Abstract
Bovine serum albumin nanospheres carrying cyclodextrin complexes for the delivery of progesterone were produced. Inclusion complexes composed of progesterone and hydroxypropyl-β -cyclodextrin or dimethyl-β -cyclodextrin were prepared by spray-drying or freeze-drying methods. Prog alone and its inclusion complexes were incorporated into bovine serum albumin nanospheres using a coacervation method and cross-linking with heating. The nanosphere suspensions were essicated by spray-drying or freeze-drying. The inclusion complexes and the nanospheres were characterized by Fourier Transform-Infrared Spectroscopy (FT-IR) and Differential Scanning Calorimetry (DSC). Phase-solubility diagrams and stability constants were determined in distilled water at different temperatures (10, 25, and 37°C). Size of nanospheres, their drug loading capacity and swelling ability were evaluated, as well as the in vitro controlled release profiles at pH 5.5 and 7.4.
Most of the promising antitumoral agents present poor water solubility, and intravenous administration of these drugs is delayed frequently due to the lack of appropriate delivery carriers. Various approaches were employed such as emulsions, micelles, liposomes, nanospheres, and cyclodextrins. Cyclodextrins have been used extensively to increase the solubility, the dissolution rate, and the bioavailability of poorly soluble drugs (Uekama and Otagiri Citation1987). They also have been used to increase the stability of labile drugs (Uekama et al. Citation1983) and improve the performance of intravenous formulations (Etes et al. Citation1991). Unfortunately, high cyclodextrin concentrations may cause hemolysis, and other formulation approaches must be taken into consideration, including drug conjugation to macromolecular carriers. Drug-albumin conjugates have been prepared in which the drug is covalently linked to serum albumin through different spacers (Dosio et al. Citation1997) or physically loaded in the proteic carrier (Orienti and Zecchi Citation1993). This work describes the use of bovine serum albumin (BSA) nanospheres with size of ∼100 nm able to carry inclusion complexes composed of different cyclodextrins and progesterone, chosen in this study as a well known lipophilic antitumoral drug (Grubs and Whitaker Citation1988; Higuchi and Connors Citation1965). As colloidal carriers, nanospheres present high stability in blood fluids and can accumulate in body regions with leaky vasculature (EPR effect) (Hiroshi Citation2001). Being enclosed in the nanospheres, the cyclodextrin complexes do not provoke hemolysis and the drug does not cause undesirable side effects (Kazunori, Atsushi, and Nagasaki Citation2001). The objective of our work was to find a new family of carriers whose characteristics could allow preparation of systems stable in the aqueous environment and able to control drug release. The stability of the nanospheres and their functional properties were evaluated to define the best conditions favoring the parenteral administration of progesterone.
MATERIALS AND METHODS
Materials
Progesterone, 2-hydroxypropyl-β -cyclodextrin, 2,4-dimethyl-β -cyclodextrin, and BSA were purchased from Fluka (Milan, Italy) and all the solvents employed were from Carlo Erba (Milan, Italy).
Progesterone-Cyclodextrin Inclusion Complex
Progesterone-β -cyclodextrin inclusion complexes were prepared by freeze-drying (Prog-β CDFD) or spray drying (Prog-β CDSD) techniques. Suspensions of progesterone and the different β -cyclodextrins were prepared in the molar ratio 1:1. Briefly, progesterone was dispersed in 50 ml of water containing 2-hydroxypropyl-β -cyclodextrin or 2,4-dimethyl-β -cyclodextrin and mixed for 7 days at room temperature. The suspensions were filtered through a 0.22 μ m filter (Albet-Jacs, Valencia, Spain). The filtrates were frozen and then freeze-dried (Christ, Milan, Italy) and subsequently homogenized to particle size of ∼100 μm (IKA A 11 Basic Analytical Mill) or essiccated by spray-drying (Büchi, Milan, Italy).
