ABSTRACT
The present study reports the effect of niobium oxide (NbO) phase on the microstructure, corrosion, and bioactivity of coating on ZM21 Mg alloy by plasma electrolytic oxidation (PEO) process. NbO containing coatings are obtained by adding Potassium Niobate (KNbO3) along with the base electrolyte system, while the base electrolyte system consisted of sodium phosphate (Na3PO4⋅12 H2O) and potassium hydroxide (KOH). X-Ray Diffraction (XRD) was used for phase analysis, and Scanning Electron Microscopy (SEM) with Energy Dispersive X-ray Spectroscopy (EDS) was employed to extract the microstructural features. Tafel curves were generated to study the corrosion behaviour, and bioactivity test was conducted in the simulated body fluid (SBF) for 14 days to study the bioactivity. XRD results revealed that the PEO coatings consisted of MgO phase, whereas the sample coated in the electrolyte containing KNbO3 showed NbO phase in addition to MgO phase. NbO containing coating showed higher corrosion resistance and excellent bioactivity.
Introduction
Magnesium and its alloys find wide range of applications in aerospace, automotive and biomedical applications due to their excellent high strength to weight ratio. However, their formability characteristics are poor due to the smaller number of slip systems available for deformation which in turn limits their applications. This can be addressed by severe plastic deformation processes and recently, multi-axial forging has been developed and found to be successful in weakening of the texture and ultra fine grain size in the Mg-2Zn-2Gd alloy [Citation1]. In a similar study on Mg-2Zn-2Gd alloy, tri axial forging was found to have transformed the grain boundary precipitation to nano scale precipitation [Citation2]. In the field of orthopaedics, biodegradable implant materials are of paramount importance as they reduce the secondary surgery which in turn reduces the burden on the patient both monetarily and physically. Most of the biodegradable materials fall in the category of polymers but due to their lack of strength and stiffness they cannot be used for the hard tissue applications. Magnesium alloys are hence in the research focus in view of the biodegradability for orthopaedic applications. They are also proved to be biocompatible and possess many advantages like approximately equal modulus of elasticity as that of the bone, compatibility with roentgenology. However, due to the higher degradation rates limits their application. A novel Mg alloy containing Zn and Nd (Mg-2Zn-0.5Nd) showed non-cytotoxic behaviour in ion-extract method and higher bioactivity [Citation3]. Surface modification techniques like plasma electrolytic oxidation process are highly efficient in reducing corrosion rates [Citation4]. As Mg suffers high corrosion rates and owing to the need of improving the bioactivity, these alloys are majorly researched for improving corrosion resistance and bioactivity using PEO process. These coatings play a crucial role in improving the performance of magnesium alloys by incorporating elements like hydroxyapatite, polydopamine, pectin, and metal nanoparticles. These treatments aim to address challenges related to the rapid corrosion of magnesium alloys in biomedical implants while enhancing their corrosion resistance and bioactivity. The Mg coating on TiO2 layer developed by PEO process on Ti substrate and further heat treatment of the coating has shown to improve the wear and corrosion properties and makes it suitable for biomedical applications [Citation5]. In terms of corrosion resistance, the PEO with polydopamine (PDA) coatings on AZ31 outperformed the PEO coating. The coatings also demonstrated self-healing properties through a Zipper-like mechanism, providing durable corrosion resistance. Moreover, cytocompatibility tests with MG63 cells revealed improved cell adhesion, spreading, and viability on the PDA/PEO coated samples compared to untreated or PEO-coated ones [Citation6]. Coatings containing Ca8MgY(PO4)7 and Y2O3 particles improved wear properties, degradation resistance and cell viability [Citation7]. Some studies showed that PEO surface modification could potentially optimize the degradation kinetics and tissue response of bioabsorbable magnesium implants. They highlighted the benefits of PEO modification in reducing degradation rates, improving cytocompatibility, and enhancing bone regeneration around the implants. Overall, both bare Mg-Zn-Ca and PEO coated implants demonstrated satisfactory biocompatibility and performance, with PEO modification showing promising results for long-term implant use [Citation8]. The controlled release of Ag+ ions from the PEO-coated Mg-Ag alloy is highlighted as a promising feature for maintaining anti-infective efficacy without causing cytotoxicity to mammal cells. The findings of this study contribute to the understanding and development of biodegradable metallic implants with enhanced corrosion resistance and anti-infective properties [Citation9]. The addition of NaF is found to promote the formation of MgF2 nanophases in the passivation layer, reducing the breakdown voltage and discharge voltage and alleviating continuous arcing caused by high discharge voltage. This, in