Abstract
Captopril granules of controlled release with different polymers as ethylcellulose, ethyl/methylcellulose, and immediate release with polyvinylpyrrolidone (PVP) were developed by fluid bed dryer technique. The formulations were analyzed by scanning electron microscopy, X-ray powder diffraction, and dissolution profiles. To compare the formulations an in vivo setting rat blood pressure assay was performed, using angiotensin I as a vasoconstrictor agent. The scanning electron microscopy of granules showed differences in morphology, and X-ray powder diffraction technique presented some modification in crystalline structure of captopril in granules coated with PVP and ethyl/methylcellulose. The dissolution profile of granules coated with ethylcellulose showed a median time release of 4 hr whereas for granules coated with ethyl/methylcellulose, this time was 3.5 hr. The blockage of angiotensin I-induced hypertensive effect lasted 8 hr in granules coated with PVP and of more than 12 hr in the granules coated with ethylcellulose and ethyl/methylcellulose.
The pharmacological and therapeutic benefits of controlled release forms for compounds with short half-lives have been reported in the literature (Rhodes and Portner Citation1998; Royce, Weaver, and Shah Citation2004). Captopril (2S-1-[3-mercapto-2-methylpropioniyl]-L-proline) is a highly selective competitive inhibitor of angiotensin converting enzyme (ACE), and used for the treatment of hypertension and heart failure. Given orally, captopril is rapidly absorbed and has a bioavailability of ∼75%. The duration of action after a single oral dose is 6-8 hr (Seta et al. Citation1988; Franklin et al. Citation1998). Due to its relative short half-life, a controlled released captopril formulation would bring benefits to patients, including reduction in frequency of administration, increased patient compliance and effectiveness of treatment, reduction in plasma concentration fluctuations, as well as a reduction in side effects (Pastrana et al. Citation2000; Agnihotri and Aminabhavi Citation2004). Some efforts targeting these goals have been published. These studies include the use of polymers for coated capsules and tablets and some pharmaceutical formulations like pellets, bioadhesive systems, hard gelatin capsules, pulsed-delivery systems, microcapsules, enteric-coated granules, floating tablets, and oily semisolid matrices. As captopril degrades in the presence of water or moisture, difficulties in either development or stability of these formulations have been reported (Singh and Robinson Citation1988; Matharu and Shangavi Citation1992; Wilding et al. Citation1992; Ho, Wang, and Sheu Citation1997; Saks and Gardner Citation1997; Efentakis and Vlachou Citation2000; Nur and Zhang Citation2000; Khattab et al. Citation2001).
Application of different coating materials onto multiparticles allows the improvement/obtention of several desirable functional properties such as resistance to acid, sustained release and targeted release. Fluid bed coating is a popular method of coating multiparticles due to its high throughput and excellent coating (Heng, Chan, and Tang Citation2006). Our group has described excellent results regarding extended captopril stability by the use of fluid bed coating in association with ethylcellulose and methylcellulose polymers (Stulzer and Silva Citation2006).Therefore, in the present report we describe a controlled release system for captopril granules coated with ethylcellulose and ethyl/methylcellulose using fluid bed dryer. In addition, we show that polyvinylpyrrolidine (PVP) can be used as a water-soluble polymer to produce granules of immediate release.
MATERIALS AND METHODS
Captopril was purchased from Shenyang Fine Chemical (China). Microcrystalline cellulose 101, ethylcellulose (Ethocel 10STD), methylcellulose (15 PR), monohydrate lactose, and PVP K30 were kindly provided by Colorcon (São Paulo, SP, Brazil). All other materials were of analytical grade.
Granule Formulations
The coated granules of captopril were produced at Colorcon Brazil in a fluid bed dryer (Narong model FBS-1). Single polymer or polymer mixtures for coating were diluted in ethanol 90%. Coating process was carried out in the following conditions: temperature inlet = 56°C; product = 38°C; air volume = 240 m3/h; atomizer pressure = 2.5 bar; and peristaltic pump flow = 4.5 g/ml. Following coating, granules were sifted through 20-mesh sieves. Granule formulations are presented in .
