Abstract
In an attempt to fabricate floating beads of ciprofloxacin, drugloaded alginate beads were prepared by simultaneous external and internal gelation. The effect of blending of alginate with gellan, hydroxypropyl methylcellulose, starch, and chitosan on the bead properties were evaluated. Beads were spherical with incorporation efficiency in the range of 52.81 ± 2.64 to 78.95 ± 1.92%. Beads exhibited buoyancy over a period of 7–24 hr based on the formulation variables. In vitro release of ciprofloxacin from the alginate beads in simulated gastric fluid (SGF) (0.1 N HCl, pH 1.2), was influenced significantly (p < 0.001) by the properties and concentration of additives. Among the polymers incorporated into alginate beads. Hydroxy propyl methylcellulose (HPMC) provided an extended release over 7 hr. The drug release predominately followed Higuchi's square root model.
Oral drug administration has received much attention because it is a most convenient and flexible route. During the past few years, there has been an increased effort to deliver the drugs to the site of action or region of maximum absorption. This is achieved partially by modification of drug delivery devices, which utilize polymers to control drug release. Although, polymers of various origin are used in drug delivery systems, there has been a progressive and divergent study on the application of natural polymers in oral controlled release formulations (CitationBonferoni and Rosi 1993; CitationSujja-areevath et al. 1996).
Sodium alginate, an anionic water-soluble natural polysaccharide, is extracted from marine brown algae. The dilute solutions of sodium alginate undergo gelation on addition to solution of metal cations by a cooperative process involving consecutive guluronic residues in the α-L-guluronic acid (G) blocks of the alginate chain (CitationMorris et al. 1973 and Citation1978; CitationGrant et al. 1973; CitationLiang et al. 1980). The reaction between the divalent metal ions (e.g., Ca2+) and guluronic acid moiety of alginic acid is stronger than that with monovalent sodium ions (Tang et al. 1995; CitationMiyazaki 1998, Citation1999) and this phenomenon has been utilized in the development of alginate beads for sustained release of drug.
The use of gas-generating agents, calcium carbonate and sodium bicarbonate, in floating single-unit systems is well reported. However, there is a limited attempt in developing multiparticulate systems employing gas-generating agents. Although CitationChoi et al. (2002), have prepared and evaluated the influence of gas-forming agent on bead characters, the drug release was for a short period (2 hr). The pharmacokinetics of a drug is altered by the slow drug release at the site of action or absorption window that improves therapeutic efficacy of the drug.
This study was envisaged with an aim to investigate the possible formulation approaches to sustain the drug release from alginate devices fabricated by simultaneous external and internal gelation. Ciprofloxacin, an antibiotic with activity against a wide spectrum of microbial organisms and with absorption window at the upper gastrointestinal tract, was used as a model drug for this study. Targeting of ciprofloxacin to stomach or small intestine helps in improving therapeutic effectiveness at lower administered dose (CitationDavis 2005).
MATERIALS AND METHODS
Ciprofloxacin was generously gifted by Dr Reddy's Laboratories, Hyderabad, India. Sodium alginate (viscosity of 2% w/v solution 250 cps at 25°C) was procured from Sigma Chemicals, St Louis, MO, USA. Chitosan (Mol. wt. 2 × 105 degree of deacetylation 82–85%) was a generous gift from Central Institute of Fisheries Technology, Cochin, India. Gellan gum (Kelcogel®) was procured from CpKelco Biopolymers, Chicago, IL, USA. HPMC K4M (viscosity 4000 cps) was procured from Colorcon Limited, Goa, India. Calcium carbonate was procured from Sd-Fine Chemicals, Mumbai, India. All other chemicals were of analytical grade and used as received.
Preparation of Floating Alginate Beads
Alginate beads. Beads were prepared according to the procedure described by CitationChoi et al. (2002) with minor modifications. Briefly, ciprofloxacin solution (1% w/v) was mixed with previously prepared alginate solution (2% w/v) with constant agitation for 2–3 hr on a magnetic stirrer (Remi Equipments, Mumbai, India). Gas-generating agent, calcium carbonate (1% w/w), was dispersed in the solution and mixing was continued till thorough dispersion was achieved. The resulting drug–polymer solution was added drop wise to the calcium chloride solution (2% w/v) in acetic acid (10% v/v) using a syringe fitted with a blunt-end needle (23 G, internal diameter 0.7 mm). The gelled beads were allowed to cure for 30 min. Beads were collected by filtration, washed with distilled water, and dried in a desiccator over fused calcium chloride at 25°C for 48 hr.