Solubility Studies
Solubility studies were performed according to the method reported by Higuchi and Connors (Citation1965). Progesterone in amounts that exceeded its solubility (50 mg) was weighed carefully into a 50-ml Erlenmeyer flask to which 20 ml of aqueous solutions containing the different β CDs at various concentrations (0–0.016 mol/l) were added. The flasks were sealed and equilibrated by shaking at 10°C, 25°C, and 37°C in distilled water. When equilibrium had been reached (5 days), the samples were filtered through a 0.22 μ m filter (Albet-Jacs) and the concentration of progesterone was measured spectrophotometrically at 244 nm (UV-VIS, Shimadzu, Kyoto, Japan).
The apparent 1:1 stability constants, Ks, were calculated from the slope of the initial straight line portion of the phase solubility diagram according to the following equation (Ashwinkumar and Moji Citation2001):
where So is the saturation concentration of the drug measured without cyclodextrin (intercept of the line).
BSA Nanospheres Containing Inclusion Complexes
First, 0.25 g of BSA was dissolved in 50 ml of water. The different Prog-β CD complexes were dissolved in this solution. Second acetone was added dropwise to this solution (stirred continuously with a magnetic stirrer at room temperature) to produce a ratio 2:1 (v/v) acetone:BSA solution. Magnetic stirring was continued at 70°C for 30 min to evaporate the acetone and yield an aqueous suspension of albumin nanospheres. Then the nanosphere suspension was frozen and freeze-dried and subsequently homogenized to particle size of ∼100 μm or essicated by spray-drying.
Morphology Analysis
The morphologies of the spray-dried and freeze dried nanospheres were observed using a scanning electron microscope (LEO 420, LEO Electron Michroscopy Ltd., Cambridge, England). The microspheres were mounted onto brass stubs and sputter-coated with gold in an argon atmosphere prior to examination under scanning electron microscopy (SEM).
FT-IR/DSC
Infrared (IR) spectra were recorded with a Jasco FT-IR-410 spectrophotometer. The samples were prepared by processing compressed KBr disks. Differential scanning calorimetric (DSC) analysis were performed using a Perkin-Elmer DSC 6. All samples (7–10 mg) were heated in crimped aluminium pans at a scanning rate of 10°C/min in the temperature range 25–180°C.
Nanospheres Swelling Behavior
To evaluate the swelling behavior in alkaline and acidic environments, all the nanospheres were suspended in pH 7.4 or pH 5.5 buffers and their size was monitored for 24 hr by photon correlation spectroscopy (PCS). Particle size distributions were measured by PCS using an instrument (Brookhaven 90-PLUS) with an He–Ne laser beam at a wavelength of 532 nm (scattering angle of 90°) (Chu Citation1974; Berne and Pecora Citation1976). Nanospheres suspensions were used for particle size measurement without filtering.
In Vitro Release Studies
In vitro release profiles of the Prog, Prog-HPβ CDFD, Prog-DMβ CDFD, Prog-HPβ CD-BSAFD, Prog-DMβ CD-BSAFD, Prog-HPβ CDSD, Prog-DMβ CDSD, Prog-HPβ CD-BSASD, and Prog-DMβ CD-BSASD were examined in pH 7.4 and pH 5.5 phosphate buffers. The drug, the complexes, or the nanospheres were introduced into a donor cell containing 3 ml of the aqueous phase separated by a dialysis membrane (cellulose acetate, Mw cut off = 14.000, Delchimica Scientific Glassware) from a receiving compartment containing 10 ml of the same aqueous buffer. The solution was replaced after time intervals suitable to guarantee sink conditions throughout the runs. The studies were performed at 37°C and the drug was detected in the receiving phase by an HPLC method. The amount of Prog in the complexes (Mo) was determined supplementing 10 mg of each sample with 10 ml acetonitrile/water 3:2 (v/v) mixture. The amount of Prog in the nanospheres (Mo) was determined supplementing 10 mg of each sample with 10 ml water/ethanol/NaOH 1M 4:5:1 (v/v) mixture and subsequently purifing this solution by means of a Sep-Pak C18 cartridge obtained from Waters (Milford, MA, USA). Finally, all the solutions were filtered (Albet, Valencia, 0.45 μ m) and the drug amount detected by HPLC. All experiments were carried out in triplicate and average values were plotted.
