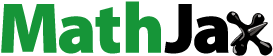
Abstract
Schizophrenia and bipolar disorder are severe chronic neuropsychiatric diseases, affecting hundreds of millions of people worldwide. Asenapine maleate (ASM) has been demonstrated as a safe and effective therapeutic agent under twice-daily administration. However, lower compliance is observed when patients are treated with ASM, which significantly limits its application in schizophrenia and bipolar disorder. Moreover, the low bioavailability of ASM caused by first-pass metabolism and poor aqueous solubility also impairs the treatment effect. A formulation of ASM with the property of long-term sustained release and improved bioavailability can be a solution to overcome these weaknesses. In this article, we prepared ASM-loaded poly(lactic-co-glycolic acid) (ASM-PLGA) microspheres through different techniques, including emulsification-solvent evaporation (ESE), Shirasu porous glass membrane emulsification (SPG-ME), and microfluidic method. In vitro and in vivo assessments demonstrated that uniform-sized microspheres generated by the microfluidic process sustainably released ASM throughout 40-days, showing low burst release and significantly improved bioavailability. The results suggest that ASM-PLGA microspheres prepared by the microfluidic method provide an efficient strategy to enhance the drug exposure of ASM as the treatment of chronic neuropsychiatric diseases. It is also evident that this microfluidic strategy has the potential to construct with other drugs, establishing long-acting formulations.
1. Introduction
Schizophrenia and bipolar disorder are two of the most common chronic neuropsychiatric diseases that lack of effective treatment, affecting about 1 and 2–5% of the world population, respectively (Tarazi & Shahid, Citation2009; McIntyre et al., Citation2010). The severe disability and functional impairment associated with both diseases result in tremendous social burden and cost (Insel, Citation2010). Asenapine maleate (ASM) is a second-generation antipsychotic agent with good tolerability to treat schizophrenia and bipolar disorder. However, a significant first-pass metabolism through oral administration leads to less than 2% bioavailability (Citrome & toxicology, Citation2014; Plosker & Deeks, Citation2016; Vieta et al., Citation2019). Furthermore, it has been found that more than 45% of patients do not show therapeutic effects after treated with ASM, indicating poor compliance (Vieta & Montes, Citation2018). The market product of ASM that approved in Europe (Sycrest®) and United States (Saphris®) is the twice-daily administrated sublingual tablets, which is not very convenient for clinical use (Buoli et al., Citation2017).
According to the literature, several new formulations of ASM have been published (Avachat & Kapure, Citation2014; Shreya et al., Citation2016; Patel et al., Citation2018; Singh et al., Citation2018; Vaishali & Gambhire, Citation2019), while most of them solely focused on improving its bioavailability not the long-term therapeutic effects. However, as is reported in many articles (Citrome, Citation2014; Suresh et al., Citation2020), patients with schizophrenia and bipolar disorder would not be cooperative enough to be compliant with the dosage regimen. Missing as few as 1–10 days of therapy nearly doubles the risk of hospitalization. Therefore, it is important to prolong the release of drug to reduce the number of administrations, thereby increasing patient compliance with the dosage regimen. Compared to traditional long-acting implants, biodegradable microspheres are attracting attentions for numbers advantages, such as no organic solvents used during administration, low burst release and acceptable discomforts. Zhou et al. (Citation2018) prepared PLGA microspheres for ASM, but animal experiments were lacked to prove therapeutic effects in vivo. Thus, the long-acting sustained-release dosage form of ASM with improved bioavailability is in necessary to reduce dosing frequency and enhance patient compliance.
Biodegradable polymeric microsphere-based drug products with the properties of parenteral controlled release have been widely used for long-term delivery of both small molecules and proteins, which shows the ability to decrease the dosing frequency and facilitate patient compliance Burgess et al. (Citation2004). Poly(lactic-co-glycolic acid) (PLGA) is a biodegradable copolymer approved by the U.S. Food and Drug Administration (FDA). PLGA microspheres have been widely used for the development of drug delivery approaches in vivo (Kakazu et al., Citation2010; Kulkarni & Avachat, Citation2017). The particle size of microspheres is a crucial parameter that influences the release kinetics and treatment efficacy of drug loaded PLGA microspheres. On the other hand, the size distribution is another critical factor to determine the in vivo effects of the particles by adjusting the foreign body reaction Wu et al. (Citation2013). Therefore, particle size and size distribution control play essential roles in improving therapeutic efficacy and reproducibility.
