Abstract
Ring-opening terpolymerization of L-lactide (LA), ϵ-caprolactone (CL), and glycolide (GA) was performed in the presence of tin (II) 2-ethylhexanoate at 170°C. Random terpolyesters with weight-average molecular weight up to 130,000 g/mol were obtained. These terpolyesters, especially those with LA:CL:GA composition of 3:1:1, provided good coating integrity following spraying onto bare metal stents. The semi-synthetic macrolide immunosuppressant, everolimus, was incorporated into the terpolyester coating, and its release from the stent was evaluated. Unlike PLLA homopolymers, which are immiscible with the drug and non-optimal for controlled release, these terpolymers gave excellent control in a screening study, by tuning terpolymer molecular weight, relative monomer ratio, and drug-to-polymer ratio. Adjusting the polymer properties to improve drug solubility (or miscibility) in the polymer coating was found beneficial to the release profile.
Keywords::
Introduction
Aliphatic polyesters synthesized by ring-opening polymerization of lactones and lactides are useful in pharmaceutical and biomedical applications, including for example surgical sutures and drug delivery systems (CitationGreenwald et al., 1994; CitationLanger, 2000; CitationAlbertsson & Varma, 2003; CitationNasongkla et al., 2004). Poly(L-lactide) (PLLA) is one of the most widely studied polymer biomaterials, attractive for its biodegradable and biocompatible properties. However, PLLA is not ideally suited for many aspects of drug delivery, including those involving drug-eluting stents. The degradation rate of PLLA is difficult to control, often showing burst or shut-down features (CitationMiyajima et al., 1999; CitationCai et al., 2003), due in part to the high crystallinity and hydrophobicity of the polymer backbone. Other polymer structures as drug eluting matrices, such as polyurethanes, present similar difficulties (CitationLyu et al., 2005). The copolymerization of L-lactide with ε-caprolactone and glycolide allows tuning of the composition of the polymer to improve drug miscibility in the PLLA-based polymer matrix and the controlled release of the drug. For example, embedding ε-caprolactone character into PLLA improves drug solubility, decreases crystallinity of the polymer, and lowers Tg, such that drug release becomes more predictable and permeation controlled. On the other hand, integration of glycolide into the backbone increases hydrophilicity of the polymer, and accelerates release by polymer degradation over time.
In general, aliphatic polyesters prepared from L-lactide, ε-caprolactone, and glycolide are intended for biomaterials applications, as such polymers are generally biocompatible and biodegradable in vivo. Copolymerizing two or three of these monomers in controlled ratios allows for a tailoring of polymer properties towards a desired application. For example, varying the monomer molar ratio in the copolymers affords control over parameters such as hydrolysis rate, hydrophobicity, glass transition temperature (Tg), and elasticity. Aliphatic polyester copolymers (both random and block copolymer structures) have been prepared from various combinations of cyclic esters, including for example L-lactide/glycolide (CitationGilding & Reed, 1979; CitationReed & Gilding, 1981; CitationKricheldorf, Jonte, & Berl, 1985; CitationKasperczyk, 1996; CitationDobrzynski, Kasperczyk, & Bero, 1999; CitationDechy-Cabaret, Martin-Vaca, & Bourissou, 2004), L-lactide/ε-caprolactone (CitationKricheldorf et al., 1985; CitationChoi, Park, & Chang, 1994; CitationTomihata et al., 1998; CitationJeong et al., 2004), and glycolide/ε-caprolactone (CitationKricheldorf et al., 1985; CitationDobrzynski, Kasperczyk, & Bero, 1999; CitationKricheldorf, Mang, & Jonte, 1984; CitationBezwada et al., 1995; CitationLee et al., 2003). Terpolymers composed of all three monomers have received comparatively little attention. CitationFunae et al. (2000) reported the synthesis of a polyester copolymer consisting first of a lactide/ε-caprolactone random copolymer block prepared at 180°C in the presence of tin(II) 2-ethylhexanoate (Sn(oct)2). A second block of glycolide, ranging from 95 to 50 mole percent of the structure, was then added by chain extension at 215°C. In another example, CitationSrisa-ard et al. (2001) described a random copolymer of L-lactide (LA), ε-caprolactone (CL) and glycolide (GA) by Sn(oct)2 initiation at 130°C. The resulting terpolymer had a LA:CL:GA composition of 74:15:11, with a random chain microstructure, and a molecular weight of ~ 10,000 g/mole. Finally, CitationDobrzynski (2002) described the synthesis of LA:CL:GA terpolymers, initiated by zirconium(IV) acetylacetonate, having molecular weight up to 65,000 g/mole.