Chromatographic Conditions
Chromatographic separations were performed using a Shimadzu (model SPD-10A) liquid chromatograph connected to a UV-Vis detector (SPD-10AV) and to a computerized integration system ChromatoPlus (Shimadzu, Kyoto, Japan). Manual injections were made using a Rheodyne 7125 injector with a 20-μ l sample loop. Separations were obtained on a C18 Phenomenex Luna (4 μ m, 150 × 4.60 mm i.d.) (Chemtek Analitica, Bologna, Italy) column at room temperature using 60:40 (v/v) acetonotrile:water mixture at a flow rate of 1.0 ml/min. Ultraviolet absorption was read at 244 nm.
Statistics
All data are presented as arithmetic mean values ± standard deviation. Level of significance was analyzed using Student's t-test.
RESULTS
Progesterone-β -Cyclodextrin Complexation
The phase solubility diagrams for complex formation between Prog and HPβ CD or Prog and DMβ CD are presented in . The plots show typical AL-type solubility curves classified by Higuchi and Connors (Citation1965), showing that soluble complexes were formed. The solubility of Prog increased in a linear fashion as a function of HPβ CD or DMβ CD concentration. The stability constant calculated at 37°C for HPβ CD (KsHPβ CD = 34894 M−1) was lower than the stability constant calculated at 37°C for DMβ CD (KsDMβ CD = 64508 M−1), indicating the higher ability of the methylated cyclodextrin to interact with progesterone in aqueous environment.
Morphology Analysis
The scanning electron micrographs of the spray-dried and freeze-dried nanospheres are shown in . The spray-dried nanospheres formed spherical microspheres with an average diameter of 2 μ m. The surface of microspheres observed by SEM was spherical and smooth. Moreover, some free nanospheres can be observed on the microspheres surfaces. The freeze-dried nanospheres after homogenization formed irregularly shaped microparticles at ∼100 μ m. Both spray-dried and freeze-dried samples do not aggregate and could be easily dispersed in acqueous phase.
FT-IR
shows the IR spectra of Prog, HPβ CD, Prog-HPβ CDSD, and Prog-HPβ CDFD. shows the IR spectra of Prog, DMβ CD, Prog-DMβ CDSD, and Prog-DMβ CDFD. The peaks at 1661 cm− 1 and 1698 cm− 1 are assigned to carbonyl-stretching bands of C3 and C20 in progesterone, respectively. In the spectrum of the spray-dried and freeze-dried complexes, the stretching bands of the carbonyl group appear markedly reduced (DMβ CD complexes) or totally reduced (HPβ CD complexes) in intensity. This probably can be due to a deeper inclusion complexation of Prog into the HPβ CD cavity with respect to DMβ CD and consequently to a greater dissociation of the intermolecular hydrogen bonds of progesterone through inclusion complexation (Uekama et al. Citation1982). shows the IR spectra of Prog, BSA, Prog-BSASD, Prog-HPβ CD-BSASD, and Prog-DMβ CD-BSASD. The presence of albumin in the nanospheres totally hides the progesterone peaks and weakly masks the characteristic absorption bands of the cyclodextrins indicating protein ability to encapsulate the pure drug and the complexes.
DSC
Differential scanning calorimetry (DSC) was performed on the pure drug, on the inclusion complexes, and on the nanospheres. The thermogram of Prog shows the characteristic endothermic peak at 125.3°C, corresponding to drug melting. The thermal behavior of the other components used for complex or nanosphere formation, i.e., HPβ CD, DMβ CD, and BSA, show no phenomena in the same temperature interval ().
FIG. 5. DSC thermograms of pure drug, inclusion complexes, and nanospheres: Prog (a), DMβ CD (b), Prog-DMβ CDSD (c), HPβ CD (d), Prog-HPβ CDSD (e), BSA (f), Prog-DMβ CD-BSASD (g), and Prog-HPβ CD-BSASD (h).
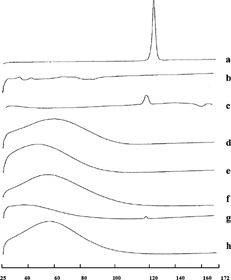
The Prog-HPβ CD and Prog-DMβ CD complexes showed disappearance and the reduction of the endothermal peak of Prog, respectively, indicating that the drug penetrates into the cyclodextrin cavity replacing the water molecules confirming inclusion complex formation. This behavior is more evident in the presence of albumin: the disappearance of Prog crystalline peak confirmed the stronger drug amorphization effect due to the combined action of albumin and cyclodextrins.