The typical techniques for preparing PLGA microspheres include emulsification-solvent evaporation (ESE), phase separation, spray drying, precipitation, etc. (Husmann et al., Citation2002; Doan & Olivier, Citation2009; Cozar-Bernal et al., Citation2011; Nath et al., Citation2013). Moreover, Shirasu porous glass membrane emulsification (SPG-ME) and microfluidic method have been recently developed (Ito et al., Citation2008; Zhao et al., Citation2014). However, it is still challenging to generate uniform-sized and reproducible long-term PLGA microspheres loaded with ASM.
In the present work, we compared the fabrication of ASM-PLGA microspheres using ESE, SPG-ME, as well as microfluidic method, and discussed the influence of particle size and fabrication procedures on drug release kinetics. After systematic optimization and characterization, the microfluidic approach can easily produce uniform and smooth ASM-PLGA microspheres (denoted as ASM-PLGA-M microspheres). The afforded ASM-PLGA-M microspheres facilitated continuous drug long-term sustained release over 40 days without burst. In addition, we investigated the release mechanism of ASM from microspheres, examined the release kinetic behaviors of these three types of ASM-PLGA microspheres in vitro, and determined the pharmacokinetic profiles of ASM-PLGA-M microspheres in vivo. We concluded that the microfluidic method is the most suitable for producing monodisperse droplets. It provides easy control of the particle size, indicating this strategy is applicable to the generation of long-term microspheres loaded with other drugs.
2. Material and methods
2.1. Materials
PLGA (L/G = 75:25, MW = 50,000) was purchased from the Shandong Academy of Pharmaceutical Sciences (Pilot Plant; Jinan, China). Poly(vinyl alcohol) 210 (PVA 210) was obtained from Aladdin Bio-Chem Technology Co., Ltd. (Shanghai, China). ASM was supplied by Haotian Chemical Technology Co. Ltd. (Huizhou, China). All other chemicals and reagents were of analytical reagent grade.
2.2. Preparation of ASM-loaded PLGA microspheres
2.2.1. Microfluidic approach
ASM and PLGA at a ratio of 1:5 were dissolved in dichloromethane (DCM, 10 wt% polymer), serving as the dispersed phase. Aqueous PVA solution (0.5%, w/v) was served as the continuous phase. The microfluidic system consisted of two digitally controlled syringe pumps: one containing 1% PVA solution and the other containing the polymer solution. Both syringes were connected to a cross-shaped microchannel. The flow rate of the dispersed phase was set to 0.3 mL/h, while the flow rate of the continuous phase was 6.0 mL/min. Simultaneous injections of both phases were fused in the cross-shaped microfluidic chip, resulting in self-assembled oil-in-water (O/W) emulsions at the cross-junction. Then the emulsion droplets were collected from the outlet tubing for further solvent removal. After evaporating DCM at room temperature for 24 h, the obtained microspheres were washed with distilled water and lyophilized for another 24 h to remove the remaining solvent completely.
2.2.2. SPG-ME
ASM and PLGA at a ratio of 1:5 were dissolved in DCM (5 wt% polymer), which served as the dispersed phase, and they were transferred to an oil tank on the SPG membrane emulsification apparatus; 0.5 wt% PVA aqueous solution acted as the continuous phase. With the help of nitrogen pressure, the PLGA solution passed through the SPG membrane pores (30 μm) and was dispersed in the continuous phase to form the monodispersed O/W emulsion. After the evaporation of DCM for 24 h, the obtained microspheres were washed with distilled water and lyophilized for another 24 h, and then ASM-PLGA microspheres were finally obtained.
