Abstract
NADPH oxidase 4 (NOX4) inhibition has been reported to mitigate diabetes-induced beta-cell dysfunction and improve survival in vitro, as well as counteract high-fat diet-induced glucose intolerance in mice. We investigated the antidiabetic effects of the selective NOX4 inhibitor GLX7013159 in vivo in athymic diabetic mice transplanted with human islets over a period of 4 weeks. The GLX7013159-treated mice achieved lower blood glucose and water consumption throughout the treatment period. Furthermore, GLX7013159 treatment resulted in improved insulin and c-peptide levels, better insulin secretion capacity, as well as in greatly reduced apoptotic rates of the insulin-positive human cells, measured as colocalization of insulin and cleaved caspase-3. We conclude that the antidiabetic effects of NOX4 inhibition by GLX7013159 are observed also during a prolonged study period in vivo and are likely to be due to an improved survival and function of the human beta-cells.
1. Introduction
Oxidative stress is proposed to play a pivotal role both in the development of diabetes and in many of the disease’s long-term complications [Citation1]. The NADPH-oxidase (NOX) enzymes serve as sources of reactive oxygen species (ROS), which contribute to various physiological processes [Citation2], but may also be a source for oxidative stress, with deleterious effects when excessively activated [Citation3]. Among the seven identified NOX isoforms (NOX1-5 and DUOX1-2), several have been studied for their potential role in diabetes [Citation4], with particular attention being given to NOX4 due to its potential involvement in renal and cardiovascular complications in diabetes [Citation5,Citation6].
NOX4 shares a common catalytic core with NOX1-3, but differs from other NOX isoforms in that it predominantly generates hydrogen peroxide (H2O2) rather than significant amounts of superoxide [Citation7]. NOX4 is expressed in most tissues [Citation8–11] and its activity has been observed to be regulated by altered gene expression [Citation12], hypoxia [Citation7], direct ATP binding [Citation13], and protein–protein interactions [Citation14,Citation15]. As diabetic conditions, such as elevated levels of cytokines, palmitate and glucose, may alter the activity of NOX4 in islet cells [Citation16–18], it is possible that NOX4 plays a harmful role in metabolic disease by impairing beta-cell function. Besides diabetes, NOX4 is also thought to participate in the pathogenesis of diseases such as cancer [Citation19], hypertension [Citation20], cardiac infarction [Citation21], Parkinson’s disease [Citation22], and kidney fibrosis [Citation23]. Interestingly, NOX4 has been reported to promote not only detrimental, but also beneficial effects [Citation24], indicating that NOX4-mediated effects are highly cell type- and situation-specific.
Excessive ROS production by NOX4 has the potential to disrupt redox balance within the beta cells, triggering oxidative stress-induced signaling cascades and ultimately contributing to beta-cell dysfunction and apoptosis [Citation4,Citation25,Citation26]. NOX4-mediated alterations in mitochondrial function and redox balance also have the potential to be important in the development of beta-cell dysfunction and death in type 2 diabetes [Citation27]. We previously demonstrated that selective NOX4 inhibition can protect human islets from cytokine as well as metabolic stress in vitro and that NOX4 inhibition counteracts high-fat diet-induced glucose intolerance in mice [Citation28,Citation29]. Furthermore, we recently demonstrated that NOX4 plays a role in beta-cell mitochondrial regulation, with inhibition of this enzyme improving the survival and function of human beta-cells in short-term in vitro-induced stress conditions [Citation30]. These findings are however yet to be assessed on human beta-cells in vivo and for a longer time period than hours or a couple of days. In the present study, we aimed to investigate the potential antidiabetic effects of NOX4 inhibition in an in vivo model of type 2 diabetes, using athymic diabetic mice transplanted with human islets and studied over a period of 4 weeks.
2. Materials and methods
2.1. Materials
The NOX4 inhibitor GLX7013159 was kindly provided by Glucox Biotech AB, Stockholm, Sweden. Compared to the previously characterized GLX7013114 [Citation22], GLX7013159 similarly inhibits NOX4 in the submicromolar range, with some cross-selectivity for NOX2, but has superior solubility in water and better metabolic stability. Alloxan was obtained from Sigma-Aldrich (Irvine, UK) and Carprofen from Norbrook Laboratories (Monaghan, Ireland). Isoflourane was obtained from Baxter (Lessines, Belgium). Glucose (300 mg/ml) and saline (9 mg/ml) solutions were purchased from Fresenius-Kabi (Uppsala, Sweden).
2.2. Animals
Male NMRI nu/nu mice, chosen to be used as their lack of thymus allows for xenotransplantation of human tissue without the need for immunosuppressants, were purchased from Taconic, Denmark. The mice were housed until 8–16 weeks-of-age before transplantation. All animal experimental procedures were approved by the local animal ethics committee, Uppsala, Sweden (ethical permission numbers C83/15 and 12150/2020).
2.3. Islet culture and transplantation
Human pancreatic islets were kindly provided by the Nordic Network for Clinical Islet Transplantation. Only donors who had agreed to donate for scientific purposes were included. The use of human islets in the studies was approved by the regional ethics committee, Uppsala, Sweden (ethical permission number 2017-283). Islets were cultured free-floating in Sterilin dishes in CMRL 1066 medium (Gibco 21530-027), containing 5.6 mmol/L glucose, 10% (vol/vol) fetal bovine serum, and 2 mmol/L L-glutamine. Human islet recipient mice were injected with alloxan (75 mg/kg) in the tail vein 2–3 days before being transplanted. Alloxan was freshly prepared immediately before the rapid intravenous injection. Blood glucose concentrations were monitored daily from blood withdrawn from the tip of the tail and analyzed using FreeStyle Lite (Abbott, Alameda, CA) test reagent strips. Mice with P-glucose >12.2 mmol/L on two consecutive days were considered to have diabetes. Diabetic mice were transplanted with 200 hand-picked and, between animals, size-matched islets to the left subcapsular kidney space. The islets were individually hand-picked from each donor to account for size variation and ensure equal proportions of islets of different shape and size between the different mice and donors. Islets from each donor (5 donors) were transplanted to multiple mice (2–4 mice), after transplantation the mice were randomized into the different treatment groups so that both control and experimental groups received islets from each donor and kept simultaneously. Donor characteristics can be found in the supporting information Table S1. Carprofen (5 mg/kg s.c.) was administered preoperatively and 24 h postoperatively for analgesia. Half of the mice were supplemented with 2 mg/kg/day GLX7013159 in their drinking water for 4 weeks (amount continuously adjusted according to water intake and body weight), whereas the remaining mice received drinking water with no additives. Mice were housed separately or together in groups of 2 per cage. Water consumption, food consumption, weight, health scoring, and blood glucose concentrations were monitored daily for the first 5 days and bi-weekly throughout the rest of the experiment. Blood for blood glucose measurements was sampled from the tip of the tail vein, while larger quantities of blood for other analysis were withdrawn from the saphenous vein. Mice reaching a score of 0.3 p or more on the Uppsala university extended assessment template (available in the supporting information) were terminated as a humane endpoint. After 26–28 days, the animals were subjected to an intravenous insulin tolerance test and intravenous glucose tolerance test. After 28–30 days, the animals were killed during isoflourane anesthesia, with both islet grafts and blood-samples being collected for further analysis.
2.4. Intravenous insulin and glucose tolerance test
1–2 days prior to termination, the transplanted mice were subjected to intravenous glucose and insulin tolerance tests (IVGTT and IVITT). An intravenous injection was administered to a tail vein, consisting of D-glucose (100 mg/ml) at a dose of 2 g/kg or insulin (Novorapid 100 U/ml; Novo Nordisk Bagsvaerd, Denmark) at a dose of 2 U/kg. For the IVGTT, blood samples were collected from all mice 10 min after glucose injection for insulin analysis. For both the IVGTT and IVITT, blood glucose was measured at 0, 10, 30, 60, and 120 min.
2.5. Perifusion analysis of human islet grafts
The graft-bearing kidneys were transferred to cold KRBH, and the islet grafts dissected free. Individual islet grafts were taken to glucose perifusion to investigate their glucose responsiveness. The grafts were inserted into filter-covered perifusion chambers (Suprafusion 1000, 6-channel system, Brandel, Gaithersburg, MD) and perifused (200 µl/min) with KRBH buffer. To obtain baseline secretion, the samples were perifused at 2 mmol/l glucose for 30 min. Perifusion was then performed with 20 mmol/l glucose for 26 min. Released insulin concentrations were analyzed by a Human Insulin ELISA kit (Mercodia).
2.6. Immunohistochemical analysis of human islet grafts
After perifusion, the grafts were fixated for 24 h in 4% (vol/vol) paraformaldehyde and thereafter frozen in Tissue Tech (Sakura Finetech, CA, USA) at −80 °C. The frozen grafts were cryosectioned (8 µm thickness) and stained with guinea pig anti-insulin (Fitzgerald, Acton, MA, Cat No. 20-IP30) and rabbit anti-cleaved caspase-3 (Cell Signaling Technology, MA, Cat No. #9661). Immunostaining was performed with overnight incubation of primary antibodies, followed by one-hour incubation of secondary antibodies, Alexa 594 conjugated donkey anti-guinea pig (Jackson ImmunoResearch Laboratories, West Grove, PA, Code No. 706-585-148) and Alexa 488 conjugated donkey anti-rabbit (Jackson ImmunoResearch Laboratories, West Grove, PA, Code No. 711-545-152). Incubation for another 5 min was carried out in DAPI solution. Images were acquired using a Zeiss LSM 780 (Zeiss, Jena, Germany) confocal.
Image analysis was performed in ZEISS ZEN lite, and ImageJ. Cells co-expressing insulin and caspase-3, as well as the total amount of insulin-expressing cells, were manually counted in nonconsecutive sections from each graft. Quota of caspase-3 expressing insulin-positive cells was calculated both by manual counting and signal quantification.
2.7. DCFH-DA fluorescence
EndoC-betaH1 cells were labeled with 10 µM Dichlorodihydrofluorescein Diacetate (DCFH-DA), a probe that is oxidized in response to ROS (including H2O2) production, for 45 min at room temperature. Cells were then trypsinized at room temperature and resuspended in Hank’s calcium-free buffer to prevent reaggregation of the cells, containing 22 mM glucose and increasing concentrations of GLX7013159. After 15 min preincubation at 37 °C, to allow uptake of the inhibitor, basal DCF-fluorescence was recorded by flow cytometry. The cells were then incubated for another 60 min at 37 C and analyzed again by flow cytometry. Changes in DCF fluorescence during the 60 min incubation period were expressed as ratio to control cells (0 µM GLX7013159).
2.8. Statistical analysis
Prism 9.0 for Macintosh, Graphpad Software, LLC, was used for all statistical computations. Results are presented as means ± SEM. Average blood glucose levels, water, and food consumption were calculated using AUC. Student’s unpaired t-test was used for comparisons between two groups. For multiple comparisons, repeated measurements one- and two-way ANOVA followed by Holm–Sidak post hoc test were used.
3. Results
3.1. Synthesis and characterization of GLX7013159
GLX7013159 is a further development of GLX7013114, which has been previously described [Citation28]. For methodology on the development and pharmacological methodology of GLX7013114 and GLX7013159, please refer to the previous paper. Selected hits from the high-throughput screen (HTS) were processed by medicinal chemistry (structure–activity relationship (SAR)) to modify the primary hits, and new efficient NOX4 inhibitors were identified. One of these, the highly selective and specific NOX4 inhibitor GLX7013114, was further SAR developed to improve solubility and metabolic stability. The SAR campaign resulted into the selection of GLX7013159 with similar inhibiting quality as GLLX7013114, but more water soluble and with some improved metabolic stability (Tables S2 and S3, Figures S1 and S2). It was observed however that NOX4 selectivity was slightly changed in this process to be a combination of NOX4/NOX2 selectivity in GLX7013159, while other isoforms still not affected, including XO (Figures S3, S4, S6) [Citation31,Citation32]. Of note is that GLX7013159 is still preferential for inhibiting NOX4 over NOX2 by a factor of five (IC50 0.3 µM NOX4, 1.5 µM NOX2). GLX7013159 did not display any redox activity, as assessed by the DPPH assay (Figure S5) [Citation33]. The results of the characterization of the novel NOX inhibitor GLX7013159 are given in . The different cell types used for IC50 analysis for Nox1-5 are described in Table S4.
Figure 1. GLX7013159 treatment decreases blood glucose levels and water consumption in athymic diabetic mice with human islet transplants.
(A) Average blood glucose values for GLX7013159-treated and control mice, following alloxan injection on day 0, and human islet transplantation on days 2 and 3. Values of HI (>27.8 mmol/L) were registered as 27.8 mmol/L. Mean AUC (post-transplantation) for control and GLX7013159-treated mice (GLX mice) were 752 ± 8 and 684 ± 21 mmol/L × 30 days, respectively (p = 0.01, n = 7–8 animals in each group). All values are given as means ± SEM. (B) Water consumption was lower throughout the treatment period for GLX7013159-treated mice, with AUC 1113 ± 83 mL for control and 888 ± 51 mL for the treatment group (p < 0.05). All values are given as means ± SEM.
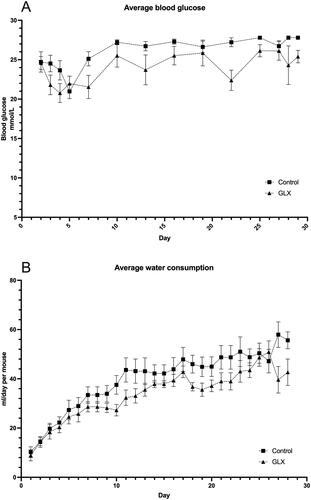
Figure 2. GLX7013159-treated mice display higher insulin levels 10 min post IVGTT and higher human C-peptide.
(A) An intravenous glucose tolerance test (2g glucose/kg BW) was conducted 1-2 days prior to end of the experiment. Blood samples were withdrawn 10 minutes after glucose injection. Mice receiving GLX7013159 (GLX) had higher serum insulin levels compared to control mice (p < 0.05 using Student’s unpaired t-test; n = 7–8 animals in each group). All values are given as means ± SEM. (B) Plasma collected at the termination of the experiment was analyzed using an ultrasensitive human C-peptide ELISA (Mercodia), GLX7013159-treated mice (GLX) had higher levels of human C-peptide compared to controls (p < 0.05 using Student’s unpaired t-test; n = 7–8 animals in each group). All values are given as means ± SEM.
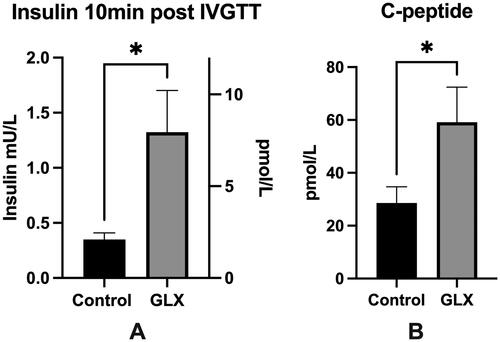
Figure 3. GLX70131159-treated mice grafts are more responsive to glucose during perifusion. Directly after collection, the transplanted islet grafts were subjected to a glucose perifusion analysis using KRBH containing 2 mmol/L and 20 mmol/L glucose. Buffer samples were collected at multiple time points and analyzed for human insulin using an ultrasensitive insulin ELISA (Mercodia). Stimulation indexes were calculated by dividing the AUCs of high glucose perifusion with low glucose perifusion for each islet graft. Grafts from GLX7013159 treated mice (GLX) displayed a higher stimulation index compared to control grafts (p < 0.05 using Student’s unpaired t-test; n = 7–8 grafts in each group). All values are given as means ± SEM.
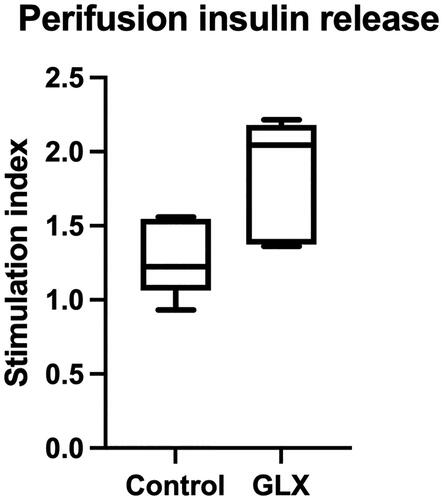
Table 1. Characteristics of the novel NOX inhibitor GLX7013159 compared to the previously described highly selective NOX4 inhibitor GLX7013114. NA: not active.
To verify that the NOX4 inhibitor GLX7013159 reduces H2O2 production in EndoC-betaH1 cells in vitro, we studied DCFH-DA oxidation during 60 min at a high glucose concentration (22 mM) and in response to increasing concentrations of the inhibitor. As DCFH-DA allows for superior detection of intracellular ROS in beta cells, it was chosen over Amplex Red. We observed that a low concentration of GLX7013159 (0.2 µM) did not significantly decrease ROS production (Figure S7). However, at 1 and 5 µM, the inhibitor reduced DCF fluorescence by 15 and 25%, respectively.
3.2. Effects of GLX7013159 on diabetic athymic mice
A total of 24 mice were included. Six mice were excluded prior to transplantation for failing to reach diabetic blood glucose levels (>12.2 mmol/L) after alloxan injection, whereas two mice perished in perioperative complications to transplantation. The remaining 16 mice were successfully transplanted (8 in treatment group and 8 in control group), with 14 mice completing the four-week trial. One mouse from each group was terminated during the experiment for reaching the humane endpoint. Treatment with GLX7013159 was well tolerated, with no observations of increased complications or adverse effects in the treated mice.
Blood glucose levels were during the 4-week period overall lower in the GLX7013159-treated group, as was water consumption (). Treatment with GLX7013159 affected neither food consumption nor the weight of the animals (results not shown). Intravenous glucose tolerance tests and insulin sensitivity tests were performed on the different study groups in the final treatment days (Figure S8). While these showed no differences between the groups, analyzing stimulated human insulin levels in blood gathered 10 min after glucose injection revealed higher levels of insulin in the GLX7013159 treated mice (). Human C-peptide levels were also assessed in the terminal blood samples of all mice, with the results again showing greater levels in the GLX7013159 treated mice ().
3.3. Perifusion analysis
We next studied the functional insulin secretion capacity of the isolated islet grafts using glucose perifusion. A constant flow of KRBH containing 2 mM or 20 mM glucose perifused the islet grafts, and buffer samples were collected at multiple time points and analyzed for human insulin using an ultrasensitive insulin ELISA (Mercodia). To account for different baseline insulin secretion, stimulation indexes were calculated by dividing the AUCs of the high glucose perifusion with the low glucose perifusion for each islet graft. Grafts from GLX7013159 treated mice were more responsive to changes in glucose levels, displaying a significantly higher stimulation index compared to control grafts ().
3.4. Effects of GLX7013159 on caspase-3 expression in transplanted human islets
There was no difference in the number of total insulin-positive cells between groups (). However, the human islet grafts of GLX7013159-treated mice had an almost threefold decrease in the frequency of cells with colocalized insulin and cleaved caspase-3 when compared to control (15.5% vs 44.7%, p < 0.001) (). Caspase-3 staining in insulin-positive cells was lower in GLX7013159-treated mice, while non-insulin-positive islet cells were unaffected by treatment ().
Figure 4. GLX7013159 treatment decreased cleaved caspase-3 (green) expression in insulin-positive cells (red). Top: The retrieved islet grafts were sectioned and stained for Insulin (RED), Cleaved caspase-3 (GREEN) and DAPI (BLUE). Top left shows a section from a control mouse, whereas top right shows a section from a GLX7013159-treated mouse. Bottom: Immunohistochemistry was assessed both by manually counting, by an investigator not aware of the identity of the sections, of the number of cells positive for insulin and/or cleaved caspase 3, and by measuring signal intensity using the ImageJ software. GLX7013159 treatment did not affect the total amount of insulin-positive cells (A, y-axis = number of cells per graft), but resulted in a marked decrease of cells co-expressing cleaved caspase 3 and insulin when manually counted (B, p < 0.001). The total cleaved caspase-3/DAPI signal intensity was lower in GLX7013159 treated mice (C, p < 0.05), GLX7013159 treated mice expressed lower cleaved caspase-3 signaling in insulin-positive areas (D, p = 0.01), no difference was observed in insulin negative areas (D). All images were analyzed blindly. All comparisons were performed by Student’s unpaired t test. Values are given as means ± SEM for 7–8 grafts in each group.
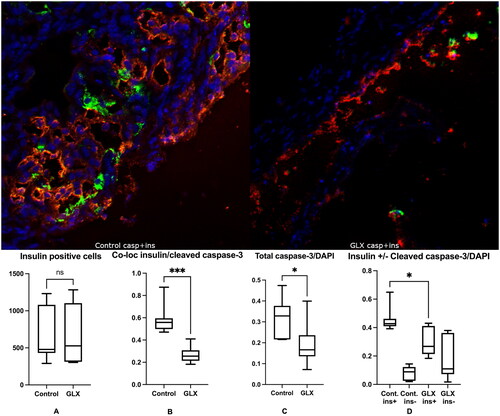
4. Discussion
We have previously shown that selective NOX4 inhibition counteracts human islet cell death after in vitro-induced stress, seemingly by modulating mitochondrial activity and altering cellular redox status through both increased mitochondrial ROS and decreased general ROS [Citation28,Citation30]. Here, we demonstrate that the human beta-cell protective effects of NOX4 inhibition persist over a longer time period in an in vivo setting. In the present study, a marginal mass of 200 islets, which is insufficient to restore normoglycemia [Citation34], was transplanted to each mouse in order to examine the effects of prolonged severe hyperglycemia. Thus, an improvement in blood glucose, but not a cure for diabetes, was an anticipated outcome. Our results indicate that NOX4 inhibition protects against hyperglycemia-induced beta-cell dysfunction, with NOX4 inhibition improving glycemic control and lowering water consumption throughout the 4-week treatment period. As alloxan-injected mice are almost completely devoid of endogenous beta cells [Citation35–37], the lowered glucose levels likely reflect an improved function of the transplanted human beta-cells. The greater insulin levels during the IVGTT, higher human C-peptide levels in the final plasma as well as the improved islet graft stimulation index in the GLX7013159-treated mice further support this. While remaining mouse beta-cell mass after alloxan injection was not assessed, there were no notable differences in blood-glucose levels between the mice before transplantation (, day 2), and after islet transplantation, the mice were randomized between the treatment groups without preference. We also did not observe any signs of auto-reversion reversion from the alloxan injections as all mice maintained high blood glucose levels throughout the experiment. So, any small remaining beta-cell mass, or beta-cell regeneration, should have been randomly distributed between the two groups. Nevertheless, this may pose as a potential limitation. Moreover, the potential effects of NOX4 inhibition on mouse and human beta-cell proliferation remain to be studied. Another limitation is that exact concentrations of the compound were not measured in the treated mice; this would also be of interest regarding assessment of the potential impact of cross-NOX selectivity.
The used glucose monitoring device (Freestyle Lite) has an upper measuring range of 27.8 mmol/L. This maximum threshold was frequently reached within the control group, in contrast to in the GLX7013159-treated mice. In the statistical analysis, values above measuring range (HI) were however conservatively set as 27.8 mmol/L. The difference in glycemic control was therefore probably even greater between the treated and nontreated mice than presently reported. Both our present results as well as our previous findings using GLX351322 indicate that NOX4 inhibition does not appear to affect insulin sensitivity [Citation30], making it more likely that the observed difference in blood glucose indeed stems from an improved islet function with increased insulin production. As all mice were highly diabetic in the current study, the glucose tolerance test produced blood glucose measurements of “HI” for most of the IVGTT in both treatment groups, likely explaining why no significant differences could be observed here.
Loss of beta-cell mass is believed to occur already before the onset of type 2 diabetes, with a substantial decrease present at the time of diabetes diagnosis [Citation38]. Caspase-3 is recognized as a main effector in the apoptotic cascade both in autoimmune-driven islet apoptosis in type 1 diabetes and in type 2 diabetes [Citation39]. In the present study, we found a substantial decrease in cleaved caspase-3 and insulin co-expression in the islet grafts of GLX7013159-treated mice, possibly suggesting that the anti-diabetic effects observed from NOX4 inhibition could also origin from an increased survival of beta-cells. However, it is not clear why the total beta-cell numbers were not improved in the NOX4-inhibited mice. First, retrieving grafts from under the kidney capsule is technically challenging, so we might have obtained partial grafts from some of the mice that confounded the quantification of the number of beta-cells. Second, it may be that NOX4 inhibition protects against beta-cell death but also stimulates beta-cell dedifferentiation, which combined could explain the rather low beta-cell number/caspase-3 staining. Third, it is also possible beta-cells with lowered caspase-3 staining might eventually die by necrosis or some other non-caspase-3-dependent mechanism. This third alternative implies that NOX4 improves glucose homeostasis via a better beta-cell function rather than lowering beta-cell death.
The role of ROS, and the NOX enzymes in particular, in beta-cell function and dysfunction and the development of diabetes is complex, and it is likely that a delicate redox balance between beneficial physiological processes and the detrimental effects of oxidative stress exists. Moderate levels of ROS are inevitable and necessary in normal beta-cell functioning and have been found to stimulate cell proliferation [Citation1]. Furthermore, NOX4 has recently been shown to have fundamental roles in glucose-stimulated insulin secretion [Citation40], as well as in skeletal muscle insulin sensitivity [Citation41]. Furthermore, we have recently observed that NOX4-produced mitochondrial ROS may function as a negative feedback system to reduce mitochondrial respiration and ATP production [Citation30]. Nevertheless, excessive NOX4 activation and oxidative stress can be detrimental to islet functioning and survival and a driving force toward both the development of type 2 diabetes [Citation30, Citation42], and diabetes complications such as diabetic nephropathy [Citation5, Citation43]. The present findings on GLX7013159 are in line with our previous results, where two-week treatment with the NOX4 inhibitor GLX351322 decreased blood glucose levels in high-fat-diet-fed mice [Citation29]. In that study, NOX4 inhibition also improved glucose tolerance with no significant effects on insulin sensitivity. While knock-out models have suggested both protective and harmful roles of NOX4 in different diseases [Citation44–47], the nontotal pharmacological inhibition used in the present study, in lieu of total gene deletion likely translates better into a potential future clinical setting. Moreover, the highest GLX7013159 plasma concentrations should have coincided with feeding, as mice consume most of their water immediately before and after food intake [Citation48].
This study is the first to show that pharmacological NOX4 inhibition has beneficial effects on human beta-cells in vivo using an athymic mouse model of type 2 diabetes. The anti-diabetic effect persisted over the four-week study period and were likely due to an improved function of the beta-cells.
Author contributions
Conceptualization, A.E., P.-O.C., and N.W.; methodology, A.E., P.W. and J.L.; original draft preparation, A.E.; writing—review and editing, A.E., P.W., J.L., P.-O.C., and N.W. All authors have read and agreed to the published version of the manuscript.
Supplemental Material
Download MS Word (2.5 MB)Disclosure statement
The funder Glucox Biotech AB provided support in the form of salaries for P.W. Glucox Biotech AB has supported this study by generating, characterizing, and providing NOX inhibitors. The funders had no role in study design, data collection and analysis, decision to publish, or preparation of the manuscript.
Additional information
Funding
References
- Elksnis A, Martinell M, Eriksson O, et al. Heterogeneity of metabolic defects in type 2 diabetes and its relation to reactive oxygen species and alterations in beta-cell mass. Front Physiol. 2019;10:107. doi: 10.3389/fphys.2019.00107.
- Chen K, Craige SE, Keaney JF. Jr. Downstream targets and intracellular compartmentalization in nox signaling. Antioxid Redox Signal. 2009;11(10):2467–2480. doi: 10.1089/ars.2009.2594.
- Veith C, Boots AW, Idris M, et al. Redox imbalance in idiopathic pulmonary fibrosis: a role for oxidant cross-talk between NADPH oxidase enzymes and mitochondria. Antioxid Redox Signal. 2019;31(14):1092–1115. doi: 10.1089/ars.2019.7742.
- Guichard C, Moreau R, Pessayre D, et al. NOX family NADPH oxidases in liver and in pancreatic islets: a role in the metabolic syndrome and diabetes? Biochem Soc Trans. 2008;36(Pt 5):920–929. doi: 10.1042/BST0360920.
- Sedeek M, Callera G, Montezano A, et al. Critical role of Nox4-based NADPH oxidase in glucose-induced oxidative stress in the kidney: implications in type 2 diabetic nephropathy. Am J Physiol Renal Physiol. 2010;299(6):F1348–F1358. doi: 10.1152/ajprenal.00028.2010.
- Chen F, Haigh S, Barman S, et al. From form to function: the role of Nox4 in the cardiovascular system. Front Physiol. 2012;3:412. doi: 10.3389/fphys.2012.00412.
- Nisimoto Y, Diebold BA, Cosentino-Gomes D, et al. Nox4: a hydrogen peroxide-generating oxygen sensor. Biochemistry. 2014;53(31):5111–5120.
- Clempus RE, Sorescu D, Dikalova AE, et al. Nox4 is required for maintenance of the differentiated vascular smooth muscle cell phenotype. ATVB. 2007;27(1):42–48. doi: 10.1161/01.ATV.0000251500.94478.18.
- Schröder K, Wandzioch K, Helmcke I, et al. Nox4 acts as a switch between differentiation and proliferation in preadipocytes. ATVB. 2009;29(2):239–245. doi: 10.1161/ATVBAHA.108.174219.
- Goettsch C, Babelova A, Trummer O, et al. NADPH oxidase 4 limits bone mass by promoting osteoclastogenesis. J Clin Invest. 2013;123(11):4731–4738. doi: 10.1172/JCI67603.
- Cheng G, Cao Z, Xu X, et al. Homologs of gp91phox: cloning and tissue expression of Nox3, Nox4, and Nox5. Gene. 2001;269(1-2):131–140. doi: 10.1016/S0378-1119(01)00449-8.
- Serrander L, Cartier L, Bedard K, et al. NOX4 activity is determined by mRNA levels and reveals a unique pattern of ROS generation. Biochem J. 2007;406(1):105–114. doi: 10.1042/BJ20061903.
- Shanmugasundaram K, Nayak BK, Friedrichs WE, et al. NOX4 functions as a mitochondrial energetic sensor coupling cancer metabolic reprogramming to drug resistance. Nat Commun. 2017;8(1):1–15. doi: 10.1038/s41467-017-01106-1.
- Guo S, Chen X. The human Nox4: gene, structure, physiological function and pathological significance. J Drug Target. 2015;23(10):888–896. doi: 10.3109/1061186X.2015.1036276.
- Bernard K, Logsdon NJ, Miguel V, et al. NADPH oxidase 4 (Nox4) suppresses mitochondrial biogenesis and bioenergetics in lung fibroblasts via a nuclear factor erythroid-derived 2-like 2 (Nrf2)-dependent pathway. J Biol Chem. 2017;292(7):3029–3038. doi: 10.1074/jbc.M116.752261.
- Gerber PA, Rutter GA. The role of oxidative stress and hypoxia in pancreatic beta-cell dysfunction in diabetes mellitus. Antioxid Redox Signal. 2017;26(10):501–518. doi: 10.1089/ars.2016.6755.
- Fred RG, Kappe C, Ameur A, et al. Role of the AMP kinase in cytokine-induced human EndoC-betaH1 cell death. Mol Cell Endocrinol. 2015;414:53–63. doi: 10.1016/j.mce.2015.07.015.
- Carlsson C, Borg LA, Welsh N. Sodium palmitate induces partial mitochondrial uncoupling and reactive oxygen species in rat pancreatic islets in vitro. Endocrinology. 1999;140(8):3422–3428. doi: 10.1210/endo.140.8.6908.
- Pecchillo Cimmino T, Ammendola R, Cattaneo F, et al. NOX dependent ROS generation and cell metabolism. Int J Mol Sci. 2023;24(3):2086. doi: 10.3390/ijms24032086.
- Knock GA. NADPH oxidase in the vasculature: expression, regulation and signalling pathways; role in normal cardiovascular physiology and its dysregulation in hypertension. Free Radic Biol Med. 2019;145:385–427. doi: 10.1016/j.freeradbiomed.2019.09.029.
- Matsushima S, Sadoshima J. Yin and yang of NADPH oxidases in myocardial Ischemia-Reperfusion. Antioxidants (Basel). 2022;11(6):1069. doi: 10.3390/antiox11061069.
- Boonpraman N, Yoon S, Kim CY, et al. NOX4 as a critical effector mediating neuroinflammatory cytokines, myeloperoxidase and osteopontin, specifically in astrocytes in the hippocampus in Parkinson’s disease. Redox Biol. 2023;62:102698. Jundoi: 10.1016/j.redox.2023.102698.
- Thannickal VJ, Jandeleit-Dahm K, Szyndralewiez C, et al. Pre-clinical evidence of a dual NADPH oxidase 1/4 inhibitor (setanaxib) in liver, kidney and lung fibrosis. J Cell Mol Med. 2023;27(4):471–481. doi: 10.1111/jcmm.17649.
- Brown OI, Bridge KI, Kearney MT. Nicotinamide adenine dinucleotide phosphate oxidases in glucose homeostasis and diabetes-related endothelial cell dysfunction. Cells. 2021;10(9):2315. doi: 10.3390/cells10092315.
- Syed I, Kyathanahalli CN, Jayaram B, et al. Increased phagocyte-like NADPH oxidase and ROS generation in type 2 diabetic ZDF rat and human islets: role of Rac1-JNK1/2 signaling pathway in mitochondrial dysregulation in the diabetic islet. Diabetes. 2011;60(11):2843–2852. doi: 10.2337/db11-0809.
- Eguchi N, Vaziri ND, Dafoe DC, et al. The role of oxidative stress in pancreatic β cell dysfunction in diabetes. IJMS. 2021;22(4):1509. doi: 10.3390/ijms22041509.
- Block K, Gorin Y, Abboud HE. Subcellular localization of Nox4 and regulation in diabetes. Proc Natl Acad Sci US A. 2009;106(34):14385–14390.
- Wang X, Elksnis A, Wikström P, et al. The novel NADPH oxidase 4 selective inhibitor GLX7013114 counteracts human islet cell death in vitro. PLoS One. 2018;13(9):e0204271. doi: 10.1371/journal.pone.0204271.
- Anvari E, Wikstrom P, Walum E, et al. The novel NADPH oxidase 4 inhibitor GLX351322 counteracts glucose intolerance in high-fat diet-treated C57BL/6 mice. Free Radic Res. 2015;49(11):1308–1318. doi: 10.3109/10715762.2015.1067697.
- Elksnis A, Cen J, Wikström P, et al. Pharmacological inhibition of NOX4 improves mitochondrial function and survival in human beta-cells. Biomedicines. 2021;9(12):1865. doi: 10.3390/biomedicines9121865.
- Jaquet V, Marcoux J, Forest E, et al. NADPH oxidase (NOX) isoforms are inhibited by celastrol with a dual mode of action. Br J Pharmacol. 2011;164(2b):507–520. doi: 10.1111/j.1476-5381.2011.01439.x.
- Hirano K, Chen WS, Chueng AL, et al. Discovery of GSK2795039, a novel small molecule NADPH oxidase 2 inhibitor. Antioxid Redox Signal. 2015;23(5):358–374. doi: 10.1089/ars.2014.6202.
- Xiong Q, Kadota S, Tani T, et al. Antioxidative effects of phenylethanoids from cistanche deserticola. Biol Pharm Bull. 1996;19(12):1580–1585. doi: 10.1248/bpb.19.1580.
- Lopez-Talavera JC, Garcia-Ocaña A, Sipula I, et al. Hepatocyte growth factor gene therapy for pancreatic islets in diabetes: reducing the minimal islet transplant mass required in a glucocorticoid-free rat model of allogeneic portal vein islet transplantation. Endocrinology. 2004;145(2):467–474. doi: 10.1210/en.2003-1070.
- Bunnag SC, Warner NE, Bunnag S. Effect of alloxan on the mouse pancreas during and after recovery from diabetes. Diabetes. 1967;16(2):83–89. doi: 10.2337/diab.16.2.83.
- Ighodaro OM, Adeosun AM, Akinloye OA. Alloxan-induced diabetes, a common model for evaluating the glycemic-control potential of therapeutic compounds and plants extracts in experimental studies. Medicina (Kaunas, Lithuania). 2017;53(6):365–374.
- King A, Lau J, Nordin A, et al. The effect of capsule composition in the reversal of hyperglycemia in diabetic mice transplanted with microencapsulated allogeneic islets. Diabetes Technol Therap. 2003;5(4):653–663. doi: 10.1089/152091503322250677.
- Marrif HI, Al-Sunousi SI. Pancreatic β cell mass death. Front Pharmacol. 2016;7:83. doi: 10.3389/fphar.2016.00083.
- Tomita T. Immunocytochemical localisation of caspase-3 in pancreatic islets from type 2 diabetic subjects. Pathology. 2010;42(5):432–437. doi: 10.3109/00313025.2010.493863.
- Plecitá-Hlavatá L, Jabůrek M, Holendová B, et al. Glucose-Stimulated insulin secretion fundamentally requires H 2 O 2 signaling by NADPH oxidase 4. Diabetes. 2020;69(7):1341–1354. doi: 10.2337/db19-1130.
- Xirouchaki CE, Jia Y, McGrath MJ, et al. Skeletal muscle NOX4 is required for adaptive responses that prevent insulin resistance. Sci Adv. 2021;7(51):eabl4988. doi: 10.1126/sciadv.abl4988.
- Nowotny K, Jung T, Hohn A, et al. Advanced glycation end products and oxidative stress in type 2 diabetes mellitus. Biomolecules. 2015;5(1):194–222. doi: 10.3390/biom5010194.
- Yang Q, Wu FR, Wang JN, et al. Nox4 in renal diseases: an update. Free Radical Biology & Medicine. 2018;124:466–472.
- Schröder K, Zhang M, Benkhoff S, et al. Nox4 is a protective reactive oxygen species generating vascular NADPH oxidase. Circ Res. 2012;110(9):1217–1225. doi: 10.1161/CIRCRESAHA.112.267054.
- Zhang M, Brewer AC, Schröder K, et al. NADPH oxidase-4 mediates protection against chronic load-induced stress in mouse hearts by enhancing angiogenesis. Proc Natl Acad Sci USA. 2010;107(42):18121–18126.
- Kuroda J, Ago T, Matsushima S, et al. NADPH oxidase 4 (Nox4) is a major source of oxidative stress in the failing heart. Proc Natl Acad Sci USA. 2010;107(35):15565–15570.
- Radermacher KA, Wingler K, Langhauser F, et al. Neuroprotection after stroke by targeting NOX4 as a source of oxidative stress. Antioxid Redox Signal. 2013;18(12):1418–1427. doi: 10.1089/ars.2012.4797.
- Bachmanov AA, Reed DR, Beauchamp GK, et al. Food intake, water intake, and drinking spout side preference of 28 mouse strains. Behav Genet. 2002;32(6):435–443.