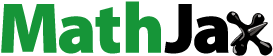
Abstract
Canagliflozin (CFZ) is a sodium-glucose cotransporter-2 inhibitor (SGLT2) that lowers albuminuria in type-2 diabetic patients, cardiovascular, kidney, and liver disease. CFZ is classified as class IV in the Biopharmaceutical Classification System (BCS) and is characterized by low permeability, solubility, and bioavailability, most likely attributed to hepatic first-pass metabolism. Nanocrystal-based sublingual formulations were developed in the presence of sodium caprate, as a wetting agent, and as a permeability enhancer. This formulation is suitable for children and adults and could enhance solubility, permeability, and avoid enterohepatic circulation due to absorption through the sublingual mucosa. In the present study, formulations containing various surfactants (P237, P338, PVA, and PVP K30) were prepared by the Sono-homo-assisted precipitation ion technique. The optimized formula prepared with PVP-K30 showed the smallest particle size (157 ± 0.32 nm), Zeta-potential (−18 ± 0.01), and morphology by TEM analysis. The optimized formula was subsequently formulated into a sublingual tablet containing Pharma burst-V® with a shorter disintegration time (51s) for the in-vivo study. The selected sublingual tablet improved histological and biochemical markers (blood glucose, liver, and kidney function), AMP-activated protein kinase (AMPK), and protein kinase B (AKT) pathway compared to the market formula, increased CFZ’s antidiabetic potency in diabetic rabbits, boosted bioavailability by five-fold, and produced faster onset of action. These findings suggest successful treatment of diabetes with CFZ nanocrystal-sublingual tablets.
1. Introduction
Sodium-glucose co-transporter (SGLT) inhibitors (including Canagliflozin, Dapagliflozin, Empagliflozin, and Ertugliflozin) are a potential class of drugs used in the management of type-II diabetes mellitus (T2DM). These drugs mediate glycated hemoglobin (HbA1C) levels and decrease glucose reabsorption, which promotes urine glucose excretion (Liu et al., Citation2020; Sammar et al., Citation2023a and Citationb). This unique T2DM-treatment approach caused great attention toward SGLT II inhibitors as it also reduces blood pressure and albuminuria in diabetics and improves insulin resistance and β-cell dysfunction. In the proximal convoluted tubule (PCT), SGLT II has a limited capacity, high-affinity Na+/K+ cotransporter that limits glucose reabsorption and increases glucose excretion (Chung et al., Citation2021; Marilly et al., Citation2022). Canagliflozin (CFZ) has been extensively used to treat T2DM through an insulin-independent mechanism that lowers blood pressure, body weight, and albuminuria in diabetics (Singh et al., Citation2020). Canagliflozin showed considerable anti-cancer activity in the DMBA-induced mammary carcinoma model. Canagliflozin regulated the mTOR-mediated signaling cascade, decreased pyroptotic and inflammatory pathways, suppressed oxidative stress, and ultimately prevented the DMBA-induced proliferation of breast cancer (Hamza et al., Citation2022; Sabaa et al., Citation2022).
Despite the promising antidiabetic and other activities of CFZ, being classified as Class IV of the Biopharmaceutical Classification System (BCS) (e.g. amphotericin B, furosemide, acetazolamide, Azelastine hydrochloride, sulfamethoxazole, aripiprazole, ritonavir, paclitaxel) and has low water solubility (0.003 mg/ml), low permeation (Papp <2 × 10−5 cm/s), high first-pass metabolism mediated by cytochrome P450 enzymes, and susceptibility to P-gp-mediated efflux that leads to low systemic bioavailability of around 65%. A drug delivery method other than oral is clinically significant (Ghadi & Dand, Citation2017; El-Gizawy et al., Citation2018).
Sublingual and buccal medication distribution is a respectable alternative to oral medication which provides advantages both locally and systemically. Such medications demonstrate rapid absorption into the systemic circulation through venous drainage to the superior vena cava. Additionally, these medications are considered prescribed for patients with high hepatic clearance or gastrointestinal degradation, and patients with swallowing issues. Drugs administered sublingually or intravenously are typically solid (tablets, wafers, films, and patches), liquid (sprays and drips), or semi-solid (gels) (Singh et al., Citation2021; Zhu et al., Citation2021).
Nanocrystal dispersion is a low-cost approach to formulate medicines that are water-insoluble into a more soluble and bioavailable form (Hoover et al., Citation2021). In light of the Noyes-Whitney formula, reducing the size of the particles through nanocrystal preparation methods (including antisolvent precipitation, emulsion method, impinging jet crystallization, supercritical fluid technology, and the top-down technique) accelerates the medication solubility rate (Leal-Júnior et al., Citation2020). Nanocrystals can be used in several approaches (including oral, ophthalmic, transdermal, pulmonary, and intravenous delivery, and targeting (both for the brain and for tumors) (Hoover et al., Citation2021).
Sodium caprate (C10) is the sodium salt of capric acid, an aliphatic medium-chain saturated 10-carbon used as a wetting agent and a penetration enhancer for medication formulations. SC (C10) has been licensed by the FDA and has been studied as a penetration enhancer to promote the adsorption of numerous medications, including ampicillin, insulin, low-molecular-weight (MV) heparin, and 5-fluorouracil (Twarog et al., Citation2019). Capric acid is mainly expressed in coconut oil (about 10%) and palm kernel oil (about 4%), but not in many other seed oils. It is also found in mammals’ milk and, to a lesser extent, in other animal fats. C10 is granted as a food additive in the US and the EU, with no daily consumption limitations; its presence in food should not affect human health (EFSA et al., Citation2018). C10 was initially discovered in a rectal ampicillin suppository and has been evaluated by Merrion Pharma as an oral solid dosage form (GIPETTM) for delivery of poorly permeable actives, including small molecules (e.g. zoledronic acid, alendronate) and macromolecules (insulin, desmopressin, and antisense oligonucleotides) (Lindmark et al., Citation1997; Walsh et al., Citation2011). C10 is an anionic surfactant that is pH and ion-sensitive. At pH units (1–3) below its pKa (∼5) in stomach fluid, the capric acid is inactive. Acidic pH reduces surface tension but has a little deterrent effect. C10 is ionized and detergent-soluble at pH levels 1–3 pH levels higher than its pKa (small intestine pH). Repulsion between the charged hydrophilic head groups prevents them from forming micelles. High concentrations of free monomeric surfactant allow epithelial plasma membrane to contact and transcellular activity. Like other ionizable surfactants, the critical micellar concentration (CMC) of C10 varies depending on the medium. In a high-ionic-strength buffer, counter-ions interact with anionic head groups, forming micelles at lower concentrations. Altering ionic strength affects the small intestine’s free monomeric C10 content (Twarog et al., Citation2019).
Poloxamers (e.g. P188, P237, P338, P407), known as Pluronic, are nonionic surfactants used for drug delivery as formulation excipients. They are used as surfactants, emulsifiers, solubilizers, dispersants, and in vivo absorbance enhancers in pharmaceutical formulations (Abdeltawab et al., Citation2020). PVP, commonly known as Povidone, is a benign, nonionic surfactant with a complicated affinity for both hydrophilic and hydrophobic medicines. PVP is a water-soluble polymer that possesses a variety of grades with variable molecular weights and viscosities. PVP is a poorly soluble drug binder, emulsion stabilizer, suspending agent, and solubility booster (Li et al., Citation2018; Jin et al., Citation2020). The necessary viscosity of the liquid dosage form can be obtained with medium and high molecular weight PVP (serving as a thickening agent), which aids in a steady drip rate, better appearance, dispersion, and physical stability (Józó et al., Citation2021). Sublingual tablet delivery systems and nanocrystals offer various benefits, such as improving solubility and permeability, preventing enzymatic drug-food and drug-food interactions that affect drug gastrointestinal absorption and pass the first-pass effect, and providing continuous-release delivery for many days (Naguib & Makhlouf, Citation2021).
