Abstract
Lung carcinomas and pulmonary fibrosis (asbestosis) occur in asbestos workers. Understanding the pathogenesis of these diseases is complicated because of potential confounding factors, such as smoking, which is not a risk factor in mesothelioma. The modes of action (MOA) of various types of asbestos in the development of lung cancers, asbestosis, and mesotheliomas appear to be different. Moreover, asbestos fibers may act differentially at various stages of these diseases, and have different potencies as compared to other naturally occurring and synthetic fibers. This literature review describes patterns of deposition and retention of various types of asbestos and other fibers after inhalation, methods of translocation within the lung, and dissolution of various fiber types in lung compartments and cells in vitro. Comprehensive dose-response studies at fiber concentrations inhaled by humans as well as bivariate size distributions (lengths and widths), types, and sources of fibers are rarely defined in published studies and are needed. Species-specific responses may occur. Mechanistic studies have some of these limitations, but have suggested that changes in gene expression (either fiber-catalyzed directly or by cell elaboration of oxidants), epigenetic changes, and receptor-mediated or other intracellular signaling cascades may play roles in various stages of the development of lung cancers or asbestosis.
In addition to malignant mesotheliomas (MM), two pulmonary diseases linked to exposure to asbestos fibers in the workplace are lung carcinomas and asbestosis. Asbestos exposure is less of a risk factor than smoking in the causation of lung carcinomas (CitationSelikoff et al., 1968). However, the incidence of lung cancers increases additively or synergistically in asbestos workers who smoke, and depends upon the cohort (CitationMossman & Gee, 1989; CitationSaracci, 1987; CitationMcDonald & McDonald, 1987). Historically, some investigators reported that adenocarcinomas and tumors developing in the lower, peripheral lung lobes are most commonly seen in asbestos-exposed lung cancer patients (CitationSoutar et al., 1974; CitationWeiss, 2000). The predominant hypothesis put forth is that pulmonary fibrosis (asbestosis) arises in the peripheral lung at sites of deposition of asbestos fibers and creates a favorable environment for development of lung cancers. Proliferating lung fibroblasts in these lesions may promote proliferation or metaplasia of lung epithelial cells through elaboration of growth factors or cell–cell communication (CitationMossman & Churg, 1998). Some studies also suggest that there is an increased risk for lung cancer in individuals with clinical asbestosis, whereas others speculate the asbestosis and asbestos-induced lung cancer are independent processes (CitationKannerstein & Churg, 1972; CitationRom, 1998; CitationHaus et al., 2001). Cigarette smoke may also be a risk factor or may exacerbate the pathogenesis of asbestosis (CitationWeiss, 1984; CitationATS 2004).
This report describes the patterns of deposition and retention of various types of fibers after inhalation, mechanisms of translocation within the lung, and dissolution of various fiber types both in lung compartments and in assays in vitro. Much of the report also focuses on mechanisms of action of asbestos fibers, as suggested by studies using in vitro models, analyses of human lungs, and inhalation experiments using rodents. Investigations in which fibers were administered by intratracheal instillation or pharyngeal administration or studies where asbestos fibers have been injected into the lung are not discussed because of their artificial nature, bypassing of normal clearance mechanisms, and association with lung overload.
DEPOSITION AND TRANSLOCATION OF ASBESTOS FIBERS IN THE LUNG
Fiber Deposition in the Respiratory Tract
Differences in airway anatomy and architecture occur as a result of age, interspecies variations, and other factors that influence fiber deposition. There are five mechanisms that are important with respect to the deposition of fibers in respiratory tract airways. These are interception, impaction, sedimentation, diffusion, and electrostatic precipitation. The relative contribution of each of these mechanisms varies in different regions of the respiratory tract (). Interception, a mechanism by which deposition occurs when the ends of the inhaled fibers intersect with the airway architecture, is an important mechanism of deposition for fibers, especially in the upper respiratory tract and at initial bifurcations of the tracheobronchial tree, where inhaled airflow is at a high velocity and encounters large directional changes. Impaction occurs when the inertia of the fiber forces it out of the airstream (CitationStöber et al., 1970; CitationTimbrell, 1972). Most impaction occurs downstream of air jets in the larger airways, where the flow velocities are high and the momentum of a fiber propels it out of the bending flow streamlines and onto relatively small portions of the epithelial surfaces (CitationBalashazy et al., 2005; CitationSu & Cheng, 2006). Sedimentation, whereby the fiber deposits due to gravitational forces, is favored by low flow velocity, long residence times, and smaller airway sizes. Interception, impaction, and sedimentation probabilities are governed by the aerodynamic diameter of the fibers, which, for long mineral fibers, may be estimated as approximately three times their physical diameters (CitationStöber et al.,1970; CitationTimbrell, 1972). However, it needs to be noted that the term aerodynamic “diameter” is a misnomer for fibers since both the length and width of a fiber determine its aerodynamic behavior (CitationCheng, 1986; CitationVincent, 2005). Aerodynamic equivalent (d ef) is defined as a function of the bivariate distribution (CitationCheng, 1986). Further, it has become an almost universal convention that fibers need to be assessed in terms of their number concentration, and need to be selected according to criteria that relate to shape and dimensions when fibers are collected on a membrane filter and viewed by microscopy for dimensional measurement post air sampling (CitationVincent, 2005). Different definitions of “respirable fibers” may exist. For example, a respirable fiber is defined by NIOSH (NIOSH 7400) as one with length (lf) greater than 5 μm and aspect ratio (lf/df) ≥3, where df is fiber width. The Asbestos International Association (AIA) criteria specify that, in addition to lf >5 μm and lf/df ≥3, the df should be <3 μm.
FIGURE 1. Fiber deposition in the lung. Lines within the airways show net axial core flow through the trachea (site of impaction), bronchi, and bronchioles (CitationLippmann & Schlesinger 1984).
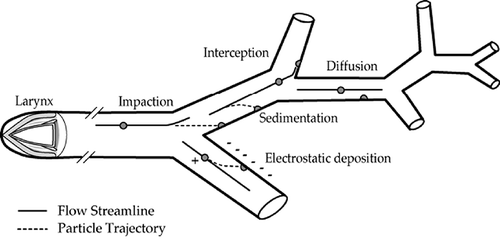
Diffusional displacement results from collisions between air molecules and airborne particles. For compact particles, diffusion becomes an important deposition mechanism for diameters smaller than about 0.5 μm. Fibers of similar diameter would be more massive and therefore be displaced less by a single molecular impact. Long fibers may have millions of impacts from air molecules, and their random trajectories may tend to damp the net displacement. In contrast, a single collision near a fiber end may rotate the fiber sufficiently to alter its interception probability. The role of diffusion in fiber deposition is poorly understood. CitationGentry et al. (1983) measured the diffusion coefficients of chrysotile and crocidolite asbestos fibers and found good agreement with theoretical predictions for chrysotile (0.4 μm mean diameter) but poor agreement with the more rod-like crocidolite (0.3 μm mean diameter).
Electrostatic precipitation occurs when charged particles induce opposite changes on airway surfaces and is dependent on the ratio of electrical charge to aerodynamic drag. CitationJones et al. (1983) showed that asbestos fiber processing operations generate fibrous aerosols with relatively high charge levels, and that these charge levels are sufficient to produce an enhancement of fiber deposition in the lungs. Such an enhancement of fiber deposition for chrysotile asbestos was seen in rats that were exposed by inhalation (CitationDavis, 1976). Interception increases with fiber length. The greater the length, the more likely it is that the position of a fiber end will cause it to touch a surface that the center of mass would have missed.
Obviously, the dimensions of the airway architecture in different species interact with these mechanisms of deposition of the inhaled fibers to define deposition efficiency in the various regions of the respiratory tract. The conductive airway region of the human lung consists of a series of bifurcating airways with the daughter airways being of nearly equal size. In contrast, bifurcations in animal lungs lead to daughter airways of different sizes. The major daughter airway is only slightly smaller than the parent airway, and the smaller daughters resemble side branches. In the human lung, the trachea is the only airway segment with a length-to-diameter ratio much greater than 3. Single symmetrical fibers suspended in a laminar flow stream tend to become aligned with the flow axis as they move through a lung airway. On the contrary, fiber agglomerates or nonfibrous particles would have more random orientations that would depend on their distributions of masses and drag forces. A fiber whose flow orientation differs from axial alignment would have an enhanced probability of deposition by interception.
A fiber's alignment is radically altered as it enters a daughter airway in the human lung, and this loss of alignment with the flow at the entry contributes to its deposition by interception at or near the cranial edge. To the extent that a fiber is entrained in the secondary flow streams that form at bifurcations, its deposition probability by interception is further enhanced.
FIBER DEPOSITION STUDIES
CitationSussman et al. (1991a) performed an experimental study of fiber deposition within the larger tracheobronchial airways of the human lung using replicate hollow airway casts. For crocidolite fibers with diameters primarily in the 0.5–0.8 μm range, interception elevated total deposition, with the effect increasing with fiber length, especially for fibers >10 μm in length. The effect was more pronounced at 60 L/min than at 15 L/min. This is consistent with greater axial alignment of the fibers during laminar flow within the airway.
CitationMorgan and Holmes (1984) and CitationMorgan et al. (1980) exposed rats for several hours by inhalation (nose only) to glass fibers 1.5 μm in diameter and 5, 10, 30, or 60 μm long. For fibers longer than 10 μm, essentially all were deposited, mostly in the head. These data, together with the results of their earlier studies on asbestos fibers, indicate that inhalability and penetrability of airborne fibers into the rat lung drops sharply with aerodynamic diameter above 2 μm. The results by CitationMorgan and Holmes (1984) provide experimental verification that increasing fiber length enhances lung deposition within the tracheobronchial airways. CitationSussman et al. (1991b) found that the deposition patterns of fibers in the larger lung airways were similar to those for particles of more compact shapes. In essence, the added deposition due to interception increased the deposition efficiencies without changing the pattern of deposition.
Most of the studies on particle deposition patterns and efficiencies in hollow bronchial airway casts of the larynx and the larger conductive airways of the human bronchial tree focused on deposition during constant flow inspirations. For studies of deposition during cyclic inspiratory flows, CitationGurman et al. (1984a, Citation1984b) used a variable-orifice mechanical larynx model (CitationGurman et al., 1980) at the inlet in place of the fixed-orifice laryngeal models used in the prior constant-flow tests. In one series of tests, two replicate casts were connected in tandem. The corresponding terminal endings were connected with rubber tubing. Deposition in the downstream cast was analyzed to determine the deposition pattern and efficiencies during expiratory flow (CitationSchlesinger et al.,1983). Concern about sites of enhanced surface deposition density is stimulated by the observation that the larger bronchial airway bifurcations, which are favored sites for deposition, are also the sites most frequently reported as primary sites for bronchial cancer (CitationSchlesinger and Lippmann 1978).
Deposition patterns within the nonciliated airways distal to the terminal bronchioles may also be quite nonuniform. CitationBrody et al. (1981) studied the deposition of chrysotile asbestos in lung peripheral airways of rats exposed for 1 h to 4.3 mg/m3 of respirable chrysotile. The animals were euthanized in groups of 3 at 0, 5, and 24 h and at 4 and 8 d after the end of the exposure. The pattern of retention on the epithelial surfaces was examined by scanning electron microscopy (SEM) of lung sections cut to reveal terminal bronchiolar surfaces and adjacent airspaces. The rat does not have recognizable respiratory bronchioles, and the airways distal to the terminal bronchioles are the alveolar ducts. In rats euthanized immediately after exposure, asbestos fibers were rarely seen in alveolar spaces or on alveolar duct surfaces except at alveolar duct bifurcations. There were relatively high concentrations on bifurcations nearest the terminal bronchioles and lesser concentrations on more distal duct bifurcations. In rats euthanized at 5 h, the patterns were similar, but the concentrations were reduced. Subsequent studies showed that crocidolite asbestos (CitationRoggli et al.,1987), Kevlar aramid synthetic fibers (CitationLee et al.,1983), and particles of more compact shape (CitationBrody & Roe, 1983) deposit in similar patterns, and that the deposition patterns seen in the rat also occur in mice, hamsters, and guinea pigs (CitationWarheit & Hartsky, 1990). The sudden enlargement in air path cross section at the junction of the terminal bronchiole and alveolar duct may play a role in the relatively high deposition efficiency at the first alveolar duct bifurcation. Few data are available on the flow profiles in this region of the lung. However, CitationBriant (1988) demonstrated a net axial core flow in a distal direction and a corresponding net annular flow in a proximal direction during steady-state cyclic flow in tracheobronchial airways, and that this might account for such concentrated deposition on the bifurcations of distal lung airways.
CitationBernstein (2007) summarized the respiratory and physiological parameters in rat and human and their influence on fiber deposition in the lung (). It is noteworthy that in this table, 100% deposition was assumed. Deposition is a function of fiber dimension and species. In general, it is estimated that the deposition of such fibers is closer to between 10 and 20%.
TABLE 1. Respiratory and Physiological Parameters in the Rat and Human and Their Influence on Fiber Deposition
FIBER RETENTION, TRANSLOCATION, DISINTEGRATION, AND DISSOLUTION
The fate of fibers deposited on surfaces within the lungs depends on both the sites of initial deposition and the physicochemical characteristics of the fibers. Thus, retained fiber burden is a function of both deposition and clearance mechanisms, which include translocation, disintegration, and dissolution. Within the first day, most fibers deposited on the tracheobronchial airways are carried proximally on the surface of the mucus to the larynx to be swallowed and passed into the gastrointestinal tract (GIT). For nonsmokers, the residence time for fibers on the surface of the tracheobronchial region is too short for any significant change in the size or composition of the fibers to take place. For smokers, there may be bronchial airway surfaces that are denuded of cilia where fibers can deposit and accumulate, possibly accounting for the greater cancer incidence in smokers than in nonsmokers due to increased residence times of fibers in the lung. Fibers deposited in the nonciliated airspaces beyond the terminal bronchioles are slowly cleared from their deposition sites by a variety of mechanisms and pathways. These can be classified into two broad categories, that is, translocation and disintegration.
Translocation
Translocation refers to the movement of the intact fiber (1) along the epithelial surface to dust foci at the respiratory bronchioles, (2) onto the ciliated epithelium at the terminal bronchioles, or (3) into and through the epithelium, with subsequent migration to interstitial storage sites within the lung, along lymphatic drainage pathways, and for very thin short fibers, access via capillary blood to distant sites, as suggested by CitationMonchaux et al. (1981).
CitationDodson et al. (1990) compared the fiber content of tissues from chronically exposed shipyard workers, and found that while 10% of amphibole fibers in pleural plaque samples were longer than 5 μm and 8% were longer than 10 μm, the corresponding figures for chrysotile fibers were 3.1 and 0%. In lymph nodes, the corresponding figures for >10 and >5 μm lengths were 6 and 2.5% for amphiboles and 0 and 0% for chrysotile. In lung tissue, they were 41 and 20% for amphiboles and 14 and 4% for chrysotile. CitationBoutin et al. (1996) showed heterogeneous accumulation of asbestos fibers where they occur preferentially in “black spots” of the parietal pleura. CitationBoutin et al. (1996) noted that the black spots were associated with primarily amphibole fibers, 22.5% greater or equal to 5 μm in length, and were in close contact with early pleural plaques. These studies indicate that fiber translocation is dependent on both fiber diameter and fiber length, and that length is one important determinant of biological responses. Translocation may also occur after ingestion of fibers by alveolar macrophages. CitationHolt (1982) proposed that fibers phagocytosed by alveolar macrophages are carried toward the lung periphery by passing through alveolar walls and that some of these cells aggregate in alveoli near larger bronchioles and then penetrate the bronchiolar wall. Once in the bronchiolar lumen, fibers might be cleared by mucociliary transport.
CitationLentz et al. (2003) examined the dimensions of fibers that may translocate to the parietal pleura, and concluded that the critical dimensions were diameter <0.4 μm and length <10 μm. CitationLentz et al. (2003) attributed the pleural plaques that developed in refractory ceramic manufacturing workers to these translocated fibers. It is unclear how inhaled fibers of specific dimensions get to the pleura and whether their dimensional properties govern direct penetration through the lung parenchyma, transport via the lymphatic pathway, or other mechanisms (CitationBroaddus et al., 2011). This differs somewhat from the work by CitationLippmann (1988), which concluded that the risk for human mesothelioma could be indexed by the number of fibers thinner than 0.15 μm and longer than 5 μm. Following a single 3-h exposure (approximately 10 mg/m3 air), CitationCoin et al. (1992) used scanning electron microscopy (SEM) to examine the clearance of chrysotile fibers through 29 d postexposure. Fibers were deposited initially within 1 to 2 mm of the visceral pleura in rats. These included fibers greater than 16 μm in length, which are cleared slowly, if at all. Data showed that the numbers of fibers in these peripheral locations increased over time due to longitudinal splitting. Thus, long fibers and the short thin fibers that result from the constant breakdown of chrysotile (see later discussion) do not have to move far or rapidly to reach the pleural membranes. Subsequently, CitationBernstein et al. (2004) examined, using quantitative three-dimensional (3-D) confocal microscopy, the distribution of Brazilian chrysotile asbestos as a function of time throughout 12 mo following a 5-d exposure. While chrysotile fibers passed through the interstitium quickly after exposure, they were no longer present in the interstitium by 6 mo.
Disintegration
Disintegration refers to a number of processes, including the subdivision of the fibers into shorter segments; partial dissolution of components of the matrix, creating a more porous fiber of relatively unchanged external size; or surface etching of the fibers, creating a change in the external dimensions of the fibers and/or complete dissolution. For synthetic vitreous fibers (SVF), fiber breakup is virtually determined by length. The breakdown into smaller diameter fibrils that is characteristic of asbestos fibers is seldom seen. For SVF, the relative importance of breakage into length segments, partial dissolution, and surface etching to the clearance of fibers depends upon the size and composition of the fiber.
In the inhalation study of CitationBrody et al. (1981) with chrysotile, examination of tissues by transmission electron microscopy (TEM) revealed that fibers deposited on the bifurcations of the alveolar ducts were taken up, at least partially, by type I epithelial cells during the 1-h inhalation exposure. In the 5-h period after exposure, significant amounts were cleared from the surfaces, and there was further uptake by both type I cells and alveolar macrophages. Within 24 h after the exposure, there was an influx of macrophages to the alveolar duct bifurcations (CitationWarheit et al., 1986). The observations provide a basis for fiber penetration of the surface epithelium and an explanation of the mechanisms by which inhaled asbestos fibers activate chemotactic complement proteins on alveolar surfaces. CitationRoggli and Brody (1984) exposed rats for 1 h to a chrysotile aerosol and showed that fiber clearance was associated with sequential dimensional changes in the retained fibers, with a tendency for long, thin fibers to be retained within the interstitium of the lung parenchyma. CitationRoggli et al. (1987) subsequently performed essentially the same study with a crocidolite aerosol. For the crocidolite, there was a progressive rise in mean fiber length with increasing time postexposure, but the change was less pronounced than that for chrysotile. In addition, there was no marked change in fiber diameter over time for crocidolite fibers. In contrast, the longitudinal splitting of the chrysotile into fibrils produced a marked reduction of diameter with time.
