Abstract
Models for drug release from bioerodible polymer matrices are proposed in this article. We consider that drug is released continually by diffusion that is influenced by polymer chain degradation, and polymer matrix erosion starts and enhances the drug release at a certain time. The models give excellent reproduction of drug release profiles within the whole release period, and the parameters can be correlated to various factors such as γ-irradiation dose, copolymer composition, and initial drug loading, this correlation indicates that the new models can be used to predict the effects of various factors on drug release profiles based on limited experimental data.
Keywords :
Much more attentions were focused on drug release from bioerodible polymer matrices in the past 20 years. Many experimental investigations on the effects of various factors, such as γ-irradiation dose, copolymer composition, preparation methods, and matrix size, on the release mechanisms were carried out (Yoshioka, Otsuka, and Kojima Citation1995; Mallardé et al. Citation2003; Kim and Park Citation2004; Siepmann et al. Citation2004). In the meantime, mathematical modeling works also were performed by many researchers to reveal the mechanisms involved. For example, Lee (Citation1980) presented an approximate analytical model for drug release from thin surface eroding films by adding a moving erosion front with constant rate to the dissolution front. Charlier, Leclerc, and Couarraze (Citation2000) and Faisant et al. (Citation2002)(Citation2003) modeled the effect of polymer degradation on diffusion by relating the diffusion coefficient to the time-changing polymer molecular weight. Lemaire, Bélair, and Hildgen (Citation2003) proposed a model to describe the release of the drug immersed in a biodegradable, porous polymeric matrix, in which the pores were represented as hollow cylinders with constant cross-sectional area.
Because the underlying mechanisms of drug release from biodegradable polymer matrices are very complex and depend on various factors (Crank Citation1975; Fan and Singh Citation1989; Langer Citation1980; Langer and Wise Citation1984), these models can only take into account limited factors and thus have limited applications. In addition, they only predict a biphasic drug release kinetics of short initial burst effects subsequently followed by an approximately zero-order drug release phase. They do not reflect a second rapid drug release phase caused by the breakdown of the polymer matrix.
Recently, Siepmann, Faisant and Benoit (Citation2002) developed a triphasic model that considers all phases of drug release from bioerodible microparticles. The model is theoretical sound and has good accuracy; however, its complexity (has to be solved numerically) makes it not very easy for practical use. Therefore, it is necessary to develop an easily applied simple model capable of describing triphasic drug release kinetics from bioerodible polymer matrices, which is the motivation of our work.
THEORY
To avoid misunderstanding, we take the definition of Siepmann and Göpferich (Citation2001) for the terms “degradation” and “erosion,” that is, polymer degradation is the chain scission process by which polymer chains are cleaved into oligomers and monomers; erosion, in contrast, is defined as the process of material loss from the polymer bulk.
We describe the whole drug release process from a bioerodible polymer matrix as follows. In the whole time period, the drug is continually released by diffusion, which is accompanied by a process of polymer chain scissions. As the polymer chain cleavage continues, the polymer matrix gradually loses mechanical strength, and becomes weaker. At a certain time matrix erosion starts or becomes more obvious and enhances the drug release. Thus, the drug release kinetics can be described by a combined contribution from drug diffusion and matrix erosion.
For the drug release through diffusion, similar to Wada, Hyon, and Ikada (Citation1995), we assume that drug molecules are released from the degradable matrix in the principle of Fickian diffusion, which is influenced by polymer chain scissions (degradation) through the increased diffusion coefficient of drug. Assuming the drug is molecularly dispersed in the polymer matrix, drug release by Fickian diffusion can be described by the approximate equations of Baker and Lonsdale (Citation1974) and Ritger and Peppas (Citation1987) for spheric and disk matrix geometries as:
where md is the fraction of drug released, D is the drug diffusion coefficient, r is the radius of the sphere or disk, l is the height of the disk, and t is time.
