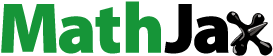
Abstract
The clinical diagnosis and treatment of malignant bone tumors are still major clinical challenges due to their high incidence are difficulty. Targeted therapies have become a critical approach to treat bone tumors. In recent years, radiopharmaceuticals have been used widely and have shown potent and efficient results in treating bone tumors, among which 32P and the labeled radiopharmaceuticals play an essential role. In this study, the 32P-labeled hydroxyapatite (HA) was prepared through chemical synthesis (32P-Hap) and physical adsorption (32P-doped-Hap). The in vitro stability of 32P-labeled HA was analyzed to assess the superiority of the new-found chemical synthesis. The radiolabeling yield and stability of chemical synthesis (97.6 ± 0.5%) were significantly improved compared with physical adsorption (92.7 ± 0.4%). Furthermore, the CT results corroborate that 32P-Hap (100 μCi) +DOX group has the highest tumor suppression rate and can effectively reduce bone destruction. The results corroborate the effectiveness of the chemical synthesis and validate the application of 32P-Hap in bone tumors. Therefore, 32P-Hap (100 μCi) + DOX may be an effective strategy for bone metastasis treatments.
1. Introduction
In recent studies, radiolabeled particulate materials have been widely applied to solid tumor treatment. Radionuclides with short-range and high-energy emissions, such as 32P (T½ = 14.3 d), 90Y (T½ = 64.1 h), and 131I (T½ = 8.04 d), are preferred to accurately target into organs of the main β-emitting tumor (Vimalnath et al., Citation2015; Rial et al., Citation2021). However, compared with the most medical radionuclides, 32P has a long half-life, and the activity declines slowly than them over time, which can be maintained a longer time for the therapy of tumor. In addition, since 32P has been adopted in more and more radiotherapy cases with a longer half-time and more practical performance than 90Y and 131I. Its safety and efficacy in tumor treatment have already been reported previously (Rajeswari et al., Citation2016; Yang et al., Citation2020).
HA, a well-performed nanomaterial, has been widely used in dental and biomedical fields for its great biocompatibility and bioactivity (Sun et al., Citation2019; Rial et al., Citation2021; De Lama-Odria et al., Citation2022). The HA is chemically defined as Ca10(PO4)6(OH)2 with a constant Ca/P ratio. In addition, P is a constituent element of hydroxyapatite, once incorporated into the molecular framework, it is highly unlikely that 32P activity would leach out from 32P-labeled hydroxyapatite particles in vivo. Thus, 32P-labeled HA will be a potential therapeutic strategy for bone tumors. Among the various synthetic methods, a straightforward aqueous precipitation route stands out by using CaCl2 and Na3PO4 as starting materials. 32P-labeled HA particles can be obtained by mixing Na2H[32P]PO4 with analytically pure Na3PO4 or NaH2PO4. This method immensely simplifies the preparation of 32P-labeled HA and maintains high radiochemical purity and favorable stability compared with previous reports. The HA has shown an inhibitory effect on various cancer cells, including breast cancer, liver cancer, gastric cancer, and carcinosarcoma cells (De Lama-Odria et al., Citation2022; Kang et al., Citation2022; Xu et al., Citation2022). Excess calcium uptake will cause toxicity in tumor cells, thus inhibiting their growth. In addition, HA is an excellent drug carrier with many advantages (Liu et al., Citation2018; Safi et al., Citation2018; Ying Liu et al., Citation2019; Liu et al., Citation2021). Firstly, its great biocompatibility can avoid adverse reactions between materials and body tissue or blood system (Zhang et al., Citation2017; Banerjee et al., Citation2018; Kavasi et al., Citation2021). Secondly, HA is stable under physiological conditions and has strong adsorption property for its large surface area, which ensures a long cycle time of drugs in body (Pandi & Viswanathan, Citation2015). Finally, appropriate biodegradability ensures that degraded products and drugs released from the core can be absorbed or naturally excluded from the body to exert a therapeutic effect.
This study aimed to synthesize 32P-labeled HA for targeted therapy of bone tumors. The 32P-labeled HA was prepared through chemical synthesis and physical adsorption. The in vitro stability of 32P-labeled HA was analyzed to assess the superiority of the new-found chemical synthesis. Furthermore, the orthotopic bone tumor-bearing mice model was established to investigate if the developed material could effectively treat bone tumors.