TABLE 1 Summary of granules composition of the different formulations
Scanning Electron Microscopy and X-Ray Powder Diffraction (XRPD)
Granule morphology was observed by scanning electron microscopy (Phillips XL30). Samples were mounted onto metal stubs using double-sided adhesive tape, vacuum-coated with gold (350 Å) in a Polaron E-5000, and analyzed. To characterize the influence of the coating process in captopril crystallinity, X-ray diffractions patterns were obtained on a Siemens X-ray diffractor, model D 5000, with tube of Cuk α, voltage of 40 kW, and current of 40 mA, in the range of 3-65 (2θ) and 1 s of pass-time, using the powder XRD method.
In Vitro Drug Release
United States Pharmacopea (USP) dissolution apparatus I (basket method) was used for release studies. A sample equivalent to 45 mg captopril was added to 900 mL phosphate buffer 0.05 N, pH 7.0 at 50 rpm. The temperature was maintained at 37 ± 0.5°C. For assessment of dissolution profiles, samples were removed at 1, 3, 5, 10, 15, and 20 min for granules of immediate release (coated with PVP) and until the release of 80% of the initial dosage for controlled release granules. Samples were centrifuged and analyzed in a UV spectrophotometer at 212 nm. The modifications of some parameters have been validated in accordance with the International Conference of Harmonization Q2A.
Theoretical Models for Drug Release
Drug release mechanism from microparticles was fitted to the following kinetic models: first order (Wagner Citation1969), zero order (Vudathala and Rogers Citation1995), Hixson-Crowell cubic root (Hixson and Crowell Citation1931), Weibull (Lagenbucher 1972), Korsmeyer-Peppas (Korsmeyer et al. Citation1983), Higuchi (Higuchi Citation1963), and Baker-Lonsdale (Baker and Lonsdale 1993). The best model to describe our results of captopril release from the microparticles was the Korsmeyer-Peppas model.
In Vivo Studies
Animals
Female Wistar rats (weighing 280–350 g) were housed in a temperature (23 ± 2°C) and light-(12 hr light/dark cycles) controlled room, with free access to water and food. All procedures were approved by our Institutional Ethics Committee and are in accordance with NIH Animal Care Guidelines.
Blood Pressure
Under anesthesia with ketamine and xylazine (90 and 15 mg/kg, respectively, supplemented at 45-to 60-min intervals), rats heparinized PE 20 and PE 50 polyethylene catheters were inserted, respectively, into the left femoral vein for drug injections and into the right carotid artery for recording mean arterial pressure (MAP), and blood withdrawal. To prevent clotting, a bolus dose of heparin (300 I.U.) was injected immediately after vein cannulation. Animals were allowed to breathe spontaneously via tracheal cannula. Body temperature was monitored by a rectal thermometer and maintained at 36°C ± 1°C. Drugs were diluted in sterile Dulbecco's phosphate-buffered saline (PBS, in millimoles: 137 NaCl, 2.7 KCl, 1.5 KH2 PO4, and 8.1 NaHPO4; pH 7.4). At the end of the experiment, animals were sacrificed with pentobarbitone overdose. Blood pressure data were recorded (at a 10-sec sampling rate) with a Digi-Med blood pressure analyzer system (model 190) connected to a Digi-Med system integrator (model 200; Louisville, KY, USA) software, running under Windows 98™ (Microsoft Corporation, Redmond, WA, USA). Results are expressed as means ± SEM of the peak changes, relative to baseline, in MAP (mmHg) recorded following administration of angiotensin I.
Experimental Protocol
Rats were randomly divided at 4 groups of 5 animals each. Group 1 received captopril granules coated with PVP; group 2 received captopril granules coated with ethylcellulose; group 3 received captopril granules coated with ethyl/methylcellulose (all at 10 mg/kg, p.o.) and group 4 (control) received sterile PBS (300 μ l). At different time points after treatment (0.5, 1, 4, 8, 16 hr), animals were instrumented for MAP recording. The vasoconstrictive response to angiotensin I (30 pmol/kg) was obtained.