Accumulation of fibers in distal lung airways may, by itself, slow the clearance of fibers and other particles from the lung. CitationFerin and Leach (1976) exposed rats by inhalation to 10, 5, or 1 mg/m3 of UICC amosite or Canadian chrysotile for periods ranging from 1 h to 22 d. Exposures at 10 mg/m3 for 1–3 h or for >11 d at 1 mg/m3 suppressed the pulmonary clearance of TiO2 particles. As more than 80% of the UICC fiber aerosol is comprised of very short fibers or particles, the reduction in pulmonary clearance of TiO2 at this exposure concentration might be explained (as described later) by particle overload. CitationBellmann et al. (2001) demonstrated that during chronic exposure to a refractory ceramic aerosol containing both fibers and shot (more compact particles), the clearance of the fibers was retarded by the presence of the shot.
OVERLOAD ASSOCIATED WITH HIGH LUNG BURDEN OF FIBERS
Based upon 6-wk inhalation studies of UICC amosite in rats, CitationBolton et al. (1983) reported evidence for an overload of clearance at high lung burdens (exceeding about 1500 μg/rat), in which a breakdown occurs of the intermediate-rate clearance mechanisms (time constants of the order of 12 d). This hypothesis is consistent with results of other inhalation studies in rats with asbestos (CitationWagner & Skidmore, 1965), quartz (CitationFerin, 1972), and diesel soot (CitationChan et al., 1984). CitationVincent et al. (1985) modified the preceding hypothesis on the basis of additional 1-yr-long rat inhalation studies using amosite asbestos fibers at 1 and 10 mg/m3. Data demonstrated lung burden to scale to exposure concentration in a way that seemed to contradict the overload hypothesis stated earlier. However, the general pattern exhibited by the results for asbestos resembles that for rats inhaling diesel fumes, suggesting that such accumulations are not specific to fibrous dust. CitationVincent et al. (1985) offered a modified hypothesis that although overload of clearance can take place at high lung burdens after exposure has ceased, it is canceled by the sustained stimulus to clearance mechanisms provided by the continuous challenge of chronic exposure. The linearity of the elevation in lung burden is explained in terms of a kinetic model involving sequestration of some inhaled material to parts of the lung where it is difficult to clear. The particular sequestration model favored by CitationVincent et al. (1985) is one in which the longer a particle remains in the lung without being cleared, the more likely it is that it will be sequestrated (and therefore less likely be cleared).
CitationMorrow (1988) developed a general hypothesis that dust overloading, which is typified by a progressive reduction of particle clearance from the deep lung, reflects a breakdown in alveolar macrophage (AM)-mediated dust removal as a result of the loss of AM mobility. The inability of the dust-laden AM to translocate to the mucociliary escalator is correlated to an average composite particle volume per alveolar macrophage in the lung. When the volume of relatively nontoxic particles exceeds approximately 60 μm3/AM, the overload effect appears to be initiated. When the distributed particulate volume exceeds approximately 600 μm3 per cell, the AM-mediated particle clearance virtually ceases, and agglomerated particle-laden macrophages remain in the alveolar region. For cytotoxic particles, these effects occur at lower loadings.
CitationOberdorster et al. (1990) performed additional lung instillation and inhalation studies to further explore the CitationMorrow (1988) hypothesis and the respective roles of both AM and polymorphonuclear leukocytes (PMN), whose influx is indicative of a cellular inflammatory response. On the basis of these studies, the following were concluded:
1. | The delivered dose rate of particles to the lung is a determinant for the acute inflammatory PMN response: The same dose delivered over days by inhalation as opposed to sudden instillation leads to a low response, conceivably reflecting the low release rate of phagocytosis-related inflammatory mediators (e.g., chemotactic factors) from AM. | ||||
2. | The process of phagocytosis of “nuisance” particles by AM rather than the interstitial access of the particles appears to initiate the influx of PMN into the alveolar space. | ||||
3. | The surface area of the retained particles correlates best with inflammatory parameters rather than the phagocytized particle numbers, mass or volume. Specifically, (a) the surface area of the fraction of particles phagocytized by AM correlates best with the influx of PMN; (b) in contrast, an increase in alveolar epithelial permeability, another sign of inflammation, correlates with the retained surface area of the particles in the total lung, rather than with the surface area retained in the alveolar space; and (c) The two inflammatory parameters “alveolar PMN influx” and “alveolar epithelial permeability” are therefore separate events triggered by different mechanisms. | ||||
4. | Interstitialization of particles appears to be important for inducing interstitial inflammatory responses, including the induction of fibrotic reactions. | ||||
5. | If the interstitialized particle fraction exceeds the particle fraction remaining in the alveolar space, the influx of PMN into the alveolar lumen decreases, conceivably reflecting a reversal of chemotactic gradients from alveolar space towards the interstitial space. |
CitationJones et al. (1988) extended the inhalation studies to lower concentrations; rats inhaled UICC amosite asbestos at approximately 0.1 mg/m3 (equivalent to 20 fibers/ml) for 7 h/d, 5 d/wk, for up to 18 mo. The lung burdens were compared with the previous results for concentrations of 1 and 10 mg/m3. Taken together, these results showed lung burdens rising pro rata with exposure concentration and exposure time. This accumulation of lung burden fit a kinetic model that takes account of the sequestration of material at locations in the lung from which it cannot be cleared. CitationTran et al. (1997) found that the overloading of the lung by fibers less than 15 μm long and more compact particles follow the same kinetics, and are similarly affected by overloading. For long fibers (>25 μm), the disappearance was independent of length and lung burden, implying that the clearance of such fibers occurs by dissolution and fragmentation into shorter lengths.
Few inhalation experiments exist at nonoverloading concentrations using particle and fiber mixtures. A recent study by CitationBernstein et al. (2008) compared the clearance of chrysotile alone to chrysolite mixed with nonfibrous joint compound particles in a 5-d biopersistence study. The aerosol exposure was in the range of 5000 WHO fibers/cm3 and 12,000 to 18,000 total fibers/cm3. The powder exposure concentration was 1.3 mg/m3. Across all size ranges there was approximately an order of magnitude decrease in the mean number of each size category of fibers remaining in the lungs in the chrysotile- and particle-exposed group as compared to the group exposed to chrysotile alone, even though the fiber aerosol exposures were similar. This study demonstrated that at nonoverloading exposures, the addition of nonfibrous particles to an aerosol containing chrysotile asbestos fibers results in a greater rate of clearance of fibers from the lung, which is likely due to an increase in the recruitment of macrophages in the lung, in which the acid degrades the fibers. The increased number of macrophages was confirmed histologically, with this being the only exposure-related finding reported.
ROLE OF FIBER RETENTION IN BIOLOGICAL RESPONSES OF VARIOUS ASBESTOS TYPES
Differential lung clearance between fibers of chrysotile and the more rod-like amphibole asbestos fibers was found in rats that underwent chronic inhalation exposures (CitationWagner et al., 1974). The lung fiber burdens of the amphiboles rose continuously throughout 2 yr of exposure, and declined slowly in the rats removed from exposure after 6 mo. In contrast, the lung burdens in rats exposed to both Quebec and Zimbabwe chrysotile increased much more slowly during exposure, and seemed to decline after 12 mo, even with further exposure.
The biopersistence of chrysotile fibers from other locations was studied by CitationBernstein and colleagues (2004) following inhalation exposures to aerosols with large number concentrations of fibers >20 μm in length. For chrysotile from the Cana Brava mine in central Brazil, the clearance half-time of the fibers >20, those 5–20, and those <5 μm in length were 1.3, 2.4, and 23 d, respectively (CitationBernstein et al., 2004). For chrysotile from the Coalinga mine in California (Calidria RG144), the clearance half-time of the fibers >20, those 5–20, and those <5 μm in length were 7 h, 7 d, and 64 d, respectively, while for tremolite asbestos there was no clearance from the rat lungs over the 1-yr period of observation (CitationBernstein et al., 2005a). The tremolite exposures produced lung inflammation, granulomas, and lung fibrosis, while the chrysotile, despite involving a much higher long fiber concentration (190.5 fibers/cm3 >20 μm for chrysotile vs. 106.2 fibers/cm3 >20 μm for tremolite), did not produce any measurable response. For textile-grade chrysotile from the Eastern Townships of Quebec, the clearance half-time for fibers >20 μm in length was 11.4 d, which was similar to that for glass and stone wools previously studied (CitationBernstein et al, 2005b), but a longer half-life than reported earlier in this article for California or Brazilian chrysotile. Data suggest differences in clearance for various types of chrysotile.
Similar differential retention of chrysotile versus amphibole types of asbestos was found in humans. CitationChurg (1994) reported on analyses of lung tissue for 94 chrysotile asbestos miners and millers from the Thetford region of Quebec, Canada. The retained chrysotile and exposure atmosphere contained a small percentage of tremolite, yet the lungs contained more tremolite than chrysotile, and the tremolite content increased rapidly with the duration of exposure. While most of the inhaled chrysotile was rapidly cleared from the lungs, a small fraction seemed to be retained indefinitely. After exposure ended, there was little or no clearance of either chrysotile or tremolite from the lungs, an unexpected finding. CitationAlbin et al. (1994) studied retention patterns in lung tissues from 69 Swedish asbestos-cement workers and 96 controls. Data showed that chrysotile underwent relatively rapid removal in human lungs, whereas amphiboles (tremolite and crocidolite) displayed a slower removal pattern. CitationAlbin et al. (1994) also noted that (1) chrysotile retention may be dependent on dose rate, (2) chrysotile and crocidolite retention may be increased by smoking, and (3) chrysotile and tremolite retention may be enhanced by the presence of lung fibrosis.
The most direct evidence for the effect of altered dust clearance rates on the retention of inhaled fibers in humans comes from studies of the fiber content of the lungs of asbestos workers in various countries. CitationTimbrell (1982) developed a model for fiber deposition and clearance in human lungs based on his analysis of the bivariate diameter and length distributions found in air and lung samples collected at an anthophyllite mine at Paakkila in Finland. The length and diameter distributions of the airborne dust at this particular mine were exceptionally broad and historic exposures were high. For workers with the highest exposure and most severe lung fibrosis (CitationAshcroft et al.,1988), the fiber distributions in some tissue segments approached those of the airborne fibers. Adjacent tissue, analyzed for extent of fibrosis, showed severe fibrotic lesions. CitationAshcroft et al. (1988) concluded that chronic retention was essentially equal to deposition in such segments. In these studies, lung fibrosis was associated with increased fiber retention, and fiber retention is clearly associated with fiber length and diameter. The critical fiber length for mechanical clearance from the lungs is greater than 17 μm. as confirmed in the inhalation model published by CitationCoin et al. (1992). More precise descriptions of the effect of fiber loading in the lung on the development of fibrosis need to be based on the use of the most appropriate index of fiber loading.
CitationPundsack (1955) explained that “From a chemical point of view chrysotile behaves in certain aspects as if it were magnesium hydroxide. This is not unexpected when one considers that the structure generally ascribed to the mineral consists of fundamental layers made up in terms of a unit cell of O6-Si4-O4(OH)2-Mg6-OH6 planes” (p. 894). CitationPundsack (1955) found that the behavior of chrysotile fibers can be understood as a magnesium hydroxide layer on a silica substrate and explained that initially at neutral pH, such as that found in lung surfactant, “in contact with relatively pure water the fiber surface dissociates partially until equilibrium of the order of that attained by pure magnesium hydroxide is reached.” In an acid environment (such as might occur in the macrophage), “It is important to note that chrysotile reacts with strong acids to form eventually a hydrated silica residue. Therefore, the particles suspended in initially acid solutions are not chrysotile in the strict sense, but represent instead intermediate reaction products of the acid and the fiber” (p. 895).
CitationBernstein et al. (1984) and CitationHammad (1984) found evidence of substantial in vivo dissolution of glass fibers. CitationLe Bouffant et al. (1984) used x-ray analysis on individual fibers recovered from lung tissue to show the exchange of cations between the fibers and tissues. For example, the fibers may lose calcium and gain potassium.
Insight on the solubility of fibers in vivo was also obtained from in vitro solubility tests. CitationGriffis et al. (1981) found that glass fibers suspended either in buffered saline or serum-like solution at 37°C for 60 d exhibited some solubility and that the sodium content of the residual fiber was reduced. CitationFörster (1984) used Gamble's saline solution for tests on samples of 18 different SVF at temperatures of 20 and 37°C and for exposure times ranging from 1 h to 180 d using static tests, tests with once-daily shaking, tests with continuous shaking, and tests with single fibers in an open bath. There was some solubility for all fibers. CitationKlingholz and Steinkopf (1982, Citation1984) studied dissolution of mineral wool, glass wool, rock wool, and basalt wool at 37°C in water and in a Gamble's solution modified by omission of the organic constituents. Most of the tests used a continuous-flow system in which the pH was 7.5–8. There was relatively little dissolution in distilled water in comparison to that produced by the modified Gamble's solution. The surfaces developed a gel layer that, for the smaller diameters, extended throughout the fiber cross section. Thus, fibers may become both smaller in outline and more plastic to deformation.
CitationScholze and Conradt (1987) performed a comparative in vitro study of the chemical durability of SVF in a simulated extracellular fluid under flow conditions. Seven vitreous, three refractory, and three natural fibers were involved. Samples of the leachate were analyzed, and the silicon concentrations were used to roughly classify the fibers according to their chemical durability in terms of glass network dissolution. A durability ranking of fiber materials was expressed in terms of a characteristic time required for the complete dissolution of single fibers of given diameter. SVF exhibited relatively poor durability (with network dissolution velocities ranging from 3.5 to 0.2 nm/d for a glass wool and an E-glass fiber, respectively), whereas natural mineral fibers were persistent against the attack of the biological fluid (e.g., less than 0.01 nm/d for crocidolite).
CitationDavies et al. (1984) exposed rats to SVF aerosols at 10 mg/m3 for 7 h/d, 5 d/wk for 1 yr as compared to the single exposure of several hours duration used by CitationMorgan and Holmes (1984). The percentage of glass fibers with diameters less than 0.3 μm recovered from the lungs was consistently less than that in the original fiber suspension, and the reduction was more marked in the animals that were sacrificed following a period of recovery from the exposures than from those sacrificed at the end of the exposure. The degree of fiber etching increased with residence times in the lungs. Glass wool with and without resin was also etched, but to a lesser extent, and the etching of the rock wool fibers was considerably less.
In a study of dissolution of inhaled fibers by CitationEastes and Hadley (1995), rats were exposed for 5 d to 4 types of airborne, respirable-sized SVF and to crocidolite fibers. The SVF included 2 glass wools, and 1 each of rock and slag wool. After exposure, animals were sacrificed at intervals up to 18 mo, and the numbers, lengths, and diameters of a representative sample of fibers in their lungs were measured. Long fibers (>20 μm) were eliminated from rat lungs at a rate predicted from the dissolution rate measured in vitro. The long SVF were nearly completely eliminated in several months, whereas most long crocidolite asbestos fibers remained at the end of the study. The number, length, and diameter distributions of fibers remaining in the rat lungs agreed well with a computer simulation of fiber clearance that assumed that the long fibers dissolved at the rate measured for each fiber in vitro, and that the short fibers of every type were removed at the same rate as short crocidolite asbestos. Thus, long SVF were cleared by complete dissolution at the rate measured in vitro, and short fibers did not dissolve and were cleared by macrophage-mediated physical removal.
In an inhalation study using 9 fiber types, CitationBernstein et al. (1996) exposed rats to an aerosol (mean diameter of ∼1 μm) at a concentration of 30 mg/m3, 6 h/day for 5 d with post-exposure sacrifices at 1 h, 1 day, 5 d, 4 wk, 13 wk, and 26 wk. At 1 h following the last exposure, the 9 types of fibers were found to have lung burdens ranging from 7.4 to 33 × 106 fibers/lung with geometric mean diameters (GMD) of 0.40–0.54 μm, reflecting the different bivariate distributions in the exposure aerosols. The fibers cleared from the lungs following exposure with weighted half-lives ranging from 11 to 54 d. The clearance was found to closely reflect the clearance of fibers in the 5–20 μm length range. An important difference in removal was seen between the long fiber (L > 20 μm) and shorter fiber (L between 5 and 20 μm and L < 5 μm) fractions, depending upon composition. For all glass wools and the stone wools, the longer fibers were removed faster than the shorter fibers. It was found that the time for complete fiber dissolution based on the acellular in vitro dissolution rate at pH 7.4 was significantly correlated with the clearance half-times of fibers >20 μm in length. No such correlations were noted with any of the length fractions using the acellular in vitro dissolution rate at pH 4.5. Examination of the fiber length distribution and particles in the lung from 1 h through 5 d of exposure indicated that, especially for those fibers that form leached layers, fiber breakage may have occurred during this early period. These results demonstrate that for fibers with high acellular solubility at pH 7.4, the clearance of long fibers is rapid.
CitationEastes and Hadley (1996) fitted much of the data just cited into a mathematical model of fiber carcinogenicity and fibrosis. Their model predicts the incidence of tumors and fibrosis in rats exposed to various types of rapidly dissolving fibers in an inhalation study or in an intraperitoneal (ip) injection experiment. This takes into account the fiber diameter and the dissolution rate of fibers longer than 20 μm in the lung, and predicts the measured tumor and fibrosis incidence to within approximately the precision of the measurements. The underlying concept for the model is that a rapidly dissolving long fiber has the same response in an animal bioassay as a smaller dose of a durable fiber. Long, durable fibers have special significance, since there is no effective mechanism by which these fibers may be removed. In particular, the postulation is that the effective dose of a dissolving long fiber scales as the residence time of that fiber in the extracellular fluid. The residence time of a fiber is estimated directly from the average fiber diameter, density, and the fiber dissolution rate as measured in simulated lung fluid at neutral pH.