Biodegradable polyesters are good candidates for coating drug-eluting stents, as the polymer coating serves as an interface between the bare metal stent and the vascular tissue. The drug is loaded into the coating, which functions as the reservoir of a controlled delivery system. The primary mechanisms governing drug release kinetics are (CitationAlbertsson & Varma, 2003) drug diffusion through the polymer matrix into the surrounding environment, and (CitationGreenwald et al., 1994) drug release aided by degradation of the surrounding polymer matrix (CitationAcharya & Park, 2006). In diffusion-controlled release, a first-order release profile can be expected, whereas the case of degradation will lead to an expected zero-order release profile. Poly(lactide-co-glycolide) (PLGA) films have been used in release studies of paclitaxel and sirolimus. CitationWang et al., (2006) evaluated the release of sirolimus from a multilayered film, in which a solution of PLGA copolymer, consisting of 53% lactide and 47% glycolide, and drug was cast over a poly(L-lactide) film. Sirolimus release occurred in two steps: an initial phase, up to 16 days, showed the release of 10% of sirolimus with first-order kinetics, followed by an increased release rate due to polymer degradation. Similarly, CitationLao and Venkatraman (2008a) demonstrated that paclitaxel in the same multilayered film underwent very slow release (less than 5%) by diffusion in the first 28 days, accelerating quickly in the later time-frame to release up to 90% of the loaded drug. More recently, CitationLao and Venkatraman (2008b) studied several polyester release matrices for paclitaxel, including pure 53:47 PLGA, and 53:47 PLGA blended with 10 wt.% poly(ethylene glycol) (PEG) and pure polycaprolactone. For the PLGA film, an induction period characterized by a very slow release (5% drug in 25 days) was seen, followed by accelerated release (up to 80 days) as the polyester degraded. The presence of PEG led to a shorter induction period (12 days), due to water adsorption, and paclitaxel was released completely in 45 days. In the case of a poly-ε-caprolactone film, an initial burst release of up to 55% paclitaxel was seen in the first day, followed by diffusion-controlled release up to drug exhaustion at day 30.
Here we describe the preparation of terpolymer coatings that serve as matrices for everolimus release, in which the terpolymer consists of L-lactide, ε-caprolactone and glycolide, prepared by polymerization at 170°C in the presence of the catalyst Sn(oct)2. High molecular weight terpolyesters were desired to optimize their performance in their dual roles of (CitationAlbertsson & Varma, 2003) robust coatings with excellent adhesion to the underlying substrate, and (CitationGreenwald et al., 1994) reservoirs to control drug release. While the preparation of such high molecular weight aliphatic polyester terpolymers has received only limited attention in the literature (CitationDobrzynski, 2002), we find that such polymers satisfy many of the requirements associated with substrate coating and small molecule release. Ultimately, we wish to achieve permeation release control by matching the hydrophobicity and phase compatibility of the polymer with the drug, which can be afforded by finding the optimal composition in comonomers, such as the terpolyester used here. Systems which experience polymer/drug phase separation are non-ideal, as these exhibit a drug burst, followed by shutdown when the drug concentration is below percolation (until hydrolysis or enzymolysis allows further drug release) (CitationLyu et al., 2005). Above percolation, drug burst is followed by a porous release mechanism, which is difficult to reproduce.