turn, enhances the Zn content in the PEO coating and decreases the pore size. The PEO coating demonstrates improved corrosion resistance, with the high-content ZnO-doped PEO-coated sample showing an increased corrosion potential, decreased corrosion current density, and increased charge transfer resistance compared to the bare AZ31B alloy. Additionally, the high-content ZnO-doped PEO-coated sample exhibits excellent corrosion resistance in salt solution, showing no obvious corrosion after more than 2000 h of neutral salt spraying and 28 days of immersion testing. The improved corrosion resistance is attributed to the relative uniform distribution of ZnO in the PEO coating, which can transform to Zn(OH)2 and form a continuous protective layer along the corrosion interface [Citation10]. The degradation rate of plasma electrolytic oxidised Mg-2.0Zn-0.6Ca-0.8Si was found to be the lowest and was found to be degradable in SBF solution, with the formation and dissolution of CaP apatite indicating excellent bioactivity [Citation11]. H. Du, D. Zhang, K. Xue et al. investigated the use of a multifunctional biodegradable curcumin (Cur)-ferrum (Fe) nanoflower coating on plasma electrolytic oxidation (PEO)-treated Mg alloy implants. The study focuses on the coating’s effects on promoting protein adsorption, cell adhesion and proliferation, corrosion resistance, antibacterial effect under simulated sunlight (SSL) irradiation, as well as its chemotherapy/photodynamic/photothermal synergetic antitumor properties and osteogenesis activity [Citation12]. M. Munoz et al. suggested that the PEO coating is effective in protecting the magnesium substrate and it can be used for protecting the implanted biodegradable stents until the remodelling of the inner vessel lumen occurs, thus offering potential for use in medical applications [Citation13]. The addition of pectin was found to fill the pores and micro-cracks within the PEO coatings, reducing porosity and roughness, and increasing the thickness of the coating. It was observed that the corrosion resistance of the coatings improved with higher concentrations of pectin, indicating that the use of pectin as an additive enhanced the corrosion resistance of the magnesium alloy, suggesting that the use of pectin has the potential to prolong the lifespan of medically implanted magnesium alloys in the human body [Citation14].
In the present study, we have developed Niobium oxide (NbO) containing coating on ZM21 Mg alloy by the addition of KNbO3 as an additive in the base electrolyte system with an aim to improve the corrosion resistance as well as the bioactivity property. It was shown that NbO containing PEO coating is favourable for increasing both corrosion resistance and bioactivity.
Materials and methods
Rectangular coupons measuring 20 mm × 20 mm and 3-mm-thick cut from a sheet of ZM21 Mg alloy was used as substrate material to perform PEO coating. The base electrolyte system was chosen to have sodium phosphate tribasic dodecahydrate (Na3PO4⋅12 H2O) and potassium hydroxide (KOH) in 1 L of distilled water. The samples were polished up to a grit size of 2000 and cleaned with ethanol followed by ultrasonication in the distilled water. A pulsed DC power (Milman Thin Film Systems Pvt. Ltd., Pune, India) was used, and the process was performed in a constant current mode at a current density of 7 A.dm−2. The frequency and the duty cycle were kept constant at 2 kHz and 70%, respectively. The PEO process was carried out using the electrolyte solution with and without the addition of potassium niobate (KNbO3) particles to the base electrolyte system in 1 L of distilled water. Since KNbO3 particles are partially dissolvable in distilled water, a colloidal suspension was made by adding 10 ml/L of ethylene glycol (C₂H₆O₂) for the suspension stability and 15 ml/L of triethanolamine (C6H15NO3) for bringing a partial negative charge on the particles, so that the particles can be attracted towards the ZM21 Mg alloy sample (anode) electrophoretically. The sample codes and their corresponding electrolyte composition are given in and henceforth the respective samples will be referred to by their sample codes. Phase analysis of the base alloy sample (B), plain PEO coated sample (P) and the coating produced using KNbO3 containing electrolyte (N) was carried out by scanning the samples using X-ray diffractometer with Cu Kα radiation at the scanning speed of 1°/min and a 0.05° step size. Scanning Electron Microscopy (SEM) with Energy Dispersive X-Ray Spectroscopy (EDS) was carried out to observe the morphology of the coating and the elemental presence in the coating, respectively. Electrochemical corrosion test was used to generate Tafel curves using a potentiostat (Gamry, Interface 1010T). Bioactivity studies were performed as per ISO23317 (in-vitro evaluation for apatite forming ability of implant materials) by immersing the samples in the Kokubo’s SBF solution, composition of which is given in [Citation15]. Samples were immersed in SBF for 14 days and for every 24 h the solution was changed, and fresh solution was added. After 14 days of immersion, the samples were subjected to SEM to observe the apatite formation on the surface of the samples.
