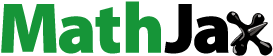
ABSTRACT
We investigated the associations and differences between dynamic strength index (DSI) calculated from different types of vertical jump (countermovement jump; CMJ and squat jump; SJ) and different isometric knee joint angles (30, 60 and 90°), and associations with force-velocity (FV) profile and its constituent variables (maximal theoretical force (V0), power (Pmax) and velocity (V0) and the slope of the FV relationship (Sfv), on a sample of young athletes and active participants (n = 28). There were moderate associations between Pmax and all DSI values (r = 0.42–0.69; p < .05), V0 and all DSI values from CMJ and DSI30 from SJ (r = 0.43–0.56; p < .05), while Sfv was associated only with DSI30 and DSI90 in CMJ (r = 0.40–0.43; p < .05). This suggests that DSI and FV methods provide similar information and can be used in a similar manner to direct an individual’s training.
Introduction
Assessment of strength and power qualities is an important part of sport science research and strength and conditioning practice. Assessing these qualities is one of the ways practitioners can evaluate the efficacy of their training interventions or recognize specific deficits to guide decision-making (Sheppard et al., Citation2008). A thorough assessment of strength and power capacities may be accomplished through testing athlete’s qualities across different loading conditions (for example: multiple progressively loaded jumps) (Morin & Samozino, Citation2016, Jiménez-Reyes et al. Citation2019; Sheppard et al., Citation2011). In this regard, force-velocity (FV) profiling has been extensively researched in recent years (Jiménez-Reyes, Samozino, Brughelli, et al., Citation2017, Jiménez-Reyes et al., Citation2019; Marcote-Pequeño et al., Citation2019), as it has been recognized as a promising tool to obtain a more comprehensive insight into individual’s capacities and deficits, and subsequently, to optimize their training (Jiménez-Reyes, Samozino, Brughelli, et al., Citation2017). The FV relationship is linear in multi-joint tasks, which enables a simple linear equation to calculate its constituent parameters such as maximal theoretical force (i.e., the F-intercept; F0), maximal theoretical velocity (V-intercept; V0) and maximal power (Pmax = F0 V0/4), with the F0 and V0 also determining the slope of the FV relationship (Jaric, Citation2015). Typically, athletes with steeper FV profile slopes (i.e., having relatively high F0 and low V0) are better at generating high forces at low velocities, and vice versa (Jiménez-Reyes, Samozino, Pareja-Blanco, et al., Citation2017). Therefore, FV profiling may be an important metric to consider for training optimization (e.g., emphasizing ballistic training in individuals with steep FV profiles and strength training in individuals with shallower FV profiles).
The dynamic strength index (DSI) is another promising method for obtaining insights into an athlete’s strength and power qualities. The DSI is calculated as the ratio between force production in ballistic conditions (e.g., vertical jumps or bench-press throw) and tasks performed in an isometric or quasi-isometric condition (Comfort, Thomas, Dos’santos, Jones, et al., Citation2018; Sheppard et al., Citation2011; Young et al., Citation2015). The DSI reflects dynamic force-producing capability of an athlete in relation to their maximum-force capacity. Including isometric maximal strength tests (e.g., isometric mid-thigh pull (IMTP)) alongside ballistic task enable the calculation of DSI, which is suggested to provide some meaningful insight into an individual’s training requirements (McMahon et al., Citation2017; Sheppard et al., Citation2011). Theoretically, a DSI < 0.60 is considered low, suggesting that the athlete should focus on ballistic training to produce higher forces during the ballistic task (Sheppard et al., Citation2011). In contrast, high DSI (>0.80) indicates that athlete should focus on maximal strength training to enhance maximum force generation capability (Sheppard et al., Citation2011). It should be noted that those benchmarks are derived from IMTP and squat jump, while exact benchmarks are highly task-dependent; for instance, lower DSI values are reported for unilateral (~0.50–0.60) (Bishop et al., Citation2021) compared to bilateral (~0.75) assessments (Comfort, Thomas, Dos’santos, Jones, et al., Citation2018; Comfort, Thomas, Dos’santos, Suchomel, et al., Citation2018; Sheppard et al., Citation2011; Thomas et al., Citation2015). Furthermore, when using the DSI ratio, it is important to consider the relative effect of each component (force component and ballistic component), as increasing both components simultaneously is likely to result in minimal changes to the DSI, but is likely to be beneficial for performance given improvements in capacity would have been made. Unlike the FV relationship, which is a promising tool for creating a profile of athlete’s strength and speed qualities (Jiménez-Reyes, Samozino, Brughelli, et al., Citation2017, Citation2019), a solid support for using DSI in practice is lacking in the literature. Information regarding the association between FV profile and DSI would offer deeper insight into biomechanical testing for quantifying strength and power qualities in athletes. Specifically, it seems logical to assume that individuals with a high DSI ratio have higher capacity of force production relative to the ability of generating high velocities, and thus, a steeper slope in the FV profile. Knowing whether DSI and FV provide similar information will help coaches decide when to use either (or both) of the metrics to guide training programs. For example, FV testing is performed in a ballistic manner using high loads and therefore may not be suitable for the competitive phase/season for safety reasons, whereas DSI testing may be more suitable in this period.
