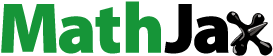
Abstract
Organophosphorus agents, also known as nerve agents, are very dangerous chemicals that were used as chemical warfare agents. HI-6 is one of the most promising reactivators which is effective in reactivating AChE inhibited by many nerve agents. However, the fast in-vivo clearance of HI-6 became a large barrier for first aid use under some sophisticated circumstances. In this study, PEGylated liposomes loading HI-6 were prepared and evaluated in vitro and in vivo. For PEG-LP-HI-6, the optimal formulation’s loading efficiency and encapsulation efficiency were 6.47 ± 0.10% and 71.2 ± 1.15%, respectively. According to the pharmacokinetic results, compared with free HI-6 and LP-HI-6, the intravenous injection of PEG-LP-HI-6 significantly extended t1/2 (1.47 ± 0.29 h), MRT (1.44 ± 0.07 h), and improved the AUC of HI-6 in vivo. Drug concentrations in the CNS also increased after the intravenous administration of PEG-LP-HI-6. For in vivo treatment study, twenty minutes after poison exposure, the survival rate of animals in saline, free HI-6, LP-HI-6 and PEG-LP-HI-6 groups were 0, 0, 30% and 70%, respectively. Compared with the non-PEGylated liposomes group and free HI-6, PEG-LP-HI-6 could prolong the survival time of experimental animals and alleviate the neurotoxic symptoms, which demonstrated great potential as a first-aid strategy for acute organophosphorus agent poisoning.
Introduction
Organophosphorus agents (OPs) typically have strong neurological effects. Some of them have high toxicity and rapid lethal effects, such as soman, sarin, tabun, and VX, which were named chemical warfare agents (Hulse et al., Citation2019). In 2013, sarin is deemed to be used in the Ghouta chemical weapons attack, killing about 1300 people and countless animals. In 2017, Kim Jong-nam was assassinated at a Malaysian airport by VX, a colorless and odorless deadly nerve agent that can kill people in minutes. Although chemical warfare agents have been banned by international convention, OP nerve agents still pose a big threat to public safety (John & Thiermann, Citation2021).
OP nerve agents can inhibit acetylcholinesterase’s (AChE) activity by phosphorylating its active site serine residue, leading to accumulation of the neurotransmitter acetylcholine and over-stimulation of cholinergic receptors (Hulse et al., Citation2019). This process could occur in both central nervous system (CNS) and peripheral nervous system (PNS), and the main symptoms of poisoning are pupil narrowing, salivation, nausea, vomiting, muscle fibrillation, spasm and nerve paralysis. (Hulse et al., Citation2019; Kloske & Witkiewicz, Citation2019). Once OP poisoning occurs, victims can lose their lives in several minutes (Diauudin et al., Citation2019; Kloske & Witkiewicz, Citation2019; Meads et al., Citation2021), thus, first aid measures are very important for the treatment of nerve agents.
The current treatment strategy is to relieve acute symptoms by administration of a muscarinic acetylcholine (ACh) receptor antagonist and an anticonvulsant drug, as well as oxime antidotes to restore AChE function (Hulse et al., Citation2019). Oxime AChE reactivators have been widely studied and proven to be effective in reactivating phosphorylated AChE (Kuca et al., Citation2010; Acharya et al., Citation2011; Gupta & Ghosh, Citation2013; Kovarik et al., Citation2013). Among these antidotes, HI-6 is one of the most promising reactivators against OP poisoning. HI-6 has good effects on reactivating AChE that inhibited by many nerve agents, in terms of soman, sarin and cyclosarin (Koplovitz & Stewart, Citation1994; Cassel & Fosbraey, Citation1996; Lundy et al., Citation2005; Myhrer et al., Citation2018; Reymond et al., Citation2018). Although HI-6 exerts a powerful effect in in vitro experiments, the treatment effect of HI-6 is still unsatisfactory due to its very fast clearance rate in plasma (Ligtenstein & Kossen, Citation1983; Myhrer et al., Citation2018). Furthermore, poor stability in plasma inevitably leads to low-level drug distribution in the CNS, leaving serious brain damage unsettled (Myhrer et al., Citation2018). Intravenous infusion is an effective way to achieve stable drug concentration in blood, but this method requires the support of professional medical personnel and medical equipment which are not easy to approach during wars or other emergency situations.