2. Materials and methods
2.1. Materials
Sodium dihydrogen phosphate (NaH2PO4), Calcium chloride (CaCl2), Sodium hydroxide (NaOH), Hydroxyapatite (HA), Potassium bromide (KBr), Sodium iodide (NaI), Saline, Na2H[32P]PO4, and NaH2PO4 were purchased from Chengdu Cologne Chemical Reagent Factory (China), Doxorubicin (DOX) were purchased from Aladdin Industrial Co. (Shanghai, China), 1% penicillin-streptomycin and 10% (v/v) fetal bovine serum were purchased from HyClone (Waltham, MA, USA). All other materials and reagents used in the study were of analytical grades.
2.2. Synthesis and characterization of HA
2.2.1. Synthesis
The preparation of acicular HA is as follows. NaH2PO4 solution (0.3 mol/L) was dropped into the same volume of CaCl2 (0.5 mol/L) at 70 °C and stirred at 600 rpm/min. After dripping, the reaction was stirred for another 2 h. During this procedure, the pH value was ensured at 10 using NaOH solution (1 mol/L), and the stoichiometric Ca/P ratio was maintained at 1.67. The mixture was vortexed thoroughly, followed by centrifugation at 1000 rpm for 5 min. After the supernatant was removed, the precipitate was washed 3–4 times with distilled water through vortexing and centrifuging. The HA powder was obtained after freeze-drying.
2.2.2. Morphological observation
The morphology of the acicular HA was inspected using a scanning electron microscope (SEM, EVOLS 25, FEI, USA) at an accelerating voltage of 5.0 kV. The samples were coated with gold for 15 s before imaging.
2.2.3. Fourier transform-infrared (FT-IR) analysis
The chemical structure of the acicular HA was characterized by an FT-IR spectrometer (Nicolet 550, USA). The HA was mixed with potassium bromide (KBr) and compressed to a thin pellet for examination with a wavenumber range of 400–4000 cm−1.
2.3. Synthesis of 32P-labeled HA
The 32P-labeled HA was prepared by two different routes. In two cases, the Ca/P ratio was both maintained at 1.67. The chemical synthesis is to mix NaH2PO4 (0.3 mol/L) solution with Na2H[32P]PO4 solution (5–6 mCi 32P) to replace NaH2PO4, and the other steps are the same as the synthesis of HA above. The physical adsorption is accomplished by mixing the prepared HA microparticles and Na2H[32P]PO4 (0.2 mL, 5–6 mCi 32P) in 1 mL saline. Then, the reaction mixture was stirred at 70 °C for 2 h. The obtained 32P-labeled particulates were washed three times with normal saline (1 mL) to remove the free 32P activity. Then, the radiolabeling yield and radiochemical purity of the obtained particulates were determined subsequently for further biological studies.
2.4. Determination of radiolabeling yield and radiochemical purity
The radiolabeling yield was measured before washing off the free 32P activity after the completion of the reaction. Firstly, the reaction mixture was vortexed thoroughly, followed by centrifugation at 1000 rpm for 5 min to stratify. Subsequently, 32P activity of half volume of the supernatant and the remaining supernatant solution together with HA particles were measured by NaI (Tl) detector. After counting, the radiolabeling yield was calculated using the following equation:
X: 32P activity of the half volume of the supernatant solution (after decay-corrected).
Y: 32P activity of the remaining supernatant solution and HA particles (after decay-corrected).
The radiochemical purity of the 32P-labeled HA was determined with the same method after the free 32P activity was removed completely by washing.
2.5. In vitro stability of 32P-labeled HA
The in vitro stability of 32P-labeled HA (32P-Hap and 32P-doped-Hap) microparticles was investigated in 0.9% (w/v) saline to measure the leached activity of 32P from the radiolabeled microparticles. 32P-HA microparticles (∼1 mCi) suspended in normal saline were added to 2 mL normal saline at different pHs of 5.0, 6.8, and 7.2 and stored at room temperature. The radiochemical purity of 32P-labeled HA obtained by two methods was repeatedly determined at the end of the time intervals of day 1, day 2, day 4, day 7, and day 14.