RESULTS AND DISCUSSION
Scanning Electron Microscopy and X-Ray Powder Diffraction
Substances in solid state can present crystalline or amorphous characteristics, and in some cases both. The crystal has an ordered arrangement of molecules and atoms, maintained in contact through noncovalent interactions. However, amorphous solids are characterized by random state. These characteristics are important in the absorption process. Amorphous solids are, in general, more soluble than the crystalline form, due to free energies involved in the dissolution process. Solids in amorphous state have their molecules arranged randomly and thus low energy is required to separate them. Consequently, their dissolution is faster than when in the crystalline form (Krist et al. 1996; Storpirts et al. Citation1999; Florence and Attwood Citation2003). Captopril () has a crystalline aspect that presents in orthorhombic crystal form (Kaul, Nguyen, and Venkataram Citation1992; Giron Citation2003).
FIG. 1 Scanning electron microscopy of captopril (panels A and B, magnification × 200 and ×400, respectively).
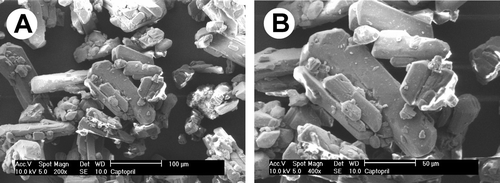
According to Markovich et al. (Citation1997), the solid-state form (i.e., crystalline polymorphs, solvates, and amorphous solids) of a given drug can have a significant impact on the solubility, dissolution rate, stability in a pharmaceutical formulation, and bioavailability. The influence of coating process by fluid bed dryer on the crystalline form of captopril was investigated using XRPD and the patterns are shown in . Granules coated with PVP and ethyl/methylcellulose presented a decrease of characteristic captopril peaks. This finding is of importance since it contributes to dissolution profiles of distinct granules formulations.
FIG. 2 X-ray diffraction spectra of captopril and granules coated with PVP, methylcellulose/ethylcellulose, and ethylcellulose.
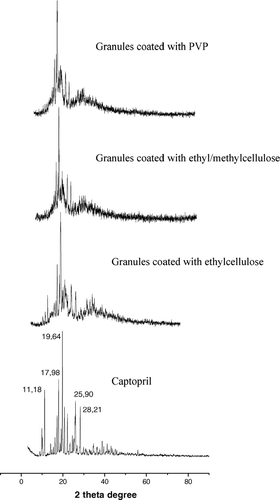
Reduction in particle size increases the surface area and accelerates the dissolution rate. Porous amorphous drug/excipients particles with high surface areas present higher dissolution rates (Wei and Thau Citation1996; Jinno et al. Citation2006; Purvis et al. Citation2006). Photomicrographs of granules () show that captopril coated with ethylcellulose yielded spherical granules with a relatively small surface area. Granules coated with ethyl/methylcellulose or with PVP resulted in irregular shaped granules with higher surface area.
FIG. 3 Scanning electron microscopy of captopril granules with different coatings. Coating of PVP (panels A and B, magnification × 50 and × 100); coating of ethyl/methylcellulose (panels C and D, magnification × 50 and × 100, respectively), and coating of ethylcellulose (panels E and F, magnification × 50 and ×100, respectively).
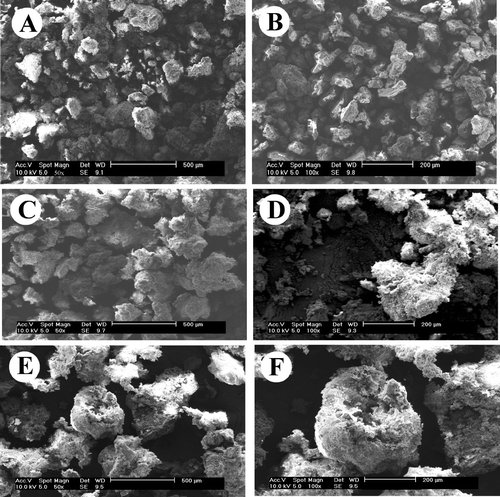
Drug Release
Release rates of the different captopril coating formulation are presented in . The results clearly indicate that the formulations had a differentiated pattern of release. Granules coated with ethylcellulose released captopril during 240 min and for granules coated with ethyl/methylcellulose, the time was 180 min. In contrast, granules coated with PVP released almost all captopril in 20 min. Thus, granules coated with celluloses had a longer release profile.