Nanocarriers play an important role in changing the pharmacokinetic characteristics of drugs (Dadparvar et al., Citation2014; Singh et al., Citation2022). Among them, PEGylated liposome drug delivery system (PEG-LP-DDS) is designed to protect active pharmaceutical ingredients from fast clearance by wrapping them in PEGylated phospholipids (Allen et al., Citation1995; Marjan & Allen, Citation1996; Pinho et al., Citation2021; Sun et al., Citation2022). PEG-LP could prevent the combination of drugs and plasma proteins, and prolong the biological half-life of HI-6, reducing the administration times of antidotes. Furthermore, PEG-LP formulations can be prepared as dry powders or auto-injectors, which are suitable for sophisticated situations, such as war or anti-terrorism activities. Theoretically, good stability in plasma also gives HI-6 molecules more opportunity to penetrate the blood–brain barrier, reactivate AChEs in CNS, and reduce brain damage during or after OP exposure.
In this study, we report a PEG-LP formulation containing HI-6 as an antidote. In vitro characterization, in vivo pharmacokinetics, and evaluation of antitoxic treatments were performed in this study. The present results suggest prolonged drug metabolism and enhanced antitoxic ability of PEG-LPs. This shows that PEG-LP-DDS may be a promising drug delivery system as a first aid in acute OPs poisoning.
Material and methods
Chemicals and reagents
HI-6 was obtained from Sigma-Aldrich (St. Louis, MO, USA); soy phosphatidylcholine (S100) was purchased from Lipoid GmbH (Ludwigshafen, Germany); 1,2-distearoyl-sn-glycero-3-phosphore-thanolamine-N-[methoxy(polyethylene glycol)-2000] (DSPE-mPEG 2000) was obtained from Xi’ an Ruixi Biological Technology Co., Ltd (Xi’ an, China); and 1,2-dioleoyl-sn-glycero-3-phospho-L-serine (DOPS) was purchased from Avanti Polar Lipids. Triton X-100 was purchased from Sigma-Aldrich (St. Louis, MO, USA). High-performance liquid chromatography (HPLC) solvents were purchased from Fisher Chemicals (Fair Lawn, NJ). All other chemicals were of analytical reagent grade or better.
Preparation of HI-6-loaded liposome
HI-6-loaded liposomes (LP-HI-6) and HI-6-loaded PEGylated liposomes (PEG-LP-HI-6) were prepared using a thin-film hydration method. Briefly, LP-HI-6 was formulated at a mass ratio of 60:20:20 (SPC: cholesterol: DOPS) and PEG-LP-HI-6 was prepared at a mass ratio of 56:20:20:4 (SPC: cholesterol: DOPS: DSPE-mPEG2000). The lipid materials were dissolved in chloroform and a thin-film was generated by rotary evaporation at 45 °C. The film was then desiccated for 12 h until completely dry. HI-6 solution was used to re-dissolve the film at different drug-to-lipid ratios (D/L ratios) (0%, 5%, 10%, and 20%). The suspension was then sonicated using a probe-type sonicator (200 W for 8 min) to obtain liposomes.
Characterizations of liposome
The size distribution, polydispersity index (PDI), and zeta potential of the liposomes were determined by dynamic light scattering (DLS), whereas morphology was assessed by transmission electron microscopy (TEM). The liposomes were dispersed in deionized water, after which 10 μL of the sample was dropped on a copper mesh for 30 min. Next, 10 μL of phosphotungstic acid was added, and the excess sample/reagent was removed with filter paper. The copper mesh was dried, and TEM analysis was performed.