Using the nanocrystal method and sodium caprate as a permeability enhancer and wetting agent, the current work modified the formulation of canagliflozin for use in children and adults in order to increase its solubility and permeability. The antisolvent Sono-homo-precipitation process, which is optimized for CFZ, was used to create the nanocrystals. The pharmacokinetics and biochemistry parameters in rabbits were used to examine the in-vivo and in-silico antidiabetic effects of the improved recipe.
2. Materials and methods
2.1. Materials
Canagliflozin (CFZ) standard was received from Sigma-Aldrich, Cairo, Egypt. Acetonitrile (HPLC grade), Polyvinylpyrrolidone (PVP-K30), Polyvinyl acetate (PVA), sodium deoxycholate (SDC), orthophosphoric acid, Poloxamers (P237 and P338), methanol (HPLC grade), Streptozotocin, and Nicotinamide (Molecular biology grade) from Sigma-Aldrich (St. Louis, MO, USA). Boric acid, Pharma burst-V® (containing mannitol, silicon dioxide, sorbitol, and povidone) from El-Nasr Pharmaceutical Company (Cairo, Egypt). Syringe filter papers of HPLC grade (0.22 μm) were received from SERVA Electrophoresis GmbH (Heidelberg, Germany). All biochemical kits for biochemical estimations were obtained from Stan bio laboratories (Texas, USA). Invokana® tablets (a market product containing 300 mg of pure canagliflozin per tablet) were manufactured by Janssen-Cilag and were received from the local market. All additional chemicals and solvents were of analytical quality and are used as obtained.
2.2. Methods
2.2.1. High-performance liquid chromatography analysis of Canagliflozin
Analysis was performed using an HPLC (Waters TM 600 controller, USA) equipped with an automatic sampling system (Waters TM 717 Plus Autosampler, USA) and a 4.6 × 150 mm, C-18 analytical column. The column oven and autosampler were both held at 8 °C. Acetonitrile and 0.01 M orthophosphoric acid were used in a low-pressure binary gradient mode with a 50:50 v/v ratio for analysis at 0.9 ml/min. Sample acquisition took 10 min. Photodiode array (PDA) detector at λ max 240 nm detected analyte (Moussa et al., Citation2021).
2.2.2. Design and optimization of formulation
Utilizing Design Expert® (Version 10.0.3.0, Stat-Ease Inc., Minneapolis, MN, USA), the impact of different formulation parameters on CFZ-NCs properties was investigated using surface response design. represents SAA Type (X1), four types (P237, P338 PVA, and PVP K30), SAA amount (X2), which varied between 100, 200, and 400 mg, and solvent volume (X3), which varied between 5, 7, and 10 ml from the dispersed phase, were the independent variables. In all formulations, CFZ, SDC, and SC were used in an amount of 100 mg, 100 mg, and 75 mg, respectively. Particle size (PS), zeta potential (ZP), polydispersity index, and saturated solubility (mg/L) were the dependent variables. The significance of the factors and the model was assessed using analysis of variance (ANOVA), and significance is denoted by probability values (p < 0.05). The number of experimental runs was thirty-six formulations. Using the plot 3D package in R software, 3D surface graphs are plotted, to get the most desirable formula, the optimization was set to have the smallest size and smallest polydispersity index, and large zeta-potential saturated solubility. All of the formulations were made three times, and the results were given as mean ± standard deviation.
Table 1. Independent variables, responses, and experimental runs for the formulations of CFZ-NCs according to surface response designs.
2.2.3. Preparation of Canagliflozin nanocrystals (CFZ-NCs)
Sono-Homo-Precipitation–method with permeability enhancer was used to make CFZ-NCs (Charoonsuk & Vittayakorn, Citation2013). First, the solvent phase was formed by adding 100 mg of CFZ, and sodium caprate (wetting agent and permeability enhancer) was dissolved in methanol (5, 7, and 10 ml). The solvent phase was then injected into 20 mL of antisolvent; at 40 °C pre-cooled water with 50 mg of sodium deoxycholate as a stabilizing agent and surface-active component (PVA, P237, P338, or PVP K30) in varying concentrations with a 50 mL beaker at room temperature. The organic phase was evaporated from the formulation for 20 min at 100 rpm and 70 °C. Then, 20 min were spent applying 100 W of ultrasonication (VCX600, Sonics and Materials, Newtown, CT, USA), and then 20 min were spent being homogenized at a speed of 7000 rpm. CFZ-NCs were lyophilized to solidify (Naguib & Makhlouf, Citation2021). An expert can freeze a nanocrystal at 20 °C for the night and then freeze-dry it at 45 °C for 24 h by using Novalyphe-NL500 lyophilize. As illustrated in , lyophilized nanocrystals were stored at room temperature on top of calcium chloride in a desiccator.
2.3. Characterization of CFZ-NCs and lyophilized CFZ-NCs
2.3.1. Particle size distribution, zeta potential, and polydispersity index
CFZ-NCA The Zeta sizer Nano (Malvern Instrument Ltd., Worcestershire, England) was used to measure the CFZ-NCs’ particle size (PS), zeta potential (ZP), and polydispersity index (PDI) at 25 °C after a sufficient dilution (1:10) (Abdelmonem et al., Citation2021). In a glass cuvette, the measurements were carried out three times at a count rate of 200–400 kilo counts per second (Kcps). The student’s t-test contrasted measured answers with pre-lyophilization responses (mean values and standard deviations).
2.3.2. Saturated solubility
Both lyophilized and purified CFZ-NCs were tested for methanol solubility (Fuguet et al., Citation2021; Naguib & Makhlouf, Citation2021). Further samples were added to methanol in 5 mL screw-capped glass vials before being put in a shaking, thermostatically controlled water bath created by (GFL, Gesellschaft Laboratories, Berlin, Germany). After 72 h, a filter with 0.45 m millipores was used to filter the supernatant, and the analysis was done using a 4.6 × 150 mm C-18 analytical column. The amount of medicine that was dissolved was then determined using an HPLC system (Nexera X2, Shimadzu Asia Pacific Limited, Japan). The column oven and autosampler were both held at 8 °C. For analysis at 0.9 ml/min, a low-pressure binary gradient mode utilizing acetonitrile and 0.01 M orthophosphoric acid at a 50:50 v/v ratio was used. Sample acquisition took 10 min. Photodiode array (PDA) detector at 290 nm detected analyte (Fuguet et al., Citation2021; Naguib & Makhlouf, Citation2021).
2.3.3. Fourier transformer infrared spectroscopy (FTIR)
The interaction of the Canagliflozin with different polymers in the formulation was examined using the Fourier transform infrared spectrometer (Perkin-Elmer spectrum 100) (Abdelmonem et al., Citation2021).
2.3.4. Morphology and transmission electron microscopy (TEM)
The morphology of Canagliflozin with various polymers in the formulation was examined using a transmission electron microscope (TEM) (JEM-1230, Joel, Tokyo, Japan). The samples were mounted onto a carbon-coated grid surface, negatively stained with a 1% phosphotungstic acid aqueous solution, and allowed to dry until observable at room temperature (Abdelmonem et al., Citation2021).
2.4. Formulation of CFZ nanocrystal-based sublingual tablets (CFZ-NCs-T)
In order to create CFZ nanocrystal-based sublingual tablets using the direct compression method, 100 mg of lyophilized CFZ-NCs-32 was combined with different amounts of Pharma burst-V®, lactose, and boric acid. The final tablet weight was 400 mg. Powder combinations were crushed using a single-punch machine (Royal Artist, Bombay, India) along with a 12 mm flat bunch and 4000 kg of force. (Bolourchian et al., Citation2009).
2.4.1. Optimization of CFZ-NCs-T formulation
Based on the USP disintegration time for sublingual tablets (less than 2 min), the ideal CFZ-NCs T formula was selected (Convention USP, Citation2012). Using the USP disintegration apparatus, six tablets of each formula were disintegrated at 370 C in distilled water (Logan instruments company, USA). The duration of the pills’ dissolution was noted (Convention USP, Citation2014).