DSI is traditionally calculated based on peak force (PF) values from countermovement jump (CMJ) and PF obtained during IMTP (Ahn et al., Citation2022; McMahon et al., Citation2017; Sheppard et al., Citation2011). However, it is not entirely clear whether this combination of the tests is optimal. It could be suggested that comparing the PF values between two tasks performed at the corresponding joint angles is a more logical and ecologically valid approach. In addition, PF in CMJ occurs at approximately the lowest body position, when the athlete is transitioning from eccentric to concentric phase (McMahon et al., Citation2018). However, PF in the isometric condition (IMTP task) has been measured at a more extended knee angle (Comfort, Thomas, Dos’santos, Jones, et al., Citation2018, Citation2018; Suchomel et al., Citation2020). Therefore, we suggest that calculating the DSI from PF values obtained in the same body position would provide a more ecologically valid approach. Moreover, previous studies have almost exclusively used the IMTP test, which, unlike the CMJ, requires substantial upper-limb effort. Recent studies show that DSI may be also reliable when obtained from isometric squat test (ISQ) (James & Comfort, Citation2022), which is more similar to CMJ. Given that larger PF is produced in ISQ compared to IMTP, ISQ may be a better test for assessing athlete’s true maximum lower-limb strength (Brady et al., Citation2018). One study reported no association (r = 0.16) between CMJ height and DSI obtained from CMJ and IMTP (Suchomel et al., Citation2020). Further investigation should be conducted to determine whether different approaches to the DSI are related to FV profile and performance indicators such as jump height. Finally, although the reliability of the DSI calculated from IMTP and squat jump (SJ) has also been verified (Thomas et al., Citation2015), no study has yet compared the DSI obtained from CMJ and SJ. Given the slightly higher PF achieved in CMJ compared to SJ (Van Hooren & Zolotarjova, Citation2017), one might expect DSI values to be larger when calculated from CMJ PF compared to SJ PF.
We believe that the currently prevailing combination of tests may not be optimal for computing DSI values, and further investigation into factors such as body position and task type need further investigation. The aim of this study was threefold: a) to investigate whether different DSI variants are correlated (depending on jump type and knee angle during the ISQ), b) to investigate the correlations between different DSI variants and jump height in the CMJ and SJ, and c) to investigate the associations between DSI and FV profile (and its constituent variables). We hypothesized that the different DSI variants would be moderately to strongly correlated. We hypothesized that only the DSI value calculated from the PF obtained from the 90° angle in the isometric task would correlate with jump height. We hypothesized that individuals with larger DSI will tend to show a more velocity-dominant FV profile, and vice versa. The results of this study will reveal the effects of isometric knee angle and jump type on DSI and its relationship with jump height and FV profile, which will provide at least partial insight into the optimal testing procedure for determining DSI and lead to further investigation of appropriate thresholds for practical decision-making.
Methods
Participants
We recruited a convenience sample of 28 young athletes and recreationally active individuals with ≥3 years of experience with resistance exercise (19 men; 9 women; age: 25.2 ± 3.9 years (range: 19–29); body height: 176.6 ± 8.1 cm (range: 160–192 cm); body mass: 74.9 ± 11.1 kg (range: 53–93 kg). The participants were excluded if they reported any musculoskeletal inju ries and pain syndromes within the last year and any other medical conditions that could be exacerbated with the measurement procedure. Participants were informed about the details of protocol and were required to sign an informed consent prior to the beginning of the measurements The protocol was conducted in accordance with the latest revision of the Declaration of Helsinki. The experimental procedures were reviewed and approved by Republic of Slovenia’s National Medical Ethic Committee (no. 0120–690/2017/8).