2.2.3. ESE method
ASM and PLGA at a ratio of 1:5 were dissolved in DCM (5 wt% polymer), serving as the oil phase. And then, the solution was added to a 10 mL of 0.5 wt% PVA aqueous solution. The mixed solution was agitated at 500 rpm to form an O/W emulsion followed by emulsification for 10 min. Next, the emulsion was kept for 5 h at room temperature to permit solvent evaporation. The afforded ASM-PLGA microspheres were collected by centrifugation at 8000 rpm, washed with distilled water, and lyophilized.
2.3. Particle size and morphology
The number of average-diameter microspheres and the value of the span for the prepared microspheres were calculated using data from 200 particles observed with the CX22 optical microscope (Olympus, Tokyo, Japan). The particle size distribution was evaluated by the value of SPAN, which was calculated using the following equation:
(1)
(1)
where D90, D10, and D50 are the average particle diameters of particles, accounting for 90%, 10%, and 50% of total particles, respectively. In addition, the surface features of the ASM-PLGA microspheres were observed by scanning electron microscopy (SEM) (Carle Zeiss, Oberkochen, Germany). Before observation, the specimens were prepared by coating a thin gold film on the sample under vacuum conditions.
2.4. Encapsulated efficiency and drug loading
ASM-PLGA microspheres (10 mg) were weighed and transferred to a 10 mL volumetric flask. Acetonitrile was added to the volumetric flasks, and the samples were sonicated until the ASM dissolved. The mobile phase was applied to dilute the samples. The solution was filtered, and the concentration of ASM was measured using a validated high-performance liquid chromatography (HPLC) protocol at 234 nm. The mobile phase consisted of methanol: acetonitrile: water = 20:60:20. The linear range was from 5 μg/mL to 100 μg/mL (r = 0.9999). Drug-loading efficacy and drug loading were calculated with the following equations:
(2)
(2)
(3)
(3)
2.5. X-ray diffraction (XRD) and differential scanning calorimetry (DSC)
The crystallinity of samples was investigated by X-ray diffraction (XRD, Panalytical, The Netherlands) in the region of 5° ≤2θ ≤ 40° with an angular increase of 5°/min (at 25 °C, by means of monochromatic Co Kα radiation at 40 kV and 40 mA). Thermal aspects were analyzed using a modulated temperature differential scanning calorimeter (DSC, Perkin Elmer Inc., San Jose, CA, USA). Under nitrogen gas purge, 10 mg sample enclosed in an aluminum pan was subjected to heating at a constant rate of 10 °C/min from 20 °C to 200 °C.
2.6. Drug release in vitro
ASM-PLGA microspheres (50 mg) were dispersed in 5.0 mL phosphate-buffered saline buffer (PBS, pH 7.4) containing 0.5% SDS, 0.02% Tween, and 0.0.2% sodium azide in a test tube with a stopper. The dispersed solution was shaken at a rate of 60 times/min (37 °C ± 0.5 °C). Then, 1 mL of sample was collected on days 1, 3, 6, 9, 12, 16, 20, 24, 28, 34, and 40, and centrifuged at 10000 rpm. At the same time, 1 mL of blank solution was added to the sample. The supernatants were measured at 234 nm by HPLC.
2.7. Pharmacokinetics
The sterile ASM-PLGA microspheres were intramuscularly (IM) injected into the right-side adductor of the beagles dissolved in 2 mL blank saline solution (3.74 mg/kg calculated from ASM sublingual tablet 10 mg/day). Furthermore, at prefixed intervals, 2 mL of blood was collected via the forelimb vein. The collected sample was immediately centrifuged at 3500 rpm for 15 min and kept at −80 °C until analysis. Each plasma sample (100 µL) was mixed with 10 µL internal standard solution (diphenhydramine in acetonitrile, 10 ng/mL) and vortexed for 30 s. Then 800 µL methyl tertiary butyl ether was added to the sample, and the mixture was vortexed for 2 min and centrifuged at 14000 rpm for 10 min (at 4 °C). The resulting supernatant (75 µL) was withdrawn and examined by HPLC-MS/MS. All pharmacokinetic parameters were obtained using DAS3.2 software (Chinese Pharmacological Society, China). The values, including plasma concentration at each time, are presented as the means ± standard deviations (SD).