2.5. CFZ nanocrystal-sublingual tablets (CFZ-NCs-32-T4) evaluation
2.5.1. Compressibility of CFZ-NCs-32-T4 powder
The CFZ-NCs-32-T4 powder compressibility was tested to guarantee that it is free-flowing and does not cause difficulties during compression. Powder compressibility is measured by Carr’s index and Hausner’s ratio. The flowability of the powder is better when Carr’s index and Hausner’s ratio are lower than 15% and 1.25, respectively (Salah et al., Citation2018). In-cylinder 15 ml, 5 grams of powder was poured into it, then the sample was pressed 500 times into it, and this process was repeated 3 times, using the bulk density (b) and the starting bulk volume, the volume occupied was computed (Vi). Then, the cylindrical graduation was tapped until uniformity of the volume was achieved. The tapped density (t) was then calculated using the measured powder’s final volume (Vf): Carr’s index and Hausner’s ratio were calculated using the following formulas (Salah et al., Citation2018; Shoman et al., Citation2023). A Carr’s index of <15 and a Hausner’s ratio of <1.8, indicate good compressibility as per the European Pharmacopeia (Council of Europe, Citation2017).
(1)
(1)
(2)
(2)
(3)
(3)
2.5.2. Characterization of CFZ-NCs-32-T4
Drug content, weight fluctuation, thickness, hardness, and friability of CFZ-NCs-32-T4 were also examined (Convention USP, Citation2014). The homogeneity of the CFZ content was examined after grinding and dissolving ten pills in methanol. HPLC was used to measure each tablet’s average CFZ content at 290 nm. Ten tablets were individually weighed, and the mean weight was determined. Ten tablets with 10 micrometers of thickness and diameter (Vogel, Germany, DIN-863). Tablets hardness was determined by the average breaking force of ten tablets (TBH 325 series, Erweka GmbH, Heusenstamm, Germany). Afterward, the mean hardness was measured in kilograms A friabilator was used to assess the friability of CFZ-NCs-T4 (Model DFI-1; Veego, Bombay, India). Ten tablets were put into a 25 rpm friabilator one at a time for four minutes. To determine the % friability of reweighed tablets:
(4)
(4)
The acceptable weight reduction is 1%.
2.5.3. CFZ-NCs-32-T4 wetting time and water absorption
Five tissue papers (diameter 10 cm) were placed in a Petri dish to test the tablet’s wetting time. The Petri dish received 10 ml of 0.1% methylene blue water. The time it took for the dye to reach the tablet’s top was documented as the wetting time. The tablet was reweighed to evaluate weight gain (Vlad et al., Citation2022).
2.6. In-vitro dissolution study
Using a U.S.P dissolution tester (Apparatus II) set at 37 °C ± 0.5 and 50 r.p.m., the dissolution profiles of CFZ from the produced sublingual tablets were compared to the market oral tablets (Invokana®, 100 mg). The dissolving solution was McIlvaine buffer (citric acid/phosphate buffer, pH 4). At set intervals (5, 10, 15, 30, 60, and 360 min), aliquots were taken, filtered, and HPLC analyses were performed to look for CFZ at a maximum of 240 nm (Shoman et al., Citation2023). An equivalent volume of the release media was used to replace the samples that were taken out. A graph showing the cumulative percentage of medications released over time was shown. The experiments were done in duplicate for each sample.
2.7. In-silico molecular modeling study
The interaction of the canagliflozin drug and the different components of the applied polymers toward the active site of sodium-glucose cotransporter 2 (SGLT2) protein was assessed by performing a computational docking utilizing the computational program Molecular Operating Environment (MOE). Recently, the 3D X-ray crystal structure of the SGLT2 protein has been resolved and the binding site and the active amino acid residues have been recognized (Niu et al., Citation2022). To explore the solubility and interaction of the different prepared drug-polymer probes, we have accomplished an extensive molecular modeling study utilizing the recently published structure of human SGLT2 protein co-crystallized with empagliflozin (PDB: 7vsi). The structure of the drug and monomers have been obtained using ChemDraw software and Discovery Studio software. The energy minimization has been applied under default parameters for the co-crystallized ligand, drug, and monomers. The 3D format of the SGLT2 protein was downloaded from the protein database website (http://www.rcsb.org/pdb, accessed on 01.08.2022) (Niu et al., Citation2022). The MOE software was used to remove the extra chains, deprotonate the SLGT2 protein, and delete the water molecules. The geometry optimization and charge expression were performed by applying a force field (MMFF94x) and search module (Conf). The modeling study was performed as previously reported, utilizing the Triangle Matcher placement method and London dG scoring function to examine the binding of empagliflozin into the pocket of the SLGT2 enzyme (Mohamed et al., Citation2021, Citation2022; Saied et al., Citation2021; Salem et al., Citation2022). The RMSD and binding poses acquired were assessed to ensure the similarity to the reported main binding interactions. The validated protocol was subsequently utilized to assess the binding affinity and bioavailability of canagliflozin and different monomers in the active pocket of the SLGT2 protein. The acquired results were analyzed to provide the best poses and interactions.
2.8. In-vivo hypoglycemic study
2.8.1. Experimental design
Forty-five New Zealand albino male rabbits free of diseases weighing (2.5 ± 3 kg) were procured from the Animal House of Cairo University’s Faculty of Pharmacy in Giza, Egypt. After acclimatization, animals were kept in propylene cages at a temperature (25 ± 2 °C) with day and dark cycles (12:12 h). The rabbits had unrestricted access to food and water. The rabbits’ handling was done in accordance with the Study Ethics Committee (REC), Faculty of Pharmacy, Cairo University rules, and according to the research procedures approved by the Animal Care Committee of the National Research Center (Cairo, Egypt) (PI 3185 on 15 January 2023) to minimize animal suffering.
2.8.2. Induction of type II diabetes mellitus (T2DM)
All rabbits fasted for 12 h prior to the in-vivo experiment but with unrestricted access to water. Thirty albino rabbits were equally allocated to groups (n = 6): control (A) and diabetic (STZ + NAD) (B). STZ + NAD-induced diabetes groups C, D, and E were given CFZ solution treatments, Market CFZ (Invokana) pills, and CFZ-NCs-32-T4. NAD (110 mg/kg) and STZ (50 mg/kg) were injected intraperitoneally into overnight-fasted rabbits. After 1 h of STZ + NAD treatment, in order to prevent hypoglycemia, rabbits received a 5 percent w/v sugar solution. During experiments, diabetic groups had low mortality.
2.8.3. Determination of the hypoglycemic effect of CFZ
Blood glucose readings confirmed diabetes after 5 days of STZ + NAD using a glucometer (Accu-Chek Aviva meter, USA) (Puri et al., Citation2022). The reading of the glucose levels obtained from T2DM-induced rabbits was more than 250 mg/dl. Both groups of rabbits—healthy and diabetic—were anesthetized using ether for around 2 min (Puri et al., Citation2022). 0.5 mL of blood was taken from the tail vein, and BGL was determined directly before treatment (i.e. at zero time) and at predetermined time intervals after treatments’ administration at 0.25, 0.5, 1, 2, 4, 6, 8, 12, and 24 h (Gajera et al., Citation2019). Centrifuging blood samples for 30 min at 5000 rpm with (2500 IU, 10 μl per sample) of heparin for separating plasma. The determined BGL was plotted versus time for each individual sample to generate the blood glucose profile for each experimental group.
2.8.4. Pharmacokinetic
CFZ plasma maximum concentration (Cmax), time maximum concentration (Tmax), the area under the curve from zero to the final sample point (AUC0–24), and the area under the curve from zero to infinity (AUC0–∞) are important pharmacokinetic parameters. The pharmacokinetic analysis was carried out by using fifteen albino rabbits in three groups, each group contained five rabbits. The pharmacokinetics parameter was produced using the software Kinetica VR (Version 5, Thermo Fisher Scientific Inc., MA, USA). The following calculation was used to evaluate the relative bioavailability of the oral market product (Abdelmonem et al., Citation2021).
