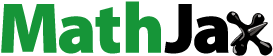
Abstract
Traditional eye drops used for topically administering drugs have poor ocular bioavailability due to the biological barriers of the eye. There is an interest to design and develop novel drug delivery systems that would extend the precorneal residence time, reduce the frequency of administration and decrease dose-related toxicity. This study aimed to prepare Nanoparticles of Gemifloxacin Mesylate and incorporate them into an in situ gel. The nanoparticles were prepared by ionic gelation technique, using 32 factorial design. Sodium tripolyphosphate (STPP) was used to crosslink Chitosan. The optimized formulation of the nanoparticles (GF4) contained 0.15% Gemifloxacin Mesylate, 0.15% Chitosan and 0.20% STPP, producing 71 nm particle size and 81.11% entrapment efficiency. The prepared nanoparticles showed biphasic release, with an initial burst release of 15% in 1.0 hr and a cumulative drug release of 90.53% at the end of 24 hrs. After that, the prepared nanoparticles were incorporated into an in situ gel, using Poloxamer 407, producing a sustained drug release with efficient antimicrobial activity against gram-positive and gram-negative bacteria as confirmed by the cup plate method.
1. Introduction
Ophthalmic/Ocular drug delivery (ODD) is a challenging problem faced by the pharmaceutical scientists. The major issues encountered with this drug delivery system is its poor bioavailability, rapid precorneal loss due to nasolacrimal drainage, and the high tear fluid turnover, causing that only 10% of the drug concentration available at the site of action (Almeida et al., Citation2014a; Pandey et al., Citation2021).
In-situ gels refer to polymeric systems that are topically applied as solutions or suspensions. They can undergo transition from a sol to a gel phase as they are exposed to ocular physiological conditions, such as temperature (Poloxamer hydrogel), pH (pseudo latexes) or in the presence of ions (sodium alginate) (Kirchhof et al., Citation2015; Paradkar and Parmar, Citation2017; Wang & Han, Citation2017). The liquid state of these systems has many advantages, such as the ease of administration, reproducibility, and the simplicity of manufacturing process. Regarding the gel state system, it has promising benefits in terms of sustained drug release, increasing ocular retention time and mucoadhesion (Abbas et al., Citation2022). It was reported that when the precorneal residence time of these drugs increased, the availability of drug at the corneal surface could significantly be improved. Previous studies had indicated that when nanoparticle forms of drug had been administered, an obvious increase in the drug absorption along with a decrease in the frequency of administration. This had the effect to decrease dose related toxicity (Destruel et al., Citation2020). In addition, it was found that the combination of in situ gel and nanoparticles will yield a promising formulation. Gemifloxacin Mesylate is a fluroquinolone water-soluble therapeutic compound that is used to treat bacterial infections (Rajalakshmi et al., Citation2013). This is because the Gemifloxacin is a DNA gyrase and topoisomerase IV inhibitor that binds to bacterial enzymes and prevents DNA replication, causing a cell death (Scoper, Citation2008).
Many studies have proved that the development of nanoparticle-laden in situ gel had the advantage to improve the ocular bioavailability of water-soluble drugs (Imam et al., Citation2018). Thermo-sensitive in situ gels are the most investigated gels since they are widely used as drug delivery systems. This is due to the simplicity of gelation process and availability of a wide range of safe polymers/biomaterials (Almeida et al., Citation2014b; Hamed et al., Citation2020). Poloxamers are nonionic triblock polymers consisting of polyoxy ethylene (PEO), polyoxy propylene (PPO) and polyoxy ethylene chains (PEOn-PPOn-PEOn) (Almeida et al., Citation2013). PPO is the hydrophobic part of the polymer, while PEO represents the hydrophilic part. Poloxamers have the advantages to be nontoxic, due to their inert nature and soluble in both water and organic solvents. When low concentrations of Poloxamers are dispersed in liquid, they act as surfactants, forming micelles. However, when Poloxamers found at higher concentrations, they tend to form multimolecular aggregates and undergo a sol-gel transition (Destruel et al., Citation2017; Bodratti & Alexandridis, Citation2018). The sol-gel transition temperature of different types of Poloxamer can be categorized, depending on the ratio of PEO and PPO (Shubhra et al., Citation2014; Wu et al., Citation2019).