Study design and procedures
This was a cross-sectional study conducted in a single visit (total time: ~90 min). First, the participants performed a standardized warm-up, consisting of 10 min of low-intensity running on a treadmill and 5 min of dynamic stretching (e.g., lateral lunges, straight leg kicks, dynamic arm rotations, trunk rotations) followed by eight familiarization repetitions of SJs and CMJs. For these repetitions, the participants were instructed to gradually increase the effort from ~ 50 to ~ 90% of perceived maximal effort. The warm-up was followed by SJ and CMJ assessment (the order between the two jump tasks was randomized), FVP profile assessments (loaded jumps) and isometric squat testing at three different angles (with the order among the angles also randomized). For all tests across all conditions, three repetitions were performed and the average value was taken for further analyses. There was a 15-min break between jumping tests and isometric assessments.
Bodyweight SJ and CMJ
For both jumps, the participants were instructed to “jump as high as possible”. The depths of the CMJ and SJ were standardized at 90° knee angle, determined with a goniometer. For SJ, an elastic band was positioned for each individual on the appropriate height to be in contact with participants’ buttocks when the desired angle was reached. For the CMJ, the elastic band was moved away from participants to avoid contact during the jump, and one examiner stood nearby the participant to ensure that the appropriate CMJ depth had been reached. A lightweight (<0.5 kg) plastic bar was held by participants on the back of their shoulders to replicate the position in further loaded jump tests. Immediately after each repetition, the force-time traces were inspected by the examiners and any irregularities resulted in trials being void and subsequently repeated after the aforementioned rest period (e.g., countermovement in SJ task). The breaks between repetitions were set at 60 s. Ground reaction force data during all tests were sampled with a piezoelectric force plate (Type 9229A, Kistler Instruments, Winterthur, Switzerland) at 1000 Hz and stored within the MARS Software (Kistler, Winterthur, Switzerland), which enables immediate and reliable calculation of the biomechanical jump variables.
Jumps with additional weights
The bodyweight jumps (CMJ and SJ) were followed by jumps performed with a barbell added (20 kg) and then load was subsequently added in 10-kg increments thereafter. The procedures were terminated when participants exhibited or reported any difficulties in maintaining balance or insecurity regarding execution of the jumping task. In addition, the procedure was terminated when the jump height was <7.5 cm. It has been suggested that the reliability of loaded jump assessments begins to decrease once the jump height is below 10 cm (Perez-Castilla & Garcia-Ramos, Citation2018). Three repetitions of each jump were performed at each load. The order of the jump types (SJ and CMJ) was randomized among participants, but was kept the same for each participant at different loads. The break between repetitions was set at 60 s, and the break between different loads was set at ~2 min. Longer breaks (3–5 min) were provided at higher loads if needed.
Isometric squat testing
Isometric squat testing was performed in three positions that represented different push-off angles during vertical jumps. The positions were determined by the knee angles of 30°, 60° and 90°, which were verified with a goniometer (joint angle was determined as the internal joint angle, with one leg of goniometer directed toward greater trochanter and the other one toward lateral malleoli). The order of knee angles was randomized among participants. Participants were required to exert maximal force against a bar, tightly strapped to the floor in a way that allowed it to be lifted only to a height corresponding to the respective knee angle condition. After two warm-up repetitions at ~ 50% and ~ 75% of the maximal effort at the middle (60°) knee angle, three repetitions with maximal exertion of 3s were performed at each knee angle, with 2-min breaks provided between repetitions and between knee angles.