FIG. 4 Release profiles of granules coated with PVP, granules coated with ethylcellulose, and granules coated with ethyl/methylcellulose.
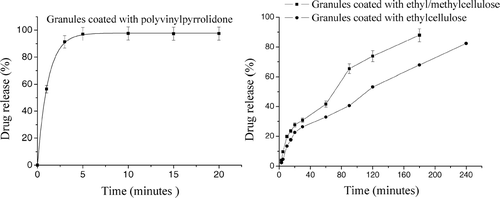
The mechanism of drug release from granules is determined by different physicochemical phenomena. According to Nixon (Citation1983), three steps lead to drug release from microparticles in aqueous medium: inhibition of the release medium into microparticles, dissolution of the drug inside microparticles, and drug release by a diffusion process into the aqueous medium. The Korsmeyer-Peppas model (equation 1), a semiempirical model correlating drug release to time by a simple exponential equation for the fraction of release drug < 0.6, has been used to evaluate drug release from controlled release polymeric devices. It is particularly useful when the drug release mechanism is unknown or when there is more than one release mechanism (Korsmeyer et al. Citation1983; Costa and Lobo Citation2001).
where Mt/M∞ is the proportion of drug released at time t, k is the kinetic constant, and the exponent n has been proposed as indicative of the release mechanism. In this context, n ≤ 0.43 indicates Fickian release and n = 0.85 indicates a purely relaxation controlled delivery that is referred to as case II transport. Intermediate values 0.43 < n < 0.85 indicate an anomalous behavior (non-Fickian kinetics corresponding to coupled diffusion/polymer relaxation; Ritger and Peppas 1987a, 1987b). Occasionally, values of n > 1 has been observed, which has been regarded as super case II kinetics (Ranga, Devi, and Buri Citation1988; Munday and Cox Citation2000). With the linear form of equation 1, plotting of ln Mt/M∞ against ln t () yielded the diffusion exponential (N), the Pearson coefficient (r2), and the diffusion constant (k). The results are summarized in .
FIG. 5 Linearity of different granules forms using equation 1.
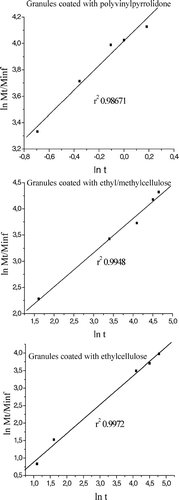
TABLE 2 Analysis of release data from captopril granules
Inspection of the results shown in indicates that granules coated with ethyl/methylcellulose presented an N value of 0.6712 indicative of anomalous behavior. This behavior probably reflects the polymer binary blend used, ethylcellulose presenting a hydrophobic and methylcellulose a hydrophilic behavior. The non-Fickian kinetics corresponds to the coupling of diffusion from ethylcelllulose and polymer relaxation from methylcellulose. However, granules coated only with ethylcellulose presented an N value of 0.4123, indicating that a Fickian release occurred only by diffusion process. The granules coated with PVP presented an immediate solubilization in dissolution medium. The k values were related with the kinetics of captopril release. These values were calculated from equation 2:
As shown in , the smaller k value for granules coated with ethylcellulose indicated a slower release, whereas for granules coated with ethyl/methylcellulose, k value was of 1.87.
In Vivo Studies
Angiotensin I is devoid of any significant vasoconstrictor activity. However, when given intravenously, angiotensin I is rapidly converted to angiotensin II by the action of angiotensin converting enzyme (ACE). Angiotensin II is a potent vasoconstrictor, whose potency is 40-fold higher than epinephrine in eliciting blood pressure increase (Ribeiro and Muscará Citation2001). ACE inhibitors are highly selective drugs that do not interact directly with other components of the renin-angiotensin system. Their pharmacological and clinical effects result for the blockade of the conversion of angiotensin I to angiotensin II. Captopril, the first ACE inhibitor to be marketed, is a potent ACE inhibitor (Regoli, Park, and Rioux Citation1974; Petrillo and Ondetti Citation1982; Romankiewicz et al. Citation1983).