The influence of polymer chain scissions (degradation) on drug release kinetics can be taken into account by a simple t function diffusion coefficient. Considering that the drug diffusion coefficient D depends on the polymer molecular weight M and varies in inverse ratio to it, and the change of polymer molecular weight M can be described by a first-order autocatalyzed polymer chain cleavage kinetics (Pitt and Gu Citation1987), the variation of D with time can be expressed by (Charlier et al. Citation2000):
where D0 is the initial drug diffusion coefficient, and ks is the polymer chain scission rate constant.
Replacing D in Equations [1] and [2] by Dt defined by Equation [3] leads to the equations for drug release from biodegradable polymer matrices through diffusion as follows:
where Dt is defined by Equation [3].
Furthermore, the drug release from pure matrix erosion can be described by the equation proposed by Fitzgerald and Corrigan (Citation1993), which describes the matrix erosion as a combined process of the branching and termination of polymer decomposition caused by the formation of the activated nuclei sites in the matrix:
where me is the fraction of drug released, ke is the acceleratory coefficient describing the probability of branching from activated sites during the time interval between oligomer generation and dissolution, and Tmax is the time to maximum matrix erosion rate.
Assuming that complete matrix erosion is achieved at the end of drug release, and combining Equations [4, 5, 6], the total fraction of drug released at time t, Mt/M∞, can be written as:
Here, FE is a factor counting the contribution of matrix erosion to drug release. It indicates that a fraction of drug originally released through diffusion is now released through matrix erosion, leading to the appearance of a second rapid drug release phase. Therefore, it can be anticipated that a slow drug diffusion rate or an early matrix erosion will cause a large FE value. The other parameters are all related to the characteristics of the release systems concerned and have definite physical meanings. The parameter D0 is related to the characteristics of the drug as well as the polymer matrix; the chain scission rate constant, ks, is directly related to the reactivity of the bond within the polymer backbone (Göpferich Citation1996); the acceleratory coefficient ke is a measure of relative ease of polymer decomposition branching within the polymer matrix; and the parameter Tmax is a description of the matrix half erosion time that is related to the polymer chain scission rate.
The new models (Equations [7] and [8]) describe a triphasic a drug release kinetics from bioerodible polymeric matrices that can capture most characteristics of drug release processes, including (1) an initial “burst” phase caused by the high initial drug release rate due to the short diffusion pathways; (2) the intermediate phase, an approximately zero-order drug release phase resulted from drug diffusion and polymer degradation; and (3) the second rapid drug release phase caused by the matrix erosion once the system becomes more weaken upon degradation.
Finally, the new models were developed based on the following four assumptions.
The kinetics of drug release from bioerodible polymer matrices follow Fickian diffusion influenced by polymer degradation and at a certain time accelerated by polymer erosion.
The drug is molecularly dispersed in the polymer matrix.
Polymer chain scission can be described by a first-order autocatalyzed hydrolysis kinetics.
Full matrix erosion is achieved during the drug release period.
RESULTS AND DISCUSSION
To evaluate the correlative accuracy and the predictive capability of the new model as well as the physical meaningfulness of the parameters involved, experimental release profiles showing triphasic drug release kinetics characterized by a second acceleration of drug release after a certain time were collected from the literature (Yoshioka and Kojima Citation1995; Wada et al., Citation1995; Ahola et al. Citation1999; Rich et al. Citation2001). The characteristics of the systems are shown in , which can be divided into two groups in general; namely, microspheres and disks. Since various affecting factors are considered in these measurements, they can be used to test not only the accuracy of the new models, but also the physical meaningfulness of the parameters involved, which also can be used to test the predictive capability of the new models.
TABLE 1 Systems used to test the new model
Drug Release from Microspheres
As a first step, drug release profiles from microspheres were collected from the literature (Wada et al. Citation1995; Yoshioka and Kojima Citation1995). They were used to evaluate the capability of the new model for spherical polymer matrices.
Aclarubicin Hydrochloride Release from DL-Lactic Acid Oligomer Microspheres
Equation [7] was used to fit the release profiles of aclarubicin hydrochloride (ACR) from DL-lactic acid oligomer microspheres with different molecular weights (Wada et al. Citation1995, data sets 1–3). The calculated results are depicted in and the parameters obtained are shown in . From it is obvious that the new model gives excellent representation of the experimental results, no matter for the release profile with a second rapid drug release phase (Mw = 3300), or without a second rapid drug release phase (Mw = 9600), or the intermediate (Mw = 6400). This illustrates that the new model is applicable to various release processes, and thus it is very useful.