The purpose of the present research was to develop an in situ gel eye drops containing GM nanoparticles using novel polymer Poloxamer 407 for enhancing the therapeutic efficacy and its bioavailability by increasing the retention time in the ocular region. The developed polymeric nanoparticles were optimized using a two-factor at three-level (32) factorial design. After carrying out the compatibility studies of FTIR and DSC, the GM nanoparticles were evaluated for surface morphology, particle size, poly dispersity index, zeta potential, drug entrapment efficiency, % drug loading, in vitro and release kinetics and mechanism of release. Secondly the optimized formulation of GM Nanoparticles were incorporated into in situ gel and evaluated for gelling effect, in vitro release, antimicrobial activity and stability.
2. Materials and Methods
2.1. Materials
Gemifloxacin Mesylate (Indian Pharmacopeial standard), Chitosan with molecular weight (300–2000 kDa) and Poloxamer 407 were purchased from Yarrow Chem products, Mumbai, India. Sodium bicarbonate, Calcium Chloride dihydrate, Sodium Chloride, Benzalkonium Chloride were supplied by Chemdyes Corporation, (Rajkot, India). Design-Expert® software (Design-Expert 11.1.2.0, State-Ease Inc., Minneapolis, USA) was used for factorial design. All other chemicals used were of analytical grade. Dialysis membrane (12000 m.w. cut off) was obtained from Himedia, Mumbai, India. Freeze dried strains of Bacillus subtilis, Klebsiella pneumoniae, Pseudomonas aeruginosa and Staphylococcus aureus were obtained from Institute of Microbial Technology (IMTECH), Chandigarh, India.
2.2. Methods
2.2.1. Preparation of calibration curve of GM
Gemifloxacin Mesylate was quantitatively analyzed by UV-Visible spectrophotometry at λmax of 266 nm using simulated tear fluid (pH 7.4) (STF) with primary stock solution of 100 ppm or 100 µg/mL and working standard of 1 to 5 µg/mL (Gouda et al., Citation2014).
2.2.2. Compatibility studies
2.2.2.1. Fourier Transform Infrared (FTIR)
The FTIR spectra of pure drug GM and physical mixture of GM, Chitosan and Poloxamer 407 were recorded using an FTIR Spectrophotometer (Nicolet iS10 AGILENT, CARY − 630). FTIR analysis was carried out to assess the physicochemical interaction of drug with excipients by Potassium bromide disk method (Wu et al., Citation2005).
2.2.2.2. Differential Scanning Colorimetry (DSC)
Thermal DSC analyzer (Shimadzu, Singapore DSC − 60) was used to obtain DSC thermograms of pure drug Gemifloxacin Mesylate and Physical mixture of Gemifloxacin Mesylate, chitosan and Poloxamer 407. The sample was precisely weighed in an aluminum pan, then crimped with an aluminum cover and heated at a rate of 10 °C per minute from 30.0 °C to 300.0 °C (Hani et al., Citation2022).
2.2.3. Preparation of GM loaded nanoparticles
Nanoparticles were produced by an ionic gelation method which involves reaction between anionic counter ion of sodium tri polyphosphate (STPP) and a cationic amino group of chitosan. In 20 mL of acetic acid solution [1% (v/v)], required quantity of Chitosan was dissolved using mechanical stirrer and added required amount of drug in it (Imam et al., Citation2018). STPP solution of 10 mL was added drop by drop using 24 G Syringe needle to GM (drug) + Chitosan solution with continuous stirring. After 30 min. of stirring, the batches were subjected to sonication process at a pulse rate of 5 cycles for 10 minutes using probe sonicator (Bandelin, Germany). Centrifuged at 17,000 rpm at −4 °C for 15 min using cooling centrifuge (REMI) and collected the supernatant to estimate drug entrapment efficiency. After centrifugation, the bottom-precipitated nanoparticles were collected and subjected to sonication process at a pulse rate of 5 cycles for 10 minutes using probe sonicator.
2.2.4. Composition of preliminary trials by ionic gelation method
All batches contain STPP of 0.2% with GM of 0.15% and Chitosan at a difference of 0.15% starting from 0.3% to 0.9% coded as F1 to F5.