Alginate beads with additives. Chitosan, gellan gum, HPMC, and starch were incorporated to alginate beads to evaluate their influence on bead properties. Beads were prepared as described earlier with minor modifications. A solution of alginate-ciprofloxacin admixed with either chitosan (10–20% w/w), gellan gum (20–40% w/w), HPMC (20–60% w/w), or starch (10–20% w/w) was prepared in distilled water (). The polymer drug solution was added drop wise through a syringe fitted with a blunt-end needle (23 G, internal diameter 0.7 mm) to the calcium chloride solution (2% w/v) in acetic acid (10% v/v). After curing for 30 min, the beads were collected by filtration, washed with distilled water, and dried as described earlier.
TABLE 1 Composition variables of the fabricated alginate beads
Evaluation of the Fabricated Beads
Bead Size Determination
The particle size of the wet and dry beads was measured by using a microscope fitted with a calibrated eyepiece micrometer. The average size of 50 beads was measured. The readings are average of three determinations ± S.D.
Measurement of Density
Density of the beads was measured by immersing the beads in a 0.02% w/v Tween 80 solution for 3 days. Beads sunken after this process were used for density measurement carried out by the displacement method using n-hexane as a nonsolvent (n = 3 ± S.D.) (CitationSoppimath et al. 2001).
Compressibility Index
The compressibility index (% CI) of the prepared beads was determined by tapping method. A weighed quantity of the beads, after measuring the initial volume, was tapped in a 100 mL measuring cylinder on a hard surface at 100 taps per min until a constant volume was observed. The compressibility index was calculated according to the following equation:
where, V and V0 are the volumes of the beads after and before the standard tapping.
Encapsulation Efficiency
100 miligram of the beads were crushed to fine powder in a glass mortar. A weighed amount of the powder was transferred into a volumetric flask (100 mL) and about 25 mL of 0.1 N HCl was added. The drug was extracted into the solution with continuous shaking for 24 hr at 30°C. The volume was made up with distilled water. The solution was filtered (G-2 filter), an aliquot was diluted suitably, and the absorbance was measured on a double beam UV-Vis spectrophotometer (Jasco 7800, Jasco, Japan) at 276 nm against a suitable blank. The mean of three determinations (± S.D) was recorded.
Water Uptake Studies
Water uptake of the beads was determined by placing 100 mg of beads in 100 mL of simulated gastric fluid (0.1 N HCl; pH 1.2) using a wire mesh basket. The beads were collected at regular intervals of time, the excess of moisture was blotted off and weighed. The percentage weight change of the wet beads and the dry beads was noted using the following expression:
where, W1 and W0 are the weight of the beads at time “t” and dry state, respectively.
In Vitro Floating Characterization
The floating lag time and the duration of floating of the fabricated beads were determined in USP XXIII dissolution apparatus (Cintex Industrial Corporation, Mumbai, India). A known number of beads were placed on 900 mL of simulated gastric fluid (0.1 N HCl, pH 1.2) with 0.02% w/v Tween 80 to stimulate the surface tension of human gastric juice (Stithit et al. 1998; CitationHe et al. 1999). The paddle speed was maintained at 50 rpm, with temperature of the medium maintained at 37 ± 0.2°C. At pre-identified time intervals, the number of beads remaining buoyant was determined by visual inspection.
In Vitro Drug Release Studies
In vitro drug release studies were performed in a USP XXIII dissolution apparatus (Cintex Industrial Corporation, Mumbai, India) with paddle speed at 50 rpm. 900 mL of simulated gastric fluid (0.1 N HCl. pH 1.2) at 37 ± 0.2°C was used as the dissolution medium. At regular intervals of time, an aliquot was removed and replenished with fresh medium. The aliquot was assayed for ciprofloxacin content on a double beam UV-Vis spectrophotometer (Jasco 7800, Japan) at 276 nm against a suitable blank.
In vitro release data were fit to various equations to analyse the kinetics and mechanism of drug release from the beads. For elucidating kinetics of drug release, data were analyzed using: zero-order equation, Q = Q0 + Kt; first-order equation, Q = Q0 e−kt (CitationGibaldi and Feldman 1967; CitationWagner 1969): and Higuchi's square root model, Q = K (CitationHiguchi 1963). Where, Q is the amount of drug released in time “t”. Q0 is the initial amount of drug in dissolution medium, K is the release constant of respective equations, and t is the release time.