(5)
(5)
The results were documented as the mean of 10 rabbits’ standard deviation. The CFZ-NCs-32-T4 and commercial product’s pharmacokinetic characteristics were compared using the student’s t-test at p < 0.05.
2.9. Estimating T2DM biochemistry
Anesthetized rabbits had cervical dislocated after 28 days of experimentation. Blood was taken from their retro-orbital plexus. Serum was extracted from blood samples by centrifuging them at 5000 rpm for 20 min for biochemical examination. Serum glucose levels (BGL) were measured. Aspartate transaminase (AST), alanine transaminase (ALT), and serum alkaline phosphatase (ALP) were tested for liver function. Kidney function was assessed using blood urea nitrogen (BUN) and serum creatinine. Microproteinuria was checked in urine samples, AMP-activated protein kinase (AMPK), and Protein kinase B (AKT) were tasted (Xia et al., Citation2010).
2.10. Histopathology
Kidneys were fixed in 4% formaldehyde solution under complete aseptic circumstances to assess the presence or absence of disease for each formula. Hematoxylin and eosin were used to stain the slides for each specimen (H&E). All H&E-stained slides were investigated under a light microscope to determine the severity of the inflammatory reaction and the presence or absence of renal spores (Abdelmonem et al., Citation2021; Vlad et al., Citation2022).
2.11. Statistical analysis of data
The results were expressed as the mean standard deviation after each measurement was done at least three times. Using SPSS version 23.0, a two-way analysis of variance (ANOVA) and the subsequent Bonferroni post hoc test or Student’s t-test were conducted to see whether there were significant differences between the results (IBM Company, Chicago, USA). At p < 0.05, differences were deemed significant.
3. Results and discussion
3.1. Formulation of CFZ-NCs
The optimal CFZ-NCs formula for further research was chosen using design-expert numerical optimization and desirability functions. The most desirable CFZ-NCs formula (near to 1) was selected (Leal-Júnior et al., Citation2020).
3.1.1. Formulation, Characterization, and Optimization of CFZ-NCs
Energy-efficient drug nanocrystal precipitation using an antisolvent is widely applicable (Leal-Júnior et al., Citation2020). Ultrasonication and homogenization during drug nanocrystal precipitation can overcome the drawbacks of a large range of particle sizes in this method. Dissolving CFZ in methanol and precipitating in water produced nanocrystals (Leal-Júnior et al., Citation2020). Antisolvents contain surface-active agents that are commonly utilized to ensure nanoscale drug precipitation. Drug nanocrystals are negatively charged, and sodium deoxycholate was added to provide electrical repulsion and balance the system. Sodium caprate is a surfactant and was added as a permeability enhancer. CFZ-NCs-32 (200 mg PVPK30, 100 mg SC, 100 mg SD, and 100 mg CFZ in 7 mL methanol) was the best formulation. Surface response designs were used to evaluate the effect of formulation variables on CFZ nanocrystals to optimize preparation conditions, as shown in and . It was found that the utilization of Poloxamer alone provides a bigger particle size, as compared to its combination with PVP-K30 (). The average particle size of the plain drug suspension was prepared under the same conditions. Nanosuspensions containing 200 mg PVP K30 in 7 ml methanol showed Zav of 157 nm with a PDI of 0.39; whereas with PVA it was 690 nm with a PDI of 0.89. The particle size of a nanosuspension comprising Poloxamers (P237, P338) and medication was average. PVA and Poloxamers alone were ineffective as stabilizers, causing flocculation. This could be owing to its high hydrophilicity (HLB = 29), which prevents preferential adsorption on the nanocrystal surface. However, when combined with PVP-K30, it synergistically functioned to reduce the particle size and provided an intermediate HLB of 13, as well as the ability to synergistically act with other surfactants or hydrophilic polymers for nanosystem stabilization. The greatest particle size reduction was seen with PVPK30, which is believed to be a protective colloid, indicating a higher adsorption potential for nanoparticles (Walsh et al., Citation2011). The zeta potential of all formulations ranged from −18 to −27 mV. The low numbers indicate that the plane of shear has shifted, causing the zeta potential to be measured at a greater distance from the particle surface. This is to be expected given that the stabilizers utilized to prepare the nanosuspensions are either hydrophilic polymers or nonionic surfactants that sterically stabilize the particles. The possibly formed negative charge may be caused by the ionization of surface functional groups in the drug molecule/stabilizer or by the adsorption of solvent ions on the particles, resulting in low zeta potential values.
Figure 2. Response 3D plots for the effect of SAA Type, SAA amount, and solvent volume on ZP, PS, PDI, and saturated solubility of nanoparticles.

Table 2. Composition of nanocrystals formulations, n = 3.
3.1.2. Polydispersity index
The polydispersity index (PDI) measures the uniformity of particle size within a dispersed system. Lower PDI values demonstrated the consistency of the system (Mohamed et al., Citation2022). The PDI value of the produced CFZ-nanocrystals had a range of 0.390.04 to 0.890.01, as shown in and . The findings were in agreement with previous research that used the Homo-sono-precipitation approach to prepare nanocrystals and showed similar PDI values (Puri et al., Citation2022). Canagliflozin nanocrystals were synthesized via sonication, and it was revealed that increasing drug concentration and supersaturation resulted in faster crystal formation and agglomeration, which eventually led to larger initial crystals with a higher PDI value (Gajera et al., Citation2019). The ANOVA test found that the difference in PDI values between formulations was not statistically significant (p > 0.05).
3.1.3. Saturated solubility
shows the saturation solubility of the produced CFZ-NCs. The saturation solubility of CFZ-NCs ranged from 14.270.30 mg/L to 24.750.31 mg/L, over eight times that of pure CFZ (3.92 0.12 mg/L). Reducing the particle size until it reaches the nano-range has enhanced the surface area that is accessible to the solvent and, as a result, enhanced the drug solubility. Furthermore, as observed in the CFZ-NCs-32 diffractogram, the increased solubility of CFZ-NCs may be related to a decrease in the drug’s crystallinity. However, the difference in saturation solubility between the different CFZ-NCs formulations was not statistically significant (p > 0.05).
3.1.4. Optimization of CFZ-NCs
An efficient method for improving pharmaceutical preparations where several variables influence the attributes of the final formulation is to use the desired function (Ramasamy et al., Citation2013). Minimum particle size, maximum zeta potential, and minimal polydispersity index were the desired constraints in CFZ-NCs. According to and desirability ratings, CFZ-NCs-32 has the highest rating of 0.891. showed that there was a good agreement between the estimated projected values for the variables that are dependent using Design Expert VR software and the results that were actually seen. As a result, the nanocrystal formulation CFZ-NCs-32, which comprises 200 mg of PVPK30, 100 mg of SC, 100 mg of SD, and 100 mg of CFZ in 7 mL of methanol, was chosen for further research.
3.1.5. Fourier transforms Infrared (FT-IR) Spectroscopy excipients
The FTIR spectra of canagliflozin nanocrystals when compared with the IR spectra of the canagliflozin pure drug, showed no interaction of the drug with the excipient, indicating the compatibility of the drug with the excipient. Hence, the principal peaks of canagliflozin were shown in the IR spectra of formulation (). There was no shifting of peaks found. These findings indicate that canagliflozin remains unaffected during the process of extrusion and spheronization in the presence of PVP-K30 and sodium caprate.
3.1.6. Morphology and transmission electron microscopy (TEM)
The morphometric characteristics of the improved formulation of Canagliflozin nanocrystals were determined using TEM analysis, as shown in . The findings showed that NPs have spherical shapes with stabilizer coated outside. Based on TEM, the particle size of the nanocrystals has a range from 100 nm to 200 nm. These findings could be attributed to the fact that the pure mechanical grinding does not provide an entirely uniform system, leading to a huge distribution in the size observed in TEM.