Materials and methods
Materials
L-lactide (LA, 98%), ε-caprolactone (CL, 99%), tin(II) 2-ethylhexanoate (Sn(oct)2, 95%), and 1,6-hexanediol (97%) were purchased from Aldrich Milwankee, WI. Glycolide (GA, 99.9%) was purchased from Polysciences, Inc Warrington, PA. L-lactide and glycolide were recrystallized from dry ethyl acetate, then dried under vacuum at room temperature. For glycolide, the recrystallization was performed twice. ε-Caprolactone and 1,6-hexanediol were distilled over calcium hydride, and stored under nitrogen. L-lactide, glycolide, and 1,6-hexanediol were lyophilized before use.
Characterization
1H and 13C NMR spectra were recorded on a Bruker Avance400 spectrometer. Polymer molecular weights and polydispersity indices (Mw/Mn) were estimated by gel permeation chromatography (GPC) equipped with a KNAUER HPLC Pump K-501, three PLgel 5 μm MIXED-D 300 × 7.5 mm columns, and an RI detector K-2301. Polystyrene standards were used for calibration. THF was used as the eluent at a flow rate of 1 mL/min. Differential scanning calorimetry (DSC) measurements were performed on a DuPont Instruments DSC 2910 at a scan rate of 10°C/min under a flow of nitrogen (50 mL/min).
Representative synthesis of poly(L-lactide-co-ε-caprolactone-co-glycolide)
L-lactide (4.88 g, 33.8 mmol), ε-caprolactone (1.0 mL, 9.0 mmol), and glycolide (0.26 g, 2.3 mmol) were introduced into a flame-dried Schlenk tube under a stream of nitrogen. Tin(II) 2-ethylhexanoate and 1,6-hexanediol were added, and the Schlenk tube was placed in an oil bath at 70°C under vacuum for 15 min and purged by a short release under nitrogen. This action was repeated three times, after which the Schlenk tube was closed under vacuum. The oil bath temperature was then increased to 170°C, and the mixture was stirred until high conversion was reached (as noted by solidification of the reaction mixture). Following cooling to room temperature, the mixture was dissolved in chloroform and precipitated twice into methanol. The polymer was isolated by filtration, then dried under vacuum at room temperature overnight. The composition of the final polymer (relative ratio of incorporated monomers) was determined by 1H NMR spectroscopy in CDCl3. A typical yield of purified polymer was in the range of 60–70%.
Coating and release studies
The polymer and polymer/drug solutions were typically prepared from dilute solution (~ 1 weight%) of polymer or polymer/drug in 9:1 acetone:methyl isobutyl ketone (MIBK). Two coatings were then applied to the stents, first a polymer primer coating, then a polymer/drug coating. In the primer coating, a polymer solution was applied to form a coating of approximately 1 micron thickness. For the drug coating layer, a polymer/drug solution was first prepared, by addition of everolimus to the fully dissolved polymer, to give drug:polymer weight ratios of 1:1, 1:2, and 1:3. The stents used in this study were Abbott MULTILINK VISION® 12 mm stents, with OD 3.0 mm, and total surface area 0.56 cm2. The coating was carried out using an Abbott in-house spray-coater. A set drug dose of 100 μg/cm2 was applied in the drug layer. The stents were then crimped onto Vision™ catheters. The coated stents were baked at 50°C for 2 h, then subjected to electron-beam sterilization at 25 kGy. The stent on the catheter was delivered through a simulated use model to mimic the tortuosity of the coronary vessel, followed by expansion by immersion into water at 37°C. The coating integrity was examined by scanning electron microscopy (SEM).