In order to understand the drug release mechanism from the beads, the data were fitted to Korsmeyer–Peppas' power equation (CitationKorsmeyer et al. 1983; CitationPeppas 1985):
where, Mt/M∞ is the fraction of drug released in time “t”, K is constant, and n represents the release exponent.
Statistical Analysis
All the data were analysed by student's t-test using Sigma Stat 2.0 (Jandel Scientific Corporation. USA) to determine the statistical difference in the results. All pairwise comparisons were made by one-way ANOVA followed by Tukey analysis. A probability value of p < 0.05 was considered statistically significant.
RESULTS AND DISCUSSION
An attempt was made by CitationChoi et al. (2002) to fabricate floating beads using gas-generating agents. Although the beads exhibited satisfactory physical characters, the beads were completely depleted of incorporated drug in ∼2 hr. In the present work, beads were fabricated by simultaneous internal and external gelation. Calcium chloride in coagulation fluid and calcium carbonate dispersed in alginate solution provided source of free divalent cation (Ca2+) and respectively caused gelation externally and internally. Spherical beads with smooth surface were obtained when calcium carbonate was incorporated as a source of cation internally. The wet beads were in the size range of 2.31 ± 0.35 to 2.70 ± 0.44 mm. Beads on drying lost the moisture and were of the size of 0.81 ± 0.13 to 1.10 ± 0.22 mm. The smallest size was exhibited by the control batch whereas the incorporation of additives increased the size of the bead markedly.
Buoyancy of the beads depends on their true density. The density of the beads was in the range of 0.8134 ± 0.047 to 1.001 ± 0.031 g/cm3 that was less than the expected density of gastric juice (∼1.004 g/cm3), indicating that fabricated beads would exhibit excellent buoyancy in vivo (CitationSoppimath et al. 2001). Starch-blended beads exhibited the highest density. 1.001 ± 0.031 g/cm3. The percent compressibility index (% Cl) was in the range of 9.00 ± 1.00 to 23.67 ± 2.51 indicating good flow characteristics of the beads. Lower compressibility index (9.0 ± 1.0) was exhibited by starch-blended alginate beads, suggesting that the sphericity is the highest for starch-blended beads in comparison to beads blended with other excipients. The entrapment of ciprofloxacin improved significantly (p < 0.05) with the incorporation of excipients to the alginate solution (). Since the beads are formed and allowed to cure in an aqueous medium, the coagulation fluid diffuses to the external phase taking a part of the dissolved drug causing drug loss. This phenomenon was reduced with added additives, which increase the viscosity of the polymeric solution that impede with diffusion of the dissolved drug minimizing the drug loss.
Water Uptake Studies
Beads without blended additives, exhibited a water uptake of 75.66 ± 1.96 at pH 1.2 (0.1 N HCl). On blending with additives, the beads showed a significant increase (p < 0.05) in the water uptake (). Among the blended excipients, gellan gum and chitosan exhibited similar water uptake profile and no significant difference was observed between the groups. However, blending HPMC at various concentrations exhibited a significant (p < 0.05) water uptake in comparison to other beads.
In Vitro Buoyancy Test
The fabricated beads showed excellent floating characters. As shown in , the lag time for the beads to be buoyant was less than 130 sec, with a few beads showing buoyancy of less than a minute. The beads once buoyant remained on top of the simulated gastric fluid (0.1 N HCl. pH 1.2 with 0.02% w/v Tween 80) for duration of 7–24 hr. Floating lag time and duration corroborated well with the density of the beads. Starch-blended beads exhibited a shorter duration of buoyancy (∼7 hr) in comparison to other beads that can be corroborated to the observed higher true density 1.001 ± 0.031 g/cm3. Additionally, starch, being hydrophilic, has a greater propensity to swell in aqueous media, which possibly caused leaching of starch from the swollen beads, which allowed escape of entrapped carbon dioxide responsible for imparting buoyancy to the beads, resulting in short buoyancy duration.