2.2.5. Design of experiment study
Final batch was optimized using 32 Factorial Design with 2-factors, 3-levels and 9 runs by Design-Expert® software (Rahamathulla et al., Citation2021a; Hani et al., Citation2022). In this study, Polymer (Chitosan) concentration (X1), Stirring speed (X2) were chosen as the independent variables. Particle size (Y1), Entrapment efficiency (Y2), and the amount of % cumulative drug release (Y3) were dependent variables. The statistical analysis was performed by one-way ANOVA using Design Expert 11 to determine the impact of formulation factors on the response variables. The design was assessed by a design model, which bears the form of the following equation.
(1)
(1)
Where Y is the dependent variable, b0 is the arithmetic mean of the response from the nine runs, and bi(b1,b2,b12,b11,b22) is the estimated coefficients for the corresponding factor Xi(X1,X2,X12,X22) at which the average results represent changing one factor at a time from its low to high value. The interaction term (X1, X2) represents the changes in response when two factors are simultaneously changed. To determine the nonlinearity, polynomial terms (X11, X22) are included. The effect of chitosan concentration and stirring speed on particle size, drug entrapment efficiency and % cumulative drug release were known from the response surface plots and contour plots using Design-Expert® software 11.
2.2.6. Statistical modelling applied for optimization
In order to determine the dependant variables of particle size (nm), Entrapment efficacy (%) and % cumulative release, the chosen independent variables were Chitosan at concentrations of 0.15%, 0.3% and 0.45% with stirring speeds of 500, 1000 and 1500 rpm as shown in .
Table 1. Factorial design (32).
2.2.7 Characterization of GM loaded nanoparticles
2.2.7.1. Particle size, Polydispersity index and Zeta Potential
The particle size, zeta potential and polydispersity index of the drug loaded nanoparticles were determined using dynamic light scattering method by Malvern nanosizer (Malvern Instruments Ltd, Nano ZS, U.K.) (Bohrey et al., Citation2016).
2.2.7.2. Drug entrapment efficiency
Suspension of nanoparticles was centrifuged (REMI) at 17000 rpm at −4 °C for 15 min. The amount of free drug present in supernatant was suitably diluted with STF and analyzed by UV spectrophotometer (double beam spectrophotometer 1800, Shimadzu, India) at 266 nm. The drug entrapment efficiency of GM in nanoparticles was calculated (Tagalpallewar et al., Citation2021).
2.2.7.3. Percentage (%) drug loading
The percentage of drug loading in the nanoparticle was calculated as per the below equation.
2.2.7.4. In-vitro release studies
The in-vitro drug release of GM loaded-chitosan-nanoparticles was carried out using dialysis bag diffusion method (Bohrey et al., Citation2016). 5 mL of drug loaded nanoparticles were dispersed in a dialysis bag and then kept in a 100 mL beaker containing 50 mL of 7.4 pH dissolution medium (STF). The beaker was placed over a magnetic stirrer and the temperature was maintained at 37 ± 0.5 °C throughout the experiment. During the experiment, the rotation speed was kept constant at 100 rpm. At regular time intervals, samples (1 mL) were withdrawn and replaced with equal amounts of fresh dissolution medium. After appropriate dilutions with STF, the samples were analyzed using a UV Visible spectrophotometer at 266 nm. The measurements were carried out in triplicate.
To determine the release kinetics, the in vitro release data were subjected to Hixon-Crowell, Higuchi, Peppas, first-order and zero-order plots (Tagalpallewar et al., Citation2021).
2.2.8. Scanning Electron Microscopy (SEM)
The particle size and surface morphology of the nanoparticles were examined by SEM (Smart SEM 5.05, Zeiss, EVO LS, Jena, Germany) at different magnification. Sample was placed in gold foil film and subjected to fixation process. Then, gold foil is kept in scanning to determine the isolated particle with smooth surface in spherical shape having uniform coating at acceleration of 15 kV voltage and a 11 KX magnification at room temperature (RT) (Garala et al., Citation2013).
2.2.9. Preparation of in situ gelling polymeric nanoparticles
The in situ gel was prepared by cold method (Tagalpallewar et al., Citation2021). Briefly, 15 mL of distilled water was transferred to 100 mL beaker and Poloxamer 407 was added slowly with continuous stirring (1000 rpm) for 1 h. The temperature was maintained at 4 ± 2 °C during the preparation. Upon cooling, clear solution was obtained. Optimized freeze-dried nanoparticles (equivalent to 0.15%w/v) based on drug loading and release pattern, was suspended in Poloxamer solution. The optimization of in situ gel was carried out by varying the concentration of Poloxamer 407 (9%, 12%, 15%) coded as B1, B2, B3 respectively. Benzalkonium chloride was used as preservative.