2.6. Animal experiments
2.6.1. Orthotopic bone metastasis model
The orthotopic bone tumor-bearing mice model was established to confirm the antitumor efficacy of 32P-labeled HA. The guidelines for animal experiments were followed in accordance with the Institutional Animal Care and Use Committee of Chongqing University Cancer Hospital, China. Balb/c mice (female, 6–8 weeks old) purchased from Spitford Biotechnology Co., Ltd, were raised at room temperature (22–25 °C, light/dark cycle) with free access to food and water. Human breast cancer cell line 4 T1 used for establishing bone model was grown in RPMI 1640 medium supplemented with 1% penicillin-streptomycin and 10% (v/v) fetal bovine serum supplemented at 37 °C in an atmosphere of 5% CO2, which was provided by Chinese Academy of Sciences Cell Bank.
To establish an orthotopic bone tumor-bearing mice model, 6 weeks Balb/c mice were inoculated in their cavum medulla with 4 T1 cells (104 cells/10 μL) suspended in 50% (v/v) Matrigel. Their body weight and tumor volume were monitored every other day. The volume of the tumors was calculated according to the following equation:
2.6.2. Antitumor efficacy evaluation
The tumor-bearing mice were randomly assigned to 7 groups for different treatments when the volume of tumors reached approximately 200 mm3. Saline, doxorubicin (DOX), and different doses of 32P-labeled HA microparticles, including 32P-doped-Hap (100 μCi), 32P-Hap (50 μCi), 32P-Hap (100 μCi) 32P-Hap (150 μCi), and 32P-Hap (100 μCi) +DOX, were injected into the site of the tumor by two methods. The treatment was repeated every 3 days for 3 weeks, and tumor volume and body weight were measured and recorded every other day. The mice were sacrificed with overdose of isoflurane 1 day after the last injection, and tumor tissues were weighed and photographed. The tumor burden was calculated as follows:
W0: The weight of the tumor in the control group.
W1: The weight of the tumor in each treatment group.
3. Results and discussion
3.1. Characterization of HA
In this study, NaH2PO4 and CaCl2 were used as phosphorus and calcium sources to synthesize HA with a Ca/P ratio of 1.67. The morphology and microstructure of HA were observed on an SEM. As shown in , the achieved particles are rod-shaped. The FT-IR spectra of HA are displayed in . The characteristic absorption peak observed at 565, 605, and 1040 cm−1 corresponds to the bending and stretching vibration of PO43- of HA. The lattice OH- group has bending deformation at 3440 and 605 cm−1. The FT-IR data are consistent with the reported data, confirming that the product is HA.
3.2. Radiolabeling of 32P-labeled HA microparticles
In this study, the chemical synthesis (32P-Hap) and the physical adsorption (32P-doped-Hap) were used to prepare 32P-labeled HA to achieve maximum yield and excellent stability. The chemical synthesis exhibited higher radiolabeling yield and better stability than the common physical adsorption. NaH2PO4 and Na2H[32P]PO4 solutions were mixed to replace the NaH2PO4 in the synthesis of 32P-Hap. 32P was linked to the HA by covalent bonds, and thus the radiolabeling yield and stability were significantly improved. The yield of chemical synthesis (97.6 ± 0.5%) was higher than that of physical adsorption (92.7 ± 0.4%).
3.3. In vitro stability of 32P-labeled HA
The in vitro stability was investigated at different pHs of 5.0, 6.8, and 7.2 in saline. The results showed that pH had little effect on stability. 32P-Hap showed better stability than 32P-doped-Hap up to 14 days saline at room temperature. displays the radiochemical purity at each time interval of 32P-Hap and 32P-doped-Hap, respectively. After 14 days, the stability of 32P-Hap remained above 96% with a decrease of about 1.69%, while that of 32P-doped-Hap was only about 80% with a decrease of about 3.53%. The HA particles obtained by the physical adsorption were unstable and dissolved slowly.