The incidence of fibrosis in chronic inhalation tests, the observed lung tumor rates, and the incidence of mesothelioma in the ip model were all well predicted by this model. The model allows one to predict, for an inhalation or ip experiment, what residence time and dissolution rate are required for an acceptably small tumorigenic or fibrotic response to a given fiber dose. For an inhalation test in rats at the maximum tolerated dose (MTD), the model suggests that less than 10% incidence of fibrosis would be obtained at the maximum tolerated dose of 1-μm diameter fibers if the dissolution rate were greater than 80 ng/cm2/h. The dissolution rate that would give no detectable lung tumors in such an inhalation test in rats is much smaller. Thus, a fiber with a dissolution rate of 100 ng/cm2/h has a nonsignificant chance of producing either fibrosis or tumors by inhalation in rats, even at the MTD. This model provides manufacturers of SVF and other synthesized fibers with design criteria for fibrous products that minimize, if not eliminate, the potential for producing adverse health effects. Support for the use of biopersistence data for the prediction of fibrosis and tumor responses in rats from both ip injection studies and chronic inhalation studies for fibers >5 and >20 μm in length was also provided by CitationBernstein et al. (2001a, Citation2001b). For the inhalation studies, CitationBernstein et al. (2001a, Citation2001b) used collagen deposition at the bronchoalveolar junction as a predictor of interstitial fibrosis. Fibrosis was also associated with the development of lung cancers in rats (CitationDavis & Cowie, 1990). The consensus from these and other studies already mentioned suggests that some SVF, including ceramic fibers, are more durable than others and are less persistent than crocidolite asbestos in the lung.
As discussed earlier, the retention, dose, dimensions, durability, and composition of amphibole asbestos fibers, in contrast to disintegration and/or dissolution of SVF, are critical parameters related to adverse health effects occurrence, with chrysotile asbestos fibers falling between these two extremes. The initial database of well-conducted inhalation toxicology studies from which these concepts are based included primarily studies of SVF, many of which included asbestos-exposed groups as positive controls. More recently, studies on different types of asbestos fibers have extended these concepts, differentiating serpentine (chrysotile) asbestos from amphibole asbestos. The existing database of fiber toxicity studies indicates that human exposure to respirable fibers that are biopersistent in the lung also induces significant and persistent pulmonary inflammation, cell proliferation, or fibrosis, and therefore needs to be viewed with concern.
FACTORS THAT INDUCE FIBER TOXICITY
Mineral fiber toxicology has been associated with three key factors: dose, dimension, and durability. Particle surface activity is also likely to play some role, but at this point in time, its role remains to be established. The dose of fibers is determined by physical characteristics/dimensions and exposure levels, which are also affected by how the fibrous material is used and the control procedures that are implemented. Most asbestos fibers are thinner than SVF in commercial insulation and other products.
Fiber dimensions are important because only very thin fibers are respirable and penetrate into the deep alveolar region of the lung, and because fiber length may impact their toxicity. As emphasized earlier, short fibers of the sizes that can be fully engulfed by macrophages will be cleared by mechanisms similar to those for nonfibrous particles. These mechanisms include mucociliary clearance and macrophage phagocytosis, as well as clearance through the lymphatics. It is generally concluded that only the long fibers that the macrophage cannot fully engulf and that are biopersistent lead to disease (CitationHEI, 1991; CitationIOM, 2008). However, a role for short fibers in disease cannot be completely ruled out.
This leads to the third factor involved in fiber toxicity, which is persistence, a function of fiber durability and pathogenicity. Those fibers whose chemical composition renders them wholly or partially soluble once deposited in the lung are likely to either dissolve completely or dissolve until they are sufficiently weakened focally to undergo breakage into shorter fibers. The remaining short fibers can then be removed from the deep lung though macrophage phagocytosis and mucociliary clearance.
SVF, such as glass wool, slag wool, and rock wool, are amorphous structures. In the lung, these fibers were found to dissolve by two principal mechanisms, either through congruent or incongruent dissolution. Congruent dissolution of SVF leads to products that are completely soluble in the aqueous phase. In contrast, incongruent dissolution leads to one or more insoluble products.
The importance of fiber length in asbestos toxicity and disease was first addressed in studies by CitationVorwald et al. (1951) in experimental models of asbestosis. Subsequently, dose, dimension, and durability were shown to be important determinants of toxicity for SVF or asbestos (CitationBernstein et al. 2001a; Citation2001b; CitationHesterberg et al. 1998a;Citation1998b; CitationMiller et al. 1999; CitationOberdorster 2000; CitationStanton et al. 1981; reviewed in CitationCase et al. 2011). The importance of durability in differentiating asbestos fiber toxicity between the serpentine mineral fiber, chrysotile, and the amphibole mineral fibers, such as amosite and crocidolite, was addressed more recently (CitationBernstein & Hoskins, 2006). Because fibers tend to align with the airflow into in the airways when inhaled into the lung, the fiber diameter is the primary determinant of deposition in the lung. A fiber is unique among inhaled particles in that the fibers aerodynamic diameter is largely related to threefold greater than fiber diameter. Because of this, long thin fibers penetrate into the deep lung. Within the lung, fibers that are fully engulfed by the macrophage are removed as with any other particle. However, those fibers that are too long to be fully engulfed by the macrophage cannot be cleared by this route. CitationZeidler-Erdely et al. (2006) showed that 20-μm fibers are fully engulfed by human alveolar macrophages. Alveolar macrophages are smaller in the rat, and thus the critical length is proportionately shorter. Longer fibers remain in the lung and are cleared from the lung only if they dissolve or break apart.
Extensive work on modeling the dissolution of mineral fibers using in vitro dissolution techniques and inhalation biopersistence demonstrated that lung has a large buffer capacity (CitationMattson, 1994) These studies showed that an equivalent in vitro flow rate of up to 1 ml/min is required to provide the same dissolution rate of SVF that occurs in the lung. This large fluid flow within the lung results in the disassociation of the soluble components in the fibers. The association of longer fibers (20–50 μm) with lung disease as opposed to shorter fibers (3 μm or less) was reported by CitationVorwald et al. (1951), who concluded that “the mode of action of the long asbestos fiber in the production of asbestosis is primarily mechanical rather than chemical in nature” and that “long asbestos fibers are essential in the production of the fibrosis; short fibers are incapable of producing this reaction” (CitationVorwald et al., 1951). The relationship between chemical composition and dissolution and subsequent breakage was first reported by CitationHammad (1984). SVF <5 μm in length had the longest retention in the lung following short-term inhalation, with longer fibers clearing more rapidly and fibers >30 μm in length clearing very rapidly. CitationHammad (1984) proposed that clearance of mineral wools is a result of biological clearance and the elimination of fibers by dissolution and subsequent breakage. Data also suggested that the long fibers were leaching and breaking into shorter fibers, which explained the rapid disappearance of these fibers from the lung. The shorter fibers appeared to have a longer retention time because fibers were added to the pool by breakage of longer fibers.
CAVEATS OF CHRONIC INHALATION STUDIES USING FIBERS
Early chronic inhalation studies of fibers in the rat were often performed without consideration of the respirability of the fibers and without determining the length distribution of the fibers. In addition, studies were often performed at high total particle/fiber exposure concentrations. As mineral fibers often occur in bundles of long strands, investigators would grind the fibers to produce a more respirable fraction instead of separating the fibers from the bundles. This process frequently pulverized the long respirable fiber fraction, producing excessive particles and shorter fibers sufficient to produce lung overload in rats. High concentrations of insoluble dusts when administered by inhalation in the rat were found to overload the lung by compromising the clearance mechanisms, which resulted in inflammation and a tumorigenic response (CitationBolton et al., 1983; CitationMorrow, 1988; CitationMuhle et al., 1988; CitationOberdorster, 1995a, Citation1995b). Historically, inhalation toxicology studies were performed far above the fiber concentration levels to which humans might be exposed. When the exposure level is elevated 100,000-fold higher than human exposures, as occurred in most of the earlier fiber inhalation studies with chrysotile and amphibole asbestos, lung overload occurs.
While many chronic inhalation toxicology studies of different types of fibers were performed, their design and subsequent interpretation are often confounded by the fiber size distribution and the ratio of longer fibers to shorter fibers, as well as inclusion of nonfibrous particles. In many of these investigations the exposures often approach or exceed that which has been shown to produce “lung overload” in the rat. Thus, it may become difficult to compare the effects observed in one study with those of another. In most chronic inhalation studies on asbestos, the fiber exposure concentration was determined based upon a gravimetric concentration of 10 mg/m3 without regard for fiber number or size. High concentrations of insoluble dusts, when administered by inhalation in the rat, were found to (1) overload the lung by compromising the clearance mechanisms; (2) result in inflammation; and (3) result in a nonspecific tumorigenic response (CitationBolton et al., 1983; CitationMorrow, 1988; CitationMuhle et al., 1988; CitationOberdorster, 1995a, Citation1995b).
The issue of using equivalent fiber numbers for exposure was approached in a study reported by CitationDavis et al. (1978) where chrysotile, crocidolite, and amosite were compared on an equal mass and equal number basis. However, the fiber number was determined by phase-contrast optical microscopy (PCOM) and thus the actual fiber number, especially of the chrysotile fibers, was probably greatly underestimated. As an example, by PCOM, the 10-mg/m3 exposure to chrysotile by PCOM was approximately 2000 f/cm3 with length greater than 5 μm, while a similar mass concentration of another chrysotile sample measured by SEM was 10,000 f/cm3 with length greater than 5 μm. In contrast, CitationMast et al. (1995) reported for 10 mg/m3 a total fiber count of 100,000 f/cm3 as measured by SEM. Another problem in interpretation of results is the little quantitative data presented in these publications on nonfibrous particle concentrations in the exposures. CitationPinkerton et al. (1983) presented summary tables of length measurements of Calidria chrysotile by SEM in which the number of nonfibrous particles counted is stated. The aerosol exposure concentration of nonfibrous particles cannot be extracted from the data presented. In all studies, the asbestos was ground prior to aerosolization, a procedure that produces a lot of short fibers and nonfibrous dust. Most of the studies prior to CitationMast et al. (1995) used an apparatus (Wright Dust Feed) for aerosolizing fibers that employed a rotating steel blade to push/chop the fibers off a compressed plug and into the airstream. As some of the authors stated, the grinding of the asbestos and the flow through the apparatus often abraded the steel surfaces, resulting in considerable exposure to the metal fragments as well. These factors contribute significantly to the difficulty in interpreting the results of these earlier chrysotile and amphibole inhalation exposure studies. These discrepancies in study design also bring into question the difficulties in comparative analysis of various types of fibers. As an example, in the chronic inhalation study of refractory ceramic fibers (RCF1) reported by CitationMast et al. (1994), the exposure aerosol had a total average of 234 fibers/cm3 of which there were approximately 100 fibers/cm3 with L > 20 μm. This resulted in a total fiber number in the lung after 24 mo of exposure of approximately 1 million fibers. In the chrysotile study reported by CitationHesterberg et al. (1999), the exposure aerosol had a total average of 102,000 fibers/cm3 (the number of fibers/cm3 longer than 20 μm was not reported). This resulted in a total fiber number in the lung after 24 mo of exposure of approximately 55 million fibers.
In 1988, a series of chronic inhalation studies on SVF was performed that took into account the respirability of fibers in rats and the importance of fiber length in both the preparation of the fibers and the exposure techniques (CitationHesterberg et al. 1992, Citation1995; CitationMast et al., 1995; CitationMcConnell et al., 1995). The results of these studies indicated that the more soluble fibers that were tested showed little or no pathogenic response, while less soluble fibers demonstrated more response. To further investigate this, a 5-d inhalation protocol was developed for the evaluation of the biopersistence of SVF (CitationBernstein et al., 1994; CitationMusselman et al., 1994), with numerous fibers analyzed using this protocol (CitationBernstein et al., 1996; CitationHesterberg et al., 1998a). This 5-d inhalation exposure was proposed by the U.S. Environmental Protection Agency for evaluating the pathological response and biopersistence of inhaled fibers. The biopersistence protocol was also incorporated by the European Commission (CitationBernstein & Riego-Sintes, 1999) as part of the European Commission's synthetic fiber directive (CitationEUR, 1997). Subsequently, CitationMcConnell et al. (1999) reported on a multiple-dose study on amosite asbestos in which particle and fiber number and length were comparable to the SVF exposure groups. In this hamster inhalation study, the amosite aerosol concentrations ranged from 10 to 69 f/cm3 for fibers longer than 20 μm and were chosen based upon a previous, multidose 90-d subchronic inhalation study (CitationHesterberg et al., 1999). In the chronic study, only high-dose amosite asbestos exposure resulted in mesotheliomas (19.5%). In a short-term exposure study in the rat (6 h/d, 5 d) using tremolite asbestos at a long fiber exposure concentration of 100 f/cm2 for fibers longer than 20 μm, interstitial fibrosis developed within 28 d after cessation of the 5-d exposure (CitationBernstein et al., 2005a). No chronic inhalation studies using chrysotile asbestos with fiber selection techniques to prevent lung overload have been performed.
SUBCHRONIC INHALATION STUDIES USING FIBERS
The European Commission (CitationBernstein & Riego-Sintes, 1999) as part of the European Commission's synthetic fiber directive (CitationEUR, 1997), also stated that current short-term testing methods, defined as 3 mo or less in exposure duration, evaluate a number of endpoints that are considered relevant for lung diseases induced by fibers, such as asbestos. Subchronic studies that assessed biomarkers of lung injury including persistent inflammation, cell proliferation, and fibrosis, were considered to be more predictive of carcinogenic potential than in vitro measures of cellular toxicity. Of particular importance in the evaluation of fiber toxicity using the 90-d subchronic inhalation toxicity study is the finding that “All fibers that have caused cancer in animals via inhalation have also caused fibrosis, and at an earlier time point, that is, by 3 months. However, there have been fiber exposures that have caused fibrosis but not cancer. Therefore, in vivo studies that involve short-term exposure of rat lungs to fibers and subsequent assessment of relevant endpoints, notably fibrosis, are probably adequately conservative for predicting long-term pathology - that is, will identify fibers that have a fibrogenic or carcinogenic potential” (p. 518). The working group also recommended that specific parameters need to be measured in 90-d fiber inhalation studies (CitationEUR, 1997), parameters that were noted in the U.S. EPA Guideline for Combined Chronic Toxicity/Carcinogenicity Testing of Respirable Fibrous Particles (CitationU.S. EPA, 2001). These parameters need to include lung weight, fiber lung burden and clearance, cell proliferation, inflammatory response markers, and histopathology. The European Commission guideline for subchronic inhalation toxicity testing of synthetic vitreous fibers in rats (CitationBernstein & Riego-Sintes, 1999) and the ILSI Panel (CitationILSI, 2000) specify similar parameters.
CitationBellmann et al. (2003) reported on a calibration study to evaluate the endpoints in a 90-d subchronic inhalation toxicity study following this protocol of man-made vitreous fibers with a range of biopersistence and of amosite asbestos. One of the fibers was a calcium–magnesium–silicate (CMS) fiber for which the stock preparation had a large concentration of nonfibrous particulate material in addition to the fibers. After chronic inhalation of the fiber X607, a CMS fiber that had considerably fewer particles present, no lung tumors or fibrosis were detected (CitationHesterberg et al., 1998b). In the CitationBellmann et al. (2003) 90-d study, due to the method of preparation the mean aerosol exposure concentration for the CMS fiber was 286 fibers/cm3 with length <5 μm, 990 fibers/cm3 with length >5 μm, and 1793 particles/cm3, a length distribution that is not observed in manufacturing environments. The total mean CMS exposure concentration was 3,069 particles and fibers/cm3. Evidence indicated that “the particle fraction of CMS that had the same chemical composition as the fibrous fraction seemed to cause significant effects” (p. 1172). For the CMS fiber, results showed that the number of polymorphonuclear leukocytes (PMN) in the bronchoalveolar lavage fluid (BALF) was higher and interstitial fibrosis was more pronounced than had been expected on the basis of biopersistence data. In addition, the interstitial fibrosis persisted through 14 wk after cessation of the 90-d exposure. This effect attributed to particles was observed with an exposure concentration of 3,069 particles and fibers/cm3, of which 50% were nonfibrous particles or short fibers. A markedly more pronounced effect would be expected to occur at higher exposure concentrations.
CitationBernstein et al. (2006) reported on the toxicological response of the commercial Brazilian chrysotile following exposure in a multidose subchronic 90-d inhalation toxicity study that was performed according to the protocols mentioned above as specified by the CitationU.S. EPA (2001) and CitationBernstein and Riego-Sintes (1999). In this study, Wistar male rats were exposed to 2 chrysotile fiber levels at mean fiber aerosol concentrations of 76 fibers with L > 20 μm/cm3 (3413 total fiber/cm3; 536 WHO fiber/cm3) or 207 fibers with L > 20 μm/cm3 (8941 total fiber/cm3; 1429 WHO fiber/cm3). Animals were exposed using a flow-past, nose-only exposure system for 5 d/wk, 6 h/d, during 13 consecutive weeks, followed by a subsequent nonexposure period lasting for 92 d. Animals were sacrificed after cessation of exposure, and after 50 and 92 d of nonexposure recovery. At each sacrifice, subgroups of rats were assessed for the determination of the lung burden; histopathological examination; cell proliferation response; contents of bronchoalveolar lavage, with the determination of inflammatory cells; clinical biochemistry; and for analysis by confocal microscopy. Exposure to chrysotile for 90 d and 92 d of recovery, at a mean exposure of 76 fibers/cm3 with L > 20 μm (3413 total fiber/cm3), resulted in no apparent fibrosis (Wagner score 1.8 to 2.6) at any time point. The long chrysotile fibers were observed to break apart into small particles and smaller fibers. At a mean exposure concentration of 207 fibers/cm3 with L > 20 μm (8941 total mean fibers/cm3) slight fibrosis was observed. In comparison with other studies, chrysotile produced less inflammatory response than the biosoluble synthetic vitreous fiber CMS referred to earlier, and considerably less than amosite asbestos (CitationBellmann et al., 2003). After exposure to a commercial chrysotile product in a subchronic 90-d inhalation toxicology (total mean fiber concentrations as measured by TEM were 3413 fibers/cm3 air and 8941 fibers/cm3 air), no adverse lung pathology was observed at the lower exposure level, and only a slight fibrotic response was seen at the higher exposure level in rodents (CitationBernstein et al., 2006). In contrast, tremolite (an amphibole asbestos) exposure for 5 d (6 h/d) at a mean aerosol concentration (per cubic centimeter) of 100 fibers with L > 20 μm (2016 total fiber/cm3) resulted in extensive inflammatory response with interstitial fibrosis observed within 28 d after cessation of exposure (CitationBernstein et al., 2005a).