Data analysis
In jumping tasks, the vertical acceleration of the participant’s CoM was calculated via Newton’s second law of motion (F = m × a); subsequently, the acceleration was integrated to obtain vertical velocity using the trapezoid rule. Jump height was calculated from the takeoff velocity (TOV) as follows (Chiu & Dæhlin, Citation2020):
In addition, peak force was noted for both unloaded jumps to enable DSI calculation. Power was defined as the instantaneous product of force and velocity, with the average force, average velocity and average power during the push off phase being used to determine the F-V profile. Based on the average force and average velocity data across jumps with different loads, the F – V profile was calculated using least squares linear regressions. The intercepts with force axis (F0) and velocity axis (V0) were considered to represent participants’ maximal capacity for force and velocity expression. Slope of the F-V profile (Sfv) was calculated as (Jiménez-Reyes, Samozino, Pareja-Blanco, et al., Citation2017):
Theoretical maximal power (Pmax) was determined as (Jiménez-Reyes, Samozino, Pareja-Blanco, et al., Citation2017):
In ISQ test, the peak force was taken as the largest mean force in 1-s intervals. Subsequently, DSI variables were calculated from peak force obtained in SJ and CMJ, and peak force obtained during ISQ test at 30° (DSI30), 60° (DSI60) and 90° (DSI90) knee angles (6 DSI variables in total) as follows (Comfort, Thomas, Dos’santos, Suchomel, et al., Citation2018; Sheppard et al., Citation2011):
Statistical analysis
The data are presented as means ± standard deviations. The normality of the data distributions for all variables was verified with Shapiro-Wilk test (all p ≥ .095). The reliability across repetitions was evaluated with intra-class correlation coefficient (ICC; single measures, absolute agreement). We considered ICC values < 0.5 as indicative of poor reliability, values between 0.5 and 0.75 as moderate reliability, values between 0.75 and 0.9 as good reliability, and values greater than 0.90 as excellent reliability (Koo & Li, Citation2016). Two-way analysis of variance (ANOVA) was used to determine the influence of knee angle during isometric squat (30°, 60° and 90°), jump task (SJ and CMJ) on DSI. Outcomes were compared between SJ and CMJ with paired t-test, with the effect sizes expressed as Cohen’s d and considered as trivial (<0.2), small (0.2–0.5), medium (0.5–0.8) and large (>0.8) (Cohen, Citation1988). Pearson correlation coefficients were used to examine the association between jump heights, DSI variables and F-V variables. The associations were interpreted as following: 0.0–0.1 (no association), 0.1–0.4 (weak), 0.4–0.6 (moderate), 0.6–0.8 (strong) and > 0.8 (very strong) (Akoglu, Citation2018). The threshold for statistical significance was set at α < 0.05 and all analyses were carried out in SPSS statistical software (version 25.0, IBM, USA).
Results
There was a substantial between-participant variability of the highest load that the jumps could be performed with (range: 40–100 kg). Nevertheless, the FV-relationships achieved sufficient R2 values in both jumps for all participants (SJ: R2 = 0.94 ± 0.02, range: 0.88–0.98; CMJ: R2 = 0.96 ± 0.03, range: 0.87–0.99). The reliability (across trials) of PF in ISQ was excellent at all angles (ICC = 0.91–0.95). Similarly, jump heights and PF during both SJ and CMJ were highly reliable (ICC = 0.92–0.98), which altogether results in reliable DSI values (ICC = 0.89–0.93).
The descriptive statistics for all outcome variables are available in . CMJ height (p < .001; d = 1.25) and CMJ peak force (p < .001; d = 1.18) were statistically significantly higher compared to SJ. In addition, larger V0 and Pmax were noted for CMJ than for SJ (p < .001; d = 3.44 and 3.88, respectively). In contrast, SJ force-velocity profile was characterized by higher F0 (p = .015; d = 0.50) and was significantly steeper (p < .001; d = 2.31).
Table 1. The descriptive statistics for all outcome variables included in the study.
Associations among different dynamic strength index variants
shows correlations between different DSI variants. Within CMJ-based DSI variables, there were very strong associations between DSI60 and DSI90 (r = 0.81; p < .001) and between DSI60 and DSI30 (r = 0.82; p < .001), while the association between DSI90 and DSI30 was moderate (r = 0.59, p = .001). Similarly, for SJ-based DSI variables, there were very strong associations between DSI60 and DSI90 (r = 0.82; p < .001) and between DSI60 and DSI30 (r = 0.84; p < .001), and strong between DSI90 and DSI30 (r = 0.67, p = .001). In addition, DSI for CMJ and SJ obtained at the same angle were strongly correlated (r = 0.70–0.84; p < .001), while the correlations among non-corresponding angles were weak to moderate (r = 0.37–0.59; p = .002–0.049). also shows the DSI values across both jump tasks and knee angles.
Table 2. Correlation coefficients between dynamic strength index in CMJ and SJ.
