NaOH, Sigma Aldrich), (Mueller-Hinton agar, Sigma Aldrich), dimethyl sulphoxide (DMSO) and ethanol were used without further purification.
Sample collection
Healthy and fresh C. macrostachyus leaves and barks specimens (approximately 100 g of each) were collected around wolkite town (Gurage zone, South Nation Nationality and Regional state, Ethiopia) using a pre-cleaned plastic bag. The collected samples (both leaves and barks) were washed several times with running water and rinsed with DI water to remove adsorbed foreign particles. Finally, the specimens were dried at room temperature and ground to fine powder by using grinder and stored in a controlled environment for further studies.
Preparation of leaf and bark solution of Croton macrostachyus
A composite suspension was prepared by dispersing about 50 g of leaves powder into 300 mL of deionised water and stirring at 50°C for 30 min using a magnetic hot plate. Then, the suspension was allowed to cool at room temperature and filtered by using Wattman (90 mm diameter) filter paper. The suspension of leaf extract was then stored at 4°C refrigerator to prevent moisture effect. The same procedure was used to prepare bark composite suspension from C. macrostachyus [Citation24].
Biosynthesis of silver nanoparticles (Ag-NPs)
Biomediated silver nanoparticles were synthesised by mixing a composite solution of C. macrostachyus with 0.1 M AgNO3. A 1:1 v/v of leaf and bark composite solution of C, macrostachyus was added into a 250 mL volumetric flask. Then after three different composite solutions were prepared. The first sample comprises 1:3 v/v of 0.1 M AgNO3 and composite solution of leaf and bark, the second sample constitutes 1:1 v/v of 0.1 M AgNO3 and composite solution of leaf and bark, the third sample comprises 3:1 v/v of 0.1 M AgNO3 and composite solution of leaf and bark. Additionally, 1:1 v/v of 0.1 M AgNO3 and samples were prepared separately with leaf and bark extracts. All samples were stirred magnetically for 2:30 h under pH-controlled conditions. Lastly, 1 M NaOH solution was added drop-by-drop into each solution until a dark brown colour was obtained. The solution was subjected to centrifugation at 12,000 rpm for 15 min, followed by washing many times with ethanol and double distilled water to remove the impurities. Then, the synthesised Ag nanoparticle precipitates were dried for 12 h at room temperature and ground to a fine powder [Citation25]. We presented the synthesis mechanism of Ag NPs is shown in the .
Method of antibacterial study
Bacterial media was prepared for both gram-positive and gram-negative bacteria to examine the synthesised Ag-NPs. Incubation and determination of the antimicrobial activity of Ag NPs were performed by the disk diffusion method. The preparation of inoculums was performed by taking 1.5 g nutrient broth agar followed by dissolving it in 100 mL deionised water H2O. The prepared cultures were then sub-cultured and inoculated within a nutrient broth agar and were kept on a rotary shaker at 37°C for about 24 h at 160 rpm. Furthermore, the nutrient agar media were then prepared by taking 6 g nutrient agar and 0.8 g agar-agar dissolving the two mixtures in 100 mL of DI H2O. The formed suspension was then used to inoculate plates by dipping a sterile cotton wool swab into the resulting suspension. Before applying the antibiotic disks, the plates were confirmed complete drying. The inoculated plates were incubated at 37°C for about 24 h within an incubator for Pseudomonas aeruginosa and Streptococcus pyogen strains and were then checked for the zone of inhibition [Citation18].
Solution preparation of Ag NPs for diffusion testing method
Following previously reported procedure [Citation19], 1 mg of Ag-NPs was dispersed into 5 mL of DMSO (Note: this solvent does not affect bacteria), which yields a total concentration of 0.001854 M Ag-NP. Then, an optimum amount of Ag-NPs was dropped onto the pre-prepared bacterial spot.
Characterisation of techniques
In the current study, we used a scanning electron microscope (SEM, Hitachi S-4500) to characterise the surface morphological phenomenon of Ag-NPs. In addition, EDX analysis which employed to study the elemental constituents of Ag-NPs, with XRD (XRD-700, Shimadzu, South Korea) which was applied to study the crystalline structure of the fabricated Ag-NPs, UV-Visible spectroscopic technique (Metash UV-visible 9000 spectrophotometer) which employed to analyse the optical properties of the syntheses Ag-NPs. Moreover, Fourier transforms–infrared spectroscopy (FT-IR, Perkin Elmer 65) which was applied to identify the biomolecules that bound specifically on the silver surface and local molecular environment of surfactant on the Ag-NPs, were used for sample analysis.