In Vitro Drug Release Studies
Ciprofloxacin release from the control beads was fast and the beads were completely depleted of drug within 2 hr. Ciprofloxacin is easily soluble at gastric pH. In the absence of a retarding material, the dissolution fluid entered to the core of the bead with ease that caused faster diffusion of the dissolved drug. Gellan gum, an anionic polysaccharide, when incorporated as a part (20 and 40% w/w with respect to sodium alginate), modulated drug release significantly (p < 0.001) (). Similar to sodium alginate, gellan gum undergoes gelation in presence of cations, which occurs via a chemical bonding between the divalent cations and two COO− groups of guluronic acid molecules in gellan chains (CitationKanesaka et al. 2004). It is evident from the that even at lower concentrations of gellan gum, the drug release was sustained for an extended period. Beads with 20% w/w of gellan released ∼97% drug in 4 hr, while the beads with 40% w/w of gellan released ∼91% of drug during the same period. Although definite reasons could not be assigned for this behaviour, there is evidence that the diffusion through gellan gum gel is of the same magnitude with that of sodium alginate even at a lower concentration range reflecting difference in gel structure of these gels (CitationKubo et al. 2003).
FIG. 2 Ciprofloxacin release from gellan gum and starch containing beads in comparison to control batch. **p < 0.001 vs. control batch; **p < 0.001 vs. control and starch-blended beads.
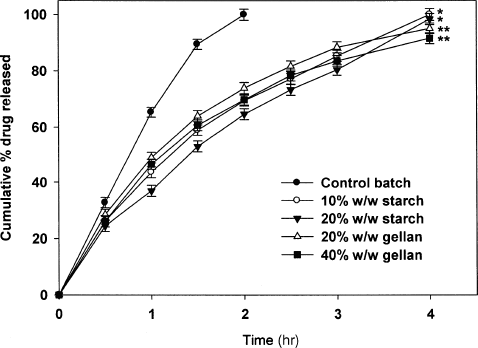
also shows the effect of blending of starch on the drug release. The drug release from the fabricated beads depends on the ingress of dissolution fluid and subsequent diffusion of dissolved drug from the bead matrix. Therefore, additives, which alter the matrix character, would modulate drug release. Starch, a hydrophilic additive, was incorporated at 10 and 20% w/w (with respect to alginate) affected the drug release significantly (p < 0.05). At these concentrations, starch act as a structural component (CitationKim et al. 2005), thereby forming a dense matrix, which alters the drug release. However, when the concentration of starch within the matrix was increased to 30% w/w, the beads disintegrated at a short duration (2–3 hr) of dissolution studies. This could be attributed to the high water uptake of the beads in presence of large concentration of starch. At relatively low concentrations of starch (10–20% w/w), the beads were intact even after dissolution study. It is likely that at these concentrations, the calcium alginate matrix was strong enough to withstand the swelling imparted by the presence of starch.
The release profile of drug from chitosan-blended beads is shown in . A nearly bi-phasic pattern of drug release from chitosan-blended beads was observed. In comparison to control beads (without chitosan blending), beads with chitosan in the matrix released the drug slowly. At the end of 5 hr, beads with 10 and 20% w/w chitosan released 98.42 ± 2.12 and 92.80 ± 1.93% of drug, respectively. which was a significant (p < 0.05) difference in comparison to control batch. These results indicate that increasing the concentration of chitosan in sodium alginate solution can effectively control the drug release from the beads. Chitosan is a cationic polymer with glucosamine side chains. The NH4 group on chitosan is protonated in acidic environment, which makes chitosan to swell in acidic dissolution media (0.1 N HCl, pH 1.2). The swelling of chitosan, within alginate beads, leads to the formation of dense matrix structure, thereby reducing the drug diffusion. Further, it might be possible that the protonated amine groups on chitosan reacted with the carboxylic group of alginate forming an effective barrier to limit the drug diffusion (CitationAral and Akbuga 1998). In a previous study, a linear release of incorporated drug (Felodipine) was observed from chitosan-blended alginate microspheres in phosphate buffer saline (pH 7.4) (CitationLee et al. 2003).
FIG. 3 Effect of HPMC and chitosan blending on drug release from floating alginate beads. *p < 0.001 vs. control batch: **p < 0.001 vs. control and chitosan-blended beads.