Drug loading efficiency and encapsulation efficiency
The drug-loading efficiency (LE%) and encapsulation efficiency (EE%) were assessed for samples containing HI-6. To determine the total amount of HI-6, 10 μL of LP-HI-6 or PEG-LP-HI-6 was diluted to 1 mL with 75% ethanol, then 10 μL diluent was removed and diluted to 1 mL with 0.5% Triton X-100, ultrasonicated for 10 min, and the content was determined by high-performance liquid chromatography (HPLC, Agilent Technologies) equipped with a C18 column (SHISEDO, 250 × 4.6 mm). The eluent consisted of 5 mM sodium n-heptanesulfonate and 0.12% trifluoroacetic acid with acetonitrile (85:15 v/v). The detection wavelength was at 300 nm. The injection volume and eluent flow rate were 20 μL and 2 mL/min, respectively. Unloaded HI-6 was removed using an ultrafiltration centrifugal tube (10 K MWCO Millipore) at 4 °C for 40 min (10375 g).
These parameters were calculated using the following equations:
(1)
(1)
(2)
(2)
In vitro HI-6 release profile
The release of HI-6 from liposomes was assessed using the dialysis method. Dialysis bags (12–14 kDa) were soaked in Milli-Q water for 6 h before use. 1 mL LP-HI-6 or PEG-LP-HI-6 was transferred to a bag and sealed using clamps. The bag was immersed in 200 mL phosphate buffer at pH 7.4 (25 mM phosphate dissolved in water and adjusted to pH 7.4 with 10 M NaOH) with agitation at 200 rpm and incubated at 37 ± 0.5 °C. At the predetermined time, 200 μL samples were withdrawn and filtered through a 0.45 μm membrane (Supor®; Pall Corporation, Shanghai, China). 200 μL fresh buffer was replenished after sampling. The HI-6 concentration in each sample was analyzed using HPLC, as described above.
Animals
All animal experiments complied with the regulations of the Animal Care and Use Ethics Committee of the Beijing Institute of Pharmacology and Toxicology. Animal Center of Academy of Military Medical Sciences provided male Wistar rats (body weight 200 ± 10 g) and Kunming mice (body weight 20 ± 2 g). Animals were maintained in cages at 18–22 °C with controlled humidity (55 ± 6%) and 12 h light-dark cycle, and a standard laboratory food and water were provided. All animals were allowed to adapt for one week before the experiment.
Evaluation of in vitro plasma protein binding
Plasma protein binding was tested in dialysis tubes. 50 μL test sample (free HI-6, LP-HI-6, or PEG-LP-HI-6) was mixed with 1 mL of fresh rat blood, and then added to a dialysis tube. The tube was immersed in PBS (pH 7.4) as the receiving medium and shaken in a thermostat air bath table with 37 °C, 50 rotations/min. At 0,1, 2, 4, 6, 8 h, the received media (0.5 mL) was sampled and analyzed by HPLC. The same volume of fresh media was replenished after every sampling. Each group contained three duplicate samples.
Pharmacokinetic study
LP-HI-6, PEG-LP-HI-6 (equivalent to 20 mg/kg HI-6), and free HI-6 (20 mg/kg) were intravenously administered to Wistar rats (200 ± 10 g, n = 6). Blood samples (0.3 mL) were collected into heparin-treated tubes at 0, 0.02, 0.17, 0.33, 0.50, 1, 2, 4, 8, and 12 h after the respective treatments. Blood samples were centrifuged at 4500 rpm for 15 min to obtain plasma. Next, 100 μL of each sample was collected and stored at −20 °C until analysis. For plasma HI-6 quantification, the plasma sample was mixed with an equivalent volume of acetonitrile and 2% trifluoroacetic acid, vortexed for 10 min, and centrifuged at 10,000 g for 10 min. The obtained supernatant was analyzed using HPLC as previously described.
Calculation of pharmacokinetic parameters was done using WinNonlin 6.3 software (t1/2, CL, MRT, AUC0-t, and AUC0-∞) and the p value was determined by t-test.