3.1.7. Preparation of CFZ nanocrystal-based sublingual tablets (CFZ-NCs-32-T)
shows the composition of CFZ-NCs by direct compression is one of the most used techniques for the preparation of nanocrystal-based sublingual tablets as it requires less setup and has a wide range of industrial applications. Furthermore, neither water nor an organic solvent is needed to be added (Sammar et al., Citation2023a, Citationb). However, it can be difficult to formulate sublingual tablets for a medication that possesses a low solubility in water because the medication needs to quickly dissolve in a tiny amount of saliva. In our study, the CFZ sublingual tablets were prepared using the lyophilized nanocrystal formula CFZ-NCs-32. In addition, Pharma burst-V® (85% D-mannitol, <10% silicon dioxide, <10% sorbitol, 5% crospovidone) served as a powerful disintegrant (Zhao et al., Citation2022). Under these conditions, the obtained Pharma burst tablets would endure transit, storage, and removal from the blister, as well as a fast oral dissolution, as CFZ has limited basic characteristics. Adding an acidic excipient could result in an acidic microenvironment around the tablet, increasing drug solubility. Therefore, the lubricant of choice in the CFZ sublingual pills was boric acid. Aside from being utilized as a lubricant, it may improve the drug’s water solubility and dissolution (Sherje et al., Citation2017).
Table 3. Composition of CFZ-NCs-T formulation.Table Footnote a
3.1.8. Characterization of CFZ-NCs-32-T
The disintegration time of sublingual pills is critical. According to USP, the breakdown duration of sublingual pills should not exceed 2 min. The disintegration time of the prepared CFZ-NCs-32-T, shown in , ranged from 51 s to 195 s, in the following sequence order CFZ-NCs-32-T1 > CFZ-NCs-32-T2 > CFZ-NCs-32-T3 > CFZ-NCs-32-T4. The disintegration period of the produced tablets decreases as the Pharma burst VR concentration in the mix increases. This is primarily due to the incorporation of crospovidone as a component of Pharma burst VR. Previous studies found that crospovidone sublingual pills disintegrated in less than 40 s (Gulsun et al., Citation2017). As a result, CFZ-NCs-32-T4 was selected for advanced research.
Wetting time is a valuable analysis for sublingual Oro dispersible tablets, as it stimulates the state under the tongue using a modest amount of saliva (1 to 5 mL) (Quodbach & Kleinebudde, Citation2016). It also indicates the tablets’ porosity and has been found to be linked to the disintegration time. The wetting time of CFZ-NCs-32-T4 was determined to be 51 s, which corresponds to the disintegration time. Based on USP requirements, the weight fluctuation, content homogeneity, and friability of CFZ-NCs-32-T4 were within acceptable limits (Convention USP, Citation2012). The weight of ten pills was 400 mg ± 10, with a CFZ content of 100 mg ± 3%. The thickness of the ten tablets was 2 and the diameter was 10 mm, with no statistically significant difference (p > 0.05). Friability, which assesses the effect of friction that may occur during tablet handling and result in tablet chipping or disintegration, was less than 1% (0.09%). Meanwhile, hardness, which assesses the force necessary to crush the tablet, was 2.5 kg, indicating that CFZ-NCs-32-T4 has strong mechanical resistance. The reasonably low hardness value is significant in sublingual tablets as it allows them to dissolve quickly in the small amount of saliva in the oral cavity (Ramasamy et al., Citation2013).
3.1.8.1 Compressibility of CFZ-NCs-32-T4
The calculation of the compressibility index (Carr’s index) and Hausner’s ratio is a quick and simple approach for predicting the flowability and the compressibility of powders (Convention USP, Citation2012; Shoman et al., Citation2023). They are affected by bulk and tapped density, which is connected to the powder’s moisture content and cohesiveness (Council of Europe, Citation2019). Our results indicated that Carr’s index and Hausner’s ratio values are 12% and 1.23 respectively, suggesting excellent flow properties according to European Pharmacopoeia 6.0 ().
Table 4. Compressibility parameters of CFZ-NCs-T4.
3.2. In-vitro drug release studies
To assure sink conditions in line with US Food and Drug Administration regulations, the CFZ-NCs-T4 dissolving test was conducted in a 250 mL McIlvaine buffer of pH 4. Comparatively, less than 35% of the drug was released within an hour with CFZ powder, whereas 85% of the medication was released after 5 min, and the total breakdown happened within 15 min (). These findings were in line with earlier studies that showed 80% of the drug in sublingual pills dissolves in under 15 min (di Prima et al., Citation2021; Sammar et al., Citation2023a, Citationb). The quick decomposition of CFZ-NCs-T4 was caused by a number of factors. The drug’s saturation solubility was first increased by the nanosized particles, as can be seen above, by roughly six times, from 4 to 23 mg/L. The Noyes-Whitney equation (Lippincott Williams & Wilkins, Citation2011) states. The drug dissolves more quickly because of the increased exposed total surface area to the dissolution media that results from the particle size reduction:
where dc/dt denotes the dissolving rate, A is the surface area, D is the drug’s diffusion coefficient, Cs is the saturation solubility, Cb is the bulk concentration, and h is the diffusional path. Particle size reduction raises the concentration slope and shortens the diffusion distance, increasing the dissolution level. reporting the total dissolution of canagliflozin nanocrystals in 120 min when compared with the original drug, which demonstrated almost minimal dissolution in the identical time frame (Shahinaze et al., Citation2023). Moreover, boric acid was used in the formulation to lower the pH of the area around the tablet, which in turn improved the conditions for drug breakdown and increased the dissolution ratio. These results corroborated earlier research by Younes et al. (Citation2021), which showed that the release of the weakly basic amisulpride occurred regardless of pH when organic acid was used as a pH modulator (Younes et al., Citation2021). Additionally, the X-ray diffractometry-measured decrease in drug crystallinity hastened breakdown. The fact that the tablets dissolve in 51 seconds is a significant factor in medicine dissolving because PharmaburstVR, which makes up 60% of the tablet weight, functions as a super disintegrant. Faster drug release occurs as a result of tablet disintegration, which causes the tablet to scatter in the dissolving liquid as a suspension. These results were reliable.