Drug release studies were performed on a type 7 USP apparatus, using porcine serum as elution media at 37°C. Sodium azide was added to the media to prevent microbial growth. USP apparatus 7 consists of a set of solution containers immersed in a water bath at a constant temperature, sample holders, and a drive assembly reciprocating the system vertically. At least three stents for each of the terpolymers were analyzed for drug release on days 1 and 3. Due to rapid everolimus degradation in the elution media, the amount of drug released at each time point was calculated based on the difference between the theoretical value (estimated from the coating weight for each individual stent just after stent coating) and that remaining on the stent. Residual drug on each stent was extracted in 5–10 mL of acetonitrile/0.02% BHT solution and sonicated for 30 min, and the resulting solutions were analyzed by High Pressure Liquid Chromatography (Waters HPLC) to quantify the amount of drug left on the stent. The HPLC system consisted of a pump, column heater, temperature-controlled autosampler, and Photodiode Array Detector (PDA) or UV detector. A 4.6 × 150 mm YMC C18 column was used, with 3 μm particle size. The mobile phase was 4:1 acetonitrile:ammonium acetate buffer (0.02 M), column temperature 50°C, and autosampler temperature 5°C. The flow rate was 1.0 mL/min, and the detection wavelength 277 nm. Quantification of drug left on the stent at each time point gave the percent of initial everolimus released from each stent.
Results and discussion
Terpolymer synthesis
Bulk copolymerizations of LA, CL, and GA were performed at 170°C in the presence of Sn(oct)2. Performing the copolymerizations at this temperature leads to a greater degree of randomness in the polymer structure relative to lower temperature polymerizations. Incomplete incorporation of ε-caprolactone into the polymer chains is observed when copolymerizing glycolide and ε-caprolactone at temperatures below 150°C, due to the lower reactivity of ε-caprolactone, as noted by Lee et al. (Citation2003) The need for high molecular weight polyesters stems from their intended dual function as stent coatings and also as barriers to control diffusion of the drugs as small molecule additives. The random distribution of ε-caprolactone and glycolide comonomers along the polylactide backbone is desired from the standpoint of reducing the otherwise high crystallinity and brittleness of polylactide, or copolymers in which polylactide dominates physical properties.
LA:CL:GA terpolymers were prepared in the presence of trace amounts of Sn(II) catalyst, and the aliphatic diol initiator 1,6-hexanediol (some polymerizations were performed in the absence of added initiator). The reaction mixture was first heated at 70°C under vacuum for 1 h, after which the temperature was increased to 170°C to perform the polymerization. By a reaction time of 10 h, the polymerization mixture solidified. The solid polymers obtained were dissolved in chloroform, precipitated into MeOH, and dried at room temperature under vacuum for several hours to give the desired polymer, typically as a white or off-white solid. As shown in , the LA:CL:GA polymerizations were run with feed ratios of 60:25:15, 65:20:15 and 75:20:5. Gel permeation chromatography of the terpolymer samples, eluting in THF at 1 mL/min and estimating molecular weight against polystyrene standards, provided number- and weight-average molecular weights, and polydispersity indices (PDIs), of the samples.
Table 1. Poly(L-lactide-co-ε-caprolactone-co-glycolide) copolymers prepared.
In the 1H NMR spectra of the terpolymers, characteristic baseline separated resonances for each monomer type were noted, and the integration values of these resonances gave the relative ratio of monomers in each polymer sample. As expected, the presence of different monomer sequences in the terpolymers results in the presence of multiple resonances in the spectra. The methylene protons of the glycolide units (Hh) appeared as a multiplet centered at 4.7 ppm, while those from the ε-caprolactone units adjacent to the ester appear at 4.1 (Hg) and 2.4 (Hc) ppm. The methine protons of the lactide units (Hb) appear at 5.15 ppm. Integration of these resonances gives monomer composition in the terpolymer, which is seen to agree reasonably well with the monomer feed ratio, as indicated in .