Table 1. Sample codes and their corresponding electrolyte composition.
Table 2. Ion concentrations of SBF solution.
Results and discussion
Phase analysis
shows the XRD patterns of the base alloy sample (B), plain PEO coated sample (P) and the coating produced using KNbO3 containing electrolyte (N). In the sample P and N, a characteristic peak of MgO (JCPDS # 96-900-6753) can be observed at 2θ = 42.65°, reflection of (020) plane, followed by the reflections at 61.8° of (022) plane and 78.15° of (222) planes. In addition, a characteristic peak of NbO (JCPDS # 042–1125) can be observed in the sample N at the 2θ = 36.52°, reflection of (111) plane, followed by the reflections at 42.58° of (200) plane and 61.83° of (220) planes. It can also be noted that the peak between the 40° to 45° in the samples P and N has a slight shift with respect to each other, which could be attributed to the presence of MgO and NbO peaks at 2θ = 42.65° and 42.58°, respectively. It can also be attributed to the strains leading to the lattice distortion because of multiple phases present in the coating of sample N. A similar phenomenon can also be observed in the peaks between 60° and 65° for the samples P and N. It can also be understood that the reason for the presence of NbO phase is the addition of KNbO3 and the possible chemical reaction is shown equation 1.
Figure 1. (a) XRD patterns of NbO containing coating (sample N) in comparison with that of plain PEO coated sample (sample P) and base metal (sample B). (b, c) The water droplet images and contact angles of the sample P and sample B, respectively. (d1, d2) SEM micrograph and 3D optical profile of the sample P, respectively. (e1, e2) SEM micrograph and 3D optical profile of the sample N, respectively.
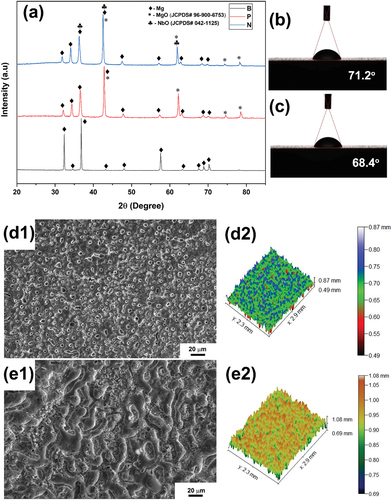
KNbO3 +3C→NbO +3CO+K2CO3(1)
In this reaction, carbon (C) acts as a reducing agent, and potassium carbonate (K2CO3) is formed as a byproduct. KNbO3 is the perovskite type of oxide that does not dissolve in the water. Hence, triethanolamine and ethylene glycol were added to the electrolyte solution for the charging of ceramic particles and increasing the suspension stability of the KNbO3 particles, respectively. The carbon present in these chemicals has acted as a reducing agent for KNbO3 to form NbO as shown in equation 1, and subsequently NbO has appeared in the coating along with MgO.
Contact angle of the PEO-coated samples
Contact angles of the PEO-coated samples were measured and presented in and the corresponding roughness parameters are listed in . It is evident that the contact angle of both the samples (P and N) have the lower contact angle of 71.2° and 68.4°, respectively, indicating that the PEO coating improves the hydrophilicity of the surface. Hydrophilic surfaces are more desirable in implants as they foster cell activity and improve adhesion and proliferation. It can be noted that the contact angle of the sample N is lower than that of sample P implying that the MgO/NbO composite coating is favourable for improving hydrophilicity.
Table 3. Amplitude parameters of roughness profile for samples P and N.