As can be seen in and , 30 min after oral treatment with captopril granules coated with PVP, the increase in the blood pressure following angiotensin I injection was reduced by 80%. This result indicates an efficient inhibition of ACE by captopril. The inhibition carried out by captopril coated with PVP lasted for a period of 8 hr. Captopril granules coated with ethylcellulose and ethyl/methylcellulose retained their potency but the permanence of their inhibitory effects was increased by 50% (from 8 to > 12 hr).
FIG. 6 Effects of captopril granules coated with ethylcellulose and PVP on vasoconstrictive response to angiotensin I. Animals were treated orally with formulations or PBS (control). At indicated times animals were prepared for mean arterial pressure measurement. Thus, the vasoconstrictive response to angiontensin I (30 pmol/kg; panel A) and mean blood pressure (panel B) were recorded. Each point or bar represents the mean of 5 animals and vertical lines are the S.E.M. * p < 0.05 compared with the control group (PBS). Statistical analysis was performed using ANOVA test followed by Bonferroni's posthoc t test.
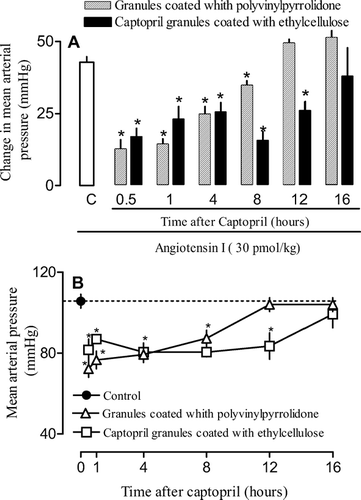
FIG. 7 Effects of captopril granules coated with ethyl/methylcellulose and PVP on vasoconstrictive response to angiotensin I. Animals were treated orally with formulations or PBS (control). At indicated times animals were prepared for mean arterial pressure measurement. Thus, the vasoconstrictive response to angiontensin I (30 pmol/kg; panel A) and mean blood pressure (panel B) were recorded. Each point or bar represents the mean of 5 animals and vertical lines are the S.E.M. * p < 0.05 compared with the control group (PBS). Statistical analysis was performed using ANOVA test followed by Bonferroni's posthoc t-test.
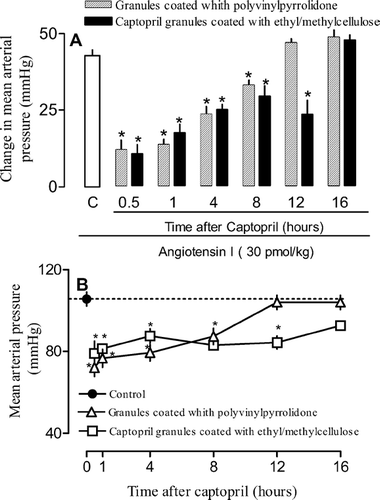
As for the effects on blood pressure, oral captopril granules coated with PVP administration induced a decrease of ∼30% below baseline levels within 30 min ( and ). The effect lingered for 8 hr and by 12 hr blood pressure had returned to baseline values. Coated granules with ethylcellulose and ethyl/methylcellulose displayed around the same time for the onset of action but their effect on blood pressure lasted 50% longer (8 to 12 hr; and ).
CONCLUSIONS
Our results show that captopril coated with PVP or as granules coated with ethyl/methylcellulose was rapidly absorbed after oral administration, with a peak of ACE inhibition about 30–60 min after administration. However, cellulose-coated granules provided a significant increase in the duration of the effect. This increase is enough to allow changing posology and hence to reduce the frequency of administrations. Captopril is largely used for chronic diseases such as hypertension and heart failure. Because these conditions usually require life-long treatment, this strategy is important to improve the quality of life and patient adherence, as well as to reduce the costs of the treatment.