2.2.10. Characterization of in situ gelling polymeric nanoparticles
The clarity of the gel was visually inspected under white and black background (Rahamathulla et al., Citation2021b). After the preparation, the pH of each formulation was immediately measured. The rheological properties of the prepared gels were carried out by Brookfield Viscometer using type DV-II + PRO spindle LV 3(63) and 37 °C with varying shear rate.
The gelling capacity of the prepared formulations was evaluated by inserting one drop of the formulation into a vial containing 2 mL of freshly prepared simulated tear fluid (STF) solution. The time required to form the gel and then to get dissolved were noted. Gel strength measurements were carried out in triplicate by evaluating the time (in seconds) required for the weight to penetrate 5 cm of the gel was noted. After that, 10 mL of the formulation was diluted to 100 mL using STF of pH 7.4 and drug content was assessed at 266 nm.
In vitro release study was carried out using dialysis bag with 1.0 mL of in situ gel previously suspended in 10 mL STF. After that, 1.0 mL of the sample was withdrawn every 30 min, with equal replacement of fresh STF, up to 24 hrs, and then sample was analyzed at 266 nm.
The optimized formulation was tested for sterility test according to IP and then carried out antimicrobial activity against Bacillus substilis (MTCC 441), Staphylococcus aureus (MTCC 96), Pseudomonas aeruginosa (MTCC 1688) and Klebsiella pneumoniae (MTCC 3384) against Streptomycin as standard.
Optimized sterile formulation was subjected to stability study as described in ICH guidelines (Hani et al., Citation2021) and estimated the gelling capacity, in vitro release and visual appearance in comparison with 0th day sample.
3. Results and Discussion
The UV absorption maxima (λmax) of the pure drug GM was found to be at 266 nm in STF of pH 7.4. The standard calibration curve of the pure GM was measured at 266 nm using STF. Linear graph was obtained in the concentration 1 to 5 ppm (1 µg–5 µg/mL) obeying Beer Lambert’s law ().
FTIR data of pure drug (GM) shows principle peak of key functional groups such as C-F bending at 1039.9 cm−1, O-CH3 bending at 1453.7 cm−1 R-COOH stretching at 1161.1 cm−1 N-H scissoring at 1630.7 cm−1, aromatic C = O stretching at 1720.2 cm−1 and C-H rocking at 728.7 cm−1. The FTIR data of physical mixture of pure drug (GM), Chitosan and Poloxamer 407 showed wave number peaks C-F bending at 1039.9 cm−1, O-CH3 bending at 1459.3 cm−1, R-COOH stretching at 1157.3 cm−1, N-H scissoring at 1630.7 cm−1, C = O stretching at 1703.4 cm−1, C-H rocking at 730.6 cm−1. In this physical mixture, characteristic peaks of Gemifloxacin Mesylate were retained (). Therefore, it is clear that GM is compatible with polymers of Chitosan and Poloxamer 407 which are to be used in the formulations.
DSC thermogram of GM revealed a distinct exothermic peak at 235.35 °C corresponding to its melting point and it is maintained in physical mixture of GM, Chitosan and Poloxamer 407 which confirmed the compatibility with polymer selected as shown in ().
3.1. Evaluation of preliminary batches of GM loaded nanoparticles
From the preliminary study revealed that, if the chitosan concentration was increased from batch F1 to F5, the particle size also increases in the preliminary study. F1 was selected as best batch on the basis of smaller particle size, higher entrapment efficiency and maximum % release up to 24 hours, as shown in .
Table 2. Evaluation of preliminary batches.
3.2. Evaluation of 32 factorial design batch
Drug release profile of GM loaded Chitosan Nanoparticles showed initial burst release within 1 hr. and sustained for 24 hrs. It was observed that decreasing the concentration of Chitosan and maintaining the stirring speed of 1000 rpm showed the sustained release for longer time. Minimum effective concentration was maintained for 24 hr. to reduce the dosing frequency as shown in .