3.4. In vivo antitumor efficacy
A 4T1 breast cancer mice model was established, and the antitumor efficacy of nano HA was started to be tested in vivo when the tumor volume reached approximately 200 mm3. We randomly divided the mice into 7 groups: saline, DOX, 32P-doped-Hap (100 μCi), 32P-Hap (50 μCi), 32P-Hap (100 μCi), 32P-Hap (150 μCi), and 32P-Hap (100 μCi) + DOX groups.
The volume of tumors within the specified time in each group is shown in . The results indicated a low tumor inhibition rate of the DOX and 32P-doped-Hap (100 μCi) compared with saline. Furthermore, the effect of 32P-Hap (50 μCi) and 32P-Hap (100 μCi) on tumor volume reduction was not significant. In comparison, it was displayed that the tumor volume was decreased compared with the saline, DOX, and 32P-doped-Hap (100 μCi), due to their good tumor targeting capacity. The 32P-Hap (100 μCi) +DOX group exhibited the highest tumor suppressive effect and almost stopped the tumor progression. The body weight of mice was measured at various periods in the therapy. The results demonstrated no significant change in body weight between the saline and the 32P-Hap (100 μCi) +DOX groups, indicating that the mice were in a normal state during the treatment in .
Figure 3. In vivo antitumor effect of 32P-Hap conjugates on bone tumor-bearing Balb/c model (female, 6–8 weeks old). Relative tumor volume (A) and body weight (B) and representative images of mice (C) and the xenograft tumors harvested form the mice (D) and weights of the harvested xenograft tumors (E) and tumor inhibition rate (F).

In addition, the tumors in the tibia of mice after treatments by different approaches and the representative images of tumors removed from the tibia of mice are shown in , respectively. The 32P-Hap (100 μCi) +DOX group has the highest tumor suppression rate, possibly due to the combination of DOX with radiotherapy. The weight of tibial tumors in mice at the end of treatment is shown in . Compared with the saline group, the tumor weight was significantly decreased in other groups, with the greatest decrease in 32P-Hap (100 μ Ci) + DOX group. Furthermore, tumor inhibition rates are shown in to evaluate the effect of antitumor after treatment. 32P-Hap (100 μCi) + DOX group exhibited the highest tumor inhibition rate (80.06%). The tumor inhibition rates in the 32P-Hap (100 μCi) (63.53%) and 32P-Hap (150 μCi) (69.93%) groups were higher than those in saline (17.36%) and DOX (26.99%) groups. Therefore, the growth of tumors can be significantly inhibited by 100 μCi 32P treatment, and tumor inhibition can be further enhanced by the DOX treatment.
In vivo antitumor effects of various groups of nano-HA can be observed through TUNEL and H&E staining. The results are shown in . The TUNEL results indicated that the number of apoptotic tumor cells in 32P-Hap (150 μCi), 32P-Hap (100 μCi) +DOX groups increased significantly compared with saline, DOX, 32P-doped-Hap (100 μCi), 32P-Hap (50 μCi), 32P-Hap (100 μCi), and 32P-Hap (150 μCi) groups. The apoptotic cells increased most after radio-chemotherapy. Furthermore, the tumor and each major organ were stained with H&E to evaluate the toxicity of each group after treatment. The results revealed that the staining of tumor sections displayed a similar regulation trend to TUNEL, and no lesioned tissue was found in the other groups. No local failure of cardiomyocytes, the morphologically intact hepatocytes without inflammatory cell accumulation, and no lesions in tissues (e.g. kidney congestion) were found.
3.5. Bone destruction assessment with CT
The incidence rate of malignant bone tumors is high, and bone tumors have a significant impact on the health of patients. The stimulated osteoclasts during the development of bone tumors lead to pathological bone destruction, which seriously affects the quality of life of patients. In this study, the skeletal morphology of tumor-bearing tibias after various treatments was further appraised using CT. As shown in , the tumor-bearing site of mice leg tibia in Saline, DOX, groups are the most severely damaged owing to inefficient tumor suppression. However, the tumor suppression rates in 32P-doped-Hap (100 μCi), 32P-Hap (50 μCi), and 32P-Hap (100 μCi) groups are higher than those in saline and DOX groups. Furthermore, the structure of the mice tibia is intact in 32P-Hap (150 μCi) group, and partial corrosive lesions can still be observed. The complete structure of tumor-bearing tibias of mice in 32P-Hap (100 μCi) +DOX indicates that the treatment can effectively reduce bone resorption and efficiently protect the skeletal structure of bone from damage in bone tumors.