In a recent study by CitationBernstein et al (2010), the translocation and pathological response of a commercial chrysotile product similar to that which was used through the mid 1970s in a joint compound intended for sealing the interface between adjacent wall boards was evaluated in comparison to amosite asbestos. This study was unique in that it presented a combined real-world exposure and was the first study to investigate whether there were differences between chrysotile and amosite asbestos fibers in time course, size distribution, and pathological response in the pleural cavity. Rats were exposed by inhalation 6 h/d for 5 d to either sanded joint compound consisting of both chrysotile fibers and sanded joint compound particles (CSP) or amosite asbestos. The mean number of fibers longer than 20 μm was 295 fibers/cm3 for chrysotile and 201 fibers/cm3 for amosite. The mean number of WHO fibers (defined as fibers >5 μm long, <3 μm wide, and with length:width ratios > 3:1) in the CSP atmosphere was 1496 fibers/cm3, which was more than 10,000 times the OSHA occupational exposure limit of 0.1 fibers/cm3. The amosite exposure atmosphere had fewer shorter fibers, resulting in a mean of 584 WHO fibers/cm3. The use of both confocal microscopy and SEM enabled the identification of fibers as well as possible inflammatory responses in the lung, visceral pleura, and parietal pleura. Subgroups were examined over time through 1 yr postexposure. No pathological responses were observed at any time point in the CSP exposure group. The long chrysotile fibers (L > 20 μm) cleared rapidly (T1/2 of 4.5 d) and were not observed in the pleural cavity. In contrast, a rapid inflammatory response occurred in the lung following exposure to amosite, resulting in Wagner grade 4 interstitial fibrosis within 28 d. Long amosite fibers had a T1/2 > 1000 d in the lung and were observed in the pleural cavity within 7 d postexposure. By 90 d the long amosite fibers were associated with a marked inflammatory response on the parietal pleura. This study shows that relevant inhalation exposure to chrysotile fibers and joint compound particles, in contrast to amosite asbestos, does not initiate an inflammatory response in the lung. Moreover, the chrysotile fibers did not migrate to, or induce an inflammatory response in, the pleural cavity.
Molecular and proliferative changes important in carcinogenicity were examined in epithelial and mesothelial cells at high (8.2 mg/m3) and lower (0.17 mg/m3) average gravimetric concentrations of NIEHS reference samples of chrysotile or crocidolite asbestos. Rat lungs were evaluated at 5 and 20 d after initial exposures to asbestos and at 20 d post cessation of a 20-d exposure (CitationBeruBe et al., 1996; CitationQuinlan et al., 1994, Citation1995) (). In both mesothelial and epithelial cells, increases in cell proliferation, as measured by incorporation of 5-bromodeoxyuridine (5’BrdU) using histochemistry, were observed at only the high dose in response to both asbestos types at 5 d. These rises persisted with exposures to crocidolite for 20 d in mesothelial cells. Fibrotic lesions and elevations in hydroxyproline, a biochemical marker of collagen synthesis, were seen only at high crocidolite exposures for 20 d, but appeared in both asbestos groups after an additional 20 d without fiber exposure. Significant elevation in mRNA levels of c-jun protooncogene and ornithine decarboxylase (odc), an enzyme necessary for cell proliferation and linked to tumor promotion, were observed exclusively at high concentrations of crocidolite asbestos in lung homogenates. Data suggested that a combination of molecular, histochemical, and biochemical markers of disease may be promising in defining dose-related asbestos effects in subchronic inhalation studies.
TABLE 2. Molecular and Phenotypic Markers Measured in Subchronic Rat Inhalation Studies Using Asbestos
CORRELATIONS BETWEEN FIBER LENGTH, BIOPERSISTENCE, AND CHRONIC TOXICITY
For all fiber exposures, there are many more fibers shorter than 20 μm in length, and even more that are less than 5 μm in length. Because of the mechanical processes in breaking the fibers, fiber length usually follows a lognormal distribution. The clearance of the shorter fibers in biopersistence studies was shown to be either similar to or faster than the clearance of insoluble nuisance dusts (CitationMuhle et al., 1987; CitationStöber et al., 1970). A report issued by the Agency for Toxic Substances and Disease Registry (ATSDR) was entitled “Expert Panel on Health Effects of Asbestos and Synthetic Vitreous Fibers: The Influence of Fiber Length.” In this report, the experts stated, “Given findings from epidemiologic studies, laboratory and animal studies, and in vitro genotoxicity studies, combined with the lung's ability to clear short fibers, that there is a strong weight of evidence that asbestos and SVFs shorter than 5 μm are unlikely to cause cancer in humans” (CitationATSDR, 2003; CitationU.S. EPA, 2003). However, this panel did not evaluate the role of short fibers in noncarcinogenic health effects in humans.
In the series of SVF chronic inhalation studies performed at the Research and Consulting Company Ltd., in the 1990s, the relationship between exposure to more durable fibers and lung disease also became apparent and resulted in the design of the inhalation biopersistence study as already described. The importance of fiber length to the potential of a fiber to produce a pathogenic effect is well documented in a number of studies (CitationGoodglick & Kane, 1990; CitationLippmann, 1990; CitationMcClellan et al., 1992; CitationWHO, 1988).
To evaluate the influence of fiber length on clearance, CitationBernstein et al. (1996) examined the biopersistence of 9 different fibers types using this inhalation biopersistence protocol determining the clearance of fibers in 3 length categories: fibers with length <5 μm, 5–20 μm, and >20 μm. The length categories were selected based upon lung physiological response. Fibers <5 μm in length can be completely phagocytized by macrophages and treated by the lung essentially as nonfibrous particles. The length fraction >20 μm was considered as greater than the size of the macrophage, and represented a fiber length that the macrophage most likely could not fully phagocytize. It was pointed out that these length fractions were not considered as strict cutoffs, but rather representative of mechanistic categories with the transition between categories thought to occur over a range of lengths. The fiber clearance half-times in each of these length fractions were then examined for association with the fiber durability, which was determined using an acellular in vitro flow-though system using a modified Gamble's solution. This analysis provides a reliable indication that the disappearance of fibers longer than 20 μm may be mediated by their dissolution at pH 7.4. While not reported in the publication, Bernstein (personal communication) chose the 20-μm cutoff for the longer fibers by performing iterative analyses starting at a fiber length of 10 μm and greater and then increasing the minimum fiber length to determine at what lower cutoff the best correlation of half-time was obtained with the in vitro durability date. It was found that the best association between in vitro dissolution and lung clearance in the rat was at approximately 18 to 20 μm. At fiber lengths >20 μm, this correlation diminished as a result of fewer and fewer fibers being available in the longer size ranges.
Subsequently, in an analysis that provided the basis for the European Commission's Directive on synthetic vitreous fibers, CitationBernstein et al. (2001a, Citation2001b) reported on the correlation between the biopersistence of fibers longer than 20 μm and the pathological effects following either chronic inhalation or ip injection studies. This analysis showed that it was possible, using the clearance half-time of the fibers longer than 20 μm as obtained from the inhalation biopersistence studies, to predict the number of fibers longer than 20 μm remaining following a 24-mo chronic inhalation exposure; the early fibrotic response (collagen deposition) observed after 24 mo of exposure in the chronic inhalation toxicology studies; and the number of tumors and fiber dose in the chronic ip injection studies. These studies, however, only include SVF.
For mineral fibers, the clearance half-time of fibers longer than 20 μm ranges from a few days to less than 100 d. This is illustrated in . In this table the results are also shown from biopersistence studies performed on serpentine (chrysotile) and amphibole asbestos using the same protocol. For synthetic vitreous fibers, the European Commission established a directive that states that if the inhalation biopersistence clearance half-time of a fiber is less than 10 d, then it is not classified as a carcinogen.
TABLE 3. Clearance Half-Times of Fibers
CitationBerman et al. (1995) conducted a statistical analysis of results from 9 different asbestos types in 13 separate studies. Due to limitations in the characterization of asbestos structures in the original studies, new exposure measures were developed from samples of the original dusts that were regenerated and analyzed by transmission electron microscopy. Results reported that while no univariate model was found to provide an adequate description of the lung tumor responses in the inhalation studies, the measure most highly correlated with tumor incidence was the concentration of structures (fibers) ≥20 μm in length. However, using multivariate techniques, measures of exposure were identified that adequately described the lung tumor responses. CitationBerman et al (1995) noted that potency appeared to rise with increasing length, with structures (fibers) longer than 40 μm being about 500 times more potent than structures between 5 and 40 μm in length. Structures <5 μm in length do not appear to make any contribution to lung tumor risk. This analysis found no marked difference in the potency of chrysotile and amphibole toward the induction of lung tumors. However, CitationBerman et al. (1995) stated that the mineralogy, i.e., the physicochemical characteristics and crystal structure, appears to be important in the induction of mesothelioma, with chrysotile being less potent than amphiboles.
Recent studies on the serpentine asbestos chrysotile showed that it is not biopersistent in the lung (CitationBernstein et al., 2010; CitationRoggli & Vollmer, 2008). As serpentine is a naturally occurring mined fiber, there appears to be some differences in biopersistence depending upon where it is mined. In addition, some deposits of chrysotile are contaminated with amphibole fibers, which are highly biopersistent. However, chrysotile, per se, lies on the soluble end of this scale and ranges from the least biopersistent fiber to a fiber with biopersistence in the range of glass and stonewools. It remains less biopersistent than amphibole fibers. The 90-d subchronic inhalation toxicity study of chrysotile in rats showed that at an exposure concentration 5000-fold greater than the U.S. ACGIH (American Conference of Industrial Hygienists) threshold limit value of 0.1 f(WHO)/cm3, chrysotile produced no significant pathological response.
In summary, recent well-designed inhalation toxicity studies demonstrated that the fiber toxicity paradigm of dose, dimension, and durability in the lung is applicable to asbestos fibers. The existing database of fiber toxicity studies suggests that human exposure to respirable fibers that are biopersistent in the lung may induce significant and persistent pulmonary inflammation, cell proliferation, or fibrosis, and inhalation exposure to such fibers needs to be viewed with concern. Chrysotile fibers are less biopersistent than ceramic and special-purpose glass fibers and are considerably less biopersistent than amphibole fibers. In contrast, amphibole asbestos fibers, especially fibers longer than 20 μm, exhibit little clearance once they are deposited in the deep lung. Chronic inhalation studies showed that amphibole fibers are carcinogenic. However, despite the fact that most chrysotile fibers are cleared rapidly, some small proportion of these fibers remains in the lung, and all of the asbestos types were noted in animal models and in epidemiological studies to induce asbestosis and lung cancer, especially in smokers.
MECHANISMS OF ACTION OF ASBESTOS IN VITRO
As it became evident that prolonged exposure to asbestos fibers produced lung fibrosis, lung cancer, and mesothelioma, concern arose regarding the potential adverse health effects of fibers in general. While epidemiology studies suggested that some fibers such as most SVF did not present carcinogenic risks, the mechanisms for the different hazards of fiber types were not well understood. Research on mechanisms of fiber toxicity was initially conducted to gain a better understanding of how fibers produce harm and to provide a sound basis for health protective measures. Toxicologists have used both in vitro and in vivo assays to study fiber toxicity. In vivo bioassays provide an understanding of how fibers behave in the body, and how the body's various protective systems react to inhaled fibers. If in vivo bioassay exposures are conducted via inhalation and with realistic exposure levels, results might potentially be used to assess the health risks for humans. However, despite their great utility, in vivo bioassays are relatively time-consuming and expensive. In comparison, many investigators are attracted to in vitro cell culture assays because they are relatively quick, inexpensive, and are used to assess toxicity of small quantities of many different fiber types and sizes, which is not practical using in vivo bioassays. Organ culture models of tracheal and bronchiolar epithelium have also been used to preserve epithelial-interstitial structure. The advantages and disadvantages of in vitro assays must be carefully considered before the test results can be used appropriately. This section reviews the advantages and disadvantages of in vitro assays, both in general and specifically using asbestos and other fibers. It is hoped that its content will provide a balanced perspective on the appropriate use of in vitro results in assessing the potential health risks of fibers.
Advantages and Uses of In Vitro Assays
The main advantage of in vitro assays is that they allow specific biological and mechanistic pathways to be isolated and tested under controlled conditions in ways that are not feasible in the whole animal. In vitro tests of toxicity yield data rapidly and provide important insights and confirmations of the mechanism of in vivo effects (CitationOberdorster et al., 2005). With in vitro assays, the molecular events that produce cellular effects can be studied (CitationDonaldson et al., 2009). Several potential pathogenic mechanisms of fiber toxicity were identified using in vitro assays (CitationMossman et al., 2007). In particular, much is now known about the role of fiber morphological and/or physicochemical properties on cellular responses (CitationNIOSH, 2009). When used as a screening procedure, in vitro assays identify potential toxic materials for further investigation and assist in prioritizing fibers for further in vivo testing (CitationBernstein et al., 2005c; CitationHesterberg & Hart, 2001; CitationNIOSH 2009; CitationVu et al., 1996).
Several cell lines were used to investigate mechanisms of toxicity (CitationBernstein et al., 2005c; CitationOberdorster et al., 2005). In vitro studies with pulmonary and pleural macrophages demonstrated how “frustrated phagocytosis” may be an important mechanism of fiber toxicity. Macrophages normally protect the body from small pathogenic bacteria and viruses by engulfing (phagocytosis) and digesting them. Macrophages similarly attack inhaled fibers that reach the lung air sacs (alveoli). Data showed that even when fibers are too long to be fully engulfed (>20 μm long), the macrophage might still attempt phagocytosis. This might lead to “frustrated phagocytosis” where the macrophage membrane is pierced by an end of the fiber, and the macrophage then dies and releases its digestive enzymes, reactive oxygen species (ROS), proteases, and chemokines/cytokines, which then affect lung tissues and other cell types, producing damage and/or proliferation (CitationBernstein et al., 2005c; CitationHesterberg & Hart, 2001; CitationMossman, 2003).
Cell lines such as bronchial and alveolar epithelial cells were also used to investigate the early pathogenic mechanisms such as chromosomal damage, cell signaling (CitationShukla et al., 2003a), and mechanisms of cell proliferation or dysregulation of cell growth (CitationBernstein et al., 2005c; CitationElespuru et al., 2009). The generation of ROS and reactive nitrogen species (RNS) was implicated in DNA damage, induction of inflammatory cytokines and growth factors (CitationHei, 2003; CitationNIOSH, 2009; CitationShukla et al., 2003b). In vitro assays also allow the study of specific signal transduction pathways leading to production of inflammatory cytokines, growth factors, and regulators of the cell cycle (CitationBernstein et al., 2005c; CitationCastranova, 2003; CitationOberdorster et al., 2005; CitationShukla et al., 2009). Inflammatory cytokines promote the influx of inflammatory cells and affect lung epithelium and fibroblasts (CitationMossman & Churg, 1998).
Mechanisms of cell death by apoptosis (programmed cell death) and necrosis (lytic death) are also of interest after exposure to asbestos fibers because they may lead to compensatory cell proliferation of surrounding cells as well as metaplastic changes (CitationMossman et al., 1980; CitationWoodworth et al., 1983). Both apoptosis and necrosis were observed in lung epithelial cells in culture (CitationBuder-Hoffmann et al., 2009; CitationLiu et al., 2010; CitationMossman & Sesko, 1990; CitationPanduri et al., 2006; CitationShukla et al., 2009; CitationYuan et al., 2004), but whether these phenomena occur after exposures to asbestos fibers by inhalation is unclear. However, these in vitro models using amphibole types of asbestos (crocidolite, amosite) showed that the duration of cell signaling pathways, specifically extracellular signal-regulated kinases (ERK), regulates whether cell proliferation or death occurs. Moreover, ERK and protein kinase C activation, as well as mitochondrial- and p53-related death pathways, are all involved in death or injury of epithelial cells via elaboration of oxidants occurring after incomplete phagocytosis of long fibers or iron-dependent reactions. Finally, mechanisms of secretion of extracellular matrix components and matrix metalloproteinases (MMP) by lung epithelial cells and fibroblasts were studied to understand the early development of fibrotic lesions (CitationShukla et al., 2000).
Disadvantages of In Vitro Assays
The fundamental disadvantage of in vitro assays is that they often employ very high nonphysiological concentrations of fibers. Further, even with the added advantage of using organ cultures or cocultures, in vitro assays do not take into account the complex interactions of the different cell types and immunologic systems found in the whole animal and lung (CitationHolsapple et al., 2009; CitationOberdorster et al., 2005). Similarly, systemic or multi-organ responses, such as the complete inflammatory response, cannot be studied (CitationDonaldson et al., 2009; CitationOberdorster et al., 2005). Furthermore, toxicokinetics cannot be studied in systems in vitro because the in vivo systems for absorption, distribution, uptake, and metabolism are not present. Although dose-response studies can be performed and subsequently validated in subchronic inhalation studies (CitationShukla et al., 2004b), fiber doses per cell in studies in vitro are generally far greater than can be achieved by in vivo bioassays (CitationBernstein et al., 2005c; CitationOberdorster et al., 2005). Because of the dosage disparities, cellular responses observed with in vitro assays may not correlate with effects that occur at much lower cellular doses in vivo. Most in vitro tests have not been validated or correlated with adverse effects observed in vivo (CitationHartung, 2010; CitationOberdorster et al., 2005; CitationVu et al., 1996). Validation is also difficult for most in vitro tests because the tests do not have standardized fiber preparations, protocols, or outcomes (CitationBernstein et al., 2005c).
The responses with in vitro assays are measured after a few hours or days, while in vivo responses to biopersistent fibers are sustained over several weeks or months. With in vitro assays, the tests are too short of duration for biopersistence of fibers to come into play (CitationBernstein et al., 2005c). Research on SVF demonstrated that biopersistence with prolonged inflammation is a key mechanism of fiber toxicity (CitationHesterberg & Hart, 2000, Citation2001). Similarly, biopersistence is an important mechanism differentiating the potential adverse health effects of chrysotile asbestos from the more durable amphibole asbestos types (CitationHesterberg, 2009). Biopersistence of fibers is related to the site in the lung and rate of deposition, rates of clearance by alveolar macrophages or mucociliary transport, their lack of solubility in lung fluids (durability), their breakage rate and pattern (longitudinal or transverse), and their rates of transport across biological membranes (NIOSH, 2011). Thus, many of the processes involved in the biopersistence of fibers cannot be assessed in vitro.