Table 3. Evaluation of 32 factorial design batch.
3.3. In vitro drug release
The in vitro drug release data reveals that percentage of drug release from the developed polymeric nanoparticle formulations GF1-GF9 shows the controlled release between 81.07% to 93.56% upto 24 h, as shown in . Among all formulation GF4 shows the highest percentage of drug entrapment and 90.53% drug release considered to be optimized formulation.
3.4. Drug loading
The optimized formulation (GF4) showed 0.29 mg of drug per mg of Nanoparticles.
3.5. Kinetic modeling and drug release mechanism
The dissolution profiles were fitted to several models, and the drug release data was evaluated using the Hixon-Crowell, Higuchi, Korsemeyer Peppa’s, first-order and zero-order kinetics as shown in (Rahamathulla et al., Citation2021b).
Table 4. Kinetic modeling
According to Korsmeyer peppa’s equation, if the diffusional exponent (n) is less than 0.45, it is Fickian release (FR). If n value ranges from 0.45 to 0.89, it is Non-Fickian release (nFR) (Anomalous release Type 1) (Tagalpallewar et al., Citation2021). The n values of all formulations (GF1-GF9) were in between 0.588 to 0.841, revealed Non-Fickian release (nFR). Coefficients of correlation (R2) were used to assess the fit’s accuracy ().
3.6. Statistical analysis
Two variables were evaluated at three levels in this design, and experimental trials were conducted across all nine possible combinations ().
The impact of formulation factors on response variables was statistically assessed using one-way ANOVA by design expert. The equation was used to evaluate the design:
(1)
(1)
Where the response variable is Y, b0 is the constant, and the regression coefficients b1 and b2 are given. X1 and X2 represent the main effects, while X12 and X22 represent the interaction terms that show how the response changes when two factors are changed simultaneously.
A numerical optimization procedure based on the desirability approach was used to determine the optimal settings for the formulation variables to produce the desired/target response. The pure error and lack of fit data were compiled in an ANOVA table, which can provide a mean response as well as an estimate of pure experimental uncertainty.
3.6.1. Statistical analysis for particle size (nm) Y1
Statistical analysis was performed on the particle size, % cumulative drug release and % entrapment efficiency data. These three variables were used as dependent variables for the study.
eqn. (1)
eqn. (1)
eqn. (2)
eqn. (2)
The ANOVA for the quadratic response surface model for particle size was found to be significant because the p-value for the model is <0.0001, which is less than 0.05. Both the independent factors have a p-value of <0.05 showing the significant effect on the response variables (Tagalpallewar et al., Citation2021). There was no significant interaction between the factors, as indicated by the interaction p-value of 0.6490. The model fit statistics R2 value was found to be 0.991, adjusted R2 value 0.9981, Predicted R2 value was 0.9933 and Adequate precision was 87.8950. This model suggested to be used to navigate the design space. The afore mentioned Equationequation (2)eqn. (2)
eqn. (2) expresses the quantitative impact of the independent variables on particle size in terms of coded variables. The surface response and contour plot for particle size showed that as the concentration of chitosan increases from low level (−1) to high level (+1) and stirring speed decreases from high level (+1) to low level (−1),an increase in particle size from 50 to 119 nm was obtained, as indicated by positive sign in polynomial equation of X1. In addition, particle size increased with increase in the concentration of chitosan to the higher, causing a significant increase in the viscosity and hence affecting the shear capacity and stirring. The response contour plot and response surface plot for Y1 (Particle size) are shown in .
3.6.2. Statistical analysis for entrapment efficiency (%) Y2
The ANOVA for the quadratic response surface model for % entrapment efficiency was found to be significant because the p-value for the model is <0.0004, which is less than 0.05. Factor A (chitosan concentration) have p-value of 0.0021 showing that factor A had significant effect on the % entrapment efficiency. While p-value for factor B was 0.0007 showing that it has significant effect on the % entrapment efficiency. Interaction p-value was 0.4446, indicating that there was no significant interaction between factors. The model fit statistics R2 value was found to be 0.991, Adjusted R2 value as 0.9797, Predicted R2 value as 0.9068 and Adequate precision was 27.4953 This model suggested to be used to navigate the design space. Above equation represents the quantitative effect of the independent factors on the % entrapment efficiency written in terms of coded factors. Contour & surface response plot for % entrapment efficiency showed that as the concentration of chitosan decreased from high level (+1) to medium level (0) and stirring speed increased from low level (−1) to medium level (0), an increase in the entrapment efficiency occurred as indicated by negative sign in polynomial equation for X1, and positive sign in polynomial equation for X2. This is because after one point from medium level (0) to High level (+1) of stirring speed, the particle size gets reduced and surface area increased. In addition, due to high concentration of polymer the viscosity of polymer increased and particles becomes rigid due to higher cross linking, which hindered the entrapment of drug inside the nanoparticles (Rahamathulla et al., Citation2021b). The response contour plot and response surface plot for Y2 (% entrapment efficiency) are shown in .
3.6.3. Statistical analysis for % cumulative drug release Y3
ANOVA for quadratic response surface model for % cumulative drug release was found to be significant as p-value for the model is <0.0001 which is ˂0.05. Factor A (Chitosan concentration) has p-value of <0.0001 showing that factor A has significant effect on the % cumulative drug release. While p-value for factor B was <0.0001, which indicated that it has significant effect on the % cumulative drug release (Silva et al., Citation2017). Interaction p-value was 0.3367 which indicated that there was no significant interaction between factors. The model fit statistics R2 value was found to be 0.9973, Adjusted R2 value as 0.9940, Predicted R2 value as 0.9767 and Adequate precision was 56.3957 This model suggested to be used to navigate the design space. Above equation represents the quantitative effect of the independent factors on the % cumulative drug release written in terms of coded factors. Contour & surface response plot for % cumulative drug release showed that as the Conc. of Chitosan increases from low (−1) to high level (+1) and Stirring speed increases from low (−1) to high level (+1), the % Cumulative drug release gets decreased which are indicated by negative sign in polynomial equation for X1 and negative sign in polynomial equation for X2. Because of the increase in the polymer density and reduction of the macromolecular chain mobility and thus the formation of more stable and rigid spheres which cause decrease in drug release and also cross linking process usually hardens the chitosan matrix and also increase the resistance for the penetration of the release medium (Youssef et al., Citation2020). The response contour plot and response surface plot for Y3 (% cumulative drug release) are shown in .
3.6.4. Optimization by overlay plot using design expert
The aim of the optimization of pharmaceutical dosage form is to determine the levels of the variable from which a robust product with high quality characteristics may be produced. An overlay plot relies on all the investigated formulation variables used to predict the ranges of variables where the optimum formulation might occur. The measured responses were optimized using Design Expert 11. The yellow region indicates the area in which optimized formulation can be formulated. The yellow portion covered one point (−1, 0) value that means formulation code GF4.
From the , it is concluded that the optimized batch with X1 = −1.000 [decoded value of 0.15% Chitosan conc.] and X2 = 0 [decoded value of 1000 rpm Stirring speed] were selected as optimized batch with highest desirability of 1 and showed the evaluation result of observed value of particle size as 71 nm, % entrapment efficiency of 81.11 and % CDR of 90.53 upto 24 hrs. with mean deviation of 0.6% which isless than 5% that indicates the selected design is valid for data obtained as shown in .
3.7. Scanning electron microscopy
The surface and size of the nanoparticle were evaluated using SEM. The micrographs showed that particles are in spherical shape with smooth surface when magnified at 11.03KX times ().
3.8. Evaluation and optimization of in situ gel
Nanoparticles incorporated In situ gel formulations B1, B2 and B3 showed translucent and clear appearance. All the three batches showed rapid gel formation where B3 remained in gel state for extended period.
3.8.1. Rheological study of in situ gel formulations
The rheological parameters such gel strength and viscosity before, after gelation revealed that B3 was optimized batch as shown in (Almeida et al., Citation2014b). The optimized formulation (B3) was subjected to in vitro studies, sterility, antimicrobial and stability studies.
Table 5. Rheological study of batch GF4 incorporated formulations.