Monomer sequence in these terpolymer structures is evaluated by 13C NMR spectroscopy (). For example, the carbonyl region is sensitive to polymer microstructure. Carbonyl resonances for glycolide, L-lactide, and ε-caprolactone in the corresponding homopolymers are seen at 166, 169 and 173 ppm (10). As observed in the 13C NMR spectrum of , the presence of multiple additional resonances, arising from mixed triad sequences, indicates that ε-caprolactone and glycolide are distributed along the terpolymer chains rather that forming distinct blocks for this (and the other) terpolymers prepared for this study. Furthermore, the characteristic peak for the CL-CL-CL triad at 173.5 ppm was not observed. Given the higher percentage of lactide monomer used, the intense carbonyl resonance at ~ 169 ppm was expected.
Figure 1. (a) 1H NMR and (b) 13C NMR spectra of poly(L-lactide-co-ε-caprolactone-co-glycolide) terpolymer (, entry 5).
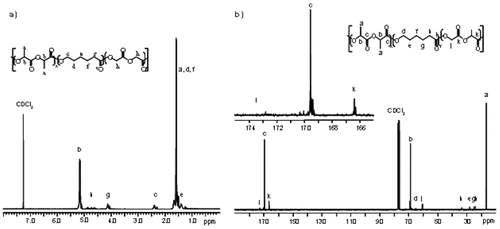
Thermal properties of these LA:CL:GA terpolymers were determined by differential scanning calorimetry (DSC). Glass transition temperature (Tg) values, shown for example in for terpolymer 5, were compared to estimated Tg values based on monomer composition using the Fox equation:
where wLA, wCL, and wGA are the weight fractions of L-lactide, ε-caprolactone, and glycolide, respectively (Tg,LA (332 K, 58°C), Tg,CL (213 K, −60°C) and Tg,GA (308 K, 35°C) represent the corresponding homopolymers). The experimental Tg values are seen to agree closely with those estimated by the Fox equation. For terpolyester 5 of , Tg values of 39 and 37°C were measured in the first and second heating runs, respectively, compared to a Fox-estimated value of 34°C. With few exceptions, melting peaks were observed at higher temperatures (between 75–125°C) in the first heating run, suggesting a semi-crystalline morphology of these terpolyesters, but as expected the terpolymer melting peaks were observed at lower temperatures than the melting temperature of pure poly(L-lactide).
Coating integrity and drug release studies
Polymer coatings on metal stents control interactions between the underlying structural material and the surrounding vessel tissue. Well-designed polymer coatings can reduce the propensity for thrombosis and inflammatory reactions associated with implantation, while concomitantly functioning to first hold, then release, drugs into the surrounding tissue and bloodstream. The use of biodegradable polymers, such as aliphatic polyesters, carries the additional potential advantage of their elimination (by degradation), allowing faster healing of the implanted region. CitationDrachman et al. (2000) reported the use of ε-caprolactone-co-lactide copolymers as coatings for stainless steel stents, in which the stent polymer was loaded with paclitaxel. The kinetic profile of the drug release showed minimal early bursting, and reached 91% release after 56 days. Six months after stenting, when it is assumed that polymer degradation and drug release are complete, no significant thickening of the neointimal area was observed with the paclitaxel- eluting copolymer-coated stents. However no details on the characteristics of the polymers were provided, such as the polymer molecular weight and the relative ratios of monomers used.
The terpolymers prepared for this study were investigated for their performance following spray-coating onto the stent substrate. The stent coating integrity was evaluated by simulated use tests, and characterized by scanning electron microscopy (SEM) after the test. summarizes qualitatively the coating integrity of the five samples, and provides two SEM images of coated stents. The SEM image of , representing a stent coated with terpolymer 2 (LA:CL:GA = 60:17:23), shows a smooth coating with little-to-no cracking or delamination either in the high strain areas or the non-linear link area. For terpolymers with higher glycolide content, smooth coatings were generally observed. However, in coatings from terpolymers having a higher lactide-to-glycolide ratio, and/or low overall molecular weight, cracks were often seen in the high strain area (). This is probably related to the higher Tg and more brittle nature of L-lactide-rich aliphatic polyesters relatives to CL and GA-containing polyester structures.