3.3. In silico prediction of solubility and interaction by molecular modeling study
Computational docking studies have been recently considered a valuable tool to explore the efficacy and bioavailability of bioactive polymer-based drug probes toward a targeted protein (Singh et al., Citation2021; Hoover et al., Citation2021; Zhu et al., Citation2021). In the current study, we prepared different formulations of polymer-based canagliflozin (CFZ) drugs. To further explore the bioavailability and affinity of the different formulations, we have examined the affinity of the different polymer-based canagliflozin formulations to target the SLGT2 protein. Toward this, we have in silico assessed the binding affinity of CFZ-SC10-PVP, CFZ-SC10-P, and CFZ-SC10-PVA. The 3D X-ray crystal structure of human SLGT2 protein co-crystallized with an empagliflozin drug has been recently reported which revealed the binding pocket and essential amino acid residues in the active site of SLGT2 protein (PDB: 7vsi). In our study, we first validated a suitable protocol to perform the molecular modeling study by redocking the co-crystallized empagliflozin ligand to affirm the presence of the main reported interactions (Liu et al., Citation2020). Subsequently, the validated molecular modeling protocol was utilized to assess the affinity score of the different polymer-CFZ formulations. As shown in , all examined formulations demonstrated the capability to bind to the pocket of SLGT2 protein in a thermodynamically favorable manner and with considerable binding scores. As indicated in , canagliflozin exhibited the ability to bind to the pocket of SLGT2 protein mainly via hydrophilically binding of the sugar moiety to a set of essential amino acid residues (Asn75, Thr87, Phe98, Ser287, Trp291, Gln457). Furthermore, the thiophene moiety contributed to the binding through interaction with the essential Thr87 amino acid. The binding of canagliflozin was further stabilized by a set of hydrophobic interactions with greasy amino acids in the pocket (−14.62 kcal/mol). Sodium caprate showed a high binding affinity score to the SLGT2 pocket through the binding of the carboxyl group to Asn75, Glu99, and Lys321 residues (−10.29 kcal/mol). These results indicate that the use of sodium caprate could improve the binding affinity and bioavailability of the formulated CFZ-drug. Next, we examined the binding affinity of the different applied polymers. The results indicated that the PVP possesses the highest binding affinity (−9.43 kcal/mol) compared to the bis-glycol ether and PVA (−9.27 and −8.55 kcal/mol, respectively) (). Indeed, the vinylpyrrolidone succeeded to form three strong hydrophilic interactions to essential amino acid residues (Asn75, Trp291, Lys321) in the SLGT2 pocket, similar to that of sodium caprate (). Although, both bis-glycol ether and PVA moieties could form two-three hydrophilic interactions to the amino acid residues in SLGT2 pocket, they could not bind to the essential amino acid Asn75 residue (). Based on these results, the CFZ-SC10-PVP formulation holds the highest affinity score toward SLGT2 pocket with the ability to establish a set of hydrophilic and hydrophobic interactions. Similarly, both of CFZ-SC10-P, and CFZ-SC10-PVA formulations displayed considerable affinity scores. Taken together, the in silico molecular modeling study suggest that the CFZ-SC10-PVP formulation could possess a high binding affinity and bioavailability than the other formulations. These findings are in agreement with the presented results in our study which showed that the CFZ-SC10-PVP formulation exhibits improved bioavailability and solubility.
Figure 6. The interactions (2D and 3D) of Canagliflozin (a), sodium caprate (b), vinylpyrrolidone (c), bis-glycol ether (d), and vinyl alcohol (e) (green in 3D interactions) into the active site of human SGLT2 protein (PDB code: 7vsi). the hydrogen interactions are shown as dotted green arrows; (C atoms are colored gray, O red, and S yellow).

Table 5. Binding affinity and interactions of Canagliflozin and different monomers into the binding site of the human SGLT2 protein.
3.4. Pharmacokinetic studies
The rabbit plasma, blank plasma, blank plasma with CFZ, and ultra-liquid CFZ chromatograms are shown in . The enhanced formulation quickly self-emulsifies in the GI lumen due to its reduced Tmax. When compared to pure drugs and marketed products CFZ in CFZ-CSs-T4 demonstrated a AUC0- 24 h (3.73 and 2.41fold) and Cmax (7.15 and 3-fold). increase in AUC0- 24h and Cmax, respectively (). Improved drug absorption and permeability via CFZ-NCs-T4 can be attributed to improved solubilization, increased CFZ permeability in the isotropic mixture, nanocrystals of the generated microemulsion following dispersion, and the increased surface area controlled by Neusilin US2’s quick desorption in biological fluids (Niu et al., Citation2022; Sammae et al., Citation2023b). Transcellular micellization of endo polymers and self-forming nanosuspension are the primary modes of absorption. In the intestinal mucosa, the formed mixed micelles create chylomicrons, which increase lymphatic absorption. The lipid excipients limit the efflux of the substrate medication CFZ out of the cells by blocking P-gp, a transporter on the epithelial surface of the intestinal membrane, elevating its therapeutic concentration. According to pharmacokinetics findings, lymphatic circulation improved CFZ-NCs-32-T4 bioavailability.
Figure 7. Comparing the time-dependent plasma concentration profile of CFZ in rabbits after sublingual administration of CFZ-NCs-32-T4 with the oral medication Invokana (Mean ± SD, n = 3).

Table 6. In comparison to oral drug delivery, sublingual CFZ-NCs-32-T4 treatment resulted in altered CFZ pharmacokinetic characteristics in rabbits (Mean ± SD, n = 3).
3.5. Pharmacodynamic studies
1-Methyl-1-nitrosourea (STZ) damages pancreatic cells to generate diabetes in animal models via pancreatic-cell pyrimidine nucleotide junctions (Mohamed et al., Citation2021). STZ’s alkylating tendency activates poly-ADP-ribose synthetase and degrades NAD in islets (Saied et al., Citation2021). Co-administration of NAD, a poly-ADP-ribose synthetase inhibitor, protects islet function and reverses excessive insulin secretion to prevent pancreas damage (Salem et al., Citation2022). STZ-NAD contributes to T2DM-like symptoms.
3.5.1. Analysis of biochemical parameters in T2DM
As shown in , liver and kidney BGL, HbA1c, and biochemical markers were evaluated. STZ + NAD-treated diabetics showed higher BGL, liver, and renal parameters than the control group. All biochemical markers in treated groups demonstrated a significant decrease (p ˂ 0.05) compared to the diabetic group. In diabetics, glucose molecules attach to hemoglobin, producing glycosylation. Our results are in agreement with previous ones (Sherje et al., Citation2017). AST, ALT, and ALP are indications of a damaged liver (Convention USP, Citation2012; Gulsun et al., Citation2017). Chronic hyperglycemia alters renal glomeruli and sub-micron blood channels, causing the protein to accumulate in the urine and changing kidney function (Council of Europe, Citation2019; di Prima et al., Citation2021). shows that AMP-activated protein kinase (AMPK), and protein kinase B (AKT) was increased in the animal group treated by nanocrystals compared to other groups. All treated groups showed normalized biochemical profiles, especially CFZ-NCs-32-T4. CFZ-NCs-32-T4 > Invokana > pure medication demonstrated potential anti-diabetic activity. Our findings indicate that the reduction in biochemical levels with adjusted CFZ-NCs-32-T4 formulation may be attributable to an improvement in the CFZ’s solubility, permeability, and oral bioavailability. Increasing CFZ levels boosts treatment efficiency.
Figure 8. Effect of control, diabetic, pure dug, Invokana (commercial product), and CFZ-NCs-32-T4 groups in AMPK and AKT value.

Table 7. biochemical markers of control, diabetic, pure dug, Invokana (commercial product), and CFZ-NCs-32-T4 Groups.
3.6. Histological analysis
The microscopic study and assessment of the renal tissues showed regular histological images in the kidneys of the control model (), and a varied range of changes in the nephropathic structures involved in the tubules (single-cell necrosis, vacuolations, and cast formation, epithelial cell attenuation, and tubular dilatation with flattening of the epithelial lining), glomeruli (mesangial expansion, sclerosis, atrophy with widen Bowman’s space, and congestion), blood vessels (hyalinosis, endothelial hypertrophy, congestion, and medial vacuolation), and interstitium (fibrous tissue proliferation, hemorrhages, and inflammatory cell infiltrate) () in the STZ-diabetic rabbits. Pure drug and market product treatment drastically diminished the frequencies and severities of diabetic-induced nephropathic changes. Furthermore, various structural changes were apparent in the renal tissue sections of the diabetic rabbits treated with CFZ. These changes involved tubular epithelial cloudy swelling, and attenuation, interstitial fibrous tissue proliferation, glomerular atrophy, cast formation, inflammatory cell infiltrates, and vascular congestion (). CFZ-NCs-32-t4 treatment demonstrated an almost normal histological appearance ().