Microstructural and compositional analysis
The surface of the samples of Plain PEO coated and NbO containing coating are shown in , respectively. It can be observed that the sample P has higher porosity as compared to that of sample N indicating that the pore closure happened due to the formation of NbO phase. It can further be confirmed from the surface roughness measurement by 3D optical profilometer, results of which are presented in for the samples P and N, respectively. shows the EDS elemental mapping of plain PEO coating where the presece of Mg, O and P indicates that the phases present could be MgO and the Mg(PO4)2. The presence of the element Nb approximately 2.71 wt.% in the sample N, as shown in of EDS elemental mapping, also confirms the presence of NbO phase, conforming with the observations made in XRD. However, as compared to the MgO phase in the sample, NbO phase content is lesser. Further, elemental presence of phosphorus in both the samples of P and N indicates that the Magnesium Phosphate [Mg(PO4)2] phase would have formed but no peaks corresponding to it were observed in the XRD as it must be below the detection level of XRD. Little observation also shows that the wt.% of the element P has reduced from 15.46% to 7.24% (approximately 50% reduction) in the sample containing NbO phase indicating the suppression of the Mg(PO4)2 phase formation.
Electrochemical corrosion analysis
shows the Tafel curves obtained from the electrochemical corrosion test and the corresponding electrochemical parameters are tabulated in . It can be observed that NbO containing coating shows the highest corrosion resistance owing to its lowest porosity in the coating. It was already observed from SEM and EDS of the PEO coated samples that the morphology of NbO containing coating appeared as if the pores were closed and it was attributed to the pore closure by NbO phase particles. This type of coating will hardly allow the electrolyte to pass through the coating and expose the substrate, thereby increasing the corrosion resistance. However, both the PEO coated samples showed higher corrosion resistance than the substrate, while the polarisation resistance of the NbO containing coating has tremendously increased with the lowest icorr value of 0.451 × 10−6 A.cm−2 as seen from .
Table 4. Electrochemical parameters of samples B, P and N obtained from Tafel test.
Bioactivity studies of PEO-coated samples
shows the EDS elemental mapping of the PEO coated samples subjected to bioactivity test under SBF environment after an immersion period of 14 days and lists the elemental presence of the corrosion products in both wt.% and atomic %. Both the PEO coated samples have shown the formation of Ca and P on the surface of the samples indicating that the bioactivity of the PEO treated samples is good. However, in comparison with the plain PEO coated sample [ (P1 through P5)], the sample containing NbO phase [ (N1 through N6)] has shown a higher content of deposition of Ca indicating a higher amount of formation of hydroxyapatite in the sample N. The presence of higher amount of Nb in the sample N (19.2 wt.%) indicates the formation of NbO in the corrosion product. Hence, it can be concluded that the NbO phase could improve the corrosion resistance and bioactivity of the PEO coated sample.
Figure 5. EDS elemental mapping of samples P (P1 through P5) and N (N1 through N6) showing the apatite layer formation after 14 days of immersion in SBF solution.
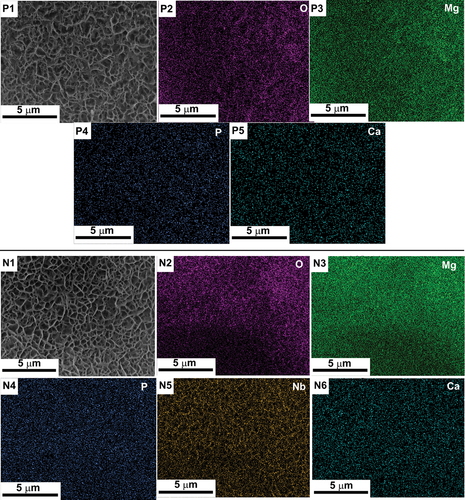
Table 5. Elemental presence in the apatite layer formed on sample P and N after the bioactivity test in SBF solution after 14 days.
Conclusion
In this study, NbO was formed in the PEO coating on ZM21 Mg alloy by using KNbO3 as an additive in the base electrolyte consisting of Na3PO4⋅12 H2O and KOH. It was understood that the KNbO3 was reduced by the carbon present in the ethylene glycol and triethanolamine resulting in the formation of NbO in the electrolyte in the first step. The formed NbO particles were electrophoretically attracted to the anode substrate during the PEO process and get embedded into the MgO phase of the coating, making it a composite coating. Furthermore, it was also noted that the NbO particles have favoured in closing of the pores, thereby increasing the corrosion resistance of the substrate. Bioactivity studies have shown that the formation of apatite layer is more in case of NbO containing coating as compared to the plain PEO coating. Hence, it can be concluded that niobium oxide could be a potential bioactive substance and can be considered for further research to explore its capabilities for biomedical applications.