3.8.2. Sterility testing
The optimized formulation B3 in situ gel formulation containing Gemifloxacin Mesylate Nanoparticles was sterilized in an autoclave. During the sterilization process, a sterile filter paper strip impregnated with spores of Bacillus stearothermophilus as a biological indicator packed in an aluminum foil was also subjected to autoclave sterilization along with optimized formulation . The Biological indicator was transferred aseptically into petriplate containing nutrient agar medium and incubated at 37° C for 24 hrs. The Petriplate did not show any growth of Bacillus stearothermophilus. This proves that autoclave sterilization is proper. Apart from this, the sterilize optimized formulation was also subjected to test for sterility as per Indian Pharmacopeia (2018). The formulation passed the test for sterility proving that there is no microbial contamination.
3.8.3. Antimicrobial activity study
Optimized in situ gel formulation B3 has shown well defined activity against selected gram positive and gram negative organisms against reference standard Streptomycin sulfate of 30 units/mL as shown in . The results indicated that formulation and sterilization processes have not altered the antimicrobial activity of an drug incorporated Nanoparticles Gel.
3.8.4. Stability study
Optimized formulation B3 was subjected to stability study as per ICH guidelines. Parameters like visual appearance, gelling capacity, and in vitro drug release were evaluated. There is no much variation in visual appearance, gelling capacity, and in vitro drug release indicating the stability of formulation based on 6 months study ().
Table 6. Stability study of optimized batch B3.
4. Conclusion
An effort was made to formulate in situ gel eye drops containing GM nanoparticles with the aim of increasing residence time and reducing the frequency of administration. Nanoparticles formulation was optimized using 32 factorial design where nine formulation (GF1-GF9) were prepared with different proportion of Chitosan . Almost, all formulations showed controlled release. Among all formulations, GF4 showed particle size of 71 nm, % entrapment efficiency of 81.11 and % CDR of 90.53 upto 24 hrs which is considered as optimized formulation. Hence, GF4 nanoparticles formulation was incorporated to prepare In situ gel formulations of B1 (GF4 + 9% Poloxamer 407), B2 (GF4 + 12% Poloxamer 407) and B3 (GF4 + 15% Poloxamer 407) respectively. Optimized B3 formulation passed the test for sterility and stability. We can conclude that gel formulation batch B3 (GF4 + 15% Poloxamer 407) has proven to be effective in achieving the aim of present research work.
Ethical approval
No Animals or Humans were involved in this work and hence no need of ethical approval.
Acknowledgments
The authors extend their sincere appreciation to the Deanship of Scientific Research at King Khalid University, Saudi Arabia and Dean of scientific research of Zarqa university, Jordan for funding this work through Research Group. Project number RGP.2/251/43.
The Indian authors are very much thankful to J.J.C.E.Trust, Junagadh and Gujarat Technological University, Chandkheda, Ahmedabad
Disclosure statement
The authors declare that they have no known competing financial interests or personal relationships that could have appeared to influence the work reported in this paper.
Correction Statement
This article has been corrected with minor changes. These changes do not impact the academic content of the article.
Additional information
Funding
References
- Abbas MN, Khan SA, Sadozai SK, et al. (2022). Nanoparticles loaded thermoresponsive in situ gel for ocular antibiotic delivery against bacterial keratitis. Polymers 14:1.
- Almeida H, Amaral MH, Lobão P, et al. (2014a). Applications of polymeric and lipid nanoparticles in ophthalmic pharmaceutical formulations: present and future considerations. J Pharm Pharm Sci 17:278–11.
- Almeida H, Amaral MH, Lobão P, Lobo JMS. (2014b). In situ gelling systems: a strategy to improve the bioavailability of ophthalmic pharmaceutical formulations. Drug Discov Today 19:400–12.
- Almeida H, Amaral MH, Lobão P, Sousa Lobo JM. (2013). Applications of oloxamers in ophthalmic pharmaceutical formulations: an overview. Expert Opin Drug Deliv 10:1223–37.
- Bodratti AM, Alexandridis P. (2018). Formulation of poloxamers for drug delivery. JFB 9:11–24.
- Bohrey S, Chourasiya V, Pandey A. (2016). Polymeric nanoparticles containing diazepam: preparation, optimization, characterization, in-vitro drug release and release kinetic study. Nano Converg 3:3–7.
- Destruel PL, Zeng N, Brignole-Baudouin F, et al. (2020). In situ gelling ophthalmic drug delivery system for the optimization of diagnostic and preoperative mydriasis: in vitro drug release, cytotoxicity and mydriasis pharmacodynamics. Pharmaceutics 12:360–19.