Associations between different dynamic strength index variants and force-velocity profile
shows the associations between DSI and force-velocity profile variables for each jump. For CMJ, all DSI variables displayed positive moderate and statistically significant correlations with V0 (r = 0.44–0.56) and Pmax (r = 0.42–0.69), and Sfv was also positively correlated with DSI30 and DSI90 (r = 0.40–0.43). In SJ, all DSI variables were in positive moderate statistically significant correlation with Pmax (r = 0.46–0.55). In addition, DSI30 in SJ was positively correlated with V0 (r = 0.43). Additional analysis shows that there were no associations between ISQ PF and FV variables in both jumps (). However, CMJ PF was associated with V0, Pmax and Sfv (r = 0.41–0.62), while SJ PF was moderately associated with F0 (r = 0.40) and highly associated with Pmax (r = 0.73).
Table 3. Correlation coefficients between dynamic strength index and force-velocity profile outcomes.
Associations with jump height
For both CMJ and SJ, only DSI90 showed positive moderate to strong associations with jump height (r = 0.41 for CMJ and r = 0.63 for SJ; p = .033 and 0.001, respectively). Jump heights were also strongly correlated with Pmax (r = 0.79 for CMJ and r = 0.73 for SJ; both p < .001), and moderately correlated with V0 (r = 0.45 for CMJ and r = 0.41 for SJ; p = .018 and 0.032, respectively). CMJ height was also moderately associated with F0 (r = 0.49; p = .009). Neither SJ nor CMJ height were associated with PF in ISQ regardless of the knee flexion angle (r = 0.02–0.21; all p > .05). CMJ height was also not associated with CMJ PF (r = 0.31; p = .105), while SJ height was largely and statistically significantly associated with SJ PF (r = 0.72; p < .001).
Discussion
The aim of the study was to examine the associations between DSI values and jump height, FV profile and its constituent variables. We observed very strong associations between DSI in nearby joint angles (i.e., 30 and 60°, 60 and 90°) within the same jump type and strong associations between DSI at the same angle in different jump types, which suggest that at least somewhat similar information is provided with different DSI values. Moreover, jump heights were in moderate to strong association only with DSI90. While further studies are needed to confirm this, it is suggested that using 90° knee angle in ISQ test may provide DSI values that best correlate with jumping performance. The fact that CMJ height was not associated with ISQ PF, nor with CMJ PF, points to the added value of calculating the DSI. Finally, regarding the associations between FV and DSI, we found: a) moderate associations between Pmax and all DSI values, V0 and all DSI values from CMJ and DSI30 from SJ, while Sfv was associated only with DSI30 and DSI90 in the CMJ. This suggest that DSI and FV methods provide similar information and can be used in a similar manner to direct an individual’s training.
The first observation of this study is that jump type and joint angle in isometric task influence DSI values and its relationship to FV profile. While as additional research is needed to specifically address this, deeper joint angles in ISQ yield significantly higher values of DSI as a consequence of lower peak force production during ISQ. Those results were expected, as previous studies have shown that a deeper squat position exhibited lower force production (Fisher et al., Citation2016; Lynch et al., Citation2021). Specifically, the 30° knee angle during the squat is thought to be approximately optimal for generating maximal force (McLaughlin et al., Citation1977). On the other hand, the 90° knee angle approximates the point of momentary muscular failure during the maximal lift (i.e., sticking point) (Drake et al., Citation2018), explaining lower PF at this angle in the present study. However, DSI values obtained at 90° was also the only one to show correlations with jump height (in both SJ and CMJ) and showed larger associations with FV profile than the DSI obtained at 30 and 60° knee angles. In addition, the correlation analysis between DSI values and jump height indicates that the ISQ test with 90° knee angle might be the optimal with regard to relevance for performance. These results are in line with movement specificity/similarity as the study by (Suchomel et al. (Citation2020) reported no association (r = 0.16) between jump height and DSI obtained from CMJ and IMTP. However, further studies using performance metrics other the jump height are needed to fully corroborate this suggestion.