FIG. 1. Comparison of the experimental aclarubicin hydrochloride release profiles (symbols) from dl-lactic acid oligomer microspheres with the theoretical profiles of this work (lines).
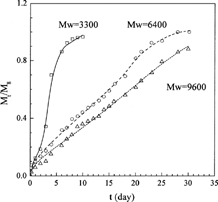
TABLE 2 Parameters obtained by fitting the drug release profiles
Since the experimental data are systematic data measured under different molecular weights, they can be used to analyze the physical meaningfulness of the parameters obtained. From , the values of D0 increase with decreasing initial molecular weight of DL-lactic acid oligomers, which is consistent with the previous reports (Wada et al. Citation1988, Citation1991, Citation1995) and can be explained theoretically. With decreasing chain length, the degree of macromolecules entanglement decreases, macromolecules exhibits better mobility, and thus large amount of free volumes are available for drug diffusion (Fan and Singh Citation1989), leading to larger D0. The polymer chain scission rate, ks, keeps constant for the two matrices of Mw = 6400 and 9600, while a smaller ks value was obtained for matrix of Mw = 3300. This may be due to the fact that the acceleration of drug release caused by matrix erosion occurs in the latter, while no matrix erosion occurs apparently in the first two matrices. The parameter FE reflects the contribution of matrix erosion to the total drug release. Thus, it should be zero if no matrix erosion occurs, and it should increase with decreasing molecular weight for the same kind of polymer since matrix erosion happens earlier with decreasing molecular weight. The values shown in are consistent with this analysis.
Since a decrease in molecular weight increases water permeability, facilitating polymer decomposition branching through the formation of secondary active sites (Fitzgerald and Corrigan Citation1993), the acceleratory coefficient ke should decrease with increasing molecular weight for the same kind of polymer. This is consistent with the values of ke shown in . Tmax reflects the matrix half erosion time; therefore, it should increase with increasing molecular weight as long as matrix erosion can happen. This is also consistent with the values for Tmax shown in .
From the above analysis it is clear that the parameters obtained are consistent with theoretical analysis, which shows that the new model is theoretical sound and may be useful for the purpose of prediction.
Progesterone Release from γ-Irradiated dl-PLA Microspheres
The release profiles of progesterone from γ-irradiated poly(DL-lactide) (dl-PLA) microspheres with different γ-irradiation dose were measured by Yoshioka and Kojima (Citation1995) (data sets 4–7). These data were used to test the capability of the new model for describing the γ-irradiation dose-dependent drug release profiles. The first three sets of data (data sets 4–6) were adopted to fit the model parameters and to establish the relationships between the parameters and the γ-irradiation dose. Then, the established relationships were used to predict the drug release profile of γ-irradiation dose = 100 kGy to test the predictive capability of the new model. The fitted results are shown in and the parameters obtained are reported in . Obviously, the new model shows excellent representation of the release profiles.
FIG. 2. Comparison of the experimental progesterone release profiles (symbols) under different irradiation dose from dl-PLA microspheres with the theoretical profiles of this work (lines).
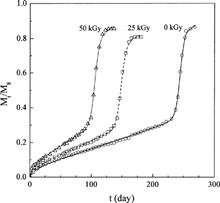
An examination of the parameters listed in shows that the parameters are theoretical sound and have good tendency. The values of D0 and ks increase with increasing irradiation dose. This is reasonable since the dl-PLA chains cleave faster under higher dose of γ-irradiation, leading to a larger decrease in molecular weight, which accelerates the subsequent polymer chain scission rate during drug release, which in turn leads to larger D0 and ks. ke is a constant due to the same polymer used. The decrease of Tmax with increasing γ-irradiation dose indicates that the matrix decomposition process is advanced as the polymer chains cleave faster. FE, on the other hand, is nearly a constant since increasing the γ-irradiation dose enhances both drug diffusion and matrix erosion, and the opposite contribution of drug diffusion and matrix erosion to FE leads to a nearly constant FE.
The values of the model parameters D0, ks and Tmax show good trend, encouraging us to establish quantum relationships between them and the γ-irradiation dose. The dependency of these parameters on γ-irradiation dose is shown in . It seems that they have simple relationships with γ-irradiation dose, and three simple correlations were proposed for them, as shown in . With the three correlations and taking the values of FE and ke as constants, the release profile for γ-irradiation dose of 100 kGy was predicted and the results are depicted in . Since the prediction is an extrapolation, the results shown in can be thought to be satisfactory. As a result, these calculations show that the new model not only gives excellent correlative accuracy, but also can be used to predict the γ-irradiation dose effects on release profiles, making the new model of practical usefulness.
FIG. 3. Dependency of DO, ks, and ln(Tmax) on the γ-irradiation dose (symbols = optimum values, solid lines = linear fit).
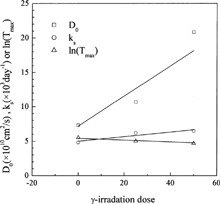
FIG. 4. Comparison of the experimental progesterone release profile from dl-PLA microspheres under 100 kGy irradiation dose with the predicted profile of this work.
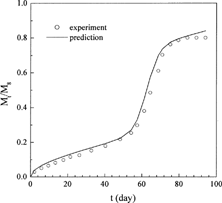
TABLE 3 Correlations for the model parameters with γ-irradiation dose and the values obtained for predicting progesterone release from dl-PLA microspheres irradiated at 100 kGy dose
Drug Release from Disks
As a second step to test our new model, experimental data of drug release profiles from disk polymer matrices were collected from the literature. Ahola et al. (Citation1999) and Rich et al. (Citation2001) measured the release profiles of toremifene citrate (TC) from poly(ε-caprolactone-co-DL-lactide) (P(LA-CL)) matrix under various conditions. These data are used to test the correlative accuracy as well as the predictive capability of the new model for describing the effects of various factors on drug release profiles.
Incorporation of Toremifene Citrate into Silica Xerogel
Rich et al. (Citation2001) measured the release profiles of TC from the (P(LA-CL)/drug) matrix device and the (P(LA-CL)/drug impregnated silica xerogel) composite device to compare the influence of the incorporation of drug into silica xerogel (data sets 8 and 9). The fitting curves of the new model (Equation [8]) are shown in , and the parameters obtained are listed in . As can be seen from , the new model gives good representation of the experimental release profiles. From , it is obvious that D0 is increased by the incorporation of TC into silica xerogel. This is reasonable since Rich et al. (Citation2001) observed that drug diffusion is enhanced through the incorporation of drug into silica xerogels, leading to an increase in D0 and an decrease in FE. The other three parameters do not change largely because the same copolymer was used for the two measurements.
Influence of P(LA-CL) Copolymer Composition on TC Release
Ahola et al. (Citation1999) measured the release profiles of TC from the (P(LA-CL)/drug) matrix device (data sets 10–13) and the (P(LA-CL)/drug impregnated silica xerogel) composite device (data sets 14–17) for different copolymer compositions. Therefore, these data can be used to test if the new model can describe the dependency of TC release profiles on copolymer composition. The calculated results for TC release from the P(LA-CL) matrix at four copolymer compositions are depicted in , and the parameters obtained are reported in . Obviously, the new model can give good representation of all the release profiles, and the parameters obtained are in good trend. Polycaprolactone provides a very good permeation for drug molecules in spite of its long degradation time (Ye and Chien Citation1996), while polylactide behaves in the opposite way (Hiljanen-Vainio, Karjalainen, and Seppälä Citation1996). As a result, an increase in weight fraction of ε-caprolactone in the P(LA-CL) copolymer leads to an increase in D0 and Tmax and a decrease in the other three parameters. The values of the parameters obtained, as shown in , agree with this analysis.
FIG. 6. Comparison of the experimental TC release profiles (symbols) from P(LA-CL) disks with the theoretical profiles (lines) of this work.
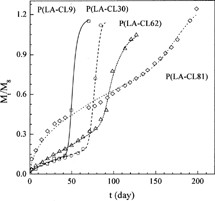
As to the TC release from the (P(LA-CL)/drug impregnated silica xerogel) composite device, the experimental data of three release profiles (data sets 15–17) were used to test the correlative accuracy of the new model. The calculated results are depicted in , and the parameters obtained are listed in . Again, good correlations were obtained and the parameters show good trend. shows the relationships between the parameters and the copolymer composition. Based on this figure, simple correlations were developed for them, as shown in . Then, the release profile for P(LA-CL9) was predicted, as shown in . The results in show that the new model is reliable for prediction and demonstrate that the new model is useful for the prediction of the effect of copolymer composition on drug release profiles based on limited experimental information.
FIG. 7. Comparison of the experimental TC-impregnated silica xerogel release profiles (symbols) from P(LA-CL) disks with the theoretical profiles (lines) of this work.
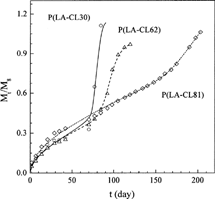
FIG. 8. Dependency of DO, ks, FE, ke, and ln(Tmax) on the CL weight fraction for P(LA-CL) copolymer (symbols = optimum values, solid lines = linear fit).
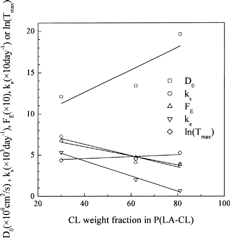
FIG. 9. Comparison of the experimental TC-impregnated silica xerogel release profile (symbols) from P(LA-CL9) disks with the predicted release profile (solid line) of this work.
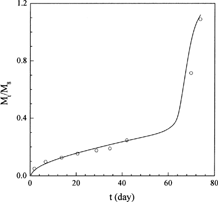
TABLE 4 Correlations for the model parameters with CL wt.% in the P(LA/CL) copolymer and the values obtained for predicting aclarubicin hydrochloride release from P(LA-CL9) disks
Influence of Initial Drug Loading on TC Release
Ahola et al. (Citation1999) also measured the release profiles of TC from P(LA-CL81) disks with different initial drug loadings (data sets 18–20). The fitting curves are given in and the parameters obtained are shown in . From , it is evident that good agreement with experimental data was obtained. The values of D0 and ks obtained are nearly constant; this is reasonable since the same drug and polymer were used. Since an increase in drug loading causes a reduction in polymer Tg that may increase water permeability, and facilitating polymer decomposition branching through the formation of secondary active sites (Fitzgerald and Corrigan Citation1993), therefore, ke should increase with increasing drug loading. For the same reasons, Tmax should decrease and FE should increase with increasing drug loading. The parameters obtained in this work, as shown in , are consistent with this analysis.
FIG. 10. Comparison of the experimental TC release profiles (symbols) from P(LA-CL81) disks with different drug loadings and the theoretical profiles (lines) of this work.
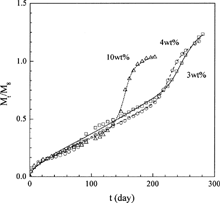
The relationships between the three parameters FE, ke, and Tmax and drug loading are shown in . Again, simple correlations between them can be obtained as shown in . These correlations can be used to predict the effects of drug loadings on release profiles with good accuracy.
FIG. 11. Dependency of FE, ke, and ln(Tmax) on the initial drug loadings (symbols = optimum values, solid lines = linear fit).
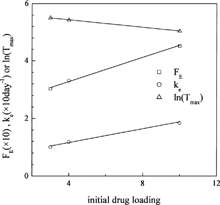
TABLE 5 Correlations for the model parameters with the initial drug loading and the values obtained for predicting aclarubicin hydrochloride release from P(LA-CL) disks
Finally, it should be pointed out that the new models can distinguish the individual contributions of diffusion and erosion to the total drug release profile as a function of time. Therefore, they are very useful for studying the underlying mechanisms of drug release from a polymer matrix.
CONCLUSION
The models proposed here are very simple and easy to apply, in which most phases involved in drug release processes from bioerodible polymer matrices are considered. The models can give excellent representation of drug release profiles under various conditions, and the parameters have good physical meanings and can be correlated to various factors, such as γ-irradiation dose, copolymer composition, and initial drug loading. Therefore, the new models can predict the effects of various factors on drug release profiles once limited experimental data are available. As a result, the new models can be used for drug delivery device design to achieve a certain desired release profile, and to minimize the number of required experimental studies. They also can be used to study the underlying mechanisms for a certain release process.
The financial support of the Teaching and Research Award Program for Outstanding Young Teachers in Higher Education Institutions of MOE, P.R.C., and the Trans-Century Training Programme Foundation for the Talents by the MOE, P.R.C., is greatly appreciated.
REFERENCES
- Ahola M., Rich J., Kortesuo P., Kiesvaara J., Seppälä J., Yli-Urpo A. In vitro evaluation of biodegradable ε-caprolactone-co-DL-lactide/silica xerogel composites containing toremifene citrate. Int. J. Pharm. 1999; 181: 181–191, [PUBMED], [INFOTRIEVE], [CSA]
- Baker R. W., Lonsdale H. K. Controlled release: mechanisms and rates. Controlled Release of Biologically Active Agents, A. C. Tanquarry, R. E. Lacey. Plenum Press, New York 1974; 15–71
- Charlier A., Leclerc B., Couarraze G. Release of mifepristone from biodegradable matrices: experimental and theoretical evaluations. Int. J. Pharm. 2000; 200: 115–120, [PUBMED], [INFOTRIEVE], [CROSSREF], [CSA]
- Crank J. The Mathematics of Diffusion. Clarendon Press, Oxford 1975
- Faisant N., Siepmann J., Benoit J. P. PLGA-based microparticles: elucidation of mechanisms and a new, simple mathematical model quantifying drug release. Eur. J. Pharm. Sci. 2002; 15: 355–366, [PUBMED], [INFOTRIEVE], [CROSSREF], [CSA]
- Faisant N., Siepmann J., Richard J., Benoit J. P. Mathematical modeling of drug release from bioerodible microparticles: effect of gamma-irradiation. Eur. J. Pharm. Biopharm. 2003; 56: 271–279, [PUBMED], [INFOTRIEVE], [CROSSREF], [CSA]
- Fan L. T., Singh S. K. Controlled Release: A Quantitative Treatment. Springer-Verlag, Berlin 1989
- Fitzgerald J. F., Corrigan O. I. Mechanisms governing drug release from poly α-hydroxy aliphatic esters, diltiazem base release from poly-lactide-co-glycolide delivery systems. Polymeric Delivery Systems, Properties and Applications, ACS Symposium Series, 520, M. A. El-Nokaly, D. M. Piatt, B. A. Charpentier. American Chemical Society, Washington, DC 1993; 311–326
- Göpferich A. Mechanism of polymer degradation and erosion. Biomaterials 1996; 17: 103–114, [CROSSREF], [CSA]
- Hiljanen-Vainio M. P., Karjalainen T., Seppälä J. V. Biodegradable lactone copolymers. I. Characterization and mechanical behaviour of ε-caprolactone and lactide copolymers. J. Appl. Polym. Sci. 1996; 59: 1281–1288, [CROSSREF], [CSA]
- Kim T. H., Park T. G. Critical effect of freezing/freeze-drying on sustained release of FITC-dextran encapsulated within PLGA microspheres. Int. J. Pharm. 2004; 271: 207–214, [PUBMED], [INFOTRIEVE], [CROSSREF], [CSA]