Disclosure statement
No potential conflict of interest was reported by the author(s).
References
- Misra RDK. Understanding the weakening of texture in multiaxial forged ultrafine-grained strong and ductile Mg-2Zn-2Gd alloy. Mater Technol. 2024;39(1). doi: 10.1080/10667857.2024.2321411
- Weaver MR, Maldonado AJ, Banuelos JL, et al. On precipitation hardening behaviour in a triaxial forged Mg-2Zn-2Gd alloy and relationship to mechanical properties. Mater Technol. 2023;38(1). doi: 10.1080/10667857.2023.2215038
- Patil SS, Misra RDK, Gao M, et al. Bioactive coating on a new Mg-2Zn-0.5Nd alloy: modulation of degradation rate and cellular response. Mater Technol. 2019;34(7):394. doi: 10.1080/10667857.2019.1574956
- Sreekanth D, Rameshbabu N, Venkateswarlu K, et al. Effect of K2TiF6 and Na2B4O7 as electrolyte additives on pore morphology and corrosion properties of plasma electrolytic oxidation coatings on ZM21 magnesium alloy. Surf Coat Technol. 2013;222:31. doi: 10.1016/j.surfcoat.2013.01.056
- Muhammad I, Yu X, Wang Q, et al. Enhancing corrosion and wear resistance of Mg coating on titanium implants by micro-arc oxidation and heat treatment. Mater Technol. 2023;38(1). doi: 10.1080/10667857.2023.2176971
- Farshid S, Kharaziha M, Atapour M. A self-healing and bioactive coating based on duplex plasma electrolytic oxidation/polydopamine on AZ91 alloy for bone implants. J Magnesium Alloys. 2023;11(2):592. doi: 10.1016/j.jma.2022.05.020
- Xiu Chen J, Yang Y, Etim IP, et al. In vitro degradation, wear property and biocompatibility of nano-Y2O3-containing micro-arc oxidation coating on ZK60 alloy. Trans Nonferrous Met Soc China. 2023;33(5):1411. doi: 10.1016/S1003-6326(23)66192-8
- Bahrampour S, Bordbar-Khiabani A, Hossein Siadati M, et al. Improving the inflammatory-associated corrosion behavior of magnesium alloys by Mn3O4 incorporated plasma electrolytic oxidation coatings. Chem Eng J. 2024;483:149016. doi: 10.1016/j.cej.2024.149016
- Zhang X, Zhang Y, Lv Y, et al. PEO coating on Mg-ag alloy: the incorporation and release of Ag species. J Magnesium Alloys. 2023;11(6):2182. doi: 10.1016/j.jma.2021.12.006
- Yang C, Huang J, Cui S, et al. NaF assisted preparation and the improved corrosion resistance of high content ZnO doped plasma electrolytic oxidation coating on AZ31B alloy. J Magnesium Alloys. 2023. doi: 10.1016/j.jma.2023.02.008.
- Jia P, Pan Y, Yu L, et al. In vitro degradation and corrosion evaluations of plasma electrolytic oxidized Mg–Zn–Ca–Si alloys for biomedical applications. J Mater Res Technol. 2023;23:2410. doi: 10.1016/j.jmrt.2023.01.156
- Du H, Zhang D, Xue K, et al. Self-assembled biodegradable herbal-based nanoflower decorative magnesium implants combine therapy with bone regeneration. J Magnesium Alloys. 2024. doi: 10.1016/j.jma.2023.11.012
- Muñoz M, Fernández JP, Torres B, et al. Plasma electrolytic oxidation of AZ31 magnesium stents for degradation rate control. Surf Coat Technol. 2024;479:130539. doi: 10.1016/j.surfcoat.2024.130539
- Guo C, Li Y, Qi C, et al. Preparation and corrosion resistance characterization of MAO coating on AZ31B magnesium alloy formed in the mixed silicate and phosphate electrolytes with pectin as an additive. Surf Coat Technol. 2024;476:130209. doi: 10.1016/j.surfcoat.2023.130209
- Kokubo T, Takadama H. How useful is SBF in predicting in vivo bone bioactivity?. Biomaterials. 2006;27(15):2907. doi: 10.1016/j.biomaterials.2006.01.017