2.8. Statistical analysis
The results were analyzed by a one-way analysis of variance followed by Tukey’s test with a significance level of p < .05. The statistical analysis was performed using GraphPad Prism (GraphPad Prism Software version 6, San Diego, CA, USA).
3. Results and discussion
3.1. Preparation of ASM-PLGA microspheres with ESE technique (ASM-PLGA-ESE)
ESE is the most commonly used method for preparing PLGA microspheres due to simplicity (Perugini et al., Citation2003; Matsumoto et al., Citation2008). Dissolved polymer and drug were mixed in a volatile organic solvent, and then the organic phase was emulsified under mechanical force in a continuous aqueous phase containing emulsifier. Subsequently, the primary droplets shrinked and transformed into solid particles during solvent evaporation. In our study, DCM and PVA were used as the organic phase and emulsifier, respectively. Eight variables of this procedure were investigated, and the results are listed in and Table S1. The encapsulation efficiency (EE), drug loading (DL), particle size, and span were chosen as response variables for comparison. Based on these results, the optimal condition was determined as follows: 100 mg/mL polymer, 1:5 ratio of drug to polymer, 0.5% of PVA, 500 r/min of emulsification speed, 10 min of emulsification time, 1:10 ratio of oil to water, 300 r/min of evaporation speed and 5 h evaporation time. The average particle size of obtained ASM-PLGA-ESE microspheres was 71 μm, while EE, DL, and span were determined as 80%, 14%, and 0.89, respectively ().
Table 1. The variables and ranges used in the experimental study.Table Footnotea.
Table 2. Summary of ASM-PLGA microspheres prepared by different techniques.
3.2. Preparation of ASM-PLGA microspheres with SPG-ME technique (ASM-PLGA-SPG)
SPG-ME is a technique that fabricates micro-sized emulsions via uniform-sized membrane pores (Doan & Olivier, Citation2009; Doan et al., Citation2011). The size of formed particles originated from emulsions can be precisely tuned by adjusting the size of membrane pores (Van-Thanh et al., Citation2011; Ramazani et al., Citation2016). Although the membrane pore mainly determines the size of particles, experimental variables can also affect the characteristics of PLGA microspheres. In this work, DCM and PVA solutions were used as the dispersed phase and the continuous phase, respectively. Six variables were adjusted and evaluated based on EE, DC, particle size, and span to obtain optimal microspheres ( and Table S2), which were finally set up as follows: 50 mg/mL polymer, 1:5 ratio of drug to polymer, 0.5% of PVA, 4 KPa of extraction pressure, 5 mL of fill volume, and 300 r/min of stirring speed. Totally, 67 μm ASM-PLGA-SPG microspheres were produced, for which EE and DL were determined as 91% and 15%, respectively ().
Table 3. The variables and ranges used in the experimental studya.
3.3. Preparation of ASM-PLGA microspheres with microfluid technique (ASM-PLGA-M)
Microfluidic technology provides an easy-to-use and low-cost platform for controlling the size of particles that are generated from emulsions (Zhao et al., Citation2014; Busatto et al., Citation2018). In the flow-focusing microfluidic devices, the aqueous phase is introduced through two side channels, and the organic phase flows into the central channel of the device with constant rates (Scheme 1). DCM and PVA solutions were used as the dispersed phase and the continuous phase, respectively. Similar to the evaluation described above, we investigated the influences of four formulation parameters on the characteristics of the PLGA microspheres, including polymer concentration, emulsifier concentration, speed of dispersed phase, and speed of continuous phase (Table S3). With the suitable variables (), 45 μm ASM-PLGA-M microspheres were obtained with a narrow particle size distribution (span = 0.17). It was noted that, compared to microspheres prepared by ESE and SPG-ME approaches, ASM-PLGA-M microspheres exhibited relatively higher drug loading efficacy (96.91%) and drug loading (16.16%). In contrast, the broad span data ASM-PLGA-ESE and ASM-PLGA-SPG microspheres were determined to be 0.88 and 0.66, respectively, suggesting the non-uniformity and wide range of distribution. These data demonstrated that microfluidic method could produce uniform-sized microspheres with controlled size.