Results and discussion
Optical and morphological Study
The extracts of Ag-NPs using different ratios of silver nitrate and Croton macrostachyus Leaf and bark solution afford different colours, as shown in . The formation of those different coloured product from similar starting materials revealed that the formation of different size Ag-NPs. The role played by leaf and bark extracts of C. macrostachyus is to acts as an end-capping agent in the formation of Ag-NPs.
Table 1. Ratio of precursor to plant extract and size of nanoparticle.
The surface morphologies and chemical composition of synthesised Ag nanoparticle at different concentrations of composite broth solution of leaf and bark extract of C. macrostachyus and the EDX images with their corresponding elemental distribution are indicated in ) and .
Figure 3. EDX images of (a) 1:3, (b) 1:1, (c) 3:1, (d) 1:1 (leaf) and (e) 1:1 (bark) of Ag-NPs with their corresponding elemental distribution [(i: Ag), (ii: O), (iii: N)] and mapping (iv)].
![Figure 3. EDX images of (a) 1:3, (b) 1:1, (c) 3:1, (d) 1:1 (leaf) and (e) 1:1 (bark) of Ag-NPs with their corresponding elemental distribution [(i: Ag), (ii: O), (iii: N)] and mapping (iv)].](/cms/asset/2443931c-0257-44e2-a11b-927b053c7b2e/ymte_a_2164647_f0003_oc.jpg)
The surface morphology of silver nanoparticles showed in ), synthesised with more leaf and bark extract composite solution concentration of C. macrostachyus shows rod shape morphology. This will efficiently inhibit rod shape microorganism because its morphology directly correlate with shape of microorganism. Since more concentrated composite solution of organic extract is used as reducing (capping) agent, small size Ag nanoparticle is obtained in 1:3 ratio. Similarly, ) shows rod like morphology of Ag nanoparticle, which has equal proportional ratio of composite solution and precursor concentration. ) shows more of spherical like morphology. Size of this nanoparticle is higher as compared with morphology of 1:3 and 1:1 [see ), respectively] because the reducing (capping) is lower than the precursor concentration. Morphology of ) shows spherical shape Ag nanoparticle, which will be favourable to inhibit spherical shape bacteria. This nanoparticle is synthesised with equal proportion of C. macrostachyus leaf extract and precursor solution. Morphology of shows flower shape, which is favourable to inhibit spherical shape microorganism. This nanoparticle is synthesised with equal proportion of C. macrostachyus bark extract and precursor solution.
Structural characterisation
In this analysis, the absorbance spectrum of the colloidal sample (i.e. 1:3, 1:1, 3:1, 1:1(l), and 1:1(b)) NPs were analysed using Metash UV-visible 9000 spectrophotometer with distilled water as a reference. The UV–visible absorption spectra of the Ag nanoparticles synthesised with various concentrations of C. macrostachyus leaf and bark extract solution are recorded and shown in ).
Figure 4. UV–Vis spectra of: (a) (1:3), b (1:1) and c (3:1) Ag-NPs synthesised by composite of extract solution, and (b) 1:1(L) and 1:1(b) was synthesised with separate leaf and bark solution [L: leaf; b: bark of Croton macrostachyus].