4. Discussion
Developing effective new drugs to cure cancer is a clinical challenge. In recent years, radiopharmaceuticals have become popular anticancer drugs. Most radionuclides are mainly used for to label materials. Nanomaterials have also attracted great attention in the biomedical field. HA is a biological material, and its chemical composition is similar to bone minerals. It has been applied widely in the medical field due to its excellent biocompatibility and bone tissue regeneration (Wijesinghe et al., Citation2014; Santos et al., Citation2017). These nanoparticles can be easily attached to osteosarcoma and osteoblast and can accelerate the growth of osteoblasts and the intake of osteosarcoma cells. In addition, the mammalian cells can be killed by the β-particles of radionuclide; 131I is preferred for thyroid carcinoma postoperative treatment (Pandi & Viswanathan, Citation2015), which has been used for decades; 90Y is marked of auxin to therapy the neuroendocrine tumor (Lorenzoni et al., Citation2018; Lawhn-Heath et al., Citation2021). 32P is suitable for treating tumors because of the long half-life nuclides.
The chemical synthesis has been proved as a promising candidate for synthesizing 32P-labeled HA particles with high radiochemical purity and stability in a simplified 32P labeling process. In addition, the 32P is a promising radionuclide for bone tumors (Yang et al., Citation2020), and DOX, also known as Adriamycin, is widely used to treat various cancers (Elsherbiny et al., Citation2016). Therefore, the 32P-Hap-DOX may be a potential drug for the targeted treatment of bone tumors. The radio-chemotherapy exhibits the most outstanding antitumor effect compared with other groups because the specific activity of radionuclide at the tumor site can emit radiation to kill tumor cells. Internal irradiation therapy has the shortest treatment time and the lowest risk of recurrence.
In this study, the 32P-labeled HA particles were obtained by physical adsorption and chemical synthesis, respectively, and a new labeling method for HA was constructed to treat bone metastases. Chemical synthesis has a higher radiolabeling rate and stability than physical adsorption. Additionally, 32P-Hap (100 μCi) + DOX has the strongest tumor suppression in vivo and effectively prevents bone destruction. This study demonstrates that chemical synthesis can improve the labeling efficiency of HA, and 32P-Hap (100 μCi) + DOX may be an effective strategy for bone metastasis treatments.
5. Conclusion
In summary, we have constructed a new labeling method for HA, which the goal of evaluation its utility as a promising candidate for the treatment of bone tumors has been successfully demonstrated. Our data demonstrated that it is possible to create stable nanosystems, which has a superior effect against bone cancer cells when compared with the physical synthesis, the radiolabeling rate and stability increased significantly. Also, the new labeling method can promote the development of radiopharmaceuticals.
Ethical approval statement
The guidelines for animal experiments were approved in accordance with the Institutional Animal Care and Use Committee of Chongqing University Cancer Hospital, China.
Acknowledgements
The authors acknowledgement the financial assistance provided by the Research Fund Program of Chongqing Key Laboratory of Translational Research for Cancer Metastasis and Individualized Treatment (CMIT201801).
Disclosure statement
No potential conflict of interest was reported by the authors.
Additional information
Funding
References
- Banerjee S, Bagchi B, Bhandary S, et al. (2018). Antimicrobial and biocompatible fluorescent hydroxyapatite-chitosan nanocomposite films for biomedical applications. Colloids Surf B Biointerfaces 171:1–7.
- De Lama-Odria MDC, Del Valle LJ. Puiggali (2022). Hydroxyapatite biobased materials for treatment and diagnosis of cancer. Int J Mol Sci 23.
- Elsherbiny NM, Younis NN, Shaheen MA, et al. (2016). The synergistic effect between vanillin and doxorubicin in ehrlich ascites carcinoma solid tumor and MCF-7 human breast cancer cell line. Pathol Res Pract 212:767–77.
- Kang NW, Lee JY, Kim DD. (2022). Hydroxyapatite-binding albumin nanoclusters for enhancing bone tumor chemotherapy. J Control Release 342:111–21.