4. Conclusion
The BCS class IV medication CFZ was the focus of this research in order to improve its solubility, permeability, and bioavailability and prepare formulations suitable for diabetic pediatrics and adult patients. Due to the intense first-pass metabolism that CFZ is subject to, sublingual administration is a preferred method of administration. However, the sublingual route presents significant difficulties for CFZ because of its limited water solubility and poor permeability. CFZ saturated solubility and the desired function technique were used to generate and refine a nanocrystal dispersion that was five times better. Direct compression was used to construct the sono-homo-assisted-precipitation approach for the nanocrystal dispersion in the presence of sodium caprate as a permeability enhancer and CFZ sublingual tablets. The tablets disintegrated in 51 seconds. The nanocrystal-based sublingual tablets considerably increased the bioavailability of CFZ by five times, according to the in vivo pharmacokinetic research. Our research suggests that sublingual CFZ nanocrystal-based tablets are a viable drug delivery method that may improve the drug’s bioavailability, hasten its onset of action, and reduce the inter- and intra-subject variability connected to oral administration. The affordable effectiveness of the excipients utilized and the ease of preparation give an additional benefit to industrial production.
Ethical approval statement
Male New Zealand albino rabbits were provided from the Animal House of Cairo University’s Faculty of Pharmacy in Giza, Egypt. All animal procedures were carried out per the Regulations for the Care and use f Laboratory Animals unrestricted access to food and water. The rabbits’ handling was done in accordance with the Study Ethics Committee (REC), Faculty of Pharmacy, Cairo University rules, and according to the research procedures. All animal experiments were approved by Institutional Review Board (or Ethics Committee) of the Faculty of Pharmacy, Cairo University, Cairo, Egypt (protocol code PI 3185 and date of approval 15.01.2023). The ARRIVE criteria were followed in all animal experiments. All precautions were taken in order to minimize animal suffering during the experiments.
Disclosure statement
No potential conflict of interest was reported by the authors.
Data availability statement
The data that support the findings of this study are available from the corresponding author and first author QW upon reasonable request.
References
- Abdelmonem R , Elhabal SF , Abdelmalak NS , et al. ( 2021). Formulation and characterization of acetazolamide/carvedilol niosomal gel for glaucoma treatment: in vitro, and in vivo study. Pharmaceutics 13: 1–16. doi: 10.3390/pharmaceutics13020221.
- Abdeltawab H , Svirskis D , Sharma M. ( 2020). Formulation strategies to modulate drug release from poloxamer based in situ gelling systems. Expert Opin Drug Deliv 17: 495–509. doi: 10.1080/17425247.2020.1731469.
- Bolourchian N , Hadidi N , Foroutan S , Shafaghi B. ( 2009). Development and optimization of a sublingual tablet formulation for physostigmine salicylate. Acta Pharm 59: 301–12. doi: 10.2478/v10007-009-0028-5.
- Charoonsuk P , Vittayakorn N. ( 2013). Perovskite phase formation of monosized lead zirconate (PbZrO3) nanoparticles prepared by the sono-assisted co-precipitation method. J Nanosci Nanotechnol 13: 3529–34. doi: 10.1166/jnn.2013.7239.
- Chung YJ , Park KC , Tokar S , et al. ( 2021). Off-target effects of sodium-glucose co-transporter 2 blockers: empagliflozin does not inhibit Na+/H + exchanger-1 or lower [Na+]i in the heart. Cardiovasc Res 117: 2794–806. doi: 10.1093/cvr/cvaa323.
- Convention USP. ( 2012). The United States Pharmacopeia 2014: USP 37.Rockville (MD): United States Pharmacopeial Convention.
- Convention USP. ( 2014). USP 37. Rockville (MD): United States Pharmacopeial Convention.
- Council of Europe. ( 2017). European pharmacopoeia. 9th ed. Strasbourg (France): Council of Europe.
- Council of Europe. ( 2019). European pharmacopoeia. 9th ed. Strasbourg (France): Council of Europe.
- di Prima G , Angellotti G , Scarpaci AG , et al. ( 2021). Improvement of resveratrol permeation through sublingual mucosa: chemical permeation enhancers versus spray drying technique to obtain fast-disintegrating sublingual mini-tablets. Pharmaceutics 13: 1370. doi: 10.3390/pharmaceutics13091370.
- EFSA Panel on Food Additives and Nutrient Sources added to Food (ANS) ; Younes M , Aggett P , Aguilar F , et al. ( 2018). Re-evaluation of sodium, potassium and calcium salts of fatty acids (E 470a) and magnesium salts of fatty acids (E 470b) as food additives. EFSA J 16: e05180. doi: 10.2903/j.efsa.2018.5180.
- El-Gizawy S , Osman M , Ibrahim S. ( 2018). Effect of cosolvents on the absorptive clearance of ketotifen fumarate from rabbit intestine, in-situ. J Adv Pharm Res 2: 168–179. doi: 10.21608/aprh.2018.3450.1055.
- Fuguet E , Subirats X , Ràfols C , et al. ( 2021). Ionizable drug self-associations and the solubility dependence on PH: detection of aggregates in saturated solutions using mass spectrometry (ESI-Q-TOF-MS/MS). Mol Pharm 18: 2311–2321. doi: 10.1021/acs.molpharmaceut.1c00131.
- Gajera BY , Shah DA , Dave RH. ( 2019). Development of an amorphous nanosuspension by sonoprecipitation-formulation and process optimization using design of experiment methodology. Int J Pharm 559: 348–359. doi: 10.1016/j.ijpharm.2019.01.054.
- Ghadi R , Dand N. ( 2017). BCS class IV drugs: highly notorious candidates for formulation development. J Control Release 248: 71–95. doi: 10.1016/j.jconrel.2017.01.014.
- Gulsun T , Ozturk N , Kaynak MS , et al. ( 2017). Preparation and evaluation of furosemide containing orally disintegrating tablets by direct compression. Pharmazie 72: 389–394. doi: 10.1691/ph.2017.6149.
- Hamza AA , Khasawneh MA , Elwy HM , et al. ( 2022). Salvadora persica attenuates DMBA-induced mammary cancer through downregulation of oxidative stress, estrogen receptor expression and proliferation, and augmenting apoptosis. Biomed Pharmacother 147: 112666. doi: 10.1016/j.biopha.2022.112666.
- Hoover A , Chitranshi P , Momot M , et al. ( 2021). In vitro evaluation of enteral tube administration of lansoprazole orally disintegrating tablets. Pharm Dev Technol 26: 846–851. doi: 10.1080/10837450.2021.1948567.
- Jin L , Yang YZ , Fan JF , Xu BS. ( 2020). Effect of surfactant polyvinyl pyrrolidone on the properties of microporous carbon nanospheres reinforced magnesium matrix composites. Nanomaterials 10: 1–21. doi: 10.3390/nano10112281.
- Józó M , Simon N , Yi L , et al. ( 2021). Improved release of a drug with poor water solubility by using electrospun water-soluble polymers as carriers. Pharmaceutics 34: 2022. doi: 10.3390/pharmaceutics.
- Leal-Júnior CC , Amorim MMR , Souza GFA , et al. ( 2020). Effectiveness of an oral versus sublingual loading dose of nifedipine for tocolysis. Int J Gynaecol Obstet 148: 310–315. doi: 10.1002/ijgo.13067.
- Li X , Wang L , She L , et al. ( 2018). Immunotoxicity assessment of ordered mesoporous carbon nanoparticles modified with PVP/PEG. Colloids Surf B Biointerfaces 171: 485–493. doi: 10.1016/j.colsurfb.2018.07.072.
- Lindmark T , Söderholm JD , Olaison G , et al. ( 1997). Mechanism of absorption enhancement in humans after rectal administration of ampicillin in suppositories containing sodium caprate. Pharm Res 14: 930–935. doi: 10.1023/A:1012112219578.
- Lippincott Williams & Wilkins. ( 2011). Martin’s physical pharmacy and pharmaceutical sciences: physical chemical and biopharmaceutical principles in the pharmaceutical sciences. 6th ed. Baltimore (MD): Lippincott Williams & Wilkins.
- Liu J , Li L , Li S , et al. ( 2020). Sodium-glucose co-transporter-2 inhibitors and the risk of diabetic ketoacidosis in patients with type 2 diabetes: a systematic review and meta-analysis of randomized controlled trials. Diabetes Obes Metab 22: 1619–1627. doi: 10.1111/dom.14075.