Nanospheres Swelling Behavior
All albumin nanospheres were able to swell in aqueous solution due to the hydrophilic nature of the protein itself. reports the mean diameter calculated immediately after suspending the nanospoheres (d0) and after 24 hr (d24) and the swelling degree (SD = d24/d0). The presence of HPβ CD in the nanospheres provided greater swelling than DMβ CD. This can be attributed to the higher hydrophilicity of HPβ CD with respect to the methylated cyclodextrin. Moreover, the swelling was more evident at pH 5.5, because despite albumin presents its lowest solubility near the isoelectric point (Lee, Ko, and Kim Citation2002; Chun and Stroeve Citation2002). Finally, all the nanospheres obtained by freeze-drying showed higher initial and lower swelling ability than nanospheres obtained by spray-drying; this can be due to the cooling process probably destabilizing the nanospheres' network.
TABLE 1 Mean diameter (nm) of Prog-β CD-BSA nanospheres calculated immediately after suspending the nanospoheres (d0) and after 24 hr (d24) and swelling degree (SD) in phosphate buffer at pH 7.4 and pH 5.5
DISCUSSION
In Vitro Release Studies
The in vitro release profiles of Prog and Prog-β CD complexes and Prog-β CD-BSA nanospheres are shown in (freeze-dried) and (spray-dried). The dissolution profiles of the complexes were compared with pure drug. Due to the amorphous nature (DSC data confirming) and an increase in water solubility following complexation, the Prog-HPβ CD and Prog-DMβ CD complexes showed significantly higher dissolution releases compared with the corresponding pure drug.
FIG. 6. Release profile of progesterone in phosphate buffer at pH 7.4 (A) and pH 5.5 (B) from the pure drug (□), Prog-HPβ CDFD (○), Prog-DMβ CDFD (×), Prog-HPβ CD-BSAFD (*), Prog-DMβ CD-BSAFD (⋄).
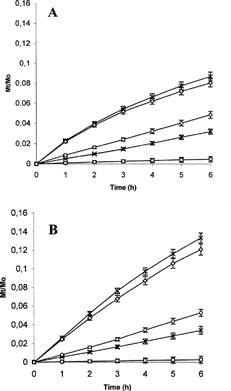
FIG. 7. Release profile of progesterone in phosphate buffer at pH 7.4 (A) and pH 5.5 (B) from the pure drug (□), Prog-HPβ CDSD (○), Prog-DMβ CDSD(×), Prog-HPβ CD-BSASD (*) and Prog-DMβ CD-BSASD(⋄).
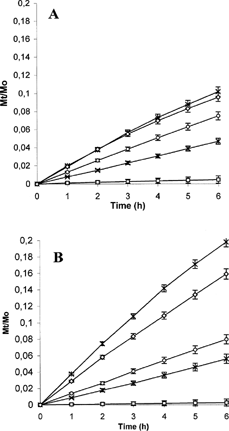
Spray-dried products presented higher release profiles than freeze-dried products at each pH analyzed. This can be due to the high dissolution surface present in the spray-dried products that are represented by spherical, homogeneous and small sized (1–5 μm) powders. Moreover, as confirmed by dynamic light scattering measurements, the nanospheres obtained by spray-drying presented lower diameter and higher swelling ability, thus providing an increased drug diffusion from the system.
The presence of albumin increased the release at pH 5.5 due to its high swelling. This is an important functional property for this carrier whose characteristics could allow preparation of systems stable in the aqueous neutral environment and able to guarantee drug release in particular acidic environments.
Finally, the nanospheres containing HPβ CD were able to release a higher fractional amounts of drug than the nanospheres containing DMβ CD. This result is in accordance with the lower stability constant calculated for HPβ CD, confirming the tendency of progesterone to diffuse in the aqueous phase rather than stand in the cyclodextrin cavity.