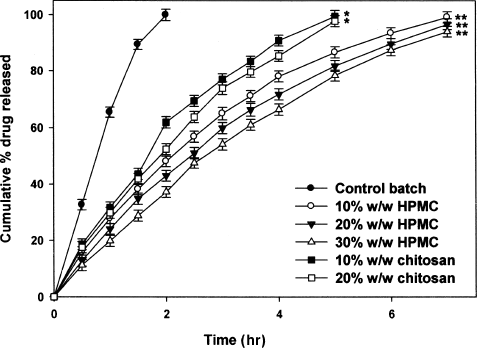
HPMC is a swellable polymer effective in controlling drug release (CitationGursoy et al. 1998; CitationSung et al. 1996). Based on the nature of HPMC, it is expected that its incorporation in the alginate beads provides sustained release of ciprofloxacin. As shown in . HPMC-blended beads showed a linear release profile. The drug release was extended to > 7 hr and was influenced by the concentration of HPMC. An increase in the concentration of HPMC slowed the ciprofloxacin release significantly (p < 0.05). Beads with 10% w/w HPMC released 99.14 ± 1.93% of drug in 7 hr. whereas 96.73 ± 1.93 and 94.07 ± 1.91% of drug was released respectively from beads with 20 and 30% w/w of HPMC. The findings are in line with an earlier report that HPMC polymers affect the drug release of alginate microspheres (CitationChan et al. 1997). The observed sustained release of drug from the fabricated alginate beads could be due to two reasons: partially coiled HPMC chains interpenetrate with sodium alginate network (CitationChan et al. 1997) and the swelling of HPMC caused formation of viscous gelatinous layer that controls the drug release (CitationConti et al. 2007).
Mechanism of Ciprofloxacin Release
The correlation coefficient (r2) values for various release models viz., zero-order, first-order, and Higuchi models, are shown in . The r2 values suggest that the drug release from fabricated beads predominately followed Higuchi's square root of time kinetics, as the values for Q vs. t1,2 (0.9763–0.9982) was always higher than that for Q, vs, t (0.8927–0.9880) and log (100–Q) vs. t (0.9183–0.9890). This was an exception to batch BH3, which exhibited a higher correlation coefficient for Q vs. t (0.9880) than that for other equations, implying the drug release was by zero-order kinetics. Release exponent, n, for all the batches was > 0.5 but < 1, indicating the release was governed by both diffusion and polymer relaxation (CitationPeppas 1985). The release of ciprofloxacin from the beads was slow and steady; which is evident from the respective t50% and t75% values. Among the four blended beads. HPMC blending provided excellent sustained release of ciprofloxacin with adequate buoyancy.
TABLE 2 Mechanism of ciprofloxacin release from the fabricated alginate beads
CONCLUSION
Very limited work has been reported on the use of gas-generating agents in the development of buoyant multiple-unit systems. However, the earlier reported work suggests that the beads were depleted of loaded drug in a short time reducing the therapeutic efficacy of the formulations. The present work was envisaged to test the effect of excipients blending on bead characters prepared by both internal and external gelation. The results suggest that it is possible to achieve slow release of the loaded drug from the beads by increasing the viscosity of the matrix within the bead by blending with excipients. Further studies are required to optimize the formulations where by a better therapeutic regimen is achieved with reduced dose of administered drug.
REFERENCES
- C. Aral, and J. Akbuga. (1998). Alternative approach to the preparation of chitosan beads. Int. J. Pharm. 168:9–15.
- M. C. Bonferoni, and S. T. Rosi. (1993). On the employment of λ-carrageenan in a matrix system. I: sensitivity to dissolution medium and comparison with Na carboxymethylcellulose and xanthan gum. J. Controll Rel. 26:119–127.
- L. W. Chan, P. W.S. Heng, and L. S. C. Wan. (1997). Effect of cellulose derivatives on alginate microspheres prepared by emulsification. J. Microencapsul. 14:545–555.
- B. Y. Choi, H. J. Park, S. J. Hwang, and J. B. Park. (2002). Preparation of alginate beads for floating drug delivery system: effect of CO2 gas-generating agents. Int. J. Pharm. 239:81–91.
- S. Conti, L. Maggi, L. Segale, E. O. Machiste, U. Conte, P Grenier, and G. Vergnault. (2007). Matrices containing NaCMC and HPMC: I. Dissolution performance characterization. Int. J. Pharm. 333:136–142.
- S. S. Davis. (2005). Formulation strategies for absorption windows. Drug Discov. Today 10:249–257.
- M. Gibaldi, and S. Feldman. (1967). Establishment of sink conditions in dissolution rate determinations. Theoretical considerations and application to non-disintegrating dosage forms. J. Pharm. Sci. 56:1238–1242.
- G. T. Grant, E. R. Morris, D. A. Rees, P. J. C. Smith, and D. Thom. (1973). Biological interactions between polysaccharides and divalent cations: the egg box model. FEBS Lett. 32:195–198.