Another problem with in vitro cell assays is that immortalized or transformed (malignant) cell lines most commonly used can exhibit abnormal biology (CitationHolsapple et al., 2009). Since the desired target cells are difficult to standardize and maintain, investigators often use immortalized cell lines (preneoplastic), which clearly do not represent the in vivo situation (CitationDevlin, 2009). For example, cell lines derived from human lung tumors (e.g., A549 cells), spontaneously immortalized cells, or cells transfected with viral oncoproteins (e.g., Met5A) possess changes that may alter their genotoxic, apoptotic, or proliferative responses to fibers (CitationBernstein et al., 2005c). For these reasons, use of primary and telomerase-immortalized human lung epithelial and mesothelial cells has been advocated for robust assays (CitationShukla et al., 2009) In vitro test exposure conditions also may not match those occurring in vivo, e.g., pH, specific protein content, etc. (CitationWalker & Bucher, 2009). Both pH and protein content affect the surface properties of fibers and their interaction with cells and tissues. Changes in those properties may lead to results that are vastly different between in vitro tests and in vivo bioassays (CitationOberdorster et al., 2005).
Another issue for in vitro assays is the questionable extrapolations of fiber dose to that occurring in vivo and the general lack of dose-response studies. Most publications compare fibers on a mass basis, which might not be an appropriate metric for evaluation of comparable potency between fiber types.
Despite the disadvantages just highlighted with in vitro tests, especially with fibers, there is a substantial movement in toxicology toward in vitro testing (CitationDevlin, 2009; CitationHartung, 2010; CitationHolsapple et al., 2009; CitationKrewski et al., 2010; CitationWalker & Bucher, 2009). Toxicologists recognize that the time when in vitro tests supplant in vivo bioassays is at least 10–20 yr off and will require major investments in research funding (CitationAndersen et al., 2010).
In Vitro Versus In Vivo Assays for Asbestos and Other Fibers
Research on asbestos fibers provides one example of how in vitro test results on toxicity do not correlate with in vivo bioassay findings. In most in vitro assays, chrysotile asbestos produces toxicity that is similar or greater than observed with amphiboles (e.g., crocidolite, amosite) (CitationMossman, 1993; CitationWHO, 1998). This does not agree with the in vivo laboratory studies and human epidemiology, which indicate that the amphiboles are more pathogenic than chrysotile asbestos, especially in mesothelioma (CitationYarborough, 2006, Citation2007; CitationNIOSH, 2009). However, it is important to note that the dosimetry in most past experiments is based on equal weights of fibers that may reflect more numbers of fibers and greater surface areas of chrysotile (CitationMossman et al., 1990). In vitro testing with other fibers may produce results that suggest hazards that may or may not be present after inhalation. For example, in vitro tests on fiberglass suggested far greater toxicity (i.e., false-positive) than what is observed in vivo (CitationHart et al., 1994; CitationHesterberg & Hart, 2001). On the other hand, in the case of single-walled nanotubes, in vitro tests indicate low toxicity when in vivo bioassays indicate otherwise (i.e., false-negative) (CitationDonaldson et al., 2009).
In summary, in vitro tests have proven to be useful tools for mechanistic investigations of fiber toxicity and other cell responses. However, in vitro tests also have some disadvantages that limit their utility in health risk assessment. At this time, in vitro tests can serve as an adjunct to and be predictive of the necessity for in vivo studies, but cannot replace inhalation studies. For these reasons, when trying to infer health risks, caution needs to be used when assessing the results of in vitro analysis using fibers, especially different types of asbestos and SVF. The NIOSH Roadmap Plan concluded that while in vitro experiments are invaluable for understanding fiber/cell interactions, none have been validated for use as predictors of carcinogenicity (CitationNIOSH, 2009). However, this may be possible in the future after one or more assays are validated as predictors of carcinogenic potential (CitationShukla et al., 2009).
LUNG CARCINOMAS—EPIGENETIC AND GENETIC EFFECTS
Asbestos has long been known as a potent occupational toxin and lung carcinogen (CitationRom, 1998; CitationRussi & Cone, 1994; CitationBlot & Fraumeni, 1996). While tobacco smoke is clearly the single greatest risk factor for lung cancer, increased risks for lung cancer were observed in both nonsmokers and smokers exposed to asbestos (CitationBlot & Fraumeni, 1996; CitationSelikoff et al., 1968). Moreover, epidemiologic evidence on some cohorts demonstrated that smoking and asbestos exposure have a more than additive action in inducing lung cancer (CitationSelikoff et al., 1968; CitationVainio & Boffetta, 1994). The relationship between these exposures has been termed “synergistic,” meaning that they act in a multiplicative fashion (CitationRothman, 1976).
Quantification of the magnitude of these risks is problematic as they clearly are dose, time, and fiber size and type dependent. Moreover, individual sensitivity and coexposure to other cocarcinogenic agents in the workplace may be important. The animal studies described previously are generally conducted in inbred strains, whereas humans are genetically heterogenous. Recent deep sequencing studies indicate that sensitivity to certain drugs and other agents may be linked to rare alleles that are not identified in genome-wide association studies. Therefore, it is likely that inhalation of asbestos fibers in humans results in a broad range of responses that vary with genotype (e.g., individual sensitivity), as well as gender and behavioral and nutritional influences. Hence, caution needs to be exerted when extrapolating mechanisms from animal or in vitro studies to disease endpoints that occur in humans individually.
Epidemiologic data addressing the lung cancer risk from asbestos exposure and the accepted interaction with tobacco smoke exposure have been intensively analyzed, but this interaction appears to hold for all histologic subtypes (CitationVainio & Boffetta, 1994). Further, although fraught with uncertainty, the dose extrapolations are also consistent with a linear dose-response for the interactive risk. Recent models also suggest that attained age is an important risk modifier, at least in the case of chrysotile asbestos exposure (CitationRichardson, 2009). While the precise mechanisms of action of asbestos acting as a lung carcinogen either alone or in combination with tobacco smoke exposure remain unclear, recent molecular investigations are beginning to suggest fruitful avenues for research (CitationHeintz et al., 2010).
Asbestos has long been known to produce numerical and structural chromosomal aberrations (CA), aneuploidy, breakage of DNA, and impairment of cell division in various cell types in vitro (CitationHuang et al., 2011, this issue; CitationHEI, 1991). Genotoxicity is observed most commonly at lethal concentrations of asbestos. Moreover, it is unclear whether genotoxicity is a mechanism of asbestos carcinogenicity in lung epithelial cells. In most of the studies, using lung cells, a single type and/or one high concentration of asbestos was used, i.e., crocidolite (CitationAult et al., 1995; CitationJensen et al., 1996; CitationCole et al., 1991) or amosite in various amounts (CitationKamp et al., 1995). Other studies using human bronchial epithelial cells exposed to crocidolite or chrysotile asbestos at various concentrations (CitationKodama et al., 1993) have proven negative. Dose-response studies revealed the absence of statistically significant gene or chromosomal endpoints, aneuploidy, or CA in Chinese hamster fibroblasts (V79 cells) at lowest concentrations of crocidolite or chrysotile (CitationPalekar et al., 1987).
Chrysotile and crocidolite asbestos induce changes in gene expression in homeostatic pathways, as well as signaling pathways critical to the induction of cancer, such as nuclear factor (NF)-κB inflammatory cascade, TP53 regulatory pathways, activator protein-1 (AP-1) and mitogen-activated protein kinases (MAPK) cascades, or PDGF (platelet-derived growth factor) pathways (CitationJanssen et al., 1997; CitationMossman et al., 2006; CitationShukla et al., 2004a, Citation2006; CitationBrody et al. 1997). Finally, investigations of human tumors in asbestos-exposed lung cancer patients found that asbestos exposure was associated with specific somatic anomalies, including the k-ras oncogenic pathway (CitationNymark et al., 2008), loss of chromosome 3p (CitationMarsit et al., 2004; CitationNymark et al., 2006) and FHIT gene deletion (CitationNelson et al.,1998; CitationPylkkanen et al., 2002), loss of 19p13 (CitationNymark et al., 2009; CitationRuosaari et al., 2008) and 2p16 (CitationKettunen et al., 2009), alterations in the TP53 pathway, and deletion or epigenetic activation of the gene encoding p16 (CitationKim et al., 2001; CitationKraunz et al., 2006). It is noteworthy that the vast majority of investigations into lung cancer, including those now delineating the spectrum of both genetic and epigenetic gene activation and inactivation, do not report (or perhaps have no knowledge of) asbestos exposure or lung content and types of fibers. This has greatly hampered the study of the distinct nature of asbestos-induced lung cancer and is the single greatest barrier to accumulation of knowledge in this field.
CitationHusgafvel-Pursiainen et al. (1993) first suggested that asbestos exposure enhanced the association of tobacco smoke exposure and the occurrence of k-ras mutations in adenocarcinoma. Mutations at k-ras are primarily G to T transversions at codon 12, and this type of alteration (transversion mutation) was associated with the action of polycyclic aromatic hydrocarbons (PAH) and likely represents the action of tobacco carcinogens at the cellular level (CitationDenissenko et al., 1996). Additional studies confirmed this observation of asbestos enhancement of smoking-related mutations (CitationHusgafvel-Pursiainen et al., 1995; CitationNelson et al., 1999; CitationWang et al., 1995). However, potential confounding by histology may obscure the ability to detect associations (CitationHusgafvel-Pursiainen et al., 1999). In addition, CitationNelson et al. (1999) reported that these types of transversion mutations at k-ras occur in asbestos-exposed individuals without evidence of fibrotic alterations. This molecular evidence implies that asbestos exposure is related to lung cancer in the absence of asbestosis. In addition, p53 mutations and aberrant protein expression in lung cancer have been associated with asbestos exposure (CitationHusgafvel-Pursiainen et al., 1995; CitationLiu et al., 1998a; CitationWang et al., 1995). The prevalence of G:C to T:A transversion mutations at p53 is enhanced by asbestos exposure in a fashion related to combined asbestos and tobacco carcinogen dose (CitationWang et al., 1995). This adds further molecular evidence to the postulation that asbestos fibers act by enhancing the delivery of PAH carcinogens and increase their DNA adduct formation in epithelial cells of the lung (CitationEastman et al., 1983).
Loss of chromosome 3p and alterations of the FHIT gene have been reported to be associated with asbestos exposure (CitationMarsit et al., 2004; CitationNymark et al., 2006; CitationWikman et al., 2007; CitationNelson et al., 1998; CitationPylkkanen et al., 2002). This region is a fragile site, and asbestos may act preferentially to disrupt fragile DNA. Alternatively, there may be additional phenotypes to clones with these deletions (e.g., epigenetic perturbations enhance asbestos-associated genotoxicity) that remain obscure. CitationKettunen et al. (2009) also showed that locus-specific copy number loss and allelic imbalance at 2p16.3-p16.2 are associated with asbestos exposure in lung tumors, whereas low-level copy number gains at 2p21 are common in lung cancer in general. Indeed, these data supplement previously identified asbestos-associated alterations in other chromosomal regions, such as 19p13 and 9q33.1 (CitationNymark et al., 2009; CitationRuosaari et al., 2008). Epigenetic changes at p16 are also correlated with carcinogen exposure (CitationKraunz et al., 2006; CitationKim et al., 2001) and further suggest that asbestos-specific genetic and epigenetic changes may exist (CitationDammann et al., 2005). The association of epigenetic changes with asbestos exposure in mesothelioma (CitationChristensen et al., 2008, Citation2009), in parallel with the known significant array of epigenetic changes that occur in lung cancer (CitationPfeifer & Rauch, 2009) argues that asbestos, acting as a lung carcinogen, also contributes in an important fashion to epigenetic gene alterations, defined as changes in methylation or autylation of genes. However, it is difficult to determine effects of specific types of asbestos in these studies because of mixed exposures to asbestos in humans. Finally, expression array experiments, designed to specifically delineate asbestos-associated pathway disruption, were recently observed (CitationRuosaari et al., 2008). These data are consistent with prior studies, again suggesting that specific pathways may be targeted for disruption by asbestos.
All of these data clearly suggest that asbestos-associated lung cancers have at least some differences in gene pathways that are inactivated. This may translate to differences in genetic change in somatic genes. The precise mechanisms responsible for the action of asbestos as a lung carcinogen either alone or in combination with tobacco smoke remain the subject of considerable scrutiny. Studies using a molecular epidemiologic approach are suggesting that combined exposures result in more DNA changes of the particular type produced by tobacco carcinogens. In addition, evidence now suggests that molecular changes may be independent of fibrosis and implies that the complex pathways for the development of fibrosis and lung cancer may be, at least in some critical ways, distinct. Finally, there is little doubt that epigenetic changes play a crucial role in asbestos-associated cancer, but this is poorly studied and not linked to specific types of asbestos.
In Vivo Studies of Inflammation and Oxidants as Mediators of Asbestos-Promoted Lung Diseases
Asbestos fibers also may act at subsequent stages in the protracted development (generally >20 yr) of lung cancers and fibrosis, as do classical tumor promoters, such as phorbol esters (CitationMossman et al., 1985, Citation1990). These agents primarily induce inflammation, cell proliferation, and further genetic instability. Inhaled asbestos fibers elicit acute and chronic inflammatory responses at sites of deposition along airways and in alveolar spaces. These responses are linked to development of both malignant (lung cancer and mesothelioma) and nonmalignant (asbestosis) lung and pleural diseases (CitationBringardner et al., 2008; CitationMossman & Churg, 1998). Human and animal studies demonstrated that ROS generation following exposures to various asbestos types is essential in signaling for cytokine-dependent innate immune responses (CitationBringardner et al., 2008; CitationDostert et al., 2008; CitationHaegens et al., 2005; CitationMossman et al., 2006), lung matrix remodeling (CitationBringardner et al., 2008), and cell proliferation or transformation (CitationShukla et al., 2003b). It is evident that asbestos elicits pathogenic ROS-dependent cell signaling and tissue matrix remodeling through multiple mechanisms as oxidants are generated both directly from surface reactions on the fibers and indirectly following partial engulfment by leukocytes and epithelial cells. These sources of oxidants initiate secondary and prolonged inflammatory signaling for recruitment of innate immune responses and tissue remodeling. This section focuses on in vivo studies of mechanisms of oxidant generation by asbestos fibers and the consequent oxidant-dependent signaling pathways that may underlie the pathogenesis of asbestos promoted lung diseases.
Asbestos-promoted inflammation and fibrosis occur in a dose-related fashion with more striking severity of injury, cell proliferation, early response protooncogene expression, and fibrosis by crocidolite compared to chrysotile in subchronic inhalation exposures (CitationShukla et al., 2004b; CitationQuinlan et al., 1994, Citation1995; CitationBeruBe et al., 1996). Fiber biopersistence and the capacity to generate ROS appear important in vivo (CitationMossman & Churg, 1998; CitationGhio et al., 2008). Various types of asbestos spontaneously catalyze the formation of ROS in aqueous solutions or after uptake by cells (CitationShukla et al., 2003b; CitationGhio et al., 2008). Moreover, in vitro oxidant production from asbestos fibers increases with the concentration of iron (Fe) and other redox-active cations in the lung tissue that catalyze Fenton reactions (CitationShukla et al., 2003b; CitationGhio et al., 2008). Iron may be mobilized from asbestos fibers, the fibers may adsorb Fe extracellularly in the lung or pleura, and asbestos fibers may alter lung Fe homeostasis with a net increase in Fe accumulation (CitationGhio et al., 2008, Citation2009). Fenton-like reactions reduce molecular oxygen to superoxide that is catalytically dismuted to hydrogen peroxide (H2O2) by intracellular and extracellular superoxide dismutases. Further reduction of H2O2 via reaction with superoxide in Fe-driven Fenton-like reactions forms highly reactive hydroxyl radical on the surface of the fibers or at sites where leached Fe(II) is free and not sequestered (CitationMossman & Churg, 1998; CitationGhio et al., 2008). Alternatively, evidence from genetic mouse models indicates that H2O2 generated either from fibers after inhalation or as a result of cellular respiratory bursts is converted to hypochlorous acid (HOCl) in a reaction catalyzed by myeloperoxidase (CitationHaegens et al., 2005). Evidence from a number of genetic rodent models with selected overexpression or absence of pro- or antioxidant enzymes suggests that that these reactions occur in rodent lungs after in vivo inhalation or intratracheal instillation of asbestos or silicates, but not inert dusts (CitationMossman & Churg, 1998; CitationGhio et al., 2008). Human studies also provide significant evidence of persistent oxidative stress and oxidant generation in patients with asbestos-related lung diseases (CitationGhio et al., 2008; CitationChow et al., 2009).
Direct generation of ROS by Fe or other metals associated with asbestos fibers, however, cannot account for all oxidative stress from inhaled asbestos. This additional oxidant stress derives from cellular respiratory bursts generated as fibers contact or are engulfed by alveolar macrophages, infiltrating neutrophils, and airway epithelial cells (CitationMossman & Churg, 1998; CitationBringardner et al., 2008). Longer, more fibrogenic fibers of asbestos produce a frustrated, ineffective phagocytosis and more protracted elevations in release of ROS (CitationMossman & Churg, 1998). The respiratory burst is mediated by activation of phagocyte or epithelial cell NADPH oxidases, multimeric protein complexes that generate superoxide and subsequent H2O2 when stimulated by microbe and particle phagocytosis, or receptor signaling (CitationDostert et al., 2008; CitationHordijk, 2006; CitationQuinn et al., 2006). As indicated earlier, neutrophil myeloperoxidase, either within the cell or leached across the epithelial cell barriers, catalyzes conversion of H2O2 to the more potent oxidant HOCl. In addition, myeloperoxidase is a source of nitric oxide-derived oxidants when sufficient nitrate and nitrite are present (CitationGaut et al., 2002). Reactive nitrogen species (RNS) are increased in alveolar macrophages recovered from rats exposed by inhalation to fibrogenic concentrations of asbestos (CitationQuinlan et al., 1998). These elevations are linked to enhanced production of nitrate and nitrite (CitationThomas et al., 1994), as well as increased expression of inducible nitric oxide synthease (iNOS) (CitationQuinlan et al., 1998). Importantly, nitration of proteins, a hallmark of inflammation, is elevated in the lungs of rats after inhalation of asbestos, and mice that lack myeloperoxidase display reduced asbestos-induced lung inflammation and epithelial cell remodeling relative to their myeloperoxidase competent littermates (CitationHaegens et al., 2005) Nitric oxide (NO . ) released by inflammatory cells might also react with O .− 2 to form peroxynitrite (ONOO−). ONOO− produces nitration of tyrosine residues on proteins and thereby modifies protein function. Nitrated proteins were identified in lung tissue following exposure to asbestos fibers (CitationTanaka et al., 1998). Furthermore, ONOO− produced dysregulation of growth factor receptor tyrosine kinase signaling (CitationZhang et al., 2000). Therefore, ONOO− generation and subsequent tyrosine nitration leading to protein dysfunction appear to contribute to disease progression following asbestos-induced lung injury.