![Figure 4. UV–Vis spectra of: (a) (1:3), b (1:1) and c (3:1) Ag-NPs synthesised by composite of extract solution, and (b) 1:1(L) and 1:1(b) was synthesised with separate leaf and bark solution [L: leaf; b: bark of Croton macrostachyus].](/cms/asset/19024226-10a4-4a13-a11d-c1412b6a2bd6/ymte_a_2164647_f0004_oc.jpg)
The UV–Vis absorption spectra of bio synthesised Ag nanoparticles with various concentrations of composite solution indicates that the absorption spectra exhibit a gradual decrease in absorbance. The spectrum of the sample was seen to be in the range of 200– 800 nm. The UV–Vis absorption spectra in ) of 1:3 which accounts small size Ag-NPs show a highest absorption efficiency in the absorption band and the surface plasmon resonance, which occurs at 300 nm, gradually shifts to left or exhibits blue shifting to the shorter wavelength [Citation26]. This band corresponds to colloidal silver nanoparticle absorption in the shifting wavelength range due to surface plasmon vibration excitation [Citation27]. Lower absorption efficiency is afforded by 1:1 composition. Similarly, in ) of leaf and bark separately templated samples, highest absorption efficiency is encountered with bark templated of 1:1(b) due to super plasmon resonance effect encountered at 300 nm. Lower absorption efficiency is accounted in leaf extract of 1:1 composition. A high concentration of C. macrostachyus leaf and bark extract increases the number of bio-compounds required to reduce Ag+ to Ag0 [Citation28]. High concentration composite solution of leaf and bark extract of C. macrostachyus will increase reduction of silver ion into silver metal nanoparticle. In this case using composite extract solution will be more favourable for reduction than separate extract solution. This silver nanoparticle synthesised by C. macrostachyus leaf extract gives lower absorption band relatively with composite and bark extract.
The diffractogram (XRD data) of synthesised nano particle is shown in .
Table 2. XRD data of synthesised Ag-NPs.
The diffractogram results obtained from XRD data for each synthesised nanoparticles, i.e. 1:3,1:1,3:1, 1:1 (leaf extract ratio) and 1:1 (park extract) gives an information of 2θ value and half width at maximum (FWHM). From the given information, the average particle size of the prepared Ag-NPs was evaluated by using Debye-Scherer formula:
where D is the average size of the particles, λ is the wavelength of X-ray radiation used, β is the FWHM intensity of the peaks, and θ is the Bragg’s angle obtained from the 2θ value corresponding to the maximum intensity peak in XRD pattern. As a result, the calculated size of synthesised 1:3, 1:1, 3:1, 1:1 (leaf extract) and 1:1 (park extract) of Ag nanoparticles are 8.9, 10.7, 12.11, 10.87 and 13.68 nm. The diffractogram results are presented in . The crystalline peaks observed at 2θ values of 49.12, 44.34, 38.06, 33.12 and 29.9 with its corresponding (hkl) values of (311), (220), (200) and (111), respectively, were obtained for Ag nanoparticles synthesised in 1:3, 1:1 and 3:1 ratios [Citation28,Citation29].
As the graphs show, crystallinity and intensity of the material increase with increasing precursor concentration. When the amount (concentration) of plant extract increase, crystallinity nature of the material decrease. Uncalcined biosynthesised nanomaterials are holding the nature of host ingredients (organic compounds) and the highest content of carbon leads to amorphous structure of the material. In this case, the nanomaterial synthesised by high volume percentage of plant extract has more amorphous structure as compared with the low percentage plant extract. Otherwise the size of the nanoparticle synthesised with high volume percentage of plant extract is smaller than the low percentage of plant extract. This is because more amount of plant extract contains large content of organic capping (reducing) agent. Therefore, in ), 1:3 ratio-synthesised nanoparticle has smaller size than 3:1 ratio synthesised nanoparticle. The composite solution is more reducing agent as compared with each individual leaf and park extract broth solution. As shown in ), amorphousity nature of 1:3 ratio is higher than 1:1 ratio and 3:1 ratio. In contrary, the crystallinity nature of 3:1 ratio is higher than 1:1 ratio and 1:3 ratio. ) shows 1:1 ratio of leaf extract is more amorphous than 1:1 ratio with park extract. This indicates that more ingredients that are organic are found in leaf extract as compared with bark extract.
Infrared spectroscopy used to determine functional group of constituent materials. It is simply the measurement of the absorption of IR frequency by organic compounds placed in the path of the beam of light. The greatest value of infrared spectroscopy certainly lies in its contribution to the elucidation of molecular structure, particularly the recognition of functional groups and their environment. The infrared spectra of these materials vary according to their composition and can possibly show the occurrence of complex formation and interaction between different components. The mutual interaction causes a change in the vibrational modes of the atoms or molecules in the material, resulting in a change in the physical and chemical properties of the complex’s components. The FTIR spectra shown in depicts the biosynthesised Ag nanoparticles.
FTIR measurements were carried out to identify the possible biomolecules responsible for the capping and efficient stabilisation of the silver nanoparticles synthesised by the plant extracts. In ), the FT-IR spectrum of Ag-NPs obtained from 3:1 composition of silver nitrate with leaf and bark extracts of C. macrostachyus revealed four absorption frequencies with weak intensity around 3000, 1670, 1500, and 1000 cm−1. Each absorption frequency showed the presence of specific functional groups for typical organic compounds. weak and broad band around 3000 cm−1 indicates the presence of O–H group, the presence of weak peak around 1670 cm−1 indicated that the presence of carbonyl functional group, weak frequency around 1500 cm−1 assigned to C=C alkenes stretching, and weak absorption band around 1000 cm−1 indicates C–O ether stretching [Citation30]. The spectrum obtained from 3:1, 1:1, and 1:3 composition of silver nitrate with leaf and bark extracts of C. macrostachyus showed different intensity with similar frequency at different absorption band. Weak absorption spectrum of Ag-NPs obtained from 3:1 composition of the starting materials tells us the concentration of inorganic compound predominate over the organic compound since the absorbance have linear dependence on concentration stated by Beer–Lambert law, absorbance (A) is directly proportional to the concentration (c) and path length of sample (l):
where ‘ε’ is the molar extinction coefficient or molar absorptivity which is characteristic for a specific substance.
On the other hand, a strong absorption spectrum obtained from 1:1 and 1:3 compositions showed that the concentration of an organic compound predominates over that of an inorganic compound. The FTIR spectrum of silver nanoparticles obtained from a 1:1 composition of silver nitrate with leaf and bark extracts of C. macrostachyus as shown in ) showed four absorption frequency ranges around 3000, 1670, 1500 and 1000 cm−1 like the spectrum in ). The only difference between the spectrums of Ag-NPs obtained from the composition of silver nitrate with composite and the 1:1 ratio of silver nitrate with leaf and silver nitrate with bark is on the intensity of absorption at specific frequency. This tells us the contents of active ingredients in the plant part for the synthesis of Ag-NPs are predominantly found in the leaf of croton macros taches.
Antibacterial activity of green Ag-NPs
The antibacterial activity evaluation of C. macrostachyus leaf and bark extract templated synthesised Ag-NPs were performed by taking 100 µg/mL of Ag-NPs against the targeted Gram-positive (S. pyogenic) and Gram-negative (P. aeruginosa) bacteria strains via disk diffusion method using Ampicillin as a standard/positive control. Of the numerous percent, compositions of green Ag-NPs, the 1:1(L) showed enhanced (13 mm ZOI) antibacterial activity against S. pyogenic strain. This might be attributed due to the presence of a high amount of bioactive ingredients within the leaf extract of C. macrostachyus. Since as the number of bioactive molecules presents within the leaf extract is high, it results in the formation of enhanced reduced superoxide radical reactive oxygen species (ROS). This intern results in enhancing the penetration of bacterial cell walls and then death [Citation18]. Moreover, the improved antibacterial activity of the 1:1 (L) might also achieve due to its smaller average crystalline size (10.87 nm), which is simple to enter into the cell wall of the S. pyogen followed by preventing and penetrating the cell. Next to the 1:1 (L) percent composition, the 1:1 (P) percent composition showed effective (12 mm ZOI) antibacterial activity against the target bacteria species. This might be achieved due to the existence of antibacterial active biomolecules within the bark extract of C. macrostachyus. supported with depicts the antibacterial activity of the green-formed Ag-NPs, which were formed within five different percent compositions of precursor salt and bark with leaf of C. macrostachyus.
Table 3. The ZOI of Croton macrostachyus templated Ag-NPs.
As can be confirmed from the zone of inhibition (ZOI), all the synthesised green Ag-NPs showed a promised antibacterial activity against the target bacterial strains. Like the 1:1 (L) and 1:1 (P), the remaining 1:1, 1:3 and 3:1 compositions of Ag-NPs also proved enhanced antibacterial activity. The Ag-NPs (1:1) showed 11 and 9 mm ZOI against the growth of S. pyogen and P. aeruginosa, respectively, as shown in .