Cerebrospinal fluid (CSF) distribution study
The rats were anesthetized and placed on the operating table in a prone position, after which HI-6, LP-HI-6, and PEG-LP-HI-6 (equivalent to 20 mg/kg HI-6) were intravenously administered to Wistar rats (200 ± 10 g, n = 3). Next, CSF (approximately 0.1 mL) was collected through the foramen occipital magnum using an intravenous needle at predetermined intervals (0.17, 0.5, 1, 2, and 4 h). For CSF HI-6 quantification, the CSF sample was mixed with PBS at an equivalent volume, vortexed for 5 min, and centrifuged at 6000 g for 10 min. The supernatant obtained was analyzed using HPLC.
In vivo treatment study
Kunming mice (20 ± 2 g) were used to establish a soman intoxication model to evaluate the treatment efficacy. After 1 min of poisoning by soman (240 µg/kg, intraperitoneally), saline, HI-6 solution, LP-HI-6, and PEG-LP-HI-6 (equivalent to 20 mg/kg HI-6) were intravenously administered to mice in each group. The ratio of the number of surviving mice after exposure to soman to the total number of experienced mice was used as a criterion for the toxicity study.
Statistical analysis
Graphpad Prism (9.0.1) was used to analyze the data. The experimental results were analyzed by t-test, p < 0.05 was considered statistically significant between the two groups.
Results
Preparation and characterization of HI-6-loaded liposomes
LP-HI-6 and PEG-LP-HI-6 were prepared using the thin-film hydration method. The DLS results are shown in , including the average diameter, polydispersity index, zeta potential, encapsulation efficiency (EE%) and loading efficiency (LE%). The hydrodynamic diameters of all the groups ranged from 100 to 140 nm, and the PDI values were less than 0.15. The zeta potential results indicate that both LP-HI-6 and PEG-LP-HI-6 were negatively charged. The LE% increased when the D/L ratio increased, but the EE% was significantly reduced at a 20% D/L ratio. Based on the current results, a 10% D/L ratio was set as the optimal formulation of liposomes, and the results of the morphological characteristics and physical stability are presented in .
Figure 1. Size distributions, morphology characteristics and physical stability of optimal formulations of LP-HI-6 and PEG-LP-HI-6. Size distributions of LP-HI-6 (A) and PEG-LP-HI-6 (B); TEM photographs of LP-HI-6 (C) and PEG-LP-HI-6 (D); average size changes of LP-HI-6 and PEG-LP-HI-6 (E); ζ-potential changes of LP-HI-6 and PEG-LP-HI-6 (F). Data are shown as mean ± SD (n = 3).

Table 1. Results of DLS, encapsulation efficiency (EE%) and loading efficiency (LE%).
In vitro drug release profile
The drug release profiles of HI-6 from both LP-HI-6 and PEG-LP-HI-6 were investigated in a phosphate buffer (pH 7.4) (). About 90% of the free drug was released in one hour; for LP-HI-6, approximately 80% cumulative drug release was achieved at the same time. Compared with other groups, PEG-LP-HI-6 had a slower drug release rate and showed a prolonged drug release profile, that approximately 75% cumulative drug release achieved at the same time point.
In vitro plasma protein binding
The in vitro plasma protein-binding results are shown in . In the first hour, the cumulative release of the HI-6 water solution group reached almost 100%, suggesting that free HI-6 could be transferred into PBS media very quickly. For the HI-6-plasma group, the cumulative drug release was approximately 40–65% within 8 h, which was significantly lower than that of the HI-6 solution.
Pharmacokinetic study
The time-dependent concentrations of free HI-6, LP-HI-6, and PEG-LP-HI-6 in the plasma at various times following intravenous administration (20 mg/kg of HI-6) were studied using male Wistar rats (). Free HI-6 was rapidly cleared from the bloodstream within 4 h. In comparison, both LP-HI-6 and PEG-LP-HI-6 showed significantly delayed systemic clearance, with detectable HI-6 in plasma up to 12 h. The pharmacokinetic parameters are summarized in . The MRT of LP-HI-6 and PEG-LP-HI-6 were 1.65 times and 2.60 times higher than free HI-6, respectively. Moreover, compared to free HI-6, the AUC of LP-HI-6 and PEG-LP-HI-6 increased by 2.36 times and 4.59 times, respectively.