Scheme 1. Schematic of the synthetic approach for the PLGA microspheres. PVA, poly (vinyl alcohol); ASM, asenapine maleate; PLGA, poly (lactic-co-glycolic acid).
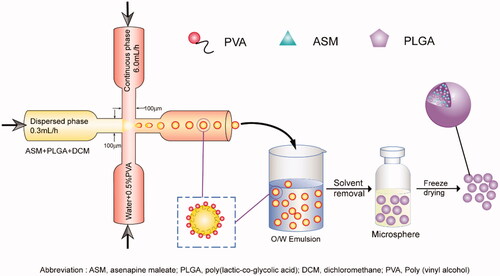
Table 4. The variables and ranges used in the experimental studyTable Footnotea.
3.4. Characterization of ASM-PLGA microspheres
The morphologies of ASM-PLGA microspheres observed by scanning electron microscopy (SEM) indicate that ASM-PLGA-ESE and ASM-PLGA-SPG microspheres were ununiform and irregular to a certain extent (). On the other hand, ASM-PLGA-M microspheres () exhibited a relatively smooth and consistently round form with a compact interior structure and mono-dispersion in accordance with the results of particle size distribution. The microfluidic method allows the properties of PLGA carriers to be flexibly controlled by adjusting the polymer concentration, emulsifier concentration, speed of dispersed phase, and speed of continuous phase, thus suggesting that this method is the most suitable approach to yield monodispersed ASM-PLGA microspheres. In addition, there is potential to develop a procedure to manufacture uniformed spherical PLGA microspheres at the industrial scale through multichannel microfluidic chips.
3.5. Analysis by XRD and DSC
The influence of temperature on the crystallization of ASM is critical to understand the interaction between ASM and PLGA and facilitate optimization of the processing technology. As it is important to monitor the changes that occur in ASM-PLGA microspheres, X-ray diffraction (XRD) and differential scanning calorimeter (DSC) analysis were further conducted on pure ASM, PLGA, as well as three types of ASM-loaded PLGA microspheres (). The XRD results revealed that the curve of ASM exhibited several sharp characteristic peaks at diffraction angles, corresponding to its crystalline state (), which were not observed in the three kinds of PLGA microspheres. On the other hand, PLGA (25:75), an amorphous polymer, did not show any pronounced peak. It has been reported that PLGA can inhibit the crystallization of API, while its amorphous form promotes homogeneous drug distribution within the PLGA microspheres (Makadia & Siegel, Citation2011). Decreased burst release in microspheres is beneficial for forming a uniform amorphous state of ASM within the PLGA carriers. (Takada et al., Citation1997). The DSC data in show that ASM produced an endothermic peak at about 146 °C, similar to its melting point. At the same time, PLGA (75:25) exhibited an endothermic peak at around 49 °C, which is the glass transition temperature of PLGA. Notably, all ASM-PLGA microspheres did not display the drug diffraction peak at 146 °C, suggesting that ASM was present in the amorphous form (). As indicated from XRD and DSC data, crystalline ASM was converted into an amorphous state within the PLGA matrix, assuring a better dissolution rate of the drug from the PLGA matrix, which may lead to an improved therapeutic concentration in vivo.
3.6. Release kinetics of ASM from PLGA microspheres
The drug-release behavior is significantly affected by the preparation method (Qi et al., Citation2014). Similarly, three PLGA microspheres showed completely different release profiles in our study. As shown in , ASM-PLGA-M microspheres exhibited a low initial burst within the first day of incubation in PBS (pH 7.4, 37 °C). Slow and continuous drug release was observed and proceeded in roughly zero-order kinetics, which likely contributed to a stable plasma concentration without side effects. ASM may exhibit an amorphous form in the PLGA matrix (Ito et al., Citation2008). Both ASM-PLGA-ESE and ASM-PLGA-SPG microspheres showed a plateau with a high burst, which was probably because of their coarse surface, poor uniformity, and large span.