- Destruel PL, Zeng N, Maury M, et al. (2017). In vitro and in vivo evaluation of in situ gelling systems for sustained topical ophthalmic delivery: state of the art and beyond. Drug Discov Today 22:638–51.
- Garala K, Joshi P, Shah M, et al. (2013). Formulation and evaluation of periodontal in situ gel. Int J Pharm Investig 3:29–41.
- Gouda AA, Amin AS, El-Sheikh R, Yousef AG. (2014). Spectrophotometric determination of gemifloxacin mesylate, moxifloxacin hydrochloride, and enrofloxacin in pharmaceutical formulations using Acid dyes. J Anal Methods Chem 2014:286379.
- Hamed R, Kamal A, Zaid Alkilani A. Zaid Alkilani (2020). A gelation and rheological characterization of carbopol® in simulated gastrointestinal fluid of variable chemical properties. Pakistan Journal of Pharmaceutical Sciences 33:923–8.
- Hani U, Osmani RAM, Alqahtani A, et al. (2021). 23 full factorial design for formulation and evaluation of floating oral in situ gelling system of piroxicam. J Pharm Innov 16:528–536.
- Hani U, Rahamathulla M, Osmani RAM, et al. (2022). Development and characterization of oral raft forming in situ gelling system of neratinib anticancer drug using 32 factorial design. Polymers 14:2520.
- Imam SS, Abbas Bukhari SN, Ahmad J, et al. (2018). Formulation and optimization of levofloxacin loaded chitosan nanoparticle for ocular delivery: in-vitro characterization, ocular tolerance and antibacterial activity. Int J Biol Macromol 108:650–9.,
- Kirchhof S, Goepferich AM, Brandl FP. (2015). Hydrogels in ophthalmic applications. Eur J Pharm Biopharm 95:227–38.
- Pandey M, Choudhury H, Aziz ABA, et al. (2021). Potential of stimuli-responsive in situ gel system for sustained ocular drug delivery: recent progress and contemporary research. Polymers 13:1340–62.
- Paradkar MU, Parmar M. (2017). Formulation development and evaluation of natamycin niosomal in-situ gel for ophthalmic drug delivery. Undefined 39:113–22.
- Rahamathulla M, Hani U, Alqahtani A, et al. (2021a). 23 factorial design and optimization of effervescent floating matrix tablet of neratinib. J Pharm Innov 102788:2–9.
- Rahamathulla M, Saisivam S, Alshetaili A, et al. (2021b). Design and evaluation of losartan potassium effervescent floating matrix tablets: in vivo X-ray imaging and pharmacokinetic studies in albino rabbits. Polymers 13:3476.
- Rajalakshmi R, Padmaja C, Radhika N, et al. (2013). Formulation and assessment of GM ocular In situ gelling system. Int Res J Pharm 4:33–8.
- Scoper SV. (2008). Review of third-and fourth-generation fluoroquinolones in ophthalmology: in-vitro and in-vivo efficacy. Adv Ther 25:979–94.
- Shubhra QTH, Tóth J, Gyenis J, Feczkó T. (2014). Poloxamers for surface modification of hydrophobic drug carriers and their effects on drug delivery. Polym. Rev 54:112–38.
- Silva MM, Calado R, Marto J, et al. (2017). D. Chitosan nanoparticles as a mucoadhesive drug delivery system for ocular administration. Mar Drugs 15, 370:2–16.
- Tagalpallewar A, Rai P, Polshettiwar S, et al. (2021). Formulation, optimization and evaluation of ion triggered ophthalmic in situ gel. Pharm Res Int 33:58–77.
- Wang K, Han Z. (2017). Injectable hydrogels for ophthalmic applications. J Control Release 268:212–24.
- Wu Y, Liu Y, Li X, et al. (2019). Research progress of in-situ gelling ophthalmic drug delivery system. Asian J Pharm Sci 14:1–15.
- Wu Y, Yang W, Wang C, et al. (2005). Chitosan nanoparticles as a novel delivery system for ammonium glycyrrhizinate. Int J Pharm 295:235–245.
- Youssef A, Dudhipala N, Majumdar S. (2020). Ciprofloxacin loaded nanostructured lipid carriers incorporated into in-situ gels to improve management of bacterial endophthalmitis. Pharmaceutics 12, 572:1–11.