We acknowledge the support of Coordenação de Aperfeiçoamento de Pessoal de Nível Superior (CAPES) for fellowships and Conselho Nacional de Desenvolvimento Científico and Tecnológico (CNPq) for the financial support.
REFERENCES
- Agnihotri S. A., Aminabhavi T. M. Controlled release of clozapine through chitosan microparticles prepared by a novel method. J. Control Rel. 2004; 96: 245–259
- Backer R. W., Lonsdale H. K. Controlled release: mechanism and rates. Controlled Release of Biological Active Agents, A. C. Tanquary, R. E. Lacey. Plenum Press, New York 1993
- Costa P., Lobo J. M. Modeling and comparison of dissolution profiles. Eur. J. Pharm. Sci. 2001; 13: 123–133
- Efentakis M., Vlachou M. Evaluation of high molecular weight poly (oxyethylene) (Polyox) polymer: studies of flow properties and release rates of furosemide and captopril from controlled-release hard gelatin capsules. Pharm. Devel. Technol. 2000; 5: 339–346
- Florence A. T., Attwood D. Princípios Físico-Químicos em Farmácia, 3rd ed. Editora da Universidade de São Paulo, São PauloBrazil 2003
- Franklin M. E., Addison R. S., Baker P. V., et al. Improved analytical procedure for the measurement of captopril in human plasma by gas chromatography mass spectrometry and its application to pharmacokinetic studies. J. Chromatogr. 1998; 705: 47–54
- Giron D. Characterization of salts of drug substances. J. Therm. Anal. Calorim. 2003; 73: 441–457
- Heng P. W. S., Chan L. W., Tang E. S. K. Use of swirling airflow to enhance coating performance of bottom spray fluid bed coaters. Int. J. Pharm. 2006; 327: 26–35
- Higuchi T. Mechanism of sustained-action medication: theoretical analysis of rate of release of solid dispersed in solid matrices. J. Pharm. Sc. 1963; 52: 1145–1149
- Hixson A. W., Crowell J. H. Dependence of reaction velocity upon surface and agitation. Ind. Eng. Chem. 1931; 23: 923–931
- Ho H. O., Wang H. Y., Sheu M. T. The evaluation of granulated excipients as matrix material for controlled delivery of captopril. J. Control. Rel. 1997; 49: 243–251
- Jinno J., Kamada N., Miyake M., et al. Effect of particle size reduction on dissolution and oral absorption of a poorly water-soluble drug, cilostazol, in beagle dogs. J. Control Rel. 2006; 111: 56–64
- Kaul D., Nguyen T. N., Venkataram S. Crystal habit modifications and altered tabletting characteristics. Int. J. Pharm. 1992; 88: 345–350
- Khattab I. S., Abdel-Rahman S., Khidr S., et al. Development and optimization of sustained-release captopril tablets and capsules. Egypt J. Biotechnol. 2001; 10: 32–445
- Korsmeyer R. W., Gurny R., Doelker E., et al. Mechanisms of solute release from porous hydrophilic polymers. Int. J. Pharm. 1983; 15: 25–35
- Kristl A., Srčič S., Vrečcer F., et al. Polymorphism and pseudopolymorphism: influencing the dissolution properties of guanine derivative acyclovir. Int. J. Pharm. Sci. 1996; 139: 231–235
- Langenbucher F. Linearization of dissolution rate curves by the Weibull distribution. J. Pharm. Pharmacol. 1972; 24: 979–981
- Markovich R. J., Evans C. A., Coscolluela C. B., et al. Spectroscopic identification of an amorphous-to-crystalline drug transition in a solid dispersion SCH 48461 capsule formulation. J. Pharm. Biomed. Anal. 1997; 16: 661–673
- Matharu R. S., Sanghavi N. M. Novel drug delivery system for captopril. Drug Dev. Ind. Pharm. 1992; 18: 1567–1574
- Munday D. L., Cox P. L. Compressed xanthan and karaya gum matrices hydration, erosion and drug release mechanisms. Int. J. Pharm. 2000; 203: 179–192