Figure 3. SEM images of LA:CL:GA terpolymer coated stents, using (a) terpolymer 2 (60:17:23) and (b) terpolymer 4 (77:17:6). Cracks in the coating of (b) are indicated by the arrows.
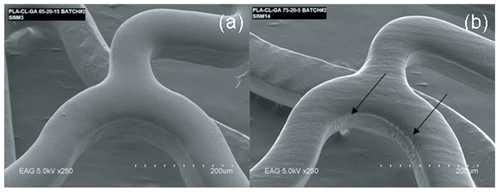
Table 2. Coating integrity and drug release with terpolymers containing a drug-to-polymer ratio of 1–3.a
Drug release profiles of the terpolymer-coated stents were investigated by incorporating the immunosuppressant drug everolimus into the top layer of the coating. The study was performed for a period of 3 days to identify the presence or absence of initial burst. We first considered a terpolyester with a composition of 68:18:14 and a number-average molecular weight of 61,000 g/mol (, entry 3). The drug release profile proved highly sensitive to drug-to-polymer ratio, as seen in for d/p ratios 1/1, 1/2, and 1/3. Testing drug release of a formulation containing d/p of 1/1 revealed a drug burst that released nearly all drug on the first day. Such a burst is suggestive of a phase separated system, in which the polymer matrix is ineffective at containing the small molecule guest. Similar experiments using a d/p of 1/2 revealed a significantly slower release, likely the result of less substantial phase separation, though possibly still above percolation. In experiments using a d/p of 1/3, no burst is seen; instead a very slow initial release is followed by a complete shut-down of the release system. These data suggested that lower d/p ratios are preferred, and that a finer tuning of polymer characteristics can be used to improve the release profiles.
Figure 4. On-stent drug release profile of a terpolymer coating with a LA:CL:GA composition of 68:18:14, and a molecular weight of 61,000 g/mole (, entry 3), using drug-to-polymer (d/p) ratios of 1/1, 1/2, and 1/3.
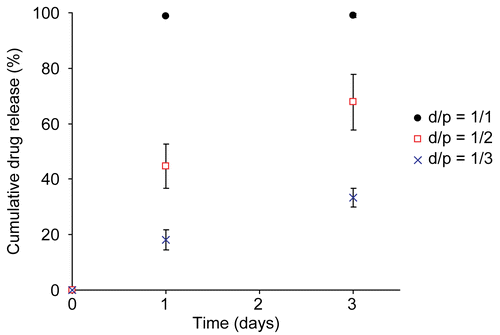
We then considered the influence of polymer molecular weight and composition on the drug release profile. For terpolymers 1 and 4, with similar molecular weights (Mn ~ 13,000 g/mole) but different compositions, drug release was much faster at higher glycolide content. For terpolymer 1, with LA:CL:GA of 61:21:18, the drug release was significantly faster, with a cumulative drug release of 84% after 3 days. Compare this to terpolymer 4, with LA:CL:GA of 77:17:6, which for the same time-frame and d/p reached only 34% of cumulative drug release (, entry 4). This increase in release rate is a function of higher glycolide content in the terpolymer, which serves to increase polymer hydrophilicity, decreases drug solubility, and promotes its release. An added factor may be that the expected crystallinity for terpolymer 4 is greater than for terpolymer 1, further contributing to the difference in release. Faster hydrolysis as a function of greater glycolide content would also increase release rate, though this would be expected over a longer time-frame than that studied here. Polyester molecular weight is also important, as seen in terpolymers 1 and 2, with an approximate LA:CL:GA composition of 60:20:20. Drug release slows with higher molecular weight (, entries 1 and 2, and ), while for a terpolymer with a lower incorporation of glycolide (, entries 4 and 5) the drug release profile shows only a modest difference on day 1, but significant difference on day 3.