It is important to note that the acute inflammatory response to asbestos fibers elicits more protracted inflammatory cell influx through gene induction and expression of pro-inflammatory cytokines (CitationMossman & Churg, 1998; CitationBringardner et al., 2008). Oxidants derived from Fe-catalyzed reactions or asbestos-activated NADPH oxidase may signal for cytokine-mediated responses by activating the macrophage Nod-like receptor protein (Nalp3) inflammasome that elicits an IL1β-driven innate immune response (CitationDostert et al., 2008). In a recent in vivo study, mice that lacked Nalp3 and inhaling asbestos displayed reduced infiltration of a number of cell types, including lymphocytes, eosinophils, and neutrophils, as well as decreased expression of cytokines that would elicit this infiltration (CitationDostert et al., 2008). Inhibition or elimination of NADPH oxidase activity or expression prevented asbestos-induced inflammasome activation and IL1β expression, as did chelating Fe from the asbestos fibers. It is significant that not all asbestos-induced cytokine expression or responses in these studies were reduced in Nalp3 –/– mice, indicating that additional signals are generated to elicit asbestos-induced inflammatory responses (CitationDostert et al., 2008). CitationSabo-Attwood et al. (2005) also suggested this in gene profiling experiments on whole lungs of mice in a chrysotile asbestos model of epithelial cell proliferation, inflammation, and fibrogenesis.
The role of inflammation, especially the transient acute phase of cellular infiltration, in the pathogenesis of particle-induced fibrosis has been controversial (CitationMossman & Churg, 1998; CitationBringardner et al., 2008). This controversy is fueled by a lack of prolonged cellular infiltrates and the fact that immunosuppressive therapies fail to treat the disease (CitationBringardner et al., 2008). However, localized inflammatory responses promote fibroblast dysfunction, and epithelial cell proliferation may be a critical factor in promoting asbestos-related disease (CitationManning et al., 2008). Localized dysfunction from pro-fibrotic agents, including asbestos, may result in further inflammatory responses, matrix remodeling, release of matrix-bound ligands and cytokines, localized activation of growth factor signaling, and altered tissue vascularization (CitationBringardner et al., 2008) There is significant evidence that implicates localized ROS generation in asbestos-induced stimulation of each of these processes (CitationShukla et al., 2003b). It is well recognized that inhalation of asbestos fibers leads to focal epithelial cell proliferation and alveolar macrophage activation at sites of deposition, especially at impacted airway and alveolar duct bifurcations (CitationLiu et al., 1997, Citation1998b; CitationBeruBe et al., 1996; CitationRobledo et al., 2000; CitationBrody et al., 1997; CitationMossman & Churg, 1998; CitationShukla et al., 2000; CitationBrody & Roe, 1983; CitationSullivan et al. 2008). In vitro and in vivo data also indicate that the asbestos-impacted epithelial cells signal for transcriptional induction of cytokines that initiate the localized inflammatory and proliferative responses (CitationMossman et al., 2006; CitationHoyle & Brody, 1996; CitationJanssen et al., 1997). Persistent increases in nuclear localization of NF-κB, a primary transcription factor regulating cytokine expression, were observed in interstitial and pleural cells of rats long after the acute inflammatory response to crocidolite asbestos subsided (CitationJanssen et al., 1997). Similarly, persistent localization of extracellular signal-regulated kinases (ERK) and AP-1 factor activation is an epithelial cell-specific response in various asbestos inhalation models (CitationMossman et al., 2006; CitationCummins et al. 2003). These processes result in cytokine and stress gene induction and are reduced by antioxidants (CitationShukla et al., 2004b). In addition, expression of the well-known tumor suppressor protein p53 is upregulated at sites of asbestos injury consequent to inhalation of chrysotile asbestos by rats (CitationMishra et al.,1997).
In conjunction with cellular activation, oxidative matrix degradation, or oxidant activation of matrix remodeling proteins, asbestos fibers activate growth factor receptor ligands and inflammatory mediators that promote cell proliferation and tissue remodeling. Tissue growth factor-β (TGF-β) is one example of a potent profibrotic stimulant of matrix remodeling and fibroblast proliferation that is affected by asbestos fibers (CitationLiu & Brody, 2001; CitationMossman & Churg, 1998; CitationSullivan et al., 2008). TGF-β is deposited on alveolar surfaces in a latent form bound in the matrix by latent associated peptide (LAP) (CitationBringardner et al., 2008; CitationSullivan et al., 2008). CitationSullivan et al. (2008) recently provided in vivo evidence that complemented earlier in vitro studies showing that asbestos-derived oxidants promote degradation of the TGF-β complex with LAP to provide active ligand for the TGF-β receptor (CitationPociask et al., 2004). TGF-β often acts in conjunction with matrix-bound connective-tissue growth factors to coordinate fibroblast proliferation, migration, and collagen synthesis (CitationSullivan et al., 2008). In addition, asbestos exposures led to increased signaling through epithelial-cell epidermal growth factor receptors (CitationManning et al., 2002), which might be stimulated both by the fibers themselves and by TGF-α released from the matrix.
In summary, inhalation of asbestos fibers may result in a number of pulmonary diseases that are mediated in part by acute and chronic inflammatory responses. Generation of ROS through surface reactions of the fibers, inappropriate Fe mobilization in the lung, and stimulation of cellular oxidant-generating enzymes are central to these inflammatory responses. It is evident that asbestos-induced oxidative stress promotes pulmonary inflammation through stimulation of innate immune responses and matrix degradation that liberates additional inflammatory mediators. In addition, long after acute inflammatory responses have subsided, focal signaling results in tissue and cellular release of growth factors and cytokines that promote chronic inflammation, fibrogenesis, matrix remodeling, and proliferative responses central to asbestos-related pulmonary diseases.
ASBESTOSIS: MECHANISMS OF ACTION OF ASBESTOS
Various inhaled particles and fibers produce injury to the lungs, but asbestos fibers have unique physical and chemical characteristics that make them particularly insidious (CitationBonner, 2007). Inhaled asbestos fibers deposit initially at the bronchiolar–alveolar duct (BAD) regions of the lung in rats and mice (CitationBrody, 1993). As summarized previously, in rodent models, inhaled fibers induce proliferation of epithelial and mesenchymal cell populations (CitationBrody et al., 1997), and early signaling events are likely to begin with epithelial cell activation (CitationManning et al., 2002). One of the first signaling events triggered on the epithelial surface of the BAD region is the cleavage of complement C5 to C5a, a potent chemoattractant for alveolar macrophages (CitationWarheit et al., 1986). Macrophages migrate to sites of fiber deposition within 1 d of inhalation exposure, engulfing short fibers (less than approximately 10 μm in length in rats) and removing these fibers via the mucociliary apparatus or via the pulmonary lymphatic system that may transport the same fibers to other lymph nodes, the pleural space, and mesothelial surfaces of the pleural (CitationBoutin et al., 1996) and peritoneal cavities (CitationDodson et al., 1990). Longer fibers may be retained more commonly in the lung due to the inability of macrophages to remove them via these processes. In addition, asbestos fibers not removed by macrophages penetrate the epithelium over time and move into the interstitial spaces and thereby interact directly with fibroblasts, smooth muscle cells, and other interstitial cell types (CitationBrody et al., 1981; CitationChang et al., 1988; CitationCoin et al., 1992).
Macrophage–Fibroblast Signaling During Asbestosis: PDGF as a Mediator of Proliferation
As mentioned earlier, macrophages play a central role in the clearance of inhaled asbestos fibers from the lungs. Macrophage-mediated clearance is effective for short fibers, but not longer fibers that persist to chronically irritate lung tissues and stimulate macrophages and other pulmonary cell types to produce soluble factors that play roles in tissue repair, remodeling, or chronic disease (see earlier discussion). One such factor is platelet-derived growth factor (PDGF), a polypeptide that binds to specific receptors on fibroblasts to stimulate cell migration and proliferation (CitationBonner, 2004). A number of studies indicate that PDGF plays a role in asbestosis. For example, levels of PDGF-A and -B mRNAs are increased in rat lung following an inhalation exposure to chrysotile asbestos (CitationLiu et al., 1997). These in situ hybridization experiments show that PDGF-A and -B chain RNAs are localized primarily in macrophages and bronchioalveolar epithelial cells at sites of initial fiber deposition and lung injury (CitationLiu et al., 1997). Two distinct molecular masses of PDGF-like factors (approximately 34 and 16 kD) are secreted by asbestos-stimulated rat alveolar macrophages in vitro. These factors were primarily identified as PDGF-B chain molecules and represented the majority of macrophage-derived mitogenic activity for cultured rat lung fibroblasts. Macrophage-derived PDGF was identified as a major chemotactic factor for rat lung fibroblasts. The PDGF-B chain isoforms are more potent as fibroblast chemoattractants as compared to PDGF-A. Asbestos fibers also influence the expression of PDGF receptors on rat lung fibroblasts in vitro, and this receptor is also increased in the lungs of mice in vivo following asbestos inhalation (CitationLasky et al., 1998). In addition to asbestos regulating PDGF ligand and receptor levels, fibers also modulate the expression of alpha-2-macroglobulin, a PDGF-binding protein (CitationBonner and Brody, 1995). While there is currently no effective treatment for the pathogenesis of asbestosis, PDGF signaling and proliferative responses can be inhibited by the PDGF receptor kinase inhibitor imatinib mesylate (Gleevec), which shows some promise as an antifibrotic therapy in experimental animal models of fibrosis (CitationIngram & Bonner, 2006).
Macrophage–Fibroblast Signaling During Asbestosis: TNF-α and TGF-β1 as Mediators of Matrix Production
Macrophages are also a rich source of TGF-β1, which promotes collagen deposition by fibroblasts and thereby serves as a potent profibrogenic mediator (CitationPerdue & Brody, 1994; CitationBrass et al., 1999). Immunohistochemistry studies demonstrated that TGF-β1 is expressed primarily in macrophages and the bronchoalveolar epithelial cells at BAD regions where asbestos fibers accumulate after inhalation (CitationPerdue & Brody, 1994). Increased TGF-β1 occurs after the PDGF-induced proliferative phase of pulmonary fibrogenesis. Fibroblasts treated with TGF-β1 undergo growth arrest and downregulate PDGF receptor expression, but enter a synthetic phase wherein collagen and other matrix proteins are produced (CitationBonner & Brody, 1995). These extracellular matrix proteins define the fibrotic lesion. Several lines of evidence indicate that TGF-β1 production is dependent on the expression of tumor necrosis factor (TNF)-α. First, human alveolar macrophages exposed to chrysotile asbestos fibers in vitro demonstrated a significant rise in release of TNF-α followed by TGF-β1 secretion (CitationLiu & Brody, 2001). Second, administration of chrysotile asbestos fibers to rats results in fibrosis, which corresponds to increased TGF-β1 and which is preceded by an elevation in bronchoalveolar lavage (BAL) fluid TNF-α expression (CitationLiu & Brody, 2001). Third, C57BL6 mice develop asbestos-induced fibroproliferative lesions at the alveolar duct bifurcations, the predominant site of fiber deposition where TNF-α expression is localized to macrophages and alveolar epithelial cells in this murine model of chrysotile asbestos-induced fibrogenesis (CitationBrass et al., 1999) However, J129 mice, which do not develop macrophage infiltration or fibroproliferative lesions in response to asbestos, do not increase their TNF-α expression, as assessed by in situ hybridization and immunohistochemistry (CitationBrass et al., 1999). In addition, double TNF-α receptor knockout mice are protected from asbestos-induced fibrosis and do not have elevated levels of TGF-β1 (CitationLiu & Brody, 2001). Finally, two separate studies on the signal transduction process through which TNF-α mediates the expression of TGF- β1 showed that signaling goes through a MEK-ERK pathway and the AP-1 transcription factor (CitationSullivan et al. 2005, Citation2009). Thus, there is convincing evidence that suggests a critical role for TNF-α in the regulation of TGF-β1 production and the pathogenesis of asbestosis.
Epithelial–Fibroblast Signaling During Asbestosis: EGFR Ligands
While initially much attention focused on the role of macrophages in mediating fibroblast proliferation and collagen deposition, later investigations emphasized the role of the epithelium as a central mediator of fibroblast responses during asbestos-induced fibrogenesis. Communication between the airway epithelium and underlying fibroblasts and smooth muscle cells via paracrine signaling is referred to as the “epithelial mesenchymal cell trophic unit.” Epithelial–mesenchymal cell interactions also are postulated to play an important role in the pathogenesis of asthema (CitationIngram & Bonner, 2006). The epithelial–mesenchymal cell trophic unit is likely important to understanding the pathogenesis of asbestosis. Epithelial cells produce a variety of factors, including epidermal growth factor receptor (EGFR) ligands. Signaling through EGFR on fibroblasts was shown to play an important role in asbestos-induced fibrosis in two major ways. First, EGFR ligands are produced by epithelial cells, and then function through paracrine signaling to activate EGFR and promote fibroblast proliferation. Asbestos exposure increases the expression of TGF-α in the lung epithelium (CitationLiu & Brody, 2001), and TGF-α was fopund to play a critical role in fibrogenesis. For example, targeted expression of TGF-α using the SP-C gene promoter causes spontaneous pulmonary fibrosis in mice (CitationHardie et al., 2004). Second, the EGFR is a central conduit through which ROS mediate their cell signaling effects on epithelial cells, fibroblasts, and other cell types (CitationShukla et al., 2006), and cell proliferation and expression of early response (fos/jun) protooncogenes are diminished in EGFR kinase-deficient mice (CitationManning et al., 2002). ROS, such as H2O2, produced by asbestos are also known to transiently inhibit protein tyrosine phosphatase (PTP) activity associated with dephosphorylation of EGFR. Inhibition of PTP by asbestos-generated ROS produced prolonged activation of EGFR in a “ligand-independent manner” that activated a variety of intracellular signaling cascades described next. The EGFR may represent a potential therapeutic target for asbestosis, especially since blocking the EGFR with a tyrosine kinase inhibitor significantly reduces pulmonary fibrosis in rats (CitationRice et al., 1999).
Intracellular Signaling Cascades in Asbestosis
The pathogenesis of asbestos-associated fibrosis involves the participation of a number of cell types and is characterized by an early and persistent inflammatory response that involves the generation of oxidants, growth factors, chemokines, and cytokines (CitationRobledo & Mossman, 1999). These mediators may also contribute directly to cell injury, proliferation, and fibrogenesis. After interaction with cells, asbestos fibers trigger a number of signaling cascades involving mitogen-activated protein kinases (MAPK) and nuclear factor kappaB (NF-κB). Activation of transcription factors such as NF-κB and activator protein-1 (AP-1) may be linked to increases in early response genes (e.g., c-jun, c-fos, fra-1) that govern proliferation, apoptosis, and inflammatory changes in the cells of the lung (CitationHeintz et al., 1993; CitationShukla et al., 2003b). Cell signaling in response to asbestos fibers may occur with rapid kinetics. For example, asbestos-induced EGFR phosphorylation, a ligand-independent process, occurs within minutes and leads to downstream MAP kinase kinase (MEK) and subsequent MAPK activation. Activated MAPK (e.g., ERK-1 and -2) translocate to the nucleus and activate transcriptional programs (CitationYuan et al., 2004). Recent studies using a genetic mouse model showed that targeting the MEK cascade in lung epithelium inhibits proliferation and fibrogenesis by crocidolite asbestos after inhalation (CitationManning et al., 2008). Therefore, the EGFR–MEK–MAPK cascade is likely an important target for therapeutic intervention, as indicated previously. It is important to note that a cell signaling cascade initiated by asbestos fibers or asbestos-generated ROS differs significantly from the identical cell signaling cascade initiated by an endogenous growth factor or cytokine, and results in a completely different phenotypic outcome. Activation of the EGFR by TGF-α induces fibroblast proliferation, or epithelial cell differentiation, whereas activation of EGFR by asbestos fibers also results in the production of inflammatory cytokines. This difference in biologic response might be due to the fact that growth factors produce transient phosphorylation of EGFR, whereas asbestos fibers (as well as certain metals) induce prolonged phosphorylation of EGFR (CitationZanella et al., 1996). Therefore, timing might be the key to understanding how asbestos fibers produce pathologic effects in the lung.
In summary, ROS generated by asbestos exposure or asbestos–cell interactions may serve as signaling intermediates to activate intracellular signaling targets, including receptor tyrosine kinases, MAPK kinases, and transcription factors. These signaling intermediates drive transcriptional activation and the up- or downregulation of genes involved in inflammation and fibrosis.
PROBLEMS IN INTERPRETATION OF PAST STUDIES AND SUGGESTIONS FOR FUTURE WORK
1. | In review of the literature, it is often difficult to evaluate the exposure or dose of asbestos administered to rodents in comparison to human exposures. Often the bivariate size distribution of the fibers, whether dose-response was evaluated, and the route of exposure are not reported in the study. The expression of fiber dosimetry is often the weakest component in studies. Many studies report only gravimetric concentration or doses, with no information provided on fiber number or bivariate fiber size distribution. The latter is of particular importance in differentiating effects of various types of fibers as fiber length is related to potency. | ||||
2. | Dose-response studies are lacking in most in vivo and in vitro studies. The large majority of earlier inhalation exposure studies and many of the more recent studies were performed at high “overload” doses. Further, there are few quantitative data presented in past publications on nonfibrous particle content of test fiber preparations. Since nonfibrous particles contribute to lung overload (CitationOberdorster, 1995a), particle/fiber dimensions need to be comprehensively evaluated in materials used in future studies. Moreover, well-designed inhalation studies need to be performed in the range of concentrations of human exposures or within one or two orders of magnitude. In addition, it is important to include dose-response studies with multiple exposure levels. | ||||
3. | Well-characterized reference samples of various types of asbestos and SVF need to be obtained and provided to investigators in the field, as older reference samples (UICC, NIEHS) are in general depleted and may be altered or contaminated after decades of storage. | ||||
4. | The mode of action (MOA) of various types of asbestos and naturally occurring asbestiform or SVF fibers may be different in various lung and pleural diseases and likely related to multiple characteristics of fibers. In addition, the relevant doses, sizes, and other characteristics of fibers may be different in elicitation of individual toxic and other phenotypic/genetic changes critical to the development of mesothelioma, lung cancers, and fibrosis. Experiments to decipher these variables are needed and will benefit from multitiered approaches to resolve these questions. |
DEFINITIONS
Genotoxicity: Property of an agent for altering the genome of cells resulting in cell death or altered function and division of cells, including alterations in the genetic material, e.g., sister chromatid exchanges, transferred to cell progeny.
Mutagenicity: Property of an agent to cause alterations in the genome that are transferred to cell progeny and subsequent generations.
Carcinogenicity: Property of an agent to alter the genome and/or cellular control processes resulting in uncontrolled cell proliferation and other functional or phenotypic changes that in turn result in malignant cancers.
