Abstract
Based on the clinical fact that norcantharidin (NCTD) injection frequently causes urinary organ damnification, we prepared a NCTD w/o microemulsion as an alternative to improve the solubility and enhance the live uptake of NCTD. The optimal microemulsion formulation consisted of 7% water, 45% soybean lecithin/ethanol (2:1), and ethyl oleate, with the droplet size of 44.5 ± 8.6 nm. Pharmacokinetics study showed that NCTD microemulsion had relatively longer circulating time in mice than NCTD injection after a single intravenous injection at a dose of 5 mg/kg. Moreover, the overall drug targeting efficiency of liver was enhanced from 3.66% to 6.10%. These results suggest that a NCTD microemulsion system is a promising candidate for the treatment of hepatogenic diseases.
Norcantharidin (NCTD), a derivative of cantharidin, is one of the most effective anticancer drugs against hepatogenic cancer, hepatocirrhosis, hepatitis carrier, esophageal carcinoma, gastric carcinoma, breast cancer, and lung cancer. One of the advantages of NCTD is that it can promote the growth of leukocytes, which is different from other anticancer drugs. However, the successful treatment of conventional NCTD formulation remains difficult because of several formulation and pharmacokinetics factors that affect its disposition and toxicity, especially in kidneys. The high concentration of NCTD in kidneys was attributed to the occurrence of the renal damage (Wang Citation1983; Dong Citation1984). The clinical usefulness of NCTD is limited by its high renal damage effect and poor liver entry. Furthermore, the solubility of NCTD is very low in ethanol and cold water but relatively high in acetone and warm water. Therefore, the utilization of novel drug delivery systems holds great promise to improve therapeutic effects and in vivo applications.
Lipid-based microemulsions have been proposed to enhance the bioavailability of drugs (Kenji and Tomohiro Citation2001). The microemulsions delivery systems configure pharmacokinetic profiles that differ from those of free drug (Uif and Kozo Citation1986). These differences are related to the characteristics of the vehicles, which affect the extent of the tissue distribution and thereafter the disposition profile and efficacy of the drug (Hao Citation2000). In our previous work, water-in-oil microemulsion (ME) systems have been developed using commercially available and pharmaceutically acceptable components. We have investigated the influence of a range of oils on the formation of lecithin-based microemulsions as well as the physiochemical properties of NCTD microemulsions in vitro (Zhang et al. Citation2004).
The purpose of this study was to formulate an optimized NCTD-loaded w/o ME system for the intravenous delivery of NCTD. The concentrations of NCTD in organs and plasma were determined after intravenous administration of NCTD-loaded ME in mice by the gas chromatography (GC) method. In addition, the biodistribution and pharmacokinetics of NCTD ME were evaluated and compared with those of NCTD injection.
MATERIALS AND METHODS
Norcantharidin standard and injection were purchased from Peking 4th Pharmaceutical factory (China). Ethyl oleate was supplied by Shanghai Chemical Co. (China). Soybean lecithin was obtained from Lucas Meyer (Epikuron 200, which has a distribution of fatty acids of C16:0 = 13.3%, C18:1 = 10.2%, C18:2 = 66.9%, and C18:3 = 6.6%). The water was purified with a Super-Q water purification system from Milipore. All the reagents were of the analytical grade and used as supplied.
Preparation of Microemulsion
The ME preparation consisted of 30% lecithin, 15% ethanol, 48% ethyl oleate, and 7% water. The NCTD-loaded ME was prepared as follows. Ethyl oleate was blended with lecithin/ethanol (2:1) in certain ratios. An aliquot of water was then added into the mixture of ethyl oleate and complex surfactants with a constant stirring until the mixture became clear or translucent ME at ambient temperature (25°C). Afterward norcantharidin was dispersed into the blank microemulsion. The final dispersion for the study was obtained after filtration through a 0.2-μm membrane.