Conclusion
In summary, terpolymers prepared from L-lactide, ε-caprolactone, and glycolide were synthesized by ring-opening polymerization in the bulk, with tin(II) 2-ethylhexanoate at 170°C. These random terpolymers were prepared with estimated weight-average molecular weights as high as ~ 100,000 g/mole, and at appropriate monomer combinations the polymers were able to provide excellent coating integrity on bare metal stents. The drug release using a terpolymer with a composition of ~ 60:20:20 (LA:CL:GA) showed good control, especially for the materials containing relatively high molecular weights. The ratio of the comonomers in the terpolyester tuned the relative hydropholicity/hydrophobicity of the polymer coating, allowing control over the release of everolimus based on its solubility in the matrix. Increased glycolide content in the terpolyester enhanced the hydrophilicity of the polymer matrix, leading to an accelerated release of everolimus. The diffusion-based release was observed in the early time-frame, and an initial burst was not seen. Thus, drug release characteristics can be altered by optimizing the polymer composition and molecular weight to retard or accelerate the release kinetics. Taken together, the data indicate that these aliphatic terpolyester materials are attractive candidates for drug delivery applications in which coatings are a critically important component of the system.
Acknowledgments
The authors gratefully acknowledge the financial support of Abbott Vascular and the National Science Foundation Materials Research Science and Engineering Center (MRSEC) on Polymers at the University of Massachusetts Amherst.
References
- Acharya, G., Park, K. (2006). Mechanisms of controlled drug release from drug-eluting stents. Adv Drug Deliv Rev, 58, 387–401.
- Albertsson, A.-.C., Varma, I.K. (2003). Recent developments in ring opening polymerization of lactones for biomedical applications. Biomacromolecules, 4, 1466–86.
- Bezwada, R.S., Jamiolkowski, D.D., Lee, I-Y., Agarwal, V., Persivale, J., Trenka-Benthin, S., Emeta, M., Suryadevara, J., Yang, A., Liu, S. (1995). Monocryl@ suture, a new ultra-pliable absorbable monofilament suture. Biomaterials, 16, 1141–8.
- Cai, Q., Zhao, Y., Bei, J., Xi, F., Wang, S. (2003). Synthesis and properties of star-shaped polylactide attached to poly(amidoamine) dendrimer. Biomacromolecules, 3, 828–34.
- Choi, E-J., Park, J-K., Chang, H-N. (1994). Effect of polymerization catalysts on the microstructure of P(LLA-co-εCL). J Polym Sci B Polym Phys, 32, 2481–9.
- Dechy-Cabaret, O., Martin-Vaca, B., Bourissou, D. (2004). Controlled ring-opening polymerization of lactide and glycolide. Chem Rev, 104, 6147–76.
- Dobrzynski, P. (2002). Synthesis of biodegradable copolymers with low-toxicity zirconium compounds. III. Synthesis and chain-microstructure analysis of terpolymer obtained from L-lactide, glycolide, and ε-caprolactone initiated by zirconium(IV) acetylacetonate. J Polym Sci A Polym Chem, 40, 3129–43.
- Dobrzynski, P., Kasperczyk, J., Bero, M. (1999). Application of calcium acetylacetonate to the polymerization of glycolide and copolymerization of glycolide with ε-caprolactone and L-lactide. Macromolecules, 32, 4735–7.
- Drachman, D.E., Edelman, E.R., Seifert, P., Groothuis, A.R., Bornstein, D.A., Kamath, K.R., Palasis, M., Yang, D., Nott, S.H., Rogers, C. (2000). Noeintimal thickening after stent delivery of paclitaxel: change in composition and arrest of growth over six month. J Am Coll Cardiol, 36, 2325–32.
- Funae, A., Uchiki, K., Akieda, H., Ono, Y. (2000). Random-block copolymer and monofilament thereof. U.S. patent 6,068,920.