- Nixon J. R. Release characterization of microcapsules. Biomedical Applications of Microcapsulation, F. Lim. CRC Press, Boca Raton, FL 1983
- Nur A. O., Zhang J. S. Captopril floating and/or bioadhesive tablets: design and release kinetics. Drug Dev. Ind. Pharm. 2000; 26: 965–969
- Pastrana Y. C., Rivera A. B., Enrique T. E., et al. Caracterización farmacocinética de formulaciones orales de liberación sostenida. Factores que influyen en el perfil farmacocinético. Acta Farm. Bonaerense 2000; 19: 25–34
- Petrillo E. W., Ondetti M. A. Angiotensin-converting enzyme inhibitors: medicinal chemistry and biological actions. Med. Res. Rev. 1982; 2: 1–41
- Purvis T., Vaughn J. M., Rogers T. L., et al. Cryogenic liquids, nanoparticles, and microencapsulation. Int. J. Pharm. 2006; 324: 43–50
- Ranga R., Devi K. D., Buri P. Cellulose matrices for zero-order release of soluble drugs. Drug Dev. Ind. Pharm. 1988; 14: 2299–2320
- Regoli D., Park W. K., Rioux F. Pharmacology of angiotensin. Pharmacol Rev. 1974; 26: 69–123
- Ribeiro W., Muscará M. N. Características farmacocinéticas de antagonistas de cálcio, inibidores da ECA e antagonistas de angiotensina II em humanos. Rev Bras de Hipertens. 2001; 8: 114–124
- Ritger P. L., Peppas N. A. A simple equation for description of solute release. I. Fickian and non-Fickian release from non-swellable devices in the form of slabs, spheres, cylinders or discs. J. Control Rel. 1987a; 5: 23–36
- Ritger P. L., Peppas N. A. A simple equation for description of solute release. II. Fickian and anomalous release from swellable devices. J. Control Rel. 1987b; 5: 37–42
- Rhodes C. T., Porter S. C. Coatings for controlled-release drug delivery systems. Drug Dev. Ind. Pharm. 1998; 24: 1139–1154
- Romankiewicz J. A., Brogden R. N., Heel R. C., et al. Captopril: an update review of its pharmacological properties and therapeutic efficacy in congestive heart failure. Drugs 1983; 25: 6–40
- Royce A. L. S., Weaver M., Shah U. In vivo in vitro evaluation of three controlled release principles of 6-N-cyclohexyl-2′-O-methyladenosine. J. Control. Rel. 2004; 97: 79–90
- Saks S. R., Gardner L. B. The pharmacoeconomic value of controlled-release dosage forms. J. Control Rel. 1997; 48: 237–242
- Seta Y., Higuchi F., Kawahara Y., et al. Design and preparation of captopril sustained release and dosage forms and their biopharmaceutical properties. Int. J. Pharm. 1988; 41: 245–254
- Singh J., Robinson D. H. Controlled release captopril microcapsules: effect of non-ionic surfactants on release from ethyl cellulose microcapsules. J. Microencapsul. 1988; 5: 129–137
- Storpirts S., Oliveira P. G., Rodrigues D., et al. Considerações biofarmacêuticas relevantes na fabricação de medicamentos genéricos: fatores que afetam a dissolução e a absorção de fármacos. Rev. Bras. Cien. Farm. 1999; 35: 1–11
- Stulzer H. K., Silva M. A. S. Estudo de estabilidade de grânulos revestidos e comprimidos contendo captopril. Acta Farm. Bonaerense 2006; 25: 497–504
- Vudathala G. K., Rogers J. A. Dissolution of fludrocortisone from biphasic polymers hydrogels. J. Control. Rel. 1995; 34: 185–192
- Wagner J. G. Interpretation of percentage dissolved-time plots derived from in vitro testing of conventional tablets and capsules. J. Pharm. Sci. 1969; 58: 1253–1257
- Wei C., Thau M. Effect of particle size on the available surface area of nifedipine from nifedipine-polyethylene glycol 6000 solid dispersions. Int. J. Pharm. 1996; 127: 261–272
- Wilding I. R., Davis S. S., Bakhshaee M., et al. Gastrointestinal transit and systemic absorption of captopril from a pulsed-release formulation. Pharm. Res. 1992; 9: 654–657