To further investigate the release mechanisms of ASM from ASM-PLGA-M microspheres, polymer degradation of microspheres was examined by SEM. Diffusion and degradation/erosion are two major release mechanisms associated with drug release from PLGA (Sinha & Trehan, Citation2003; Fredenberg et al., Citation2011). Typically, the drug released from PLGA microspheres is controlled by the interactions of water absorption, hydrolysis, erosion, and diffusion through the PLGA polymer matrix (Fredenberg et al., Citation2011). The results in show that the external surfaces of microspheres became coarse with pores on day 10, and more pores were formed as the incubation. Large pores were also observed inside the microspheres, but all of them still maintained their integrity upon to 40 days. These results demonstrated that the degradation of PLGA microspheres was through bulk degradation, indicating that the diffuse rate of release medium was faster than PLGA erosion. During the release period, most of the PLGA microspheres were consistently intact, while small pores gradually appeared within the matrix as time increased. Both erosion and diffusion were observed in the release process of ASM from PLGA microspheres.
3.7. Pharmacokinetics in vivo and correlation with drug release in vitro
The pharmacokinetic parameters in beagle dogs and plasma concentration versus time curves of ASM-PLGA-M microspheres are shown in and . According to other study (Chen et al., Citation2015), following a single 10 mg dose of ASM sublingual tablet (Saphris®) for beagle dogs, the mean Cmax was approximately 43.4 ng/ml, the AUC0−∞ and MRT0−∞ values were 118.1 ng·mL−1·d and 3.8 h, respectively. In our study, the prepared ASM-PLGA-M microspheres were intramuscularly injected into the right-side adductor of the beagles dissolved in 2 mL blank saline solution (3.74 mg/kg calculated from ASM sublingual tablet 10 mg/day). The Cmax was approximately 45.6 ng/mL at the first day, the AUC0−∞ and MRT0−∞ values were 406.1 ng·mL−1·d and 14 d, respectively. Thus, it is indicated from AUC that bioavailability is increased 4.4-fold than the ASM sublingual tablet. The increased AUC may be due to the ability of microspheres to sustain the drug release for a prolonged duration of time when compared to the ASM sublingual tablet. More importantly, the sustained-release time of ASM-PLGA microspheres could maintained for 40 days. Both improved bioavailability and greatly extended drug released time showing superiority of ASM-PLGA-M microspheres over the sublingual formulation. In addition, there was a desired correlation between accumulated release in vitro and the AUC in vivo. The fitted equation was AUC = 3.33919 Q − 7.38016 (r = 0.9975). Therefore, the accumulated drug release can well predict ASM absorption in vivo. As the ASM sublingual tablet could not be successfully administered to the beagles, we only studied the pharmacokinetics of the ASM-PLGA microspheres.
Figure 5. Plasma concentration-time curve of ASM-PLGA-M microspheres following intramuscular administration in beagles.
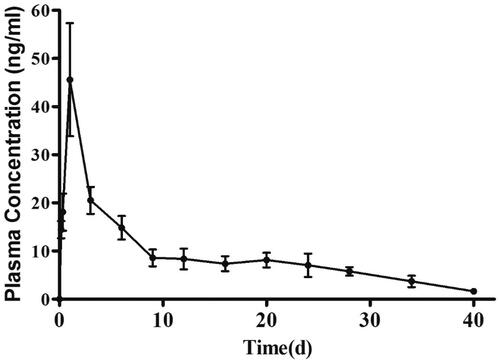
Table 5. The pharmacokinetic parameters of ASM-PLGA-M.
4. Conclusions
In this work, we systematically investigated the effects of different synthesis strategies on the characteristics of PLGA microspheres. For the first time, we report the dosage form for ASM with sustained release and improved bioavailability at the same time. We demonstrated that the microfluidic technique could provide homogeneous and controllable PLGA microspheres. The obtained ASM-PLGA-M microspheres facilitated continuous drug release without initial burst for the presence of an amorphous state of ASM in the PLGA matrix. More importantly, the microsphere not only showed sustained release of the drug over 40 days but also enhanced absorption and improved bioavailability in vivo. Moreover, a zero-order kinetics property and erosion-diffusion mechanism were observed for these microspheres. With these features, ASM-PLGA-M microspheres may exhibit better efficiency and compliance as the treatment of schizophrenia and bipolar disorder I. In addition, this strategy is also applicable to the generation of long-term microspheres for other drugs.
Supplemental Material
Download MS Word (28 KB)Acknowledgements
The authors gratefully acknowledge the support of the Science and Technology Program of Guangzhou, China (No. 201604020166).
Disclosure statement
The authors declared that there is no conflict of interest.
Additional information
Funding
References
- Avachat AM, Kapure SS. (2014). Asenapine maleate in situ forming biodegradable implant: an approach to enhance bioavailability. Int J Pharm 477:64–72.
- Buoli M, Esposito CM, Godio M, et al. (2017). Have antipsychotics a different speed of action in the acute treatment of mania? A single-blind comparative study. J Psychopharmacol 31:1537–43.
- Burgess DJ, Crommelin DJA, Hussain AS, Chen M-L. (2004). Assuring quality and performance of sustained and controlled released parenterals. Eur J Pharm Sci 21:679–90.
- Busatto C, Pesoa J, Helbling I, et al. (2018). Effect of particle size, polydispersity and polymer degradation on progesterone release from PLGA microparticles: experimental and mathematical modeling. Int J Pharm 536:360–9.
- Chen F, Yang L, Zhou Z, et al. (2015). Preparation and in vitro/in vivo evaluation of asenapine maleate sublingual films. Chinese Int J Pharm 46:843–855.
- Citrome LJE. (2014). Asenapine review, part I: chemistry, receptor affinity profile, pharmacokinetics and metabolism. Expert Opin Drug Metab Toxicol 10:893–903.
- Cozar-Bernal MJ, Holgado MA, Arias JL, et al. (2011). Insulin-loaded PLGA microparticles: flow focusing versus double emulsion/solvent evaporation. J Microencapsul 28:430–41.
- Doan TVP, Couet W, Olivier JC. (2011). Formulation and in vitro characterization of inhalable rifampicin-loaded PLGA microspheres for sustained lung delivery. Int J Pharm 414:112–7.
- Doan TVP, Olivier JC. (2009). Preparation of rifampicin-loaded PLGA microspheres for lung delivery as aerosol by premix membrane homogenization. Int J Pharm 382:61–6.
- Fredenberg S, Wahlgren M, Reslow M, Axelsson A. (2011). The mechanisms of drug release in poly(lactic-co-glycolic acid)-based drug delivery systems-a review. Int J Pharm 415:34–52.
- Husmann M, Schenderlein S, Luck M, et al. (2002). Polymer erosion in PLGA microparticles produced by phase separation method. Int J Pharm 242:277–80.
- Insel TR. (2010). Rethinking schizophrenia. Nature 468:187–93.
- Ito F, Honnami H, Kawakami H, et al. (2008). Preparation and properties of PLGA microspheres containing hydrophilic drugs by the SPG (Shirasu porous glass) membrane emulsification technique. Colloids Surf B Biointerfaces 67:20–5.
- Kakazu E, Murakami T, Akamatsu K, et al. (2010). Preparation of silver nanoparticles using the SPG membrane emulsification technique. J Membr Sci 354:1–5.
- Kulkarni JA, Avachat AM. (2017). Pharmacodynamic and pharmacokinetic investigation of cyclodextrin-mediated asenapine maleate in situ nasal gel for improved bioavailability. Drug Dev Ind Pharm 43:234–45.
- Makadia HK, Siegel SJ. (2011). Poly lactic-co-glycolic acid (PLGA) as biodegradable controlled drug delivery carrier. Polymers (Basel) 3:1377–97.