- Gilding, D.K., Reed, A.M. (1979). Biodegradable polymers for use in surgery polyglycolic/poly(lactic acid) homo- and copolymers: 1. Polymer, 20, 1459–64.
- Greenwald, D., Shumway, S., Albear, P., Gottlieb, L. (1994). Mechanical comparison of 10 suture materials before and after in vivo incubation. J Surg Res, 56, 372–7.
- Jeong, S.I., Kim, S.H., Kim, Y.H., Jung, Y., Kwon, J.H., Kim, B-S., Lee, Y.M. (2004). Manufacture of elastic biodegradable PLCL scaffolds for mechano-active vascular tissue engineering. J Biomater Sci Polym Ed, 15, 645–60.
- Kasperczyk, J. (1996). Microstructural analysis of poly[(L,L-lactide)-co-(glycolide)] by 1H and 13C NMR spectroscopy. Polymer, 37, 201–3.
- Kricheldorf, H.R., Mang, T., Jonte, J.M. (1984). Polylactones. 1. Copolymerization of glycolide and ε-caprolactone. Macromolecules, 17, 2173–81.
- Kricheldorf, H.R., Jonte, J.M., Berl, M. (1985). Polylactones 3. Copolymerization of glycolide with L,L-lactide and other lactones. Makromol Chem Suppl, 12, 25–38.
- Langer, R. (2000). Biomaterials in drug delivery and tissue engineering: one laboratory’s experience. Acc Chem Res, 33, 94–101.
- Lao, L.L., Venkatraman, S.S. (2008a). Paclitaxel release from single and double-layered poly(DL-lactide-co-glycolide)/poly(L-lactide) film for biodegradable coronary stent application. J Biomed Mater Res, 87A, 1–7.
- Lao, L.L., Venkatraman, S.S. (2008b). Adjustable paclitaxel release kinetics and its efficacy to inhibit smooth muscle cells proliferation. J Contr Rel, 130, 9–14.
- Lee, S-H., Kim, B-S., Kim, S.H., Choi, S.W., Jeong, S.I., Kwon, I.K., Kang, S.W., Nikolovski, J., Mooney, D.J., Han, Y-K., Kim, Y.H. (2003). Elastic biodegradable poly(glycolide-co-caprolactone) scaffold for tissue engineering. J Biomed Mater Res, 66A, 29–37.
- Lyu, S-P., Sparer, R., Hobot, C., Dang, K. (2005). Adjusting drug diffusivity using miscible polymer blends. J Contr Rel, 102, 679–87.
- Miyajima, M., Koshika, A., Okada, J., Ikeda, M. (1999). Effect of polymer/ basic drug interactions on the two-stage diffusion- controlled release from a poly(L-lactic acid) matrix. J Contr Rel, 61, 295–304.
- Nasongkla, N., Shuai, X., Ai, H., Weinberg, B.D., Pink, J., Boothman, D.A., Gao, J. (2004). cRGD-Functionalized polymer micelles for targeted doxorubicin delivery Angew Chem Int Ed, 43, 6323–7.
- Reed, A.M., Gilding, D.K. (1981). Biodegradable polymers for use in surgerypoly(glycolic)/poly(lactic acid) homo and copolymers: 2. In vitro degradation. Polymer, 22, 494–8.
- Srisa-ard, M., Molloy, R., Molloy, N., Siripitayananon, J., Sriyai, M. (2001). Synthesis and characterization of a random terpolymer of L-lactide, ε-caprolactone and glycolide. Polym Int, 50, 891–6.
- Tomihata, K., Suzuki, M., Oka, T., Ikadab, Y. (1998). A new resorbable monofilament suture. Polym Degrad Stab, 59, 13–18.
- Wang, X., Venkatraman, S.S., Boey, F.Y.C., Loo, J.S.C., Tan, L.P. (2006). Controlled release of sirolimus from a multilayered PLGA stent matrix. Biomaterials, 27, 5588–95.