Particle Size and Morphology
A Malvern Photon Correlation Spectrometer model ZETASIZER nano-zs90 equipped with an argon laser model 2000 from Spectra Physics was used to monitor the particle size of microemulsions. The predetermined viscosity and refractive index of microemulsions were incorporated into the computer software that calculates the mean particle size and polydispersity from intensity, mass, and number distribution. Measurements were carried out at 25°C and at a scattering angle of 90°. All sizes are given z-average-mean values.
The morphology of ME droplets was observed by transmission electron microscopy, using negative staining method. In brief, the microemulsion was stained with 1% solution of phosphotungstic acid sodium salt at pH 7.5 for contrast enhancement. The air-dried samples were then directly examined under the transmission microscope (JEM-100SX, Japan).
In Vitro Stability and Drug Release
Shelf-life stability of MEs both as a function of time and storage temperature were routinely evaluated by visual inspection of the samples initially on a daily and later on a weekly basis. Stable systems were identified as those free of any physical change, such as phase separation, flocculation, and /or precipitation. The centrifuge method, particle sizes, and viscosity measurements are used in determining the physical stability of microemulsions. With respect to the chemical stability, NCTD contents in MEs were analyzed in freshly made formulations and those after 30 days storage at 25°C.
The release of the drug was determined as described by Wakiyama et al. (Citation1981) In this case the carrier is diluted into a large volume of sink. Briefly, 0.5 ml of drug-loaded ME was poured into 500 ml of PBS (pH = 7.4) in a 1,000-ml container, equipped with a magnetic stirrer. At 10, 20, 30, 60, 120, 240, 360, 720, and 1440 min after the addition of the release medium to each beaker, 0.5 ml aliquots were sampled from the 500 ml release medium and filtered through the 0.3 μm membrane, then stored in the freezer prior to the analysis of NCTD by a GC method.
Body Distribution of NCTD Microemulsion in Mice
The Sichuan University animal ethical experimentation committee, according to the requirements of the National Act on the use of experimental animals (P. R. China), approved all procedures of the studies in vivo. First, 2 groups of Kunming mice weighing 18–22 g were intravenous given NCTD ME and NCTD injection at the dose of 5 mg·kg− 1, respectively. For each preparation and sampling time point, there are 5 mice. At predetermined times (5, 10, 20, and 30 min, and 1, 2, 3, 4, 6, 12, 24, 48, 96, 144, 240, and 360 hr), blood samples were collected from the ocular artery directly after eyeball removal and placed into test tubes containing 10 μl of heparin solution. Plasma was separated immediately by centrifugation at 8000 rpm for 15 min. The animals were dissected and the tissues, including heart, lung, liver, spleen, and kidney, were harvested and accurately weighed and stored at −20°C.
On the analysis day, tissue samples were thawed. The tissue samples were homogenized with 3 volumes of saline in a tissue homogenizer, and 10 ml/g extraction solvent (90% acetone, 10% methanol) was added to dilute the tissues. Then, 0.5 ml of aliquot were transferred into another tube, mixed with 50 μL or 70 μL cetane C16 (1 mg/ml) as the internal standard solution. Then the samples were sonicated for 1 min and centrifuged at 12000 rpm for 10 min. Next, the supernatants were transferred into other tubes in which they were evaporated to dryness under a gentle stream of nitrogen. The residues were dissolved by 100 μL of acetone and 1 μL of aliquots was injected into the GC (GC-15A, Shimadzu, Japan). For plasma sample, 150 μL of plasma was added into 600 μL of extraction solvent (90% acetone, 10% methanol). The mixture was vortexed for 5 min and centrifuged at 12000 for 10 min. Then, the supernatant were transferred into other tubes. The subsequent procedures were the as same as those for tissue samples described above.
Analysis Method
The GC assay methods were established for the determination of NCTD concentrations. The oven temperature was 130°C, and the inlet and detector temperatures were 170°C and 180°C, respectively. The column head pressure was set to 2.0 kg/cm2. The carrier gas was nitrogen with the flow rate of 40 ml/min. The injection manner was split injection. The measurements were performed at least in duplicate for each formulation.