Acknowledgments
We appreciate the comments and editing of Drs. Gene McConnell, Annie Jarabek, David Bernstein, Arnold Brody, and Maureen Gwinn. We thank Jennifer Díaz (Department of Pathology, UVM) for multiple revisions to this paper.
REFERENCES
- Agency for Toxic Substances and Disease Registry . 2003 . Report on the Expert Panel on Health Effects of Asbestos and Synthetic Vitreous Fibers: The Influence of fiber length , Atlanta, GA : Agency for Toxic Substances and Disease Registry, Division of Health Assessment and Consultation .
- Albin , M. , Pooley , F. D. , Stromberg , U. , Attewell , R. , Mitha , R. , Johansson , L. and Welinder , H. 1994 . Retention patterns of asbestos fibres in lung tissue among asbestos cement workers . Occup. Environ. Med , 51 : 205 – 211 .
- Andersen , M. E. , Al-Zoughool , M. , Croteau , M. , Westphal , M. and Krewski , D. 2009 . The future of toxicity testing . J. Toxicol Environ. Health B , 13 : 163 – 196 .
- Ashcroft , T. , Simpson , J. M. and Timbrell , V. 1988 . Simple method of estimating severity of pulmonary fibrosis on a numerical scale . J. Clin. Pathol , 41 : 467 – 470 .
- ATS . 2004 . Diagnosis and initial management of non-malignant diseases related to asbestos . Am. J. Respir. Crit. Care Med , 170 : 691 – 715 .
- Ault , J. G. , Cole , R. W. , Jensen , C. G. , Jensen , L. C. , Bachert , L. A. and Rieder , C. L. 1995 . Behavior of crocidolite asbestos during mitosis in living vertebrate lung epithelial cells . Cancer Res , 55 : 792 – 798 .
- Balashazy , I. , Moustafa , M. , Hofmann , W. , Szoke , R. , El-Hussein , A. and Ahmed , A. R. 2005 . Simulation of fiber deposition in bronchial airways . Inhal. Toxicol , 17 : 717 – 727 .
- Bellmann , B. , Muhle , H. , Creutzenberg , O. , Ernst , H. , Brown , R. C. and Sebastien , P. 2001 . Effects of nonfibrous particles on ceramic fiber (RCF1) toxicity in rats . Inhal. Toxicol , 13 : 877 – 901 .
- Bellmann , B. , Muhle , H. , Creutzenberg , O. , Ernst , H. , Muller , M. , Bernstein , D. M. and Riego Sintes , J. M. 2003 . Calibration study on subchronic inhalation toxicity of man-made vitreous fibers in rats . Inhal. Toxicol , 15 : 1147 – 1177 .
- Berman , D. W. , Crump , K. S. , Chatfield , E. J. , Davis , J. M. and Jones , A. D. 1995 . The sizes, shapes, and mineralogy of asbestos structures that induce lung tumors or mesothelioma in AF/HAN rats following inhalation . Risk Anal , 15 : 181 – 195 .
- Bernstein , D. , Castranova , V. , Donaldson , K. , Fubini , B. , Hadley , J. , Hesterberg , T. , Kane , A. , Lai , D. , McConnell , E. E. , Muhle , H. , Oberdorster , G. , Olin , S. and Warheit , D. B. 2005c . Testing of fibrous particles: Short-term assays and strategies . Inhal. Toxicol , 17 : 497 – 537 .
- Bernstein , D. , Rogers , R. and Smith , P. 2005b . The biopersistence of Canadian chrysotile asbestos following inhalation: final results through 1 year after cessation of exposure . Inhal. Toxicol , 17 : 1 – 14 .
- Bernstein , D. M. 2007 . Synthetic vitreous fibers: A review toxicology, epidemiology and regulations . Crit. Rev. Toxicol , 37 : 839 – 886 .
- Bernstein , D. M. , Chevalier , J. and Smith , P. 2003a . Comparison of Calidria chrysotile asbestos to pure tremolite: Inhalation biopersistence and histopathology following short-term exposure . Inhal. Toxicol , 15 : 1387 – 1419 .
- Bernstein , D. M. , Chevalier , J. and Smith , P. 2005a . Comparison of Calidria chrysotile asbestos to pure tremolite: final results of the inhalation biopersistence and histopathology examination following short-term exposure . Inhal. Toxicol , 17 : 427 – 449 .
- Bernstein , D. M. , Donaldson , K. , Decker , U. , Gaering , S. , Kunzendorf , P. , Chevalier , J. and Holm , S. E. 2008 . A biopersistence study following exposure to chrysotile asbestos alone or in combination with fine particles . Inhal. Toxicol , 20 : 1009 – 1028 .
- Bernstein , D. M. , Drew , R. T. , Schidlovsky , G. and Kuschner , M. 1984 . “ Pathogenicity of MMMF and the contrasts with natural fibres ” . In Biological effects of man-made mineral fibres , Vol. 2 , 169 – 195 . Copenhagen : World Health Organization (WHO) .
- Bernstein , D. M. and Hoskins , J. A. 2006 . The health effects of chrysotile: Current perspective based upon recent data . Regul. Toxicol. Pharmacol , 45 : 252 – 264 .
- Bernstein , D. M. , Mast , R. , Anderson , R. , Hesterberg , T. W. , Musselman , R. , Kamstrup , O. and Hadley , J. 1994 . An experimental approach to the evaluation of the biopersistence of respirable synthetic fibers and minerals . Environ. Health Perspect , 102 ( suppl. 5 ) : 15 – 18 .
- Bernstein , D. M. , Morscheidt , C. , Grimm , H. G. , Thévenaz , P. and Teichert , U. 1996 . Evaluation of soluble fibers using the inhalation biopersistence model, a nine-fiber comparison . Inhal. Toxicol , 8 : 345 – 385 .
- Bernstein , D. M. and Riego-Sintes , J. M. R. 1999 . Methods for the determination of the hazardous properties for human health of man-made mineral fibers (MMMF).: European Commission Joint Research Centre, Institute for Health and Consumer Protection, Unit: Toxicology and Chemical Substances, European Chemicals Bureau , Ispra, , Italy : In Bernstein & Riego-Sintes . 1999
- Bernstein , D. M. , Riego Sintes , J. M. , Ersboell , B. K. and Kunert , J. 2001a . Biopersistence of synthetic mineral fibers as a predictor of chronic inhalation toxicity in rats . Inhal. Toxicol , 13 : 823 – 849 .
- Bernstein , D. M. , Riego Sintes , J. M. , Ersboell , B. K. and Kunert , J. 2001b . Biopersistence of synthetic mineral fibers as a predictor of chronic intraperitoneal injection tumor response in rats . Inhal. Toxicol , 13 : 851 – 875 .
- Bernstein , D. M. , Rogers , R. and Smith , P. 2003b . The biopersistence of Canadian chrysotile asbestos following inhalation . Inhal. Toxicol , 15 : 1247 – 1274 .
- Bernstein , D. M. , Rogers , R. and Smith , P. 2004 . The biopersistence of Brazilian chrysotile asbestos following inhalation . Inhal. Toxicol , 16 : 745 – 761 .
- Bernstein , D. M. , Rogers , R. , Smith , P. and Chevalier , J. 2006 . The toxicological response of Brazilian chrysotile asbestos: A multidose subchronic 90-day inhalation toxicology study with 92-day recovery to assess cellular and pathological response . Inhal. Toxicol , 18 : 313 – 332 .
- Bernstein , D. M. , Rogers , R. A. , Sepulveda , R. , Donaldson , K. , Schuler , D. , Gaering , S. , Kunzendorf , P. , Chevalier , J. and Holm , S. E. 2010 . The pathological response and fate in the lung and pleura of chrysotile in combination with fine particles compared to amosite asbestos following short-term inhalation exposure: Interim results . Inhal. Toxicol , 22 : 937 – 962 .
- BeruBe , K. A. , Quinlan , T. R. , Moulton , G. , Hemenway , D. , O'Shaughnessy , P. , Vacek , P. and Mossman , B. T. 1996 . Comparative proliferative and histopathologic changes in rat lungs after inhalation of chrysotile or crocidolite asbestos . Toxicol. Appl. Pharmacol , 137 : 67 – 74 .
- Blot , W. J. and Fraumeni , J. F. Jr . 1996 . “ Cancers of the lung and pleura ” . In Cancer epidemiology and prevention , Edited by: Schottenfeld , D. and Fraumeni , J. F. Vol. 2 , 637 – 665 . New York : Oxford University Press .
- Bolton , R. E. , Vincent , J. H. , Jones , A. D. , Addison , J. and Beckett , S. T. 1983 . An overload hypothesis for pulmonary clearance of UICC amosite fibres inhaled by rats . Br. J. Ind. Med , 40 : 264 – 272 .
- Bonner , J. C. 2004 . Regulation of PDGF and its receptors in fibrotic diseases . Cytokine Growth Factor Rev , 15 : 255 – 273 .
- Bonner , J. C. 2007 . Lung fibrotic responses to particle exposure . Toxicol. Pathol , 35 : 148 – 153 .
- Bonner , J. C. and Brody , A. R. 1995 . Cytokine-binding proteins in the lung . Am. J. Physiol , 268 : L869 – L878 .
- Boutin , C. , Dumortier , P. , Rey , F. , Viallat , J. R. and De Vuyst , P. 1996 . Black spots concentrate oncogenic asbestos fibers in the parietal pleura. Thoracoscopic and mineralogic study . Am. J. Respir. Crit. Care Med , 153 : 444 – 449 .
- Brass , D. M. , Hoyle , G. W. , Poovey , H. G. , Liu , J. Y. and Brody , A. R. 1999 . Reduced tumor necrosis factor-alpha and transforming growth factor-beta1 expression in the lungs of inbred mice that fail to develop fibroproliferative lesions consequent to asbestos exposure . Am. J. Pathol , 154 : 853 – 862 .
- Briant , J. K. 1988 . Distinction of reversible and irreversible convection by high-frequency ventilation of a respiratory airway cast , New York : PhD thesis, New York University .
- Bringardner , B. D. , Baran , C. P. , Eubank , T. D. and Marsh , C. B. 2008 . The role of inflammation in the pathogenesis of idiopathic pulmonary fibrosis . Antioxid. Redox Signal , 10 : 287 – 301 .
- Broaddus , V. C. , Everitt , J. I. , Black , B. and Kane , A. B. 2011 . Nonneoplastic and neoplastic pleural endpoints following fiber exposure . J. Toxicol. Environ. Health B , 14 : 153 – 178 .
- Brody , A. R. 1993 . Asbestos-induced lung disease . Environ. Health Perspect , 100 : 21 – 30 .
- Brody , A. R. , Hill , L. H. , Adkins , B. Jr. and O'Connor , R. W. 1981 . Chrysotile asbestos inhalation in rats: Deposition pattern and reaction of alveolar epithelium and pulmonary macrophages . Am. Rev. Respir. Dis , 123 : 670 – 679 .
- Brody , A. R. , Liu , J. Y. , Brass , D. and Corti , M. 1997 . Analyzing the genes and peptide growth factors expressed in lung cells in vivo consequent to asbestos exposure and in vitro . Environ. Health Perspect , 105 ( suppl. 5 ) : 1165 – 1171 .
- Brody , A. R. and Roe , M. W. 1983 . Deposition pattern of inorganic particles at the alveolar level in the lungs of rats and mice . Am. Rev. Respir. Dis , 128 : 724 – 729 .
- Buder-Hoffmann , S. A. , Shukla , A. , Barrett , T. F. , MacPherson , M. B. , Lounsbury , K. M. and Mossman , B. T. 2009 . A protein kinase Cdelta-dependent protein kinase D pathway modulates ERK1/2 and JNK1/2 phosphorylation and Bim-associated apoptosis by asbestos . Am. J. Pathol , 174 : 449 – 459 .
- Case , B. W. , Abraham , J. , Meeker , G. D., P. F. and Pinkerton , K. E. 2011 . Applying definitions of “asbestos” to environmental and “low-dose” exposure levels and health effects, particularly malignant mesothelioma . J. Toxicol. Environ. Health B , 14 : 3 – 39 .
- Castranova , V. 2003 . Mechanisms of fiber-induced lung disease , Chicago : U.S. EPA Asbestos Mechanisms of Toxicity Workshop .
- Chan , T. L. , Lee , P. S. and Hering , W. E. 1984 . Pulmonary retention of inhaled diesel particles after prolonged exposures to diesel exhaust . Fundam. Appl. Toxicol , 4 : 624 – 631 .
- Chang , L. Y. , Overby , L. H. , Brody , A. R. and Crapo , J. D. 1988 . Progressive lung cell reactions and extracellular matrix production after a brief exposure to asbestos . Am. J. Pathol , 131 : 156 – 170 .
- Cheng , Y. S. 1986 . Bivariate lognormal distribution for characterizing asbestos fiber aerosols . Aerosol Sci. Technol , 5 : 359 – 368 .
- Chow , S. , Campbell , C. , Sandrini , A. , Thomas , P. S. , Johnson , A. R. and Yates , D. H. 2009 . Exhaled breath condensate biomarkers in asbestos-related lung disorders . Respir. Med , 103 : 1091 – 1097 .
- Christensen , B. C. , Godleski , J. J. , Roelofs , C. R. , Longacker , J. L. , Bueno , R. , Sugarbaker , D. J. , Marsit , C. J. , Nelson , H. H. and Kelsey , K. T. 2008 . Asbestos burden predicts survival in pleural mesothelioma . Environ. Health Perspect , 116 : 723 – 726 .
- Christensen , B. C. , Houseman , E. A. , Godleski , J. J. , Marsit , C. J. , Longacker , J. L. , Roelofs , C. R. , Karagas , M. R. , Wrensch , M. R. , Yeh , R. F. , Nelson , H. H. , Wiemels , J. L. , Zheng , S. , Wiencke , J. K. , Bueno , R. , Sugarbaker , D. J. and Kelsey , K. T. 2009 . Epigenetic profiles distinguish pleural mesothelioma from normal pleura and predict lung asbestos burden and clinical outcome . Cancer Res , 69 : 227 – 234 .
- Churg , A. 1994 . Deposition and clearance of chrysotile asbestos . Ann. Occup. Hyg , 38 : 625 – 633 . 424–625
- Coin , P. G. , Roggli , V. L. and Brody , A. R. 1992 . Deposition, clearance, and translocation of chrysotile asbestos from peripheral and central regions of the rat lung . Environ. Res , 58 : 97 – 116 .
- Cole , R. W. , Ault , J. G. , Hayden , J. H. and Rieder , C. L. 1991 . Crocidolite asbestos fibers undergo size-dependent microtubule-mediated transport after endocytosis in vertebrate lung epithelial cells . Cancer Res , 51 : 4942 – 4947 .
- Cummins , A. B. , Palmer , C. , Mossman , B. T. and Taatjes , D. J. 2003 . Persistent localization of activated extracellular signal-regulated kinases (ERK1/2) is epithelial cell-specific in an inhalation model of asbestosis . Am. J. Pathol , 162 : 713 – 720 .
- Dammann , R. , Strunnikova , M. , Schagdarsurengin , U. , Rastetter , M. , Papritz , M. , Hattenhorst , U. E. , Hofmann , H. S. , Silber , R. E. , Burdach , S. and Hansen , G. 2005 . CpG island methylation and expression of tumour-associated genes in lung carcinoma . Eur. J. Cancer , 41 : 1223 – 1236 .
- Davies , R. , Griffiths , D. M. , Johnson , N. F. , Preece , A. W. and Livingston , D. C. 1984 . The cytotoxicity of kaolin towards macrophages in vitro . Br. J. Exp. Pathol , 65 : 453 – 466 .
- Davis , J. M. , Beckett , S. T. , Bolton , R. E. , Collings , P. and Middleton , A. P. 1978 . Mass and number of fibres in the pathogenesis of asbestos-related lung disease in rats . Br. J. Cancer , 37 : 673 – 688 .
- Davis , J. M. and Cowie , H. A. 1990 . The relationship between fibrosis and cancer in experimental animals exposed to asbestos and other fibers . Environ. Health Perspect , 88 : 305 – 309 .
- Davis , J. M. G. 1976 . “ Pathological aspects of the injection of glass fiber into the pleural and peritoneal cavities of rats and mice ” . In Occupational exposure to fibrous glass , 141 – 199 . Washington, DC : U.S. Department of Health, Education, and Welfare, (DHEW) Publ. No. 76–151, National Institute for Occupational Safety and Health (NIOSH) .
- Denissenko , M. F. , Pao , A. , Tang , M. and Pfeifer , G. P. 1996 . Preferential formation of benzo[a]pyrene adducts at lung cancer mutational hotspots in P53 . Science , 274 : 430 – 432 .
- Devlin , R. Toxicology testing in the 21st century: Role of in vitro systems . Paper read at Health Effects Institute Annual Conference . May 3–5 , Portland, OR.
- Dodson , R. F. , Williams , M. G. Jr. , Corn , C. J. , Brollo , A. and Bianchi , C. 1990 . Asbestos content of lung tissue, lymph nodes, and pleural plaques from former shipyard workers . Am. Rev. Respir. Dis , 142 : 843 – 847 .
- Donaldson , K. , Borm , P. J. , Castranova , V. and Gulumian , M. 2009 . The limits of testing particle-mediated oxidative stress in vitro in predicting diverse pathologies; Relevance for testing of nanoparticles . Part. Fibre Toxicol , 6 : 13
- Dostert , C. , Petrilli , V. , Van Bruggen , R. , Steele , C. , Mossman , B. T. and Tschopp , J. 2008 . Innate immune activation through Nalp3 inflammasome sensing of asbestos and silica . Science , 320 : 674 – 677 .
- Eastes , W. and Hadiey , J. G. 1995 . Dissolution of fibers inhaled by rats . Inhal. Toxicol , 7 : 179 – 196 .
- Eastes , W. and Hadley , J. G. 1996 . A mathematical model of fiber carcinogenicity and fibrosis in inhalation and intraperitoneal experiments in rats . Inhal. Toxicol , 8 : 323 – 343 .
- Eastman , A. , Mossman , B. T. and Bresnick , E. 1983 . Influence of asbestos on the uptake of benzo(a)pyrene and DNA alkylation in hamster tracheal epithelial cells . Cancer Res , 43 : 1251 – 1255 .
- Elespuru , R. K. , Agarwal , R. , Atrakchi , A. H. , Bigger , C. A. , Heflich , R. H. , Jagannath , D. R. , Levy , D. D. , Moore , M. M. , Ouyang , Y. , Robison , T. W. , Sotomayor , R. E. , Cimino , M. C. and Dearfield , K. L. 2009 . Current and future application of genetic toxicity assays: The role and value of in vitro mammalian assays . Toxicol. Sci , 109 : 172 – 179 .
- EUR (European Commission). 1997. Adapting to technical progress for the 23rd time Council Directive 67/548/EEC on the approximation of the laws regulations and administrative provisions relating to the classification, packaging and labeling of dangerous substances: European Commission.
- Ferin , J. 1972 . Observations concerning alveolar dust clearance . Ann. NY Acad. Sci , 200 : 66 – 72 .
- Ferin , J. and Leach , L. J. 1976 . The effect of amostie and chrysotile asbestos on the clearance of TiO2 particles from the lung . Environ Res , 12 : 250 – 254 .
- Förster , H. 1984 . “ The behaviour of mineral fibres in physiological solutions ” . In Biological effects of man-made mineral fibers , 27 – 59 . Copenhagen : World Health Organization (WHO) .
- Gaut , J. P. , Byun , J. , Tran , H. D. , Lauber , W. M. , Carroll , J. A. , Hotchkiss , R. S. , Belaaouaj , A. and Heinecke , J. W. 2002 . Myeloperoxidase produces nitrating oxidants in vivo . J. Clin. Invest , 109 : 1311 – 1319 .
- Gentry , J. W. , Spumy , K. R. , Sehormann , J. and Opiela , H. 1983 . “ Measurement of the diffusion coefficient of asbestos fibers ” . In Aerosols in the mining and industrial work environments , Edited by: Marple , V. A. and Liu , B. Y. H. Vol. 2 , 593 – 612 . Ann Arbor, MI : Ann Arbor Science .
- Ghio , A. , Tan , R. J. , Ghio , K. , Fattman , C. L. and Oury , T. D. 2009 . Iron accumulation and expression of iron-related proteins following murine exposure to crocidolite . J. Environ. Pathol. Toxicol. Oncol , 28 : 153 – 162 .
- Ghio , A. J. , Stonehuerner , J. , Richards , J. and Devlin , R. B. 2008 . Iron homeostasis in the lung following asbestos exposure . Antioxid. Redox Signal , 10 : 371 – 377 .
- Goodglick , L. A. and Kane , A. B. 1990 . Cytotoxicity of long and short crocidolite asbestos fibers in vitro and in vivo . Cancer Res , 50 : 5153 – 5163 .
- Griffis , L. C. , Henderson , T. R. and Pickrell , J. A. 1981 . A method for determining glass in rat lung after exposure to a glass fiber aerosol . Am. Ind. Hyg. Assoc. J , 42 : 566 – 569 .
- Gurman , J. L. , Lippmann , M. and Schlesinger , R. B. 1984a . Particle deposition in replicate casts of the human upper tracheobronchial tree under constant and cyclic inspiratory flow. I. Experimental . Aerosol Sci. Technol , 3 : 245 – 252 .
- Gurman , J. L. , Lioy , P. J. , Lippmann , M. and Schlesinger , R. B. 1984b . Particle deposition in replicate casts of the human upper tracheobronchial tree under constant and cyclic inspiratory flow. II. Empirical model . Aerosol Sci. Technol , 3 : 253 – 258 .
- Gurman , J. L. , Schlesinger , R. B. and Lippmann , M. 1980 . A variable-opening mechanical larynx for use in aerosol deposition studies . Am. Ind. Hyg. Assoc. J , 41 : 678 – 680 .
- Haegens , A. , van der Vliet , A. , Butnor , K. J. , Heintz , N. , Taatjes , D. , Hemenway , D. , Vacek , P. , Freeman , B. A. , Hazen , S. L. , Brennan , M. L. and Mossman , B. T. 2005 . Asbestos-induced lung inflammation and epithelial cell proliferation are altered in myeloperoxidase-null mice . Cancer Res , 65 : 9670 – 9677 .
- Hammad , Y. Y. 1984 . “ Deposition and elimination of MMMF ” . In Biological effects of man-made mineral fibers , 126 – 142 . Copenhagen : World Health Organization (WHO) .
- Hardie , W. D. , Le Cras , T. D. , Jiang , K. , Tichelaar , J. W. , Azhar , M. and Korfhagen , T. R. 2004 . Conditional expression of transforming growth factor-alpha in adult mouse lung causes pulmonary fibrosis . Am. J. Physiol. Lung Cell Mol Physiol , 286 : L741 – L749 .
- Hart , G. A. , Kathman , L. M. and Hesterberg , T. W. 1994 . In vitro cytotoxicity of asbestos and man-made vitreous fibers: Roles of fiber length, diameter and composition . Carcinogenesis , 15 : 971 – 977 .
- Hartung , T. 2010 . Lessons learned from alternative methods and their validation for a new toxicology in the 21st century . J. Toxicol. Environ. Health B , 13 : 277 – 290 .
- Haus , B. M. , Razavi , H. and Kuschner , W. G. 2001 . Occupational and environmental causes of bronchogenic carcinoma . Curr Opin Pulmonary Med , 7 : 220 – 225 .
- Health Effects Institute . 1991 . Asbestos in public and commercial buildings: A literature review and synthesis of current knowledge , Cambridge, MA : Health Effects Institute-Asbestos Research .
- Hei , T. K. June 12–13 2003 . “ Genetic effects of asbestos fibers ” . In Asbestos Mechanisms of Toxicity Workshop , June 12–13 , Chicago : U.S. Environmental Protection Agency .
- Heintz , N. H. , Janssen-Heininger , Y. M. and Mossman , B. T. 2010 . Asbestos, lung cancers, and mesotheliomas: From molecular approaches to targeting tumor survival pathways . Am. J. Respir. Cell Mol. Biol , 42 : 133 – 139 .
- Heintz , N. H. , Janssen , Y. M. and Mossman , B. T. 1993 . Persistent induction of c-fos and c-jun expression by asbestos . Proc. Natl. Acad. Sci. USA , 90 : 3299 – 3303 .
- Hesterberg , T. W. 2009 . “ Comments on NIOSH asbestos roadmap—Animal bioassays ” . In Workshop on the NIOSH Research Roadmap on Asbestos Fibers and Other Elongated Mineral Particles , Washington, DC : National Institute of Occupational Safety and Health .
- Hesterberg , T. W. and Hart , G. A. 2000 . Lung biopersistence and in vitro dissolution rate predict the pathogenic potential of synthetic vitreous fibers . Inhal. Toxicol , 12 : 91 – 97 .
- Hesterberg , T. W. and Hart , G. A. 2001 . Synthetic vitreous fibers: A review of toxicology research and its impact on hazard classification . Crit. Rev. Toxicol , 31 : 1 – 53 .
- Hesterberg , T. W. , McConnell , E. E. , Miller , W. C. , Hamilton , R. and Bunn , W. B. 1992 . Pulmonary toxicity of inhaled polypropylene fibers in rats . Fundam. Appl. Toxicol , 19 : 358 – 366 .
- Hesterberg , T. W. , Miiller , W. C. , Thevenaz , P. and Anderson , R. 1995 . Chronic inhalation studies of man-made vitreous fibres: Characterization of fibres in the exposure aerosol and lungs . Ann Occup Hyg , 39 : 637 – 653 .
- Hesterberg , T. W. , Chase , G. , Axten , C. , Miller , W. C. , Musselman , R. P. , Kamstrup , O. , Hadley , J. , Morscheidt , C. , Bernstein , D. M. and Thevenaz , P. 1998a . Biopersistence of synthetic vitreous fibers and amosite asbestos in the rat lung following inhalation . Toxicol. Appl. Pharmacol , 151 : 262 – 275 .
- Hesterberg , T. W. , Hart , G. A. , Chevalier , J. , Miiller , W. C. , Hamilton , R. D. , Bauer , J. and Thevenaz , P. 1998b . The importance of fiber biopersistence and lung dose in determining the chronic inhalation effects of X607, RCF1, and chrysotile asbestos in rats . Toxicol. Appl. Pharmacol , 153 : 68 – 82 .
- Hesterberg , T. W. , Axten , C. , McConnell , E. E. , Hart , G. A. , Miiller , W. , Chevalier , J. , Everitt , J. , Thevenaz , P. and Oberdorster , G. 1999 . Studies on the inhalation toxicology of two fiberglasses and amosite asbestos in the Syrian golden hamster. Part I. Results of a subchronic study and dose selection for a chronic study . Inhal. Toxicol , 11 : 747 – 784 .
- Holsapple , M. P. , Afshari , C. A. and Lehman-McKeeman , L. D. 2009 . Forum series: The “vision” for toxicity testing in the 21st century: Promises and conundrums . Toxicol. Sci , 107 : 307 – 308 .
- Holt , P. F. 1982 . Translocation of asbestos dust through the bronchiolar wall . Environ. Res , 27 : 255 – 260 .
- Hordijk , P. L. 2006 . Regulation of NADPH oxidases: the role of Rac proteins . Circ Res , 98 : 453 – 462 .
- Hoyle , G. W. and Brody , A. R. 1996 . Gene expression in rodent model of environmental lung disease . Ann. NY Acad. Sci , 796 : 162 – 172 .
- Huang , S. X. L. , Jaurand , M. C. , Kamp , D. W. , Whysner , J. and Hei , T. K. 2011 . Role of mutagenicity in mineral fiber-induced carcinogenicity and other diseases . J. Toxicol. Environ. Health B , 14 : 179 – 245 .
- Husgafvel-Pursiainen , K. , Hackman , P. , Ridanpaa , M. , Anttila , S. , Karjalainen , A. , Partanen , T. , Taikina-Aho , O. , Heikkila , L. and Vainio , H. 1993 . K-ras mutations in human adenocarcinoma of the lung: Association with smoking and occupational exposure to asbestos . Int. J. Cancer , 53 : 250 – 256 .
- Husgafvel-Pursiainen , K. , Karjalainen , A. , Kannio , A. , Anttila , S. , Partanen , T. , Ojajarvi , A. and Vainio , H. 1999 . Lung cancer and past occupational exposure to asbestos. Role of p53 and K-ras mutations . Am. J. Respir. Cell Mol. Biol , 20 : 667 – 674 .
- Husgafvel-Pursiainen , K. , Ridanpaa , M. , Anttila , S. and Vainio , H. 1995 . p53 and ras gene mutations in lung cancer: Implications for smoking and occupational exposures . J. Occup. Environ. Med , 37 : 69 – 76 .
- ILSI . 2000 . The relevance of the rat lung response to particle overload for human risk assessment: A workshop consensus report. ILSI Risk Science Institute workshop participants . Inhal. Toxicol , 12 : 1 – 17 .
- Ingram , J. L. and Bonner , J. C. 2006 . EGF and PDGF receptor tyrosine kinases as therapeutic targets for chronic lung diseases . Curr. Mol. Med , 6 : 409 – 421 .
- Institute on Medicine . 2008 . Committee on Asbestos: Selected health effects , Washington, D.C : Board on Population Health and Health Practices, Institute of Medicine of the National Academies (IOM) .
- Janssen , Y. M. , Driscoll , K. E. , Howard , B. , Quinlan , T. R. , Treadwell , M. , Barchowsky , A. and Mossman , B. T. 1997 . Asbestos causes translocation of p65 protein and increases NF-kappa B DNA binding activity in rat lung epithelial and pleural mesothelial cells . Am. J. Pathol , 151 : 389 – 401 .
- Jensen , C. G. , Jensen , L. C. , Rieder , C. L. , Cole , R. W. and Ault , J. G. 1996 . Long crocidolite asbestos fibers cause polyploidy by sterically blocking cytokinesis . Carcinogenesis , 17 : 2013 – 2021 .
- Jones , A. D. , Johnston , A. M. and Vincent , J. H. 1983 . “ Static electrification of airborne asbestos dust ” . In Aerosols in the mining and industrial work environments: Characterization , Edited by: Marple , V. A. and Liu , B. Y. H. Vol. 2 , 613 – 622 . Ann Arbor, MI : Ann Arbor Science .
- Jones , A. D. , McMillan , C. H. , Johnston , A. M. , McIntosh , C. , Cowie , H. , Bolton , R. E. , Borzucki , G. and Vincent , J. H. 1988 . Pulmonary clearance of UICC amosite fibres inhaled by rats during chronic exposure at low concentration . Br. J. Ind. Med , 45 : 300 – 304 .
- Kamp , D. W. , Israbian , V. A. , Preusen , S. E. , Zhang , C. X. and Weitzman , S. A. 1995 . Asbestos causes DNA strand breaks in cultured pulmonary epithelial cells: role of iron-catalyzed free radicals . Am. J. Physiol , 268 : L471 – L480 .
- Kannerstein , M. and Churg , J. 1972 . Pathology of carcinoma of the lung associated with asbestos exposure . Cancer , 30 : 14 – 21 .
- Kettunen , E. , Aavikko , M. , Nymark , P. , Ruosaari , S. , Wikman , H. , Vanhala , E. , Salmenkivi , K. , Pirinen , R. , Karjalainen , A. , Kuosma , E. and Anttila , S. 2009 . DNA copy number loss and allelic imbalance at 2p16 in lung cancer associated with asbestos exposure . Br. J. Cancer , 100 : 1336 – 1342 .
- Kim , D. H. , Nelson , H. H. , Wiencke , J. K. , Zheng , S. , Christiani , D. C. , Wain , J. C. , Mark , E. J. and Kelsey , K. T. 2001 . p16(INK4a) and histology-specific methylation of CpG islands by exposure to tobacco smoke in non-small cell lung cancer . Cancer Res , 61 : 3419 – 3424 .
- Klingholz , R. and Steinkopf , B. 1982 . Das Verhalten von künstlichen Mineralfasern in einer physiologischen Modellflüssigkeit und in Wasser . Staub-Reinhalt Luft , 42 : 69 – 76 .
- Klingholz , R. and Steinkopf , B. 1984 . “ The reactions of MMMF in a physiological model fluid and in water ” . In Biological effects of man-made mineral fibers , Vol. 2 , 60 – 86 . Copenhagen : World Health Organization (WHO) .
- Kodama , Y. , Boreiko , C. J. , Maness , S. C. and Hesterberg , T. W. 1993 . Cytotoxic and cytogenetic effects of asbestos on human bronchial epithelial cells in culture . Carcinogenesis , 14 : 691 – 697 .
- Kraunz , K. S. , Nelson , H. H. , Lemos , M. , Godleski , J. J. , Wiencke , J. K. and Kelsey , K. T. 2006 . Homozygous deletion of p16INK4a and tobacco carcinogen exposure in nonsmall cell lung cancer . Int. J. Cancer , 118 : 1364 – 1369 .
- Krewski , D. , Acosta , D. , Andersen , M. , Anderson , H. , Bialar , J. C. , Boekelheide , K. , Brent , R. , Charnley , G. , Cheung , V. G. , Green , S. , Kelsey , K. T. , Kerkvliet , N. I. , Li , A. A. , L , McCray. , Meyer , O. , Patterson , R. D. , Pennie , W. , Scala , R. A. , Solomon , G. M , Stephens , M. , Yager , J. and Zeiss , L. 2010 . Toxicity testing in the 21st century: A vision and strategy . J. Toxicol. Environ. Health B , 13 ( 51–138 )
- Lasky , J. A. , Tonthat , B. , Liu , J. Y. , Friedman , M. and Brody , A. R. 1998 . Upregulation of the PDGF-alpha receptor precedes asbestos-induced lung fibrosis in rats . Am. J. Respir. Crit. Care Med , 157 : 1652 – 1657 .
- Le Bouffant , L. , Henin , J. P. , Martin , J. C. , Normand , C. , Tichoux , G. and Trolard , F. 1984 . “ Distribution of inhaled MMMF in the rat lung—Long-term effects ” . In Biological effects of mineral fibres , Vol. 2 , 143 – 168 . Copenhagen : World Health Organization (WHO) .
- Lee , K. P. , Kelly , D. P. and Kennedy , G. L. Jr. 1983 . Pulmonary response to inhaled Kevlar aramid synthetic fibers in rats . Toxicol. Appl. Pharmacol , 71 : 242 – 253 .
- Lentz , T. J. , Rice , C. H. , Succop , P. A. , Lockey , J. E. , Dement , J. M. and LeMasters , G. K. 2003 . Pulmonary deposition modeling with airborne fiber exposure data: a study of workers manufacturing refractory ceramic fibers . Appl. Occup. Environ. Hyg , 18 : 278 – 288 .
- Lippmann , M. 1988 . Asbestos exposure indices . Environ. Res , 46 : 86 – 106 .
- Lippmann , M. 1990 . Effects of fiber characteristics on lung deposition, retention, and disease . Environ. Health Perspect , 88 : 311 – 317 .
- Lippmann , M. and Schlesinger , R. B. 1984 . Interspecies comparisons of particle deposition and mucociliary clearance in tracheobronchial airways . J. Toxicol. Environ. Health , 13 : 441 – 469 .
- Liu , B. C. , Fu , D. C. , Miao , Q. , Wang , H. H. and You , B. R. 1998a . p53 gene mutations in asbestos associated cancers . Biomed. Environ. Sci , 11 : 226 – 232 .
- Liu , G. , Beri , R. , Mueller , A. and Kamp , D. W. 2010 . Molecular mechanisms of asbestos-induced lung epithelial cell apoptosis . Chem. Biol. Interact , 188 : 309 – 318 .
- Liu , J. Y. , Brass , D. M. , Hoyle , G. W. and Brody , A. R. 1998b . TNF-alpha receptor knockout mice are protected from the fibroproliferative effects of inhaled asbestos fibers . Am. J. Pathol , 153 : 1839 – 1847 .
- Liu , J. Y. and Brody , A. R. 2001 . Increased TGF-beta1 in the lungs of asbestos-exposed rats and mice: reduced expression in TNF-alpha receptor knockout mice . J. Environ. Pathol. Toxicol. Oncol , 20 : 97 – 108 .
- Liu , J. Y. , Morris , G. F. , Lei , W. H. , Hart , C. E. , Lasky , J. A. and Brody , A. R. 1997 . Rapid activation of PDGF-A and -B expression at sites of lung injury in asbestos-exposed rats . Am. J. Respir. Cell Mol. Biol , 17 : 129 – 140 .
- Manning , C. B. , Cummins , A. B. , Jung , M. W. , Berlanger , I. , Timblin , C. R. , Palmer , C. , Taatjes , D. J. , Hemenway , D. , Vacek , P. and Mossman , B. T. 2002 . A mutant epidermal growth factor receptor targeted to lung epithelium inhibits asbestos-induced proliferation and proto-oncogene expression . Cancer Res , 62 : 4169 – 4175 .
- Manning , C. B. , Sabo-Attwood , T. , Robledo , R. F. , Macpherson , