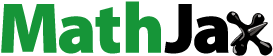
ABSTRACT
The increased use of medicinal plants has raised questions regarding their safety, efficacy, and utility. Since a result, a thorough understanding of plant phytochemical components is essential, since this information will be useful in the development of innovative therapeutic medications. Copper nanoparticles (CuNPs) have received a lot of interest due to their numerous applications. The physical and chemical features of CuNPs influence their uses. In the current study, CuNPs were synthesised utilising the chemical reduction process, with Tinospora cordifolia extract serving as a reducing agent due to their high phenolic and flavonoid content and their antibacterial and anti-inflammatory properties were also assessed. The majority of the absorption peaks found in the T. cordifolia leaves extract can also be found in the FT-IR spectrum of CuNPs, often at the same locations or with just little variations in the peak’s location and intensity. Scanning Electron Microscopy (SEM) and Transmission Electron Microscopy (TEM) found that the particles were generally spherical, with an average particle size of 15 to 70 nm. Their potential as anti-bacterial and antifungal drugs was demonstrated by the antimicrobial activity, they exhibited against S. aureus (18 mm), L. bacillus (22 mm), S. mutans (24 mm), and C. albicans (15 mm). Studies on the anti-inflammatory properties of biologically produced Cu NPs were determined.
Introduction
Today, the synthesis and application of nanoscience research are expected. Noble metal nanoparticles are rapidly being employed in antimicrobial medicine, microelectronics, biotechnology, optics, catalysis, information storage, and energy conversion due to their unique features. Nanotechnology has begun a new era in biotechnology by applying nanomaterials to accelerate applications and provide unique properties. Bioactive Nanoparticles are clinically relevant nanomaterials due to their low cost, high surface area-to-volume ratio, stability, and safety. Researchers have recently evaluated several methods to synthesise organic and inorganic NPs. Copper, silver, gold, and zinc are ideal integration candidates because of their characteristics and low toxicity.
Copper nanoparticles are used in cosmetology, textiles, biotechnology, etc. Due to their unique system, high surface area, excellent thermal and mechanical stability, and ideal optical, magnetic, and catalytic properties, copper nanoparticles are widely used [Citation1]. Green synthesis of copper nanoparticles extracts a capping agent from plant extracts with different properties to protect and provide therapeutic benefits [Citation2]. The synergistic action of bioactive chemicals from medicinal plants combined with Cu NPs has been shown to be effective against infections and also proved cytotoxic against cancer cells [Citation3,Citation4]. Microorganisms, enzymes, and plant extracts can be used to synthesize nanoparticles in an eco-friendly way. Plant extracts acts as reducing and capping agents for the synthesis of nanoparticle and can be climbed for large-scale synthesis [Citation5].
A significant medicinal plant, the deciduous climbing shrub Tinospora cordifolia is a member of the Menispermaceae family. It is a component of multiple remedies used in the traditional, age-old Ayurveda medicine of India to treat conditions like general sluggishness, dyspepsia, and urinary infections [Citation6,Citation7]. Alkaloids, phenolics, diterpenoid lactones, and their glycosides, sesquiterpenoids, aliphatic compounds, and polysaccharides are only a few of the many substances that have been identified from T. cordifolia [Citation8]. Parts of this plant species are widely used to treat a variability of human ailments and disorders. The herb functions as an adaptogen effective to relieve stress, disease, and boost human body resistance. Tinospora cordifolia leaf broth combined with Ocimum sanctum (Tulsi) is used as a treatment for dengue patients to boost their immunity and platelet count. Besides from treating allergies, high fever, gout, arthritis, and rheumatic illnesses of inflammation, this plant’s parts are also used to treat chronic skin conditions like psoriasis and dermatitis, lessen the negative effects of chemotherapy, and regulate blood sugar levels. For its anti-oxidant qualities and capacity to assist the liver and immune system, Tinospora species are frequently used as dietary supplements [Citation9]. Thus, our study was based on the synthesis of copper nanoparticles from Tinospora cordifolia and examined the reducing capacity of T. cordifolia leaf extract. The antibacterial and anti-inflammatory potential of copper nanoparticles was investigated.
Materials
Fresh Tinospora cordifolia leaves were collected from Pudhupet, Tamil Nadu, India and and authenticated by Prof. P. Jayaraman, Director, Plant Anatomy Research Centre, (Reg. no PARC/2020/4253). Tinospora cordifolia leaves were cleaned with distilled water to wash away the dust particles and left for 10 days to dry to remove the moisture.
2,2-diphenyl-1-picrylhydrazyl (95%, Alfa Aesar), iron(III) chloride (99%, Biochem Chemopharma), iron(II) sulphate (99%, Biochem Chemopharma), ascorbic acid (99%, Biochem Chemopharma), Copper sulphate (98%, Sigma-Aldrich), ethanol absolute (99%, Sigma-Aldrich), methanol (CH3OH, 96%, Sigma-Aldrich).
Preparation of Tinospora cordifolia aqueous extract
The dried Tinospora cordifolia leaves were crushed to fine powdered with the help of an electrical mixer. The dried T. cordifolia fractions (10 g) were heated in a flask containing 100 mL distilled water for 90 min at 70º C. It was then cooled to room temperature and then filtrated with a filter paper. The filtered extracts were stored at 4°C and used for further studies [Citation10].
Phytochemical analysis
Qualitative analysis
The qualitative phytochemical analysis of the aqueous extracts of T. Cordifolia leaves extracts were prepared by using standard method to spot the elements as described [Citation11, Citation12].
Test for alkaloids (Mayers test)
To a few mL of extract, a few drops of Mayer’s reagent was added by the side of tube. A creamy white precipitate indicates the presence of Alkaloids.
Test for carbohydrates (Benedict’s test)
To 0.5 mL of the extract, 0.5 mL Benedict’s reagent was added. The mix was heated on a boiling water bath for 2 minutes. A characteristic red colour precipitate indicates the presence of sugar.
Test for cardiac glycosides (Borntragers test)
To 2 mL of filtrate, 3 mL of chloroform was added and shaken, the chloroform layer separated and 10% ammonia solution was added to it. Pink colour indicates the presence of cardiac glycosides.
Test for flavonoid (Alkaline reagent test)
Two drops of AlCl3 solution were added to 1 mL of extract and addition of Concentrated H2SO4 added appearance of yellow colour indicates the presence of flavonoids.
Test for terpenoids (Salkowaski test)
To 5 mL of the extract was mixed with 2 mL of chloroform and concentrated sulphuric acid was added to form a layer. A reddish-brown colour indicates the presence of terpenoids.
Test for protein (Ninhydrin test)
To 1 mL of extract few drops of ninhydrin reagent added and heated in a boiling water bath a purple colour indicated the presence of protein.
Test for resins
2 mL of extract was heated for 30 minutes in a water bath. 1 mL of 1% FeCl3 was added to the mixture followed by the addition of 1% potassium ferricyanide. The mixture was filtered and formation of green (or) blue colour indicates the presence of resins.
Test for saponins (Lead acetate test)
To 1 mL of extract and 1 mL of lead acetate solution was added. The appearance of white precipitates presence of saponin.
Detection of steroids
2 mL of Chloroform was added to the few drops of extract and a few drops of acetic anhydride was poured. Followed by Conc. Sulphuric acid A mixture of blue and green colour showed the presence of steroid.
Test for tannins (Ferric chloride test)
To 2 mL of extract few drops of ferric chloride solution was added. The appearance of violet or greenish colour formed indicates the presence of tannin.
Determination of phenolic compounds
Using the Folin-Ciocalteau reagent using a spectrophotometric method, the quantity of phenolic compounds in the extract was measured [Citation13]. Gallic acid was used as the standard, with concentrations of 50, 100, 150, 200, and 250 mg/mL for the calibration curve. The Folin-Ciocalteau test (1: 10 dilutions of the reagent with distilled water) and 4 mL of 1 M aqueous sodium carbonate were used. were combined with 0.5 mL of diluted plant extracts. After 15 minutes, the mixtures were allowed to sit, and the total amount of phenolic compounds was measured colorimetrically at 765 nm. The values for total phenol are given as milligrams per gram of gallic acid.
Determination of flavonoids compounds
The total flavonoids of the leaf extract were determined by aluminium chloride colorimetric method [Citation14]. 0.5 mL of plant extracts were prepared in methanol (1:10 ratio) are mixed with 1.5 mL of methanol, 0.1 mL of 10% aluminium chloride, 0.1 mL of 1 M potassium acetate and 2.8 mL of distilled water are incubated at room temperature for 30 minutes. The absorbance of the reaction mixture was read spectrophotometrically at 415 nm.
Antioxidant activity
According to the approach described by George et al. [Citation15], the scavenging activity of the extract of leaves was measured by changes in the optical density of 2,2 diphenyl-1-picrylhydrazyl (DPPH) (Eq.1). The methanol used to dissolve the copper nanoparticles, which ranged in concentration from 0.1 to 0.5 mg/mL, was combined with 0.2 mL of 0.1 mM DPPH. With ascorbic acid as a reference, the degenerated colour of DPPH was measured at 517 nm after they had been thoroughly combined and incubated at 37°C for 30 minutes.
FRAP assay
By using the Benzie and Strain method [Citation16], the reducing antioxidant power (FRAP) of the leaf extract was determined. The FRAP reagent was freshly made in a concentration of 1:1:10 of 10 mM 2,4,6 tripyridyl-2 triazine (TPTZ) within 40 mM Hydrochloric acid, 20 mM ferric chloride, and 250 mM Sodium acetate buffer (pH 3.6). 1 mL of the FRAP reagent was combined with 1 mL of the copper nanoparticle solution. The combination was maintained at 37°C for 4 minutes after which the change in absorbance at 593 nm was measured.
Bio-synthesis of copper nanoparticles using Tinospora cordifolia extract
To 100 mL of a 1 M copper sulphate solution, 100 mL of newly produced Tinospora cordifolia extract was added. At 70°C, the mixture was continuously swirled for two hours. The synthesis of the copper nanoparticles was indicated by the solution’s hue changing from blue to dark green. It was then brought to ambient temperature and filtered via filter paper. Finally, drying at room temperature for 12 hours was applied to the synthesized nanoparticles [Citation10].
Characterization of the bio-synthesized copper nanoparticles
With a UV-3600 Plus UV-Vis double beam spectrometer (Shimadzu, Kyoto, Japan), the synthesis’s conclusion was evaluated. An ALPHA-E spectrometer with FTIR (Bruker, Billerica, MA, U.S.A.) was used to confirm the formation of copper nanoparticles and the contribution of biomolecules to this synthesis. An X-ray diffractometer (Malvern Panalytical) running at a voltage of 40 kV and an output current of 30 mA on Cu K radiation was used to characterize zinc oxide nanoparticles. The morphology and size of the samples were studied using a SEM (Thermo Scientific Apreo S) paired with energy-dispersive X-ray (EDX) spectrometry at a potential of 15 kV and a resolution of 1 nm. Transmission electron microscopy (TEM) was used to evaluate the morphology of the synthesised nanoparticles using a JEOL-TEM-100 plus transmission electron microscope (JEOL, Tokyo, Japan). For TEM analysis, the material was coated on the grid, dispersed in ethanol, and dried.
Antibacterial activity
Different harmful microbes were used to investigate the antibacterial efficacy of Cu NPs produced by biosynthesis. The gram-positive bacteria Lactobacillus Sp, Staphylococcus aureus, and Streptococcus mutans were employed to test the antibacterial efficacy of bio-reduced Cu NPs. A common Kirby-Bauer disc diffusion experiment was used to evaluate the antibacterial properties of biosynthesized Cu NPs [Citation17]. The test bacteria were cultured in nutrients in broth for 24 hours before being made available for further research. Plates made of nutrient agar were produced, sterilized, and set. After solidifying, bacterial lawns were created with a sterile glass rod to disseminate 100 l of each organism’s overnight culture onto the Petri plates. Cu NPs were loaded at the necessary volumes of 50 l, 100 l, and 150 l on the corresponding discs and maintained at 37°C for 24 hours on sterile discs with a diameter of 3 mm that were placed on these plates. Zones of inhibition were seen around the discs after the incubation period.
Anti-fungal activity
The Kirby-Bauer disc diffusion technique was used to assess the anti-fungal properties of bio-Cu NPs [Citation18] Candida albicans has been chosen as the test fungus. For additional research, the fungi pathogens were cultured in potato dextrose broth for 72 hours. Using a spreader and 200 l of the appropriate culture on potato dextrose agar (PDA) media, a lawn culture of the relevant organism was created using the pour plate method. The discs were then retained on PDA plates and filled with particular amounts of Cu NPs solution on each disc—50 l, 100 l, and 150 l – before the plates were allowed to incubate for 72 hours at room temperature. The zone of inhibition enclosing the discs served as evidence of the fungicidal activity that followed. All of these zones’ diameters were calculated, and the averages for every fungus pathogen were documented and expressed in millimetres.
Anti-inflammatory activity
The technique outlined by Elias and Rao [Citation19] and Padmanabhan and Jangle [Citation20] was followed, with a few minor adjustments, to assess the extracts’ anti-inflammatory activities. An amount of 1 mL of extracts (aqueous and ethanolic) or diclofenac sodium was homogenized with a volume of 1 mL of a water-based solution of bovine serum albumin (5%) and kept at 27°C for 15 minutes. The concentrations used were 200, 400, 600, 800, and 1000 g/mL. The control tube was made up of distilled water and BSA. By putting the mixture in a water bath at 70°C for 10 minutes, the proteins were denaturized. Each mixture’s activity was monitored at 660 nm as it cooled inside the confines of room temperature. Three times were given to each test.
The following formula was used to calculated inhibition percentage:
% inhibition= ((absorbance of control-absorbance of sample)/Absorbance of control)) *100
Results and discussion
Qualitative phytochemical screening
These tests were carried out using standard procedures of Harbone (1973) [Citation12]. The qualitative phytochemical screening of aqueous leaf extract of Tinospora cordifolia. The report was summarized in . The preliminary phytochemical screening showed that Tinospora cordifolia contains most of the phytochemicals. Tinospora cordifolia extract contains Alkaloids, Flavonoid, carbohydrates, Glycoside, Tannins, proteins, Phenol, as phytochemical constituents.
Table 1. Preliminary phytochemical constituent of Tinospora cordifolia leaf extracts.
Phytochemical analysis of the plant reveals the presence of several secondary metabolites, which are represented in . With the exception of terpenoids, T. cordifolia tested positive for all of the examined metabolites (reducing sugar, saponins, alkaloids, steroids, cardiac glycosides, and flavonoids). The early phytochemical analysis of T. cordifolia leaves and stems found a variety of bioactive secondary metabolites that may be the source of their therapeutic properties [Citation6,Citation21].
Total phenolic and flavonoid content
The Folin-Ciocalteau technique was used to determine the overall phenolic content of the T. cordifolia leaf extract, and the outcomes are presented as equivalent of gallic acid (). also includes the findings of the overall flavonoid amount found in T. cordifolia leaf extracts.
Table 2. Quantitative analysis of phytochemical constituents of T. cordifolia leaf extract.
Since their chemical reducing ability in relation to gallic acid is taken into consideration, the percentages of total phenolics obtained in this manner are not absolute assessments of the total phenolic compounds. The phenol antioxidant index has been found to be a combination indicator of the quantity and quality of antioxidants in vegetables. Reactive oxygen species are widespread and extensively dispersed in the plant kingdom, and phenolic compounds are the predominant phytochemicals that display scavenging efficacy on free radicals [Citation22].
Phenolic substances act as antioxidants via scavenging free radicals because they contain hydroxyl groups. Due to their redox properties, they can also function as reducing agents, metal chelators, hydrogen donors, and singlet oxygen quenchers [Citation23].
The majority of natural phenolic compounds are flavonoids, which have exceptional anti-free radical and antioxidant characteristics. The total amount of flavonoid was calculated utilising the aluminium chloride technique. Flavonoids’ capacity to scavenge radicals that are unstable and prevent their production is what gives them their anti-oxidant properties form the largest group of natural phenolic compounds and possess excellent free radical scavenging and antioxidant properties [Citation24]. Flavonoids are phenolic acids which assist as an significant basis of antioxidant, antimicrobial that were found in different medicinal plants and related phytomedicines [Citation25].
DPPH free radical scavenging activity
As a stable radical, DPPH is frequently employed to assess the capacity of natural chemicals to scavenge free radicals [Citation26]. A significant absorption at 517 nm, caused by the unpaired electron, gives the radical its purple colour. It reduces when exposed to antioxidants, decreasing absorbance as a result of the synthesis of yellow anti-radical diphenyl picryl hydrazine (DPPH-H). The amount of change in colour from purple to yellow is a measurement of the antioxidants’ capacity to scavenge free radicals by hydrogen-donating [Citation27–29]. Both the extract and the positive control (ascorbic acid) demonstrated a sizable, dose-dependent capability to scavenge free radicals in the current investigation (). The DPPH values are consistent with the phenol contents found for each sample (). Plant polyphenols function as reducing agents and antioxidants due to the hydrogen-donating characteristic of their hydroxyl groups [Citation30]. As a result, we may conclude that these polyphenols are responsible for the antioxidant activity shown in this study.
Table 3. Free radical-scavenging activity of T. cordifolia leaf extract at different concentrations using DPPH method.
Assay of reductive potential
Several mechanisms were proposed to explicate the antioxidant actions [Citation31], including reducing capacity, binding of transition metal ion catalysts, chain initiation prevention, peroxide decomposition, preventing further hydrogen abstraction, and radical scavenging. The extracts and BHT showed effective lowering capacity in the current study at all concentration points (). With an increase in concentration, both the extracts and BHT had greater reducing power (). It was discovered that BHT had a greater lowering capability than the extract at each concentration point. Antioxidant activity and lowering capability of specific plant extracts are directly correlated, according to earlier authors [Citation32]. It was discovered that the reductones found in these medicinal plants may be responsible for the extracts’ reducing properties, and the reaction may be dependent on inhibiting the free radical chain by providing a hydrogen atom, or it may be by reaction with certain peroxide compounds to avoid peroxide formation.
Table 4. Reducing capacity of T. cordifolia leaf extract at different concentrations.
Synthesis of copper nanoparticles
Cu NPs were produced by reducing copper ions in copper (II) sulphate pentahydrate solution using the aqueous extract. The parameters that affected the synthesis of Cu NPs included extract and copper (II) sulphate pentahydrate solution content, temperature, pH, and incubation time. At pH 12, temperature 70°C, volume ratio of 1.5, 0.01 M copper (II) sulphate, and incubation time of 2 hours, the greatest surface plasmon resonance (SPR) peak magnitude was found. The hue of the combination started to change when T. cordifolia extract was added to copper (II) sulphate pentahydrate solution, going from light blue to light green to dark brown. This colour shift may be related to the SPR of Cu Nanoparticles.
The following stages are expected to result in the creation of Cu NPs in this study by reducing copper ions by flavonoids or phenolic acids found in T. cordifolia extracts: Complexation with copper ions, concurrent reduction of copper ions to copper, and capping of the nanoparticles with oxidised polyphenol are the three steps. The FeCl3 test showed that the T. cordifolia extract in this study included phenolic chemicals, which were indicated by changes in colour that were blackish. Due to their redox characteristics, phenolic compounds can function as bio-reducing agents in the production of nanoparticles. The decrease of nanoparticles is caused by the -OH groups in flavonoids. It has been suggested that hydrogen ions are produced during the tautomeric conversion of flavonoids from their enol form to their keto form, which causes metal ions to be reduced and metal nanoparticles to be formed [Citation33].
Here, we hypothesised one potential mechanism and potential stepwise method for the creation of Cu NPs based on the experimental results. Copper hydroxide (Cu (OH)2) is first created when the metal precursor (CuSO4-5 H2O) combines with the hydroxyl anion (−OH) that is produced by water. Numerous phytochemicals, including amino acids, proteins, complex carbohydrates, alkaloids, saponins, phenols, oligosaccharides, and flavonoids, are present in the aqueous extract of T. cordifolia leaves. These substances act as reducing and encapsulating agents and reduce the copper hydroxide into copper nanoparticles [Citation34].
UV–visible spectroscopy analysis
UV-Vis spectra spectroscopy was used to confirm the Cu NPs’ stability and method of synthesis. The produced NPs’ absorption spectrum showed a typical peak for Cu NPs at about 350 nm (). The peak was still visible one week later, demonstrating the durability of Cu NPs. A clear sign of nanoparticle production is a progressive rise in the distinctive peak with a rise in reaction period as well as attention of biological extract with salt ions [Citation35].
FT-IR spectra analysis
The nature of the related functional groups and structural characteristics of biological samples with nanoparticles are identified using infrared intensity measurements vs. wavelength [wave number] of light. The estimated spectra amply demonstrate the well-known dependence of the resonance wavelength, extinction cross-section, and scattering/absorption ratio of nanoparticle optical characteristics on the nanoparticle sizes.
The potential biomolecules in charge of the capping of the Cu NPs and the decrease of copper (II) sulphate pentahydrate solution were identified using FT-IR analysis. Figuring 5. The absorption peaks for O-H alcohol and phenol, N-H stretching of amine, C-H stretch of aromatic compound, C = O stretch in carbonyl group, and -C -O stretching of ester, respectively, can be seen in the T. cordifolia extract at 3417, 2927, 1625, 1407, and 1078 cm-1. Stretching vibrations of C-O-C bonds are responsible for the strong signal at 1078 cm-1 [Citation36,Citation37]. The majority of the absorption peaks found in the T. cordifolia leaves extract can also be found in the FT-IR spectrum of CuNPs, often at the same locations or with just little variations in the peak’s location and intensity (for example, the points at 3470, 2925, 1741, 1462, and 1141 cm-1 in ). The results showed that the phenolic and terpenoid compounds, which can be distinguished by their peak of hydroxyl group at 3417 cm-1 in the T. cordifolia leaves extract, not only act as a reducing agent but also as capping ligands on the surface of the phyto synthesised Cu NPs [Citation38].
X-ray diffraction of copper nanoparticles
shows an XRD configuration of copper nanoparticles with peaks corresponding to the JCPDS card No. (36–1451) and no presence of secondary phase or impurity levels that confirmed the full decrease of the precursor. The planes (−111) (110) (−202) (020) (202) (−113) are confirmed by the two XRD values at 36.23°, 38.43°, 43.68°, 50.72°, 53.67°, 62.13° and 74.35°, respectively. Broad peaks indicate nanoparticle particle size, whereas sharp peaks reflect nanoparticle crystallinity [Citation35]. The mean size of the particles of copper nanoparticles was determined using the Scherrer equation D = kλ/βcosθ, and it was around 22.52 nm which is in agreement with the SEM particle size.
SEM-EDAX of copper nanoparticles
SEM was used to examine the morphological form and dimensions of the produced Cu nanoparticles. The SEM study of Cu NPs revealed that the spherical-shaped particles varied in size from 15 to 40 nm, which agrees with X-ray diffraction (XRD). 4 depicts a SEM picture of biosynthesized Cu NPs. EDX analysis verified the existence of copper (). The optical absorption peak of the produced NPs was around 1 keV, which is characteristic of Cu NP absorption. Carbon and oxygen indications are the most common impurities in Cu NPs. The C peak is owing to the grid used, while the oxygen peak is caused by the physical absorption of the oxygen from the environment during sample processing [Citation35].
Morphology and size analysis by transmission electron microscope
The size, shape, and size distributions of nanoparticles were identified by TEM investigation. T. cordifolia leaf extract was used to create Cu NPs, and their sizes ranged from 2–65 nm. Compared to the sonication method, the size distribution of Cu NPs produced by stirring appeared to be more uniform (). This outcome, which is most likely caused by the ultrasonic wave oscillating at a high frequency, could split agglomerated nanoparticles into distinct particles, changing the size distribution. This discovery was due to the fact that several biomolecules in T. cordifolia leaf extract are likely inactive in acidic environments, which can decrease the amount of chemicals that acts as reducing and capping agents for the creation of nanoparticles and change the sizes of the resulting Cu NPs [Citation39].
Antimicrobial activity
The Cu NPs that were synthesized from the leaf extract of T. cordifolia mediated Cu NPs showed a wide range of antibacterial activities against all tested pathogens: Lactobacillus sp, Staphylococcus aureus, Streptococcus mutans and anti-fungal activity against Candida albicans with a zone of inhibition showed in the and . The Cu NPs have a record of an outstanding antimicrobial activity [Citation40]. In light of this finding, it may be concluded that produced Cu NPs are more effective at combating gram-positive bacteria. The bioactive chemicals that act as capping and stabilizing agents on the outermost layer of NPs are responsible for their antibacterial activity.
Table 5. Zone of inhibition of different bacterial and fungal pathogens with respect to bio-Cu NPs volumes.
Due to the copper ions generated from Cu nanoparticles penetrating the membrane of bacterial cells and destroying the framework of the cell membrane by binding to the negative-charged cell wall, this superior antibacterial activity was achieved [Citation41]. By attaching to the DNA molecule of bacteria, copper ions play a role in the cross-linking of nucleic acid strands. This causes the DNA molecule’s helical structure to become disordered, which leads in denatured state of proteins and other metabolic reactions that eventually culminate in the total annihilation of bacterial cells [Citation42]. The size of the particles, the temperature at which they were created, the composition of the bacterial cell wall, and the amount of contact the nanoparticles have with the bacteria are all factors that determine how sensitive bacteria are to cupric oxide nanoparticles [Citation43].
Anti-inflammatory activity
The use of animals in experimental pharmaceutical studies has some drawbacks, including ethical concerns and the lack of a justification for their usage in cases where more acceptable alternatives are available. Because of this, a protein denaturation bioassay was used for the current study’s in vitro evaluation of the anti-inflammatory potential of copper nanoparticles created by T. cordifolia. According to the presence study, protein denaturation is the primary driver of inflammation. The potential of the extract to suppress protein denaturation was examined as part of the inquiry into the biological basis of the anti-inflammatory effect. A few extracts were efficient at preventing heat-induced denaturation of albumin. Cu NPs were shown to be mediate by T. cordifolia leaf extract as healed extraction was 56.35% and diclofenac 89.31%, respectively. As depicted in and , diclofenac was employed as a typical anti-inflammatory medication.
Table 6. Anti-inflammatory activity of bio-synthesized Cu NPs.
Results show that all evaluated quantities of synthesised silver nanoparticles were efficient in preventing thermally induced albumin denaturation, demonstrating their potential to modulate protein denaturation related to the inflammatory process. Inflammation is well-known to be caused by the denaturation of proteins. Denatured proteins no longer perform biological roles. Protein denaturation causes the formation of autoantigens in several arthritic diseases. Electrostatic hydrogen, hydrophobic, and disulphide bonding changes have a role in the mechanism of denaturation [Citation44].
Conclusion
A low-cost eco-friendly biosynthesis method for the production of Cu NPs using Tinospora cordifolia leaves extract was presented in this work. Understanding the processes that produce copper nanoparticles would enable much more precise control of nanoparticle morphology and production. The reducing mechanism of T. cordifolia leaf extract was evidenced by their antioxidant activity. Additionally, we have demonstrated that the copper nanoparticles created using this technique exhibit inhibiting selects on the growth of a S. aureus, L. bacillus, S. mutans and C. albicans indicating their potential as with respectively anti-bacterial and antifungal agents. We have the potential to transition to large-scale bio manufacturing by utilising bacteria’s innate capacity to make useful nanoparticles and enhancing it through engineering. This would allow us to manage nanoparticle synthesis to obtain the required morphologies, compositions, and activities. Anti-inflammatory activity of bio synthesised Cu NPs were studied, and free radical scavenging activity was observed.
Disclosure statement
No potential conflict of interest was reported by the author(s).
Data availability statement
The authors confirm that the data supporting the findings of this study are available within the article.
Additional information
Funding
References
- Sharma P, Pant S, Poonia P, et al. Green synthesis of colloidal copper nanoparticles capped with tinospora cordifolia and its application in catalytic degradation in textile dye: an ecologically sound approach. J Inorg Organomet Polym Mater. 2018;28(6):2463–13. doi: 10.1007/s10904-018-0933-5
- Sharma P, Pant S, Dave V, et al. Green synthesis and characterization of copper nanoparticles by Tinospora cardifolia to produce nature-friendly copper nano-coated fabric and their antimicrobial evaluation. J Microbiol Methods. 2019;160:107–116. doi: 10.1016/j.mimet.2019.03.007
- Ananda Murthy HC, Desalegn T, Kassa M, et al. Synthesis of Green copper nanoparticles using medicinal plant Hagenia abyssinica (Brace) JF. Gmel. Leaf extract: Antimicrobial properties. J Nanomater. 2020;2020. Article ID 3924081:12. doi: 10.1155/2020/3924081
- Gholami M, Azarbani F, Hadi F, et al. Eco-Friendly Synthesis of Copper Nanoparticles Using Mentha Pulegium Leaf Extract: Characterisation, Antibacterial and Cytotoxic Activities. Mater Technol. 2022;37:1523–1531. doi: 10.1080/10667857.2021.1959214
- Selvam K, Sudhakar C, Govarthanan M, et al. Eco-friendly biosynthesis and characterization of silver nanoparticles using Tinospora cordifolia (Thunb.) Miers and evaluate its antibacterial, antioxidant potential. J Radiat Res Appl Sci. 2017;10(1):6–12. doi: 10.1016/j.jrras.2016.02.005
- Singh K, Panghal M, Kadyan S, et al. Antibacterial activity of synthesized silver nanoparticles from Tinospora cordifolia against multi drug resistant strains of Pseudomonas aeruginosa isolated from burn patients. J Nanomed Nanotechnol. 2014;5(2): doi: 10.4172/2157-7439.1000192
- Singh SS, Pandey SC, Srivastava S, et al. Chemistry and Medicinal Properties of Tinospora Cordifolia (Guduchi). Indian Journal of Pharmacology. 2003;35:83–91.
- Jayaseelan C, Rahuman AA, Rajakumar G, et al. Synthesis of pediculocidal and larvicidal silver nanoparticles by leaf extract from heartleaf moonseed plant, Tinospora cordifolia Miers. Parasitol Res. 2011;109(1):185–194. doi: 10.1007/s00436-010-2242-y
- Gollapudi VR, Mallavarapu U, Seetha J, et al. In situ generation of silver and silver oxide nanoparticles on cotton fabrics using Tinospora cordifolia as bio reductant. SN Applied Sci. 2020;2(3):1–10. doi: 10.1007/s42452-020-2331-1
- Sebeia N, Jabli M, Ghith A, et al. Eco-friendly synthesis of Cynomorium coccineum extract for controlled production of copper nanoparticles for sorption of methylene blue dye. Arabian J Chem. 2020;13(2):4263–4274. doi: 10.1016/j.arabjc.2019.07.007
- Harborne AJ Phytochemical Methods A Guide to Modern Techniques of Plant Analysis. Springer Science & Business Media, 1998.
- Harborne JB. Phytochemical Methods. Netherlands, Dordrecht: Springer Netherlands; 1984. doi: 10.1007/978-94-009-5570-7
- Singleton VL, Orthofer R, Lamuela-Raventós RM. [14] analysis of total phenols and other oxidation substrates and antioxidants by means of folin-ciocalteu reagent. In: Methods in enzymology. Elsevier; 1999. pp. 152–178. doi: 10.1016/S0076-6879(99)99017-1.
- Dasgupta N, De B. Antioxidant activity of piper betle L. leaf extract in vitro. Food Chem. 2004;88(2):219–224. doi: 10.1016/j.foodchem.2004.01.036
- Scherer R, Godoy HT. Antioxidant activity index (AAI) by the 2,2-diphenyl-1-picrylhydrazyl method. Food Chem. 2009;112(3):654–658. doi: 10.1016/j.foodchem.2008.06.026
- Benzie IFF, Strain JJ. The Ferric Reducing Ability of Plasma (FRAP) as a Measure of “antioxidant power”: The FRAP assay. Anal Biochem. 1996;239(1):70–76. doi: 10.1006/abio.1996.0292
- Biemer JJ. Antim icrobial susceptibility testing by the K irby-B auer disc diffusion method. Ann Clin Lab Sci. 1971;3(2):135–140.
- Liu Y, He L, Mustapha A, et al. Antibacterial activities of zinc oxide nanoparticles against Escherichia coli O157: H7: antibacterial ZnO nanoparticles. J Appl Microbiol. 2009;107:1193–1201. doi: 10.1111/j.1365-2672.2009.04303.x
- EG RM, Rao MN. Inhibition of albumin denaturation and antiinflammatory activity of dehydrozingerone and its analogs. Indian J Exp Biol. 1988;26(7):540–542.
- Padmanabhan P, Jangle SN. EVALUATION of IN-VITRO ANTI-INFLAMMATORY ACTIVITY of HERBAL PREPARATION, a COMBINATION of FOUR MEDICINAL PLANTS. International Journal of Basic and Applied Medical Sciences. 2012;2(1):109–116.
- Rani J, Singh L, Singh H, et al. Dyke-Davidoff-Masson syndrome: imaging features with illustration of two cases. Quant Imaging Med Surg. 2015;5(3):469–471. doi: 10.3978/j.issn.2223-4292.2014.11.17
- Tungmunnithum D, Thongboonyou A, Pholboon A, et al. Flavonoids and other phenolic compounds from medicinal plants for pharmaceutical and medical aspects: an overview. Medicines. 2018;5(3):93. doi: 10.3390/medicines5030093
- Liang T, Yue W, Li Q. Comparison of the phenolic content and antioxidant activities of Apocynum venetum L. (Luo-Bu-Ma) and two of its alternative species. IJMS. 2010;11(11):4452–4464. doi: 10.3390/ijms11114452
- Sulaiman C, Balachandran I. Total phenolics and total flavonoids in selected Indian medicinal plants. Indian J Pharm Sci. 2012;74(3):258. doi: 10.4103/0250-474X.106069
- Xu D-P, Li Y, Meng X, et al. Natural antioxidants in Foods and medicinal plants: Extraction, Assessment and Resources. IJMS. 2017;18(1):96. doi: 10.3390/ijms18010096
- Kedare SB, Singh RP. Genesis and development of DPPH method of antioxidant assay. J Food Sci Technol. 2011;48(4):412–422. doi: 10.1007/s13197-011-0251-1
- Gangwar M, Gautam MK, Sharma AK, et al. Antioxidant capacity and radical scavenging effect of polyphenol rich Mallotus philippenensis fruit extract on human erythrocytes: an in vitro study. Sci World J. 2014;2014:1–12. doi: 10.1155/2014/279451
- MdM R, MdB I, Biswas M, et al. In vitro antioxidant and free radical scavenging activity of different parts of Tabebuia pallida growing in Bangladesh. BMC Res Notes. 2015;8(1):621. doi: 10.1186/s13104-015-1618-6
- Sharma SK, Singh AP. In vitro antioxidant and free radical scavenging activity of nardostachys jatamansi DC. J Acupunct Meridian Stud. 2012;5(3):112–118. doi: 10.1016/j.jams.2012.03.002
- Aberoumand A Nutritional Evaluation of Edible Portulaca Oleracia as Plant Food. Food Anal Methods. 2009;2:204–207. doi: 10.1007/s12161-008-9049-9
- Wang H, Gao XD, Zhou GC, et al. In vitro and in vivo antioxidant activity of aqueous extract from Choerospondias axillaris fruit. Food Chem. 2008;106(3):888–895. doi: 10.1016/j.foodchem.2007.05.068
- Govindan P, Muthukrishnan S. Evaluation of total phenolic content and free radical scavenging activity of Boerhavia erecta. J Of Acute Med. 2013;3(3):103–109. doi: 10.1016/j.jacme.2013.06.003
- Some S, Bulut O, Biswas K, et al. Effect of feed supplementation with biosynthesized silver nanoparticles using leaf extract of Morus indica L. V1 on Bombyx mori L. (Lepidoptera: Bombycidae). Sci Rep. 2019;9(1):14839. doi: 10.1038/s41598-019-50906-6
- Amaliyah S, Pangesti DP, Masruri M, et al. Green synthesis and characterization of copper nanoparticles using Piper retrofractum Vahl extract as bioreductor and capping agent. Heliyon. 2020;6(8):e04636. doi: 10.1016/j.heliyon.2020.e04636
- Shiravand S, Azarbani F. Phytosynthesis, characterization, antibacterial and cytotoxic effects of copper nanoparticles. Green Chem Lett Rev. 2017;10(4):241–249. doi: 10.1080/17518253.2017.1360401
- Rajathi K, Sridhar S, Author C Green synthesized silver nanoparticles from the medicinal plant Wrightia Tinctoria and its antimicrobial potential
- Rastogi L, Arunachalam J. Sunlight based irradiation strategy for rapid green synthesis of highly stable silver nanoparticles using aqueous garlic (Allium sativum) extract and their antibacterial potential. Materials Chemistry & Physics. 2011;129(1–2):558–563. doi: 10.1016/j.matchemphys.2011.04.068
- Shankar SS, Ahmad A, Sastry M. Geranium leaf assisted biosynthesis of silver nanoparticles. Biotechnol Prog. 2003;19(6):1627–1631. doi: 10.1021/bp034070w
- Li Y, Xie HQ, Wang JF, et al. Study on the preparation and properties of copper nanoparticles and their nanofluids. AMR. 2011;399–401:606–609. doi: 10.4028/www.scientific.net/AMR.399-401.606
- Din MI, Arshad F, Hussain Z, et al. Green Adeptness in the synthesis and Stabilization of copper nanoparticles: catalytic, antibacterial, Cytotoxicity, and antioxidant activities. Nanoscale Res Lett. 2017;12(1):638. doi: 10.1186/s11671-017-2399-8
- Sankar R, Manikandan P, Malarvizhi V, et al. Green synthesis of colloidal copper oxide nanoparticles using Carica papaya and its application in photocatalytic dye degradation. Spectrochim Acta A Mol Biomol Spectrosc. 2014;121:746–750. doi: 10.1016/j.saa.2013.12.020
- Yallappa S, Manjanna J, Sindhe MA, et al. Microwave assisted rapid synthesis and biological evaluation of stable copper nanoparticles using T. arjuna bark extract. Spectrochim Acta A Mol Biomol Spectrosc. 2013;110:108–115. doi: 10.1016/j.saa.2013.03.005
- Das SK, MdMr K, Guha AK, et al. Bio-inspired fabrication of silver nanoparticles on nanostructured silica: characterization and application as a highly efficient hydrogenation catalyst. Green Chem. 2013;15(9):2548. doi: 10.1039/c3gc40310f
- Matkowski A, Tasarz P. Szypuła E antioxidant activity of herb extracts from five medicinal plants from Lamiaceae, subfamily lamioideae. Journal of Medicinal Plants Research. 2008;2(11):321–330.