Assessing strength and power qualities provides coaches and athletes with information and feedback about an individual’s neuromuscular function, which enables insight into the athlete’s weaknesses and strengths, based on which individual components of training might need adjusting. The foundations for using the SI and FV for optimizing training are well established in the literature (Jiménez-Reyes, Samozino, Brughelli, et al., Citation2017; Jiménez-Reyes, Samozino, Pareja-Blanco, et al., Citation2017; McMahon et al., Citation2017; Sheppard et al., Citation2011). Higher values of DSI indicate that an individual is efficient in producing high force in a ballistic task; thus, should be navigated more toward strength training to increase their ceiling for force production and vice versa (Sheppard et al., Citation2011). Positive correlations between DSI and V0 indicate that the DSI training theory goes in line with the FV relationship training theory. Specifically, it seems that individuals with higher DSI scores have a more velocity dominant profile (higher V0) and are relatively better in producing high velocities with lower forces (Jiménez-Reyes, Samozino, Brughelli, et al., Citation2017). Conversely, individuals with lower DSI scores have a steeper FV slope (i.e., can express a lower percentage of their maximal isometric force ability at higher velocities), which implies that they need to put more emphasis on ballistic training. Knowing that the DSI and FV theories provide somewhat similar information allows practitioners to use whichever is more appropriate at a given time to guide decision making regarding training. For example, the FV investigation is performed in a ballistic manner using quite high loads (García-Ramos, Pérez-Castilla, Jaric, et al., Citation2018), which may not be appropriate during the (competition) season or the tapering phase just before competition due to safety concerns and athlete uncertainty, while, on the other hand, DSI testing might be more appropriate in this case, considering that high force testing is performed under isometric or quasi-isometric conditions, which are usually to some extent already integrated into the athletes’ training program (i.e. h., that athletes are already accustomed to training).This means that athletes are accustomed and adapted to this type of loading, so the test itself does not create additional stressful situations and is safer in this competition). Nevertheless, coaches should keep in mind that the correlations we observed were only moderate. Thus, although this shows a coherence between the theories of DSI and FV, some different information can be obtained with each of these approaches.
The ultimate goal of DSI studies is to provide practitioners with solid guidelines for decision-making related to training design. To our knowledge, there is only one study that investigated the changes in DSI after training (Comfort, Thomas, Dos’santos, Suchomel, et al., Citation2018). They reported a decrease in DSI scores in a “high DSI” group after 4-weeks of strength training, which was mainly due to the increase in isometric mid-thigh pull peak force, with minimal change in CMJ PF. This indicates that DSI should always be interpreted together with both underlying PF variables. Future interventional studies should be performed to determine if DSI is useful for training prescription (e.g., focusing on strength development in case of a high DSI). The present study demonstrated that current choices of assessment task and body positions significantly affects the DSI values. Therefore, caution is needed in further research and practice when determining training needs based on DSI values, as the current criteria (<0.60 and > 0.80 as low and high DSI, respectively) apply only to the DSI obtained from SJ and IMTP performed at ~ 40–50° knee angle. As shown in the current study, DSI values are higher when higher knee flexion is used in ISQ task, and further studies are needed to compare the DSI values between IMTP and ISQ tasks. Furthermore, the DSI seems to be lower when assessed through unilateral tasks (Bishop et al., Citation2021), which is another important consideration in terms of practical application of the DSI.
Several limitations of the present study should be acknowledged. The sample size of the study was relatively small, considering the number of statistical tests performed, which means that there was some potential for type 1 error. Further studies with higher sample sizes are therefore needed. While sufficient rest was provided between jumps, it cannot be excluded that fatigue compromised the performance when loads were highest, which could have affected the FV relationship assessment. Moreover, while knee angle was standardized, trunk and ankle position were not, thus more rigorous standardization of the tasks could have affected the results. Finally, the included sample were young athletes and recreationally active individuals with high heterogeneity regarding jump heights and the highest load that they could perform the jumps with. Thus, including athletes from a specific sport may provide different results.
Conclusion
This study investigated the associations between DSI values and FV profile. Correlations between DSI and FV additionally indicate the potential usefulness of the DSI metric for prescribing specific training based on individual’s strength and power qualities. Moreover, illustrated coherence between DSI and FV theories provide coaches with information that enables them to use the tool that suits them better at certain time point (i.e., DSI is safer and more likable by athletes due to the nature of the examination of high-forces – more suitable to use during the competitive season, while FV may provide more in-depth information – more suitable to use during the off-season) to guide training related decision making. Nevertheless, further interventional studies are warranted to determine the usefulness of this metric to guide training related decision making. Caution should be exercised in further research when interpreting the DSI values, as the current criteria apply only to DSI obtained from CMJ and IMTP performed at 40–50° knee angle.