It has been found that Ag-NPs having a ratio of 1:1 are effective towards S. pyogen as compared with P. aeruginosa; this is because Gram-negative bacteria species have double cell membrane that prevents the entering process of the green synthesised Ag-NPs (1:1) as compared with the counterpart bacteria [Citation31]. The 3:1% composition of green Ag-NPs showed low antibacterial activity as compared with the counterpart. This might be due to the presence of low percent of extract as compared with precursor salt. This again confirms the production of small amount of ROS molecules that can enhance the antibacterial activity of 1:3 of Ag-NPs [Citation32]. The present findings proves that all the various percent composites of green Ag-NPs found to be too effect against the Gram-positive bacteria as compared with Gram-negative bacteria within the same concentration (100 µg/mL). This might be contributed due to the variation in the cell membrane of the bacteria strains. Moreover, as can be presented and confirmed from and , the percentage of extract and precursor salt altered, the antibacterial potential also found to be parlay changed [Citation33].
Conclusion
In the present study, we successfully synthesised Ag-NPs from Croton macrostachyus plant for anti-bacterial activity. The synthesised Ag-NPs exhibited a low size that accounts high surface area. Also, the C. macrostachyus biomolecules were responsible for the reducing and capping agent of AgNPs. Moreover, composite of those extracts provides small size nanoparticle as compared with separately used extract broth solutions. The biosynthesised Ag-NPs were found to have a pronounced antibacterial activity against S. pyogen and P. aeruginosa. The study revealed that the phytochemicals found in bark and leaf, extracts of C. macrostachyus play avital role in the formation of active Ag-NPs.
Acknowledgments
The authors would like to acknowledge chemistry department, Wolkite University, Ethiopia for financial support, Adama Science and Technology University, Ethiopia for allowing us to use XRD and SEM instruments.
Disclosure statement
The authors declare that they have no known competing financial interests or personal relationships that could influence the work reported in this paper.
References
- Chung IM, Rajakumar G, Gomathi T, et al. Nanotechnology for human food: advances and perspective, Front. Life Sci. 2017;10:63–10.
- Saji VS, Choe HC, Yeung KWK. Nanotechnology in biomedical applications: a review. Int. J. Nano Biomater. 2010;3(2):119–139.
- Siddique S, Chow JCL. Application of nanomaterials in biomedical imaging and cancer therapy. Nanomaterials. 2020;10(9):1–41.
- Cong Jiang C, Ke Cao Y, Yong Xiao G, et al. A review on the application of inorganic nanoparticles in chemical surface coatings on metallic substrates. RSC Adv. 2017;7(13):7531–7539.
- Banerjee P, Satapathy M, Mukhopahayay A, et al. Leaf extract mediated green synthesis of silver nanoparticles from widely available Indian plants: synthesis, characterization, antimicrobial property and toxicity analysis, Bioresour. Bioprocess. 2014;1:1–10.
- Premasudha P, Venkataramana M, Abirami M, et al. Biological synthesis and characterization of silver nanoparticles using Eclipta alba leaf extract and evaluation of its cytotoxic and antimicrobial potential, Bull. Mater Sci. 2015;38:965–973.
- Hoseinnejad M, Jafari SM, Katouzian I. Inorganic and metal nanoparticles and their antimicrobial activity in food packaging applications. Crit Rev Microbiol. 2018;44(2):161–181.
- Singh J, Dutta T, Kim KH, et al. Green” synthesis of metals and their oxide nanoparticles: applications for environmental remediation. J Nanobiotechnology. 2018;16(1):1–24.
- Arumugam A, Karthikeyan C, Haja Hameed AS, et al. Synthesis of cerium oxide nanoparticles using Gloriosa superba L. leaf extract and their structural, optical and antibacterial properties, Mater. Sci Eng C. 2015;49:408–415.
- Qamar SUR, Ahmad JN. Nanoparticles: mechanism of biosynthesis using plant extracts, bacteria, fungi, and their applications. J Mol Liq. 2021;334:116040.
- Wakjira K, Negash L. Germination responses of Croton macrostachyus (Euphorbiaceae) to various physico-chemical pretreatment conditions. South African J Bot. 2013;87:76–83.
- Guilger-Casagrande M, De lima R. Synthesis of silver nanoparticles mediated by fungi: a review. Front Bioeng Biotechnol. 2019;7:1–16.
- Khan T, Ullah N, Khan MA, et al. Plant-based gold nanoparticles; a comprehensive review of the decade-long research on synthesis, mechanistic aspects and diverse applications. Adv Colloid Interface Sci. 2019;272:102017.