- Matsumoto A, Kitazawa T, Murata J, et al. (2008). A novel preparation method for PLGA microspheres using non-halogenated solvents. J Control Release 129:223–7.
- McIntyre RS, Cohen M, Zhao J, et al. (2010). Asenapine in the treatment of acute mania in bipolar I disorder: a randomized, double-blind, placebo-controlled trial. J Affective Disord 122:27–38.
- Nath SD, Son S, Sadiasa A, et al. (2013). Preparation and characterization of PLGA microspheres by the electrospraying method for delivering simvastatin for bone regeneration. Int J Pharm 443:87–94.
- Patel MR, Hirani SN, Patel RB. (2018). Microemulsion for nasal delivery of Asenapine maleate in treatment of schizophrenia: formulation considerations. J Pharm Investig 48:301–12.
- Perugini P, Genta I, Conti B, et al. (2003). PLGA microspheres for oral osteopenia treatment: preliminary “in vitro”/“in vivo” evaluation. Int J Pharm 256:153–60.
- Plosker GL, Deeks EDJCd. (2016). Asenapine: a review in schizophrenia. CNS Drugs 30:655–66.
- Qi F, Wu J, Yang T, et al. (2014). Mechanistic studies for monodisperse exenatide-loaded PLGA microspheres prepared by different methods based on SPG membrane emulsification. Acta Biomater 10:4247–56.
- Ramazani F, van Nostrum CF, Storm G, et al. (2016). Locoregional cancer therapy using polymer-based drug depots. Drug Discov Today 21:640–7.
- Shreya AB, Managuli RS, Menon J, et al. (2016). Nano-transfersomal formulations for transdermal delivery of asenapine maleate: in vitro and in vivo performance evaluations. J Liposome Res 26:221–32.
- Singh SK, Hidau MK, Gautam S, et al. (2018). Glycol chitosan functionalized asenapine nanostructured lipid carriers for targeted brain delivery: Pharmacokinetic and teratogenic assessment. Int J Biol Macromol 108:1092–100.
- Sinha VR, Trehan A. (2003). Biodegradable microspheres for protein delivery. J Controlled Release 90:261–80.
- Suresh A, Narayan R, Nayak UY. (2020). Recent advances in the development of asenapine formulations. Expert Opin Drug Delivery. 1–17.
- Takada S, Kurokawa T, Miyazaki K, et al. (1997). Utilization of an amorphous form of a water-soluble GPIIb/IIIa antagonist for controlled release from biodegradable microspheres. Pharm Res 14:1146–50.
- Tarazi FI, Shahid M. (2009). Asenapine maleate: a new drug for the treatment of schizophrenia and bipolar mania. Drugs Today 45:865–76.
- Vaishali M, Gambhire NSR. (2019). Enhanced oral delivery of asenapine maleate from solid lipid nanoparticles: pharmacokinetic and brain distribution evaluations. Asian J Pharm 12:152–61.
- Van-Thanh T, Benoit J-P, Venier-Julienne M-C. (2011). Why and how to prepare biodegradable, monodispersed, polymeric microparticles in the field of pharmacy? Int J Pharm 407:1–11.
- Vieta E, Manuel Montes J, Iborra P, et al. (2019). Management of asenapine treatment in clinical practice: Recommendations from a panel of experts. Rev Psiquiatr Salud Ment 12:163–9.
- Vieta E, Montes JM. (2018). A review of asenapine in the treatment of bipolar disorder. Clin Drug Investig 38:87–99.
- Wu J, Kong T, Yeung KW, et al. (2013). Fabrication and characterization of monodisperse PLGA-alginate core-shell microspheres with monodisperse size and homogeneous shells for controlled drug release. Acta Biomater 9:7410–9.
- Zhao H, Xu J-H, Wang T, Luo G-S. (2014). A novel microfluidic approach for preparing chitosan–silica core–shell hybrid microspheres with controlled structures and their catalytic performance. Lab Chip 14:1901–6.
- Zhou X, He D, Yang J, et al. (2018). Preparation and in vitro release of asenapine maleate-loaded microspheres. Chin J New Drugs 2:17.