Figure 4. Concentration of HI-6 in plasma. Free HI-6, LP-HI-6 and PEG-LP-HI-6 were administered at 0 h. All data are presented as mean ± SD, n = 6.

Table 2. Pharmacokinetic results.
Cerebrospinal fluid (CSF) distribution
The concentration of HI-6 in CSF was evaluated to study the intracerebral delivery abilities of LP-HI-6 and PEG-LP-HI-6 as shown in . In the free HI-6 group, rapid drug clearance was observed in CSF. In contrast, the time-dependent concentrations of HI-6 loaded into LP and PEG-LP were significantly higher than those of free HI-6 at all-time points. Both the LP-HI-6 and PEG-LP-HI-6 groups reached the highest concentration in the CSF at approximately 30 min.
In vivo treatment study
To test the in vivo efficacy of HI-6-loaded LP and PEG-LP against soman poisoning, we used a Kunming mouse model of soman toxicity. showed the survival numbers within 20 minutes, and showed the number of mice that still survived at 1 h in each group. All mice in the saline group exhibited severe convulsions and respiratory depression and died within 10 min. Administration of HI-6 had limited treatment effects, as it prolonged the maximum survival time to approximately 15 min. LP-HI-6 and PEG-LP-HI-6 showed stronger detoxification, as the death rate in 20 min was lower than that in other groups. At 1 h, three mice survived in the LP-HI-6 group, while six mice survived in the PEG-LP-HI-6 group.
Table 3. Number of mice survived at 1 h.
Discussion
Organophosphorus chemical nerve agents are the most toxic substances around the world. Their strong, fast neurotic toxicity makes it very difficult to prevent and cure. Therefore, on-site first-aid measures play an important role in improving the survival rate and recovery after intoxication. Oxime antidotes are among the therapeutic drug group that can effectively revive phosphorylated AChE and restore its original function. The common oxime antidotes include pralidoxime iodide, pyraloxime methylchloride, obidoxime, HI-6 and MMB-4. HI-6 has been proven to be an effective AChE reactivator with a wide spectrum of therapeutic activity, especially for soman, GF, and VR. However, pharmacokinetic studies have revealed rapid plasma clearance of HI-6, which means that HI-6 is not suitable for on-site first aid of OP poisoning as a one-shot injection. The slow intravenous infusion may maintain a stable drug concentration, but this method is difficult to achieve in the absence of adequate medical personnel and equipment, especially during war.
Liposomes are spherical concentric vesicles composed of the phospholipid bilayer. Their characteristics of drug-carrying capacity and biocompatibility make liposomes one of the best drug carriers for therapeutics. Additionally, liposomes could protect the encapsulated drugs from enzymatic attacks and premature decomposition, improving the stability of drugs in vivo environments. A major obstacle for liposomes is their recognition and sequestration by the phagocytes of the reticuloendothelial system, which promoted the new generation of long-circulating formulation, PEGylated liposomes. By adding polyethylene glycol derivatives of phosphatidyl ethanolamine in the structure, liposomes are not readily taken up by macrophages in the reticuloendothelial system and hence remain in circulation for a relatively long period (Deodhar & Dash, Citation2018). Drug-loaded PEGylated liposomes could be prepared as liquid or dry powder for injection, for example, Doxil® and Lipodox®. Because of its portability and prolonged drug retention time, this system is ideal for on-site first aid for OP poisoning.
In this study, the formulation of HI-6-loaded PEGylated liposomes was optimized. The drug to lipids ratio was a major factor affecting the formulation. Increasing the drug-to-lipid ratio could increase the drug-loading efficiency, however, the diameters and zeta potentials of PEG-LP-HI-6 and LP-HI-6 would change a lot; at the same time, the encapsulation efficiency decreased significantly. Therefore, a 10% D/L ratio was finally determined as the optimized formulation. TEM results presented that liposomes had intact spherical morphology, and DLS results suggested uniform particle size distribution and well stability of formulations. The in vitro drug release showed that liposome formulations could prolong the drug release by encapsulation of HI-6 molecular inner the phospholipid bilayer. Thus, compared with free HI-6, liposomes could offer protection against the enzymatic attack and improve the chemical stability in vivo.