- Marilly E , Cottin J , Cabrera N , et al. ( 2022). SGLT2 inhibitors in type 2 diabetes: a systematic review and meta-analysis of cardiovascular outcome trials balancing their risks and benefits. Diabetologia 65: 2000–2010. doi: 10.1007/s00125-022-05773-8.
- Mohamed DI , Abou-Bakr DA , Ezzat SF , et al. ( 2021). Vitamin D3 prevents the deleterious effects of testicular torsion on testis by targeting MiRNA-145 and ADAM17: in silico and in vivo study. Pharmaceuticals 14: 1222. doi: 10.3390/ph14121222.
- Mohamed DI , El-Waseef DAEDA , Nabih ES , et al. ( 2022). Acetylsalicylic acid suppresses alcoholism-induced cognitive impairment associated with atorvastatin intake by targeting cerebral MiRNA155 and NLRP3: in vivo, and in silico study. Pharmaceutics 14: 529. doi: 10.3390/pharmaceutics14030529.
- Moussa BA , Mahrouse MA , Fawzy MG. ( 2021). Application of experimental design in HPLC method optimization and robustness for the simultaneous determination of canagliflozin, empagliflozin, linagliptin, and metformin in tablet. Biomed Chromatogr 35: e5155. doi: 10.1002/bmc.5155.
- Naguib MJ , Makhlouf AIA. ( 2021). Scalable flibanserin nanocrystal-based novel sublingual platform for female hypoactive sexual desire disorder: engineering, optimization adopting the desirability function approach and in vivo pharmacokinetic study. Drug Deliv 28: 1301–1311. doi: 10.1080/10717544.2021.1938755.
- Niu Y , Liu R , Guan C , et al. ( 2022). Structural basis of inhibition of the human SGLT2–MAP17 glucose transporter. Nature 601: 280–284. doi: 10.1038/s41586-021-04212-9.
- Puri V , Froelich A , Shah P , et al. ( 2022). Quality by design guided development of polymeric nanospheres of terbinafine hydrochloride for topical treatment of onychomycosis using a nano-gel formulation. Pharmaceutics 14: 2170. doi: 10.3390/pharmaceutics14102170.
- Quodbach J , Kleinebudde P. ( 2016). A critical review on tablet disintegration. Pharm Dev Technol 21: 763–774. doi: 10.3109/10837450.2015.1045618.
- Ramasamy T , Ruttala HB , Shanmugam S , Umadevi SK. ( 2013). Eudragit-coated aceclofenac-loaded pectin microspheres in chronopharmacological treatment of rheumatoid arthritis. Drug Deliv 20: 65–77. doi: 10.3109/10717544.2012.762434.
- Sabaa M , Sharawy MH , El-Sherbiny M , et al. ( 2022). Canagliflozin interrupts MTOR-mediated inflammatory signaling and attenuates DMBA-induced mammary cell carcinoma in rats. Biomed Pharmacother 155: 113675. doi: 10.1016/j.biopha.2022.113675.
- Saied EM , El-Maradny YA , Osman AA , et al. ( 2021). A comprehensive review about the molecular structure of severe acute respiratory syndrome coronavirus 2 (SARS-CoV-2): insights into natural products against COVID-19. Pharmaceutics 13: 1759 doi: 10.3390/pharmaceutics13111759.
- Salah S , Awad GEA , Makhlouf AIA. ( 2018). Improved vaginal retention and enhanced antifungal activity of miconazole microsponges gel: formulation development and in vivo therapeutic efficacy in rats. Eur J Pharm Sci 114: 255–266. doi: 10.1016/j.ejps.2017.12.023.
- Salem MG , El-Maaty DMA , El-Deen, et al. ( 2022). Novel 1,3-thiazole analogues with potent activity against breast cancer: a design, synthesis, in vitro, and in silico study. Molecules 27: 4898. doi: 10.3390/molecules27154898.
- Sammar FE , Hanan Mohamed E , Soha H , et al. ( 2023a). Biosynthesis and characterization of gold and copper nanoparticles from Salvadora persica fruit extracts and their biological properties. Int J Nanomedicine 17: 6095–6112.
- Sammar FE , Shrouk AG , Raghda H , et al. ( 2023b). Development of thermosensitive hydrogel of Amphotericin-B and Lactoferrin combination-loaded PLGA-PEG-PEI nanoparticles for potential eradication of ocular fungal infections: in-vitro, ex-vivo and in-vivo studies. Int J Pharm X 5: 100174. doi: 10.1016/j.ijpx.2023.100174.
- Shahinaze AF , Mahmoud HT , Mostafa IG , et al. ( 2023). Progress against cancer in Kuwait: trends in incidence, survival and mortality. Gulf J Oncolog 1: 23–31. doi: 10.1080/10717544.2023.2181747.
- Shoman NA , Saady M , Teaima M , et al. ( 2023). Merging konjac glucomannan with other copolymeric hydrogels as a cutting-edge liquid raft system for dual delivery of etoricoxib and famotidine. Drug Deliv 30: 2189630. doi: 10.1080/10717544.2023.2189630.
- Sherje AP , Kulkarni V , Murahari M , et al. ( 2017). Inclusion complexation of etodolac with hydroxypropyl-beta-cyclodextrin and auxiliary agents: formulation characterization and molecular modeling studies. Mol Pharm 14: 1231–1242. doi: 10.1021/acs.molpharmaceut.6b01115.
- Singh D , Tiwary AK , Kang TS , Bedi N. ( 2021). Polymeric precipitation inhibitor based supersaturable self-microemulsifying drug delivery system of canagliflozin: optimization and evaluation. Curr Drug Deliv 18: 1352–1367. doi: 10.2174/1567201818666210217155909.
- Singh D , Singh AP , Singh D , et al. ( 2020). Enhanced oral bioavailability and anti-diabetic activity of canagliflozin through a spray dried lipid based oral delivery: a novel paradigm. Daru 28: 191–208. doi: 10.1007/s40199-020-00330-3.
- Twarog C , Fattah S , Heade J , et al. ( 2019). Intestinal permeation enhancers for oral delivery of macromolecules: a comparison between salcaprozate sodium (SNAC) and sodium caprate (C10). Pharmaceutics 11: 78. doi: 10.3390/pharmaceutics11020078.
- Vlad RA , Antonoaea P , Todoran N , et al. ( 2022). Development and evaluation of cannabidiol orodispersible tablets using a 23-factorial design. Pharmaceutics 14: 1467. doi: 10.3390/pharmaceutics14071467.
- Walsh EG , Adamczyk BE , Chalasani KB , et al. ( 2011). Oral delivery of macromolecules: rationale underpinning Gastrointestinal Permeation Enhancement Technology (GIPET®). Ther Deliv 2: 1595–1610. doi: 10.4155/tde.11.132.
- Xia D , Quan P , Piao H , et al. ( 2010). Preparation of stable nitrendipine nanosuspensions using the precipitation–ultrasonication method for enhancement of dissolution and oral bioavailability. Eur J Pharm Sci 40: 325–334. doi: 10.1016/j.ejps.2010.04.006.
- Younes NF , el Assasy AE-HI , Makhlouf AIA. ( 2021). Microenvironmental PH-modified amisulpride-labrasol matrix tablets: development, optimization and in vivo pharmacokinetic study. Drug Deliv Transl Res 11: 103–117. doi: 10.1007/s13346-019-00706-2.
- Zhao H , Zhao L , Lin X , Shen L. ( 2022). An update on microcrystalline cellulose in direct compression: functionality, critical material attributes, and co-processed excipients. Carbohydr Polym 278: 118968. doi: 10.1016/j.carbpol.2021.118968.
- Zhu Y , Kang Y , Zhu L , et al. ( 2021). Investigation of solubility behavior of canagliflozin hydrate crystals combining crystallographic and Hirshfeld surface calculations. Molecules 26: 298. doi: 10.3390/molecules26020298.