- Aga WS, Fantaye SK, Jabasingh SA. Biodiesel production from Ethiopian ‘Besana’- Croton macrostachyus seed: characterization and optimization, Renew. Energy. 2020;157:574–584.
- Maroyi A. Pharmacological properties of Croton macrostachyus Hochst. Ex Delile: a comprehensive review, Evidence-Based Complement. Altern Med. 2017;2017. DOI:10.1155/2017/1694671
- Mahomoodally MF. Evidence-based complementary and alternative medicine traditional medicines in africa : an appraisal of ten potent african medicinal plants, evidence-based complement. Altern Med. 2013;2013:1–14.
- Muthukumaran U, Govindarajan M, Rajeswary M. Green synthesis of silver nanoparticles from Cassia roxburghii—a most potent power for mosquito control, Parasitol. Res. 2015;114:4385–4395.
- Bekele ET, Murthy HCA, Muniswamy D, et al. Solanum tuberosum leaf extract templated synthesis of Co3O4 nanoparticles for electrochemical sensor and antibacterial applications. Bioinorg Chem Appl. 2022 ;2022:1–15.
- Bindhu MR, Umadevi M, Esmail GA, et al. Green synthesis and characterization of silver nanoparticles from Moringa oleifera flower and assessment of antimicrobial and sensing properties. J Photochem Photobiol B Biol. 2020;205:111836.
- Nicholson LB. The immune system. Essays Biochem. 2016;60(3):275–301.
- Düpjan S, Dawkins MS. Animal welfare and resistance to disease: interaction of affective states and the immune system. Front Vet Sci. 2022;9:1–6.
- Pathak VM, Verma VK, Rawat BS, et al. Current status of pesticide effects on environment, human health and it’s eco-friendly management as bioremediation: a comprehensive review. Front Microbiol. 2022;13:1–29.
- Bruna T, Maldonado-Bravo F, Jara P, et al. Silver nanoparticles and their antibacterial applications. Int J Mol Sci. 2021;22(13):7202.
- Garibo D, Borbón-Nuñez HA, de León JND, et al. Green synthesis of silver nanoparticles using Lysiloma acapulcensis exhibit high-antimicrobial activity. Sci Rep. 2020;10(1):1–11.
- Liu X, Le Chen J, Yang WY, et al. Biosynthesis of silver nanoparticles with antimicrobial and anticancer properties using two novel yeasts. Sci Rep. 2021;11:1–12.
- Diaz-Egea C, Abargues R, Martínez-Pastor JP, et al. High spatial resolution mapping of individual and collective localized surface plasmon resonance modes of silver nanoparticle aggregates: correlation to optical measurements. Nanoscale Res Lett. 2015;10. DOI:10.1186/s11671-015-1024-y
- Wu C, Zhou X, Wei J. Localized surface plasmon resonance of silver nanotriangles synthesized by a versatile solution reaction. Nanoscale Res Lett. 2015;10(1):1–6.
- Mollick MMR, Bhowmick B, Maity D, et al. green synthesis of silver nanoparticles using paederia foetida l. leaf extract and assessment of their antimicrobial activities. Int. J. Green Nanotechnol. Biomed. 2012;4(3):230–239.
- Meng Y. A sustainable approach to fabricating ag nanoparticles/PVA hybrid nanofiber and its catalytic activity. Nanomaterials. 2015;5(2):1124–1135.
- Wan Mat Khalir WKA, Shameli K, Jazayeri SD, et al. Biosynthesized silver nanoparticles by aqueous stem extract of entada spiralis and screening of their biomedical activity. Front Chem. 2020;8:1–15.
- Parmar S, Kaur H, Singh J, et al. Recent advances in green synthesis of Ag NPs for extenuating antimicrobial resistance. Nanomaterials. 2022;12(7):1115.
- Gul A, Wahab A, Fozia F, et al. Protein kinase inhibition, antibacterial activity, and characterization of phytoextract-mediated silver nanoparticles using aqueous extracts of ifloga spicata. J Nanomater. 2022 ;2022:1–9.
- Murthy HCA, Desalegn T, Kassa M, et al. Synthesis of green copper nanoparticles using medicinal plant hagenia abyssinica (Brace) JF Gmel. Leaf Extract: antimicrobial properties. J Nanomater. 2020;2020. DOI:10.1155/2020/3924081