Disclosure statement
No potential conflict of interest was reported by the authors.
Additional information
Funding
References
- Ahn, N., Kim, H., Krzyszkowski, J., Roche, S., & Kipp, K. (2022). Joint-level determinants of the dynamic strength index: Implications for testing and monitoring. Sports Biomechanics, 1–11. https://doi.org/10.1080/14763141.2022.2131614
- Akoglu, H. (2018). User’s guide to correlation coefficients. Turkish Journal of Emergency Medicine, 18, 91–93. https://doi.org/10.1016/j.tjem.2018.08.001
- Bishop, C., Read, P., Lake, J., Loturco, I., & Turner, A. (2021). A novel approach for athlete profiling: the unilateral dynamic strength index. Journal of Strength & Conditioning Research, 35(4), 1023–1029. https://doi.org/10.1519/JSC.0000000000002871
- Brady, C. J., Harrison, A. J., Flanagan, E. P., Haff, G. G., & Comyns, T. M. (2018). A comparison of the isometric midthigh pull and isometric squat: Intraday reliability, usefulness, and the magnitude of difference between tests. International Journal of Sports Physiology & Performance, 13(7), 844–852. https://doi.org/10.1123/ijspp.2017-0480
- Chiu, L. Z. F., & Dæhlin, T. E. (2020). Comparing numerical methods to estimate vertical jump height using a force platform. Measurement in Physical Education and Exercise Science, 24(1), 25–32. https://doi.org/10.1080/1091367X.2019.1650044
- Cohen, J. (1988). Statistical power analysis for the behavioral sciences. Lawrence Erlbaum Associates.
- Comfort, P., Thomas, C., Dos’santos, T., Jones, P. A., Suchomel, T. J., & McMahon, J. J. (2018). Comparison of methods of calculating dynamic strength index. International Journal of Sports Physiology & Performance, 13(3), 320–325. https://doi.org/10.1123/ijspp.2017-0255
- Comfort, P., Thomas, C., Dos’santos, T., Suchomel, T., Jones, P., & McMahon, J. (2018). Changes in dynamic strength index in response to strength training. Sports, 6(4), 176. https://doi.org/10.3390/sports6040176
- Drake, D., Kennedy, R., & Wallace, E. (2018). Familiarization, validity and smallest detectable difference of the isometric squat test in evaluating maximal strength. Journal of Sports Sciences, 36(18), 2087–2095. https://doi.org/10.1080/02640414.2018.1436857
- Fisher, H., Stephenson, M. L., Graves, K. K., Hinshaw, T. J., Smith, D. T., Zhu, Q., Wilson, M. A., & Dai, B. (2016). Relationship between force production during isometric squats and knee flexion angles during landing. Journal of Strength & Conditioning Research, 30(6), 1670–1679. https://doi.org/10.1519/JSC.0000000000001264
- García-Ramos, A., Haff, G. G., Pestaña-Melero, F. L., Pérez-Castilla, A., Rojas, F. J., Balsalobre-Fernández, C., & Jaric, S. (2018). Feasibility of the 2-point method for determining the 1-repetition maximum in the bench press exercise. International Journal of Sports Physiology & Performance, 13(4), 474–481. https://doi.org/10.1123/ijspp.2017-0374
- James, L. P., & Comfort, P. (2022). The reliability of novel, temporal-based dynamic strength index metrics. Sports Biomechanics, 1–12. https://doi.org/10.1080/14763141.2022.2032298
- Jaric, S. (2015). Force-velocity relationship of muscles performing multi-joint maximum performance tasks. International Journal of Sports Medicine, 36(9), 699–704. https://doi.org/10.1055/s-0035-1547283
- Jiménez-Reyes, P., Samozino, P., Brughelli, M., & Morin, J. B. (2017). Effectiveness of an individualized training based on force-velocity profiling during jumping. Frontiers in Physiology, 7. https://doi.org/10.3389/fphys.2016.00677
- Jiménez-Reyes, P., Samozino, P., Morin, J. B., & Boullosa, D. (2019). Optimized training for jumping performance using the force-velocity imbalance: Individual adaptation kinetics. PLoS One, 14(5), e0216681. https://doi.org/10.1371/journal.pone.0216681