- Langer R. Polymeric delivery systems for controlled drug release. Chem. Eng. Commun. 1980; 6: 1–48, [CSA]
- Langer R., Wise D. L. Medical Applications of Controlled Release. Classes of Systems. CRC Press, Boca Raton, FL 1984; vol. 1
- Lee P. I. Diffusional release of a solute from a polymeric matrix—approximate analytical solutions. J. Membr. Sci. 1980; 7: 255–275, [CROSSREF], [CSA]
- Lemaire V., Bélair J., Hildgen P. Structural modeling of drug release from biodegradable porous matrices based on a combined diffusion/erosion process. Int. J. Pharm. 2003; 258: 95–107, [PUBMED], [INFOTRIEVE], [CROSSREF], [CSA]
- Mallardé D., Boutignon F., Moine F., Barré E., David S., Touchet H., Ferruti P., Deghenghi R. PLGA-PEG microspheres of teverelix: influence of polymer type on microsphere characteristics and on teverelix in vitro release. Int. J. Pharm. 2003; 261: 69–80, [CROSSREF], [CSA]
- Pitt C. G., Gu Z. Modification of the rates of chain cleavage of poly(ε-caprolactone) and related polyesters in the solid state. J. Control. Rel. 1987; 4: 283–292, [CROSSREF], [CSA]
- Rich J., Kortesuo P., Ahola M., Yli-Urpo A., Kiesvaara J., Seppälä J. Effect of the molecular weight of poly(ε-caprolactone-co-DL-lactide) on toremifene citrate release from copolymer/silica xerogel composites. Int. J. Pharm. 2001; 212: 121–130, [PUBMED], [INFOTRIEVE], [CROSSREF], [CSA]
- Ritger P. L., Peppas N. A. A simple equation for description of solute release. I. Fickian and non-Fickian Release from non-swellable devices in the form of slabs, spheres, cylinders or disks. J. Control Rel. 1987; 5: 23–36, [CROSSREF], [CSA]
- Siepmann J., Göpferich A. Mathematical modeling of bioerodible, polymeric drug delivery systems. Adv. Drug Deliv. Rev. 2001; 48: 229–247, [PUBMED], [INFOTRIEVE], [CROSSREF], [CSA]
- Siepmann J., Faisant N., Benoit J. P. A new mathematical model quantifying drug release from bioerodible microparticles using Montro Carlo simulations. Pharm. Res. 2002; 19: 1885–1893, [PUBMED], [INFOTRIEVE], [CROSSREF], [CSA]
- Siepmann J., Faisant N., Akiki J., Richard J., Benoit J. P. Effect of the size of biodegradable microparticles on drug release: experiment and theory. J. Control. Rel. 2004; 96: 123–134, [CROSSREF], [CSA]
- Wada R., Hyon S. H., Ikada Y., Nakao Y., Yoshikawa H., Muranishi S. Lactic acid oligomer microspheres containing an anticancer agent for selective Imphatic delivery: 1. In vitro studies. J. Bioact. Compat. Polym. 1988; 3: 126–136, [CSA]
- Wada R., Hyon S. -H., Nkamura T., Ikada Y. In vitro evaluation of sustained drug release from biodegradable elastomer. Pharm. Res. 1991; 8: 1292–1296, [PUBMED], [INFOTRIEVE], [CROSSREF], [CSA]
- Wada R., Hyon S. H., Ikada Y. Kinetics of diffusion-mediated drug release enhanced by matrix degradation. J. Control. Rel. 1995; 37: 151–160, [CROSSREF], [CSA]
- Ye W. -P., Chien Y. W. Dual-controlled drug delivery across biodegradable copolymer. I. Delivery kinetics of levonorgestrel and estradiol through (caprolactide/lactide) bolock copolymer. Pharm. Dev. Technol. 1996; 1: 1–9, [PUBMED], [INFOTRIEVE], [CSA]
- Yoshioka S., Otsuka Y. A. T., Kojima S. The effect of γ-irradiation on drug release from poly(lactide) microspheres. Radiat. Phys. Chem. 1995; 46: 281–285, [CROSSREF], [CSA]
- Yoshioka S., Kojima Y. A.S. Drug release from poly(dl-lactide) microspheres controlled by γ-irradiation. J. Control. Rel. 1995; 37: 263–267, [CROSSREF], [CSA]