According to the pharmacokinetic results, compared with free HI-6 and LP-HI-6, the intravenous injection of PEG-LP-HI-6 significantly extended t1/2, MRT, and improved the AUC of HI-6 in vivo. Notably, drug concentrations in the CNS, including the brain tissue and CSF, also increased after the intravenous administration of PEG-LP-HI-6. Considering that in vitro studies suggested protein binding of HI-6 in plasma, the mechanism might be that PEG-LP reduced the interaction between HI-6 and endogenous proteins, thus more drugs could pass through the blood–brain barrier in its unbound form.
In a soman intoxication study, intravenous injection of LP-HI-6 or PEG-LP-HI-6 could increase the survival rate compared to a simple HI-6 solution. All groups presented poisoning symptoms in the experiment. In the first 20 min and 1 h after acute poisoning, the survival rate in the PEG-LP-HI-6 group was much higher than that in other groups. The results showed that PEG-LP-HI-6 had a better therapeutic effect than LP-HI-6 and free HI-6 could effectively prolong the survival time of poisoned animals.
Although the treatment effect of PEG-LP-HI-6 was obvious within 1 h, it was also found that the animal mortality rate was still more than 60% after 48 h without follow-up intervention. Therefore, supportive treatment after poisoning is still necessary, and the main role of PEG-LP-HI-6 is to obtain a longer response time for comprehensive treatment for poisoning. Although the treatment effect is limited, the PEG-LP system still makes sense because every extra living minute is vital to victims that suffered from nerve agent poisoning, and every extra living minute increases the possibility of successful treatment.
Conclusion
In this study, PEG-LP-HI-6 was prepared to demonstrate the possibility of it as a first aid formulation on the soman poisoning model. The results showed that the protection from PEGylated liposomes could effectively prolong the circulation time of HI-6 in vivo and the distribution of drugs in the CSF was also increased. Compared with free drugs, the survival time of animals can be significantly prolonged if PEG-LP-HI-6 is given immediately after soman poisoning.
At present, the spectrums of oxime antidotes are quite different, thus in the case of unknown nerve agent poisoning, the use of a single-component formulation for treatment has a certain risk of low efficiency or ineffectiveness. Multi-drug delivery might be an alternative strategy to stress the problem, however, because drugs often have complex interactions, this hypothesis still needs to be carefully verified by experiments.
Ethical approval
All animal experiments complied with the regulations of the Animal Care and Use Ethics Committee of the Beijing Institute of Pharmacology and Toxicology.
= | Abbreviations: LP-HI-6 | |
HI-6-loaded liposomes | = | OPs |
Organophosphorus agents | = | HPLC |
high-performance liquid chromatography | = | LE% |
drug-loading efficiency | = | EE% |
encapsulation efficiency | = | TEM |
transmission electron microscopy | = | AChE |
acetylcholinesterase | = | CNS |
central nervous system | = | PNS |
peripheral nervous system | = | PEG-LP-DDS |
PEGylated liposome drug delivery system | = |
Acknowledgments
We thank Dr. Ying-Jie Zhu for her helpful comments and suggestions.
Data availability statement
The datasets used or analyzed during the current study are available from the corresponding author on reasonable request.
Disclosure statement
The authors report no conflicts of interest in this work.
Additional information
Funding
References
- Acharya J, Dubey DK, Srivastava AK, Raza SK. (2011). In vitro reactivation of sarin-inhibited human acetylcholinesterase (AChE) by bis-pyridinium oximes connected by xylene linkers. Toxicol in Vitro 25:251–6.
- Allen TM, Hansen CB, de Menezes DEL. (1995). Pharmacokinetics of long-circulating liposomes. Adv Drug Delivery Rev 16:267–84.