Calibration curves of NCTD were prepared with plasma and respective tissues spiked with known amounts of the drug utilizing its GC peak height ratio to the internal standard. Concentrations of NCTD in plasma and tissue samples were read from the respective calibration curve. The retention time of NCTD was about 5.6 min and the detection limits were 10 ng/ml for plasma and 15 ng/ml for tissue homogenates. The recoveries of NCTD from the plasma and tissue samples were more than 90 and 70%, respectively. Inter- and intraday variations were less than 6% for plasma samples and 10% for tissue samples.
Pharmacokinetic Analysis
Plasma and tissue concentration data of NCTD-loaded ME and NCTD injection obtained from mice were pooled to provide mean concentration data. The Akaike's information criterion (AIC) was used to select the best model. The pharmacokinetics of both were described as a two-compartment open model. The pharmacokinetic parameters in plasma and tissues were obtained as follows. The area under the concentration-time curve (AUC) was calculated using the linear trapezoidal rule and extrapolated to infinity by dividing the last measurable concentration by the elimination rate constant.
Tissue distribution data were evaluated using tissue uptake clearances (Shigeru, Nao, and Ryu Citation2002). The change of the drug amount in tissue with time can be described as follows:
where T(t) is the amount of drugs in 1g tissue, C(t) is the plasma concentration of drugs, Clin is the tissue uptake rate index (clearance) from blood to tissue, and Kout is the efflux rate constant from the tissues. To estimate the uptake rate index, distribution in the first 30 min after intravenous administration was measured, allowing the contribution of efflux processes and metabolism to be ignored. If efflux is ignored, Equation 1 is integrated as follows:
According to Equation 2, the tissue uptake rate index is calculated using the amount of drugs in tissue at 30 min and the area under the blood concentration-time curve (AUC) up to the time obtained through curve fitting to the blood concentration-time course based on a two-compartment model. Tissue uptake clearance (CLtissue) is expressed as follows:
where W(g) is the total weight of the tissues.
Targeting index (Re) and relative overall targeting efficiency (RTe) of NCTD were calculated to evaluate the liver targeting accumulation property of NCTD-loaded ME (Wang et al. Citation2002).
Overall targeting efficiency (Te) can be calculated as
where the denominator refers to the sum total of drug exposure to all the tissues, including the target tissue.
Statistical Analysis
The statistical significance of the differences between NCTD injection and NCTD ME in terms of pharmacokinetic parameters of NCTD after intravenoos (i.v.) administration was determined using the one-way analysis of variance (ANOVA). A p value of < 0.05 was chosen as the level of statistical significance. All results are expressed as mean ± standard deviation (S.D.).
RESULTS AND DISCUSSION
Characteristics of NCTD-Loaded Microemulsion
The particle size distribution is one of the most important characteristics of microemulsion for the evaluation of in vitro stability (Park et al. Citation1999) and the in vivo fate of microemulsion. Spherical particles could be detected under electronic microscope (). The system was optically transparent and gave a mean droplet diameter of the disperse phase ranging from 30 to 50 nm.
FIG. 1. Transmission electron microscopy photographs (× 20000) of NCTD-loaded microemulsion following negative staining with dilution factor of 1000×.
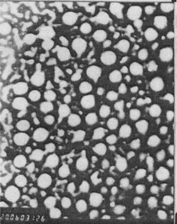
After storage at 4°C, 25°C, and 40°C for 1 month, NCTD-loaded ME showed no change in appearance and redispersing ability. The drug content after storage for 1 month at 25°C was 9.98 ± 0.21% (n = 3), which had no significant difference with the drug content before storage (9.87 ± 0.51%; n = 3; p < 0.05).
In Vitro Release
What makes MEs fundamental in the pharmaceutical field, apart from their high stability and ease of preparation, is their ability to considerably increase the bioavailability of sparingly water-soluble drugs and speed up the drug uptake by the living tissues as for loading process of a lipophilic drug into hydrophilic carriers. The release rates are determined by surfactant types and concentration.
As shown in , the in vitro release of NCTD from ME has a rapid releasing phase followed by a sustained releasing phase. The sustained release of NCTD may be due to using lecithin, which have a critical packing parameter, as an amphiphile for the ME preparation. Formation of lamellar phase or bilayers is thus favored (Cornell, Middlehurst, and Separovic Citation1986; Israelachvidi, Mitchell, and Ninham Citation1976). The liquid crystals have a highly ordered microstructure and increased viscosity. The liquid crystals formed on contact with the release medium prolonged the NCTD release remarkably. The release curve is in agreement with data concerning the diffusing of a drug within a liquid crystalline phase reported in the literature (Mueller-Goymann and Hamenn Citation1993; Trotta, Gallarate, and Gasco Citation1995). The characterization of the molecular arrangement of the drug and the lipid needs to be further investigated by nuclear magnetic resonance (NMR) and electron spin resonance (ESR) techniques.
Pharmacokinetics of NCTD in Plasma
The concentration-time course data of the plasma were analyzed by compartmental techniques and a two-compartment model provided better mathematical fit results (AIC = −35.011, r2 = 0.9940). Therefore, the two-compartment model was selected as the most appropriate one to describe the time course of NCTD in plasma.
The compartmental pharmacokinetic analysis is presented in . The pharmacokinetic parameters of NCTD in ME present differences from those of NCTD injection. The distribution and elimination phases of NCTD in ME were clearly differentiated from those of NCTD injection. With respect to the t1/2, the NCTD microemulsion presented a value of t1/2α 0.45 ± 0.08 hr, t1/2β 103.3 ± 12.4 hr which were much longer than those found for NCTD injection (t1/2α 0.27 ± 0.07 hr and t1/2β 41.77 ± 4.23 hr). Moreover, the plasma levels of NCTD microemulsion were significantly (p < 0.05) higher than those of NCTD injection after 20 min administration. The AUC of NCTD in microemulsion increased 3.2-fold compared with that of the NCTD injection (29.68 ± 0.86 vs 9.25 ± 0.09 mg·h·L−1). The mean retention time (MRT) of NCTD incorporated in the microemulsion increased 1.3-fold compared with that of NCTD injection (109.80 ± 11.23 vs 86.68 ± 0.8 hr). These results indicate that the removal of NCTD-loaded microemulsion from circulation is delayed by phospholipids-based microemulsions. The central distribution volumes and the plasma clearance (CL) of NCTD after intravenous administration of NCTD ME were approximately 9% and 2.6% of those after the intravenous administration of NCTD injection. These results suggest that MEs suppress both the distribution volume and the clearance of the model drug.
TABLE 1 Comparison of the blood pharmacokinetic parameters in mice between NCTD injection and NCTD-loaded microemulsion (mean) (n = 5, x ± s)
It is evident from the i.v. administration pharmacokinetic parameters of plasma shown in that the clearance rate of NCTD injection from blood (CL L·h−1) is much faster than that of the NCTD-loaded ME. There were significant differences of biodistribution and pharmacokinetics in mice between NCTD-loaded ME and NCTD injection (AUC or MRT, when α = 0.05, p < 0.01) Compared with NCTD injection, the plasma targeting efficiency of NCTD-loaded ME was improved, with enhanced plasma AUC of 29.68 ± 0.86 mg· h· L−1 and MRT of 109.80 ± 11.23 hr. The NCTD-loaded microemulsion had a circulating half-life that was approximately 2.62 times longer than that of the NCTD injection. Higher concentration and retention time of the drug in the bloodstream were in favor of eradication of the disease.
Tissue Distribution of NCTD
The pharmacokinetics parameters for NCTD ME and NCTD injection in tissues are displayed in and , respectively. The percentages of total administration dosage of NCTD in liver and kidney after a single dose of i.v.-injected NCTD injection and NCTD-loaded ME are displayed in . Among the tissues for i.v.-injected NCTD microemulsion, the liver presented the highest NCTD concentration (63.78 μ g/g of tissue), followed by the heart, the spleen, the kidneys, and finally the lungs. After i.v. administration of NCTD injection, the liver also presented the highest NCTD concentration. However, the kidneys presented a rather higher NCTD concentration (18.09 μ g/g of tissue), which is much higher than that of the NCTD-loaded microemulsion in kidneys. In addition, the kidneys showed a single sharp-peak pharmacokinetic profile for NCTD injection, but the microemulsion group did not present the phenomenon.
FIG. 3. Percentage of total administration dosage of NCTD-loaded microemulsion (ME) and NCTD injection in mice liver (a), kidney (b) following i.v. administration (5 mg/kg, NCTD).
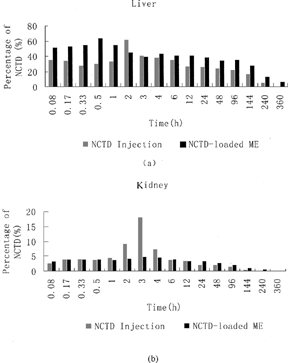
TABLE 2 Major pharmacokinetic parameters for NCTD-loaded microemulsion in mice
TABLE 3 Major pharmacokinetic parameters for NCTD injection in mice
For NCTD-loaded ME formulation, the tissue elution half-life varies among the tissues. The liver presented the longest t1/2 values (220.77 hr). The shortest tissue elution t1/2 was found in the kidneys (88.75 hr), followed by the lung (t1/2 = 89.14 hr). However, for NCTD injection, the longest elution t1/2 was found in the kidney (175.43 hr), while the shortest elution t1/2 was found in the liver (78.68 hr).
The calculated tissue uptake clearances of NCTD injection and NCTD-loaded ME after intravenous administration are shown in . The hepatic uptake clearance of NCTD-loaded ME was 6.7–8.6-fold higher than that of the kidney uptake clearance, while the hepatic uptake clearance of NCTD injection was 1.7–4.4-fold higher than that of the kidney uptake clearance. Thus, the tissue uptake clearance analysis also demonstrated that NCTD-loaded ME could modify the bioditribution of NCTD in vivo.
TABLE 4 AUC and tissue uptake clearances of NCTD injection and NCTD-loaded microemulsion after administration
Liver-Targeting Characteristics
shows the overall drug targeting efficiency (Te) of NCTD-loaded microemulsion and NCTD injection. The targeting index (Re) and the relative overall targeting efficiency (RTe), shown in , were calculated to evaluate targeting effect of NCTD-loaded microemulsion compared with NCTD injection. Te of liver was enhanced from 3.66% to 6.10%, leading to a prolonged t1/2 value in the liver from 78.68 to 220.77 hr. Re and RTe of liver were 3.55 and 0.67, respectively. It is known that higher concentrations and retention times of the drug in liver favors eradication of hepatic disease. In the mean-time, higher drug concentration in bloodstream and lower drug diffusion to other tissues ensured drug supply to liver.
TABLE 5 Overall targeting efficiency of NCTD-loaded microemulsion and NCTD injection (n = 5, x = ± s, %)
TABLE 6 Targeting evaluation of NCTD-loaded microemulsion compared with NCTD injection
showed that the kidney Te value of NCTD injection is two times higher than that of NCTD-loaded microemulsion group. This was caused by a higher distribution amount and lower elimination rate of NCTD in the kidney compared with NCTD-loaded microemulsion. It means NCTD injection may have higher toxicity than NCTD-loaded microemulsion to normal kidney cells.
showed that the values of Re and RTe in liver were much higher than those of other tissues (heart, spleen, lung, and kidney). The Re of liver (3.55) was higher than that of other tissues (no higher than 1.66), which means that NCTD-loaded ME was far less inclined to distribution to heart, lung, spleen, and kidney. Thus it had much lower toxicity to all these tissues.