- A. Gursoy, F. Kalkan, and I. Okar. (1998). Preparation and tabletting of dipyridamole alginate-Eudragit microspheres. J. Microencapsul. 15:621–628.
- P. He, S. S. Davis, and L. Illum. (1999). Sustained release chitosan microspheres prepared by novel spray drying methods. J. Microencapsul. 16:343–355.
- T. Higuchi. (1963). Mechanism of sustained action medication: theoretical analysis of rate of release of solid drugs dispersed in solid matrices. J. Pharm. Sci. 52:1145–1149.
- S. Kanesaka, T. Watanabe, and S. Matsukawa. (2004). Binding effect of Cu2+ as a trigger on the sol-to-gel and the coil-to-helix transition processes of polysaccharide, gellan gum. Biomacromolec. 5:863–868.
- Y. J. Kim, H. G. Park, Y. L. Yang, Y. Yoon, S. Kim, and E. Oh. (2005). Multifunctional drug delivery system using starch-alginate beads for controlled release. Biol. Pharm. Bull. 28:394–397.
- R. W. Korsmeyer, R. Gurny, E. M. Doelker, P. Buri, and N. A. Peppas. (1983). Mechanisms of solute release from porous hydrophilic polymers. Int. J. Pharm. 15:25–35.
- W. Kubo, S. Miyazaki, and D. Attwood. (2003). Oral sustained delivery of paracetamol from in situ gelling gellan and sodium alginate formulations. Int. J. Pharm. 258:55–64.
- D. W. Lee, S. J. Hwang, J. B. Park, and H. J. Park. (2003). Microspheres of alginate-chitosan containing isoniazid. J. Microencapsul. 20:179–192.
- J. N. Liang, E. S. Stevens, S. A. Frangou, E. R. Morris, and D. A. Rees. (1980). Cation-specific vacuum ultraviolet circular dichronism behavior of alginate solutions, gels and solid films. Int. J. Biol. Macromol. 2:204–208.
- S. Miyazaki, H. Aoyama, N. Kawasaki, W. Kubu, and D. Attwood. (1999). In situ gelling gellan formulations as vehicles for oral delivery. J. Control. Rel. 60:287–295.
- S. Miyazaki, F. Suisha, M. Kawasaki, K. Shirakawa, K. Yamatoya, and D. Attwood. (1998). Thermally reversible xyloglucan gels as vehicles for rectal drug delivery. J. Control. Rel. 56:75–83.
- E. R. Morris, D. A. Rees, and D. Thom. (1973). Characterisation of polysaccharide structure and interaction by circular dichronism: order-disorder transition in the calcium alginate system. J. Chem. Soc. Commun. 245–246.
- E. R. Morris, D. A. Rees, and D. Thom. (1978). Chiroptical and stoichiometric evidence of a specific, primary dimerisation process in alginate gelation. Carbohydr Res. 66:145–154.
- N. A. Peppas. (1985). Analysis of Fickian and non-Fickian drug release polymers. Pharm. Acta Helv. 60:110–111.
- K. S. Soppimath, A. R. Kulkarni, and T. M. Aminbhavi. (2001). Development of hollow microspheres as floating controlled-release systems for cardiovascular drugs: preparation and release characteristics. Drug Dev. Ind. Pharm. 27:507–515.
- S. Stitht, W. Chen, and J. C. Price. (1998). Development and characterization of buoyant theophylline microspheres with near zero-order release kinetics. J. Microencapsul. 15:725–737.
- J. Sujja-areevath, D. L. Munday, P. J. Cox, and K. A. Khan. (1996). Release characteristics of diclofenac sodium from encapsulated natural gum mini-matrix formulations. Int. J. Pharm. 139:53–62.
- K. C. Sung, P. R. Nixon, J. W. Skoug, T. R. Ju, P. Gao, E. M. Topp, and M. V. Patel. (1996). Effect of formulation variables on drug and polymer release from HPMC-based matrix tablets. Int. J. Pharm. 142:53–60.
- J. Tang, M. A. Tung, and Y. Zeng. (1996). Compression strength and deformation of gellan gels formed with mono- and divalent cations. Carbohydr. Polym. 29:11–16.
- J. G. Wagner. (1969). Interpretation of percent dissolved--time plots derived from in vitro testing of conventional tablets and capsules. J. Pharm. Sci. 58:1253–1257.