- Cassel GE, Fosbraey P. (1996). Measurement of the oxime HI-6 after peripheral administration in tandem with neurotransmitter levels in striatal dialysates: effects of soman intoxication. J Pharmacol Toxicol Methods 35:159–66.
- Dadparvar M, Wagner S, Wien S, et al. (2014). Freeze-drying of HI-6-loaded recombinant human serum albumin nanoparticles for improved storage stability. Eur J Pharm Biopharm 88:510–7.
- Deodhar S, Dash AK. (2018). Long circulating liposomes: challenges and opportunities. Ther Deliv 9:857–72.
- Diauudin FN, Rashid JIA, Knight VF, et al. (2019). A review of current advances in the detection of organophosphorus chemical warfare agents based biosensor approaches. Sens Bio-Sens Res 26:100305.
- Gupta B, Ghosh KK. (2013). Evaluation of biological efficiency of oxime based reactivators against organophosphate inhibited AChE: an in vitro study. Toxicol Lett 221:S147–S148.
- Hulse EJ, Haslam JD, Emmett SR, Woolley T. (2019). Organophosphorus nerve agent poisoning: managing the poisoned patient. Br J Anaesth 123:457–63.
- John H, Thiermann H. (2021). Poisoning by organophosphorus nerve agents and pesticides: an overview of the principle strategies and current progress of mass spectrometry-based procedures for verification. J Mass Spectrom Adv Clin Lab 19:20–31.
- Kloske M, Witkiewicz Z. (2019). Novichoks – the A group of organophosphorus chemical warfare agents. Chemosphere 221:672–82.
- Koplovitz I, Stewart JR. (1994). A comparison of the efficacy of HI6 and 2-PAM against soman, tabun, sarin, and VX in the rabbit. Toxicol Lett 70:269–79.
- Kovarik Z, Macek N, Sit RK, et al. (2013). Centrally acting oximes in reactivation of tabun-phosphoramidated AChE. Chem Biol Interact 203:77–80.
- Kuca K, Cabal J, Jun D, et al. (2010). Reactivation of VX-inhibited AChE by novel oximes having two oxygen atoms in the linker. Environ Toxicol Pharmacol 30:85–7.
- Ligtenstein DA, Kossen SP. (1983). Kinetic profile in blood and brain of the cholinesterase reactivating oxime HI-6 after intravenous administration to the rat. Toxicol Appl Pharmacol 71:177–83.
- Lundy PM, Hill I, Lecavalier P, et al. (2005). The pharmacokinetics and pharmacodynamics of two HI-6 salts in swine and efficacy in the treatment of GF and soman poisoning. Toxicology 208:399–409.
- Marjan JM, Allen TM. (1996). Long circulating liposomes: past, present and future. Biotechnol Adv 14:151–75.
- Meads KL, Thomas TP, Langston JL, et al. (2021). Evaluation of adenosine A1 receptor agonists as neuroprotective countermeasures against Soman intoxication in rats. Toxicol Appl Pharmacol 416:115466.
- Myhrer T, Mariussen E, Aas P. (2018). Development of neuropathology following soman poisoning and medical countermeasures. Neurotoxicology 65:144–65.
- Pinho JO, da Silva IV, Amaral JD, et al. (2021). Therapeutic potential of a copper complex loaded in pH-sensitive long circulating liposomes for colon cancer management. Int J Pharm 599:120463.
- Reymond C, Jaffre N, Taudon N, et al. (2018). Superior efficacy of HI-6 dimethanesulfonate over pralidoxime methylsulfate against Russian VX poisoning in cynomolgus monkeys (Macaca fascicularis). Toxicology 410:96–105.
- Singh A, Ujjwal RR, Naqvi S, et al. (2022). Formulation development of tocopherol polyethylene glycol nanoengineered polyamidoamine dendrimer for neuroprotection and treatment of Alzheimer disease. J Drug Target 30:777–91.
- Sun W-X, Zhang C-T, Yu X-N, et al. (2022). Preparation and pharmacokinetic study of diosmetin long-circulating liposomes modified with lactoferrin. J Funct Foods 91:105027.