CONCLUSION
Compared with NCTD injection, the better liver-targeting efficiency of NCTD-loaded microemulsion may mainly come from the enhanced drug concentration in bloodstream, improved liver uptake, and decreased drug diffusion to other tissues due to characteristics of ME vehicles. There may be other mechanisms, which contribute to the results, to be further explored. In short, the result of this study may present a potential method to promote the curative effect in hepatic cancer chemotherapy by using microemulsion as the vector of anticancer drugs.
This work was sponsored by the National Natural Fund for Distinguished Young Scholars (No. 39925039) of People's Republic of China.
REFERENCES
- Cornell B. A., Middlehurst J., Separovic F. Small unilamellar phospholipid vesicles and the theory of membrane formation. Faraday Disc. Chem. Soc. 1986; 81: 163–167, [CSA]
- Dong C. Study on distribution of 3H-norcantharidin in mice. J. Acad. Med. Jiangxi 1984; 24: 3–6, [CSA]
- Hao J. Effect of the structures of microemulsions on chemical reactions. Colloid Polym. Sci. 2000; 278: 150–154, [CROSSREF], [CSA]
- Israelachvidi J. N., Mitchell D. J., Ninham B. W. Theory of self-assembly of hydrocarbon amphilphiles into micelles and bilayers. J. Chem. Soc. Faraday Trans. 1976; 72: 1525–1568, [CROSSREF], [CSA]
- Kenji A., Tomohiro H. Effect of adding an amphiphilic solubilization improver, sucrose distearate, on the solubilization capacity of nonionic microemulsions. J. Colloid Interface Sci. 2001; 236: 14–19, [CROSSREF], [CSA]
- Mueller-Goymann C. C., Hamenn H. J. Sustained release from reverse micellar solutions by phase transformations into lamellar liquid crystal. J. Control. Release 1993; 23: 165–174, [CROSSREF], [CSA]
- Park K. M., Lee M. K., Hwang K. J., Kim C. K. Phospholipid-based microemulsions of flurbiprofen by the spontaneous emulsification process. Int. J. Pharm. 1999; 183: 145–154, [PUBMED], [INFOTRIEVE], [CROSSREF], [CSA]
- Shigeru K., Nao O., Ryu H. Biodistribution and pharmacokinetics of 0-palmitoyl tilisolol, a lipophilic prodrug of tilisolol, after intravenous administration in Rats. Biol. Pharm. Bull. 2002; 25(8)1072–1076, [CROSSREF], [CSA]
- Trotta M., Gallarate M., Gasco M. R. Effect of the variation of microemulsion water content on the in vitro permeation of hydrocortisone. Acta Tecnol. Legis Medicamenti. 1995; 6: 67–78, [CSA]
- Uif O., Kozo S. Change of the structure of microemulsions with the hydrophile-lipophile balance of nonconic surfactant as revealed by NMR self-diffusion studies. J. Phys. Chem. 1986; 90: 4083–4088, [CROSSREF], [CSA]
- Wakiyama N., Juni K., Nakano M. Preparation and evaluation in vitro of polylactic acid microspheres containing local anesthetics. Chem. Pharm. Bull. 1981; 29: 3363–3368, [PUBMED], [INFOTRIEVE], [CSA]
- Wang G. S. Hydrolyze of norcantharidin and subtracting methyla alleviates stimulation of renal system. J. Acta Pharmaceutica Sinica 1983; 7: 18–22, [CSA]
- Wang J. X., Sun X., Zhang Z. R. Enhanced brain targeting by synthesis of 3,5-dioctanoyl-5fluoro-2-deoxyuridine and incorporation into solid lipid nanoparticles. Eur. J. Pharm. Biopharm. 2002; 54(3)285–290, [PUBMED], [INFOTRIEVE], [CROSSREF], [CSA]
- Zhang L., Sun X., Xiang D., Zhang Z. R. Formulation and physicochemical characterization of norcantharidin microemulsion containing medium-chain length glycerides and phospholipid-based surfactants. J. Drug Del. Sci. Tech. 2004; 14(6)461–469, [CSA]