- Jiménez-Reyes, P., Samozino, P., Pareja-Blanco, F., Conceição, F., Cuadrado-Peñafiel, V., González-Badillo, J. J., & Morin, J.-B. (2017). Validity of a simple method for measuring force-velocity-power profile in countermovement jump. International Journal of Sports Physiology & Performance, 12(1), 36–43. https://doi.org/10.1123/IJSPP.2015-0484
- Koo, T. K., & Li, M. Y. (2016). A guideline of selecting and reporting intraclass correlation coefficients for reliability research. Journal of Chiropractic Medicine, 15(2), 155–163. https://doi.org/10.1016/j.jcm.2016.02.012
- Lynch, A. E., Davies, R. W., Jakeman, P. M., Locke, T., Allardyce, J. M., & Carson, B. P. (2021). The influence of maximal strength and knee angle on the reliability of peak force in the isometric squat. Sports, 9(10), 140. https://doi.org/10.3390/sports9100140
- Marcote-Pequeño, R., García-Ramos, A., Cuadrado-Peñafiel, V., González-Hernández, J. M., Gómez, M. A., & Jiménez-Reyes, P. (2019). Association between the force-velocity profile and performance variables obtained in jumping and sprinting in elite female soccer players. International Journal of Sports Physiology and Performance, 14(2), 209–215. https://doi.org/10.1123/ijspp.2018-0233
- McLaughlin, T. M., Dillman, C. J., & Lardner, T. J. (1977). A kinematic model of performance in the parallel squat by champion powerlifters. Medicine & Science in Sports and Exercise, 9(2), 128–133. https://doi.org/10.1249/00005768-197709020-00011
- McMahon, J. J., Jones, P. A., Dos’santos, T., & Comfort, P. (2017). Influence of dynamic strength index on countermovement jump force-, power-, velocity-, and displacement-time curves. Sports, 5(4), 72. https://doi.org/10.3390/sports5040072
- McMahon, J. J., Suchomel, T. J., Lake, J. P., & Comfort, P. (2018). Understanding the key phases of the countermovement jump force-time curve. Strength & Conditioning Journal, 40(4), 96–106. https://doi.org/10.1519/SSC.0000000000000375
- Morin, J. B., & Samozino, P. (2016). Interpreting power-force-velocity profiles for individualized and specific training. International Journal of Sports Physiology & Performance, 11(2), 267–272. https://doi.org/10.1123/ijspp.2015-0638
- Perez-Castilla, A., & Garcia-Ramos, A. (2018). Evaluation of the most reliable procedure of determining jump height during the loaded countermovement jump exercise: Take-off velocity vs. flight time. Journal of Strength & Conditioning Research, 32(7), 2025–2030. https://doi.org/10.1519/JSC.0000000000002583
- Sheppard, J. M., Chapman, D., & Taylor, K.-L. (2011). An evaluation of a strength qualities assessment method for the lower body. Journal of Australian Strength and Conditioning, 19, 4–10.
- Sheppard, J. M., Cormack, S., Taylor, K. L., McGuigan, M. R., & Newton, R. U. (2008). Assessing the force-velocity characteristics of the leg extensors in well-trained athletes: The incremental load power profile. Journal of Strength & Conditioning Research, 22(4), 1320–1326. https://doi.org/10.1519/JSC.0b013e31816d671b
- Suchomel, T. J., Sole, C. J., Bellon, C. R., & Stone, M. H. (2020). Dynamic strength index: relationships with common performance variables and contextualization of training recommendations. Journal of Human Kinetics, 74(1), 59–70. https://doi.org/10.2478/hukin-2020-0014
- Thomas, C., Jones, P. A., & Comfort, P. (2015). Reliability of the dynamic strength index in college athletes. International Journal of Sports Physiology & Performance, 10(5), 542–545. https://doi.org/10.1123/ijspp.2014-0255
- Van Hooren, B., & Zolotarjova, J. (2017). The difference between countermovement and squat jump performances: a review of underlying mechanisms with practical applications. Journal of Strength & Conditioning Research, 31(7), 2011–2020. https://doi.org/10.1519/JSC.0000000000001913
- Young, K. P., Haff, G. G., Newton, R. U., Gabbett, T. J., & Sheppard, J. M. (2015). Assessment and monitoring of ballistic and maximal upper-body strength qualities in athletes. International Journal of Sports Physiology & Performance, 10(2), 232–237. https://doi.org/10.1123/ijspp.2014-0073