- Kavasi RM, Coelho CC, Platania V, et al. (2021). In vitro biocompatibility assessment of nano-hydroxyapatite. Nanomaterials (Basel) 11:1152.
- Lawhn-Heath C, Fidelman N, Chee B, et al. (2021). Intraarterial peptide receptor radionuclide therapy using (90)Y-DOTATOC for hepatic metastases of neuroendocrine tumors. J Nucl Med 62:221–7.
- Liu Y, Wu Y, Lin H, et al. (2018). Study on an injectable biomedical paste using cross-linked sodium hyaluronate as a carrier of hydroxyapatite particles. Carbohydr Polym 195:378–86.
- Liu Y, Raina DB, Sebastian S, et al. (2021). Sustained and controlled delivery of doxorubicin from an in-situ setting biphasic hydroxyapatite carrier for local treatment of a highly proliferative human osteosarcoma. Acta Biomater 131:555–71.
- Lorenzoni A, Capozza A, Artale S, et al. (2018). Impressive response to tandem treatment with [90Y]DOTATOC and [177Lu]DOTATOC in grade 3 pancreatic neuroendocrine carcinoma. Clin Nucl Med 43:506–8.
- Pandi K, Viswanathan N. (2015). In situ precipitation of nano-hydroxyapatite in gelatin polymatrix towards specific fluoride sorption. Int J Biol Macromol 74:351–9.
- Rajeswari A, Vimalnath KV, Sarma HD, et al. (2016). Hydroxyapatite (HA) microparticles labeled with (32)P - A promising option in the radiation synovectomy for inflamed joints. Appl Radiat Isot 116:85–91.
- Rial R, Gonzalez-Durruthy M, Liu Z, et al. (2021). Advanced materials based on nanosized hydroxyapatite. Molecules 26:3190.
- Safi S, Karimzadeh F, Labbaf S. (2018). Mesoporous and hollow hydroxyapatite nanostructured particles as a drug delivery vehicle for the local release of ibuprofen. Mater Sci Eng C Mater Biol Appl 92:712–9.
- Santos C, Gomes P, Duarte JA, et al. (2017). Development of hydroxyapatite nanoparticles loaded with folic acid to induce osteoblastic differentiation. Int J Pharm 516:185–95.
- Sun J, Wu T, Fan Q, et al. (2019). Comparative study of hydroxyapatite, fluor-hydroxyapatite and Si-substituted hydroxyapatite nanoparticles on osteogenic, osteoclastic and antibacterial ability. RSC Adv 9:16106–18.
- Vimalnath KV, Chakraborty S, Rajeswari A, et al. (2015). Radiochemistry, pre-clinical studies and first clinical investigation of 90Y-labeled hydroxyapatite (HA) particles prepared utilizing 90Y produced by (n,gamma) route. Nucl Med Biol 42:455–64.
- Wijesinghe WP, Mantilaka MM, Premalal EV, et al. (2014). Facile synthesis of both needle-like and spherical hydroxyapatite nanoparticles: effect of synthetic temperature and calcination on morphology, crystallite size and crystallinity. Mater Sci Eng C Mater Biol Appl 42:83–90.
- Xu K, Wang Y, Xie Y, et al. (2022). Anti-melanoma effect and action mechanism of a novel chitosan-based composite hydrogel containing hydroxyapatite nanoparticles. Regen Biomater 9:rbac050.
- Yang X, Zhai D, Song J, et al. (2020). Rhein-PEG-nHA conjugate as a bone targeted drug delivery vehicle for enhanced cancer chemoradiotherapy. Nanomedicine 27:102196.
- Ying Liu YT, Tian Y, Wu J, et al. (2019). Gadolinium-doped hydroxyapatite nanorods as T1 contrast agents and drug carriers for breast cancer therapy. ACS Appl Nano Mater 2:1194–201.
- Zhang YG, Zhu YJ, Chen F, et al. (2017). Biocompatible, ultralight, strong hydroxyapatite networks based on hydroxyapatite microtubes with excellent permeability and ultralow thermal conductivity. ACS Appl Mater Interfaces 9:7918–28.