CONCLUSIONS
The solubility of progesterone can be increased by inclusion complexation with HPβ CD. The Prog-HPβ CDSD and Prog-HPβ CDFD complexes were characterized by enhanced dissolution rates with respect to the pure drug. The possibility of loading these complexes in albumin nanospheres with size ∼100 nm could limit cyclodextrin undesirable side effects and could allow drug accumulation in body regions with leaky vasculature. As albumin nanospheres presented a good stability in aqueous neutral environments and provided a pH-dependent drug release, they can represent a new family of carriers for the parenteral administration of progesterone.
REFERENCES
- Ashwinkumar C. J., Moji C. A. Hygroscopicity, phase solubility and dissolution of various substituted sulfobutylether β -cyclodextrins (SBE) and danazol-SBE inclusion complexes. Int. J. Pharm. 2001; 212(2)177–186, [CSA]
- Berne B., Pecora R. Dynamic Light Scattering with Application to Chemistry, Biology and Physics. Wiley-Interscience, New York 1976
- Chu B. Laser Light Scattering. Academic Press, New York 1974
- Chun K. Y., Stroeve P. Protein transport in nanoporous membranes modified with self-assembled monolayers of functionalized thiols. Langmuir 2002; 18: 4653–4658, [CROSSREF], [CSA]
- Dosio F., Brusa P., Crosasso P., Arpicco S., Cattel L. Preparation, characterization and properties in vitro and in vivo of a paclitaxel-albumin conjugate. J. Cont. Rel. 1997; 47: 293–304, [CROSSREF], [CSA]
- Etes K. S., Brewster M. E., Webb A. I., Bodor N. A non-surfactant formulation of alfaxalone based on an amorphous cyclodextrin. Activity studies in rats and dogs. Int. J. Pharm 1991; 65: 101–107, [CROSSREF], [CSA]
- Grubs C. J., Whitaker L. M. Short-term hormone treatment as a chemopreventive method against mammary cancer initiation in rats. Anticancer Res. 1988; 8: 113–118, [CSA]
- Higuchi T., Connors K. A. Phase-solubility techniques. Adv. Anal. Chem. Instrum. 1965; 4: 117–212, [CSA]
- Hiroshi M. The enhanced permeability and retention (EPR) effect in tumor vasculature: the key role of tumor-selective macromolecular drug targeting. Adv. Enzyme Regul. 2001; 41(1)189–207, [CROSSREF], [CSA]
- Kazunori K., Atsushi H., Nagasaki Y. Block copolymer micelles for drug delivery: design, characterization and biological significance. Adv. Drug Del. Rev. 2001; 47: 113–131, [CROSSREF], [CSA]
- Lee W. K., Ko J. S., Kim H. M. Effect of electrostatic interaction on the adsorption of globular proteins on octacalcium phosphate crystal film. J. Colloid Interface Sci. 2002; 246: 70–77, [CROSSREF], [CSA]
- Orienti I., Zecchi V. Progesterone-loaded albumin microparticles. J. Controll. Rel. 1993; 27: 1–7, [CROSSREF], [CSA]
- Sivaraman L., Stephens L. C., Markaverich B. M., Clarck J. A., Krnacik S., Conneely O. M., O'Malley B. W., Medina D. Hormone-induced refractoriness to mammary carcinogenesis in Wistar-Furth rats. Carcinogenesis 1998; 19: 1573–1581, [PUBMED], [INFOTRIEVE], [CROSSREF], [CSA]
- Uekama K., Fujinaga T., Hirayama F., Otagiri M., Yamasaki M. Inclusion complexations of steroid hormones with cyclodextrins in water and in solid phase. Int. J. Pharm. 1982; 10(1)1–15, [CROSSREF], [CSA]
- Uekama K., Narisawa S., Hirayama F., Otagiri M. Improvement of dissolution and absorption characteristics of benzodiazepines by cyclodextrin complexation. Int. J. Pharm. 1983; 16: 327–338, [CROSSREF], [CSA]
- Uekama K., Otagiri M. Cyclodextrins in drug carrier systems (review). CRC Crit. Rev. Ther. Carrier Sys. 1987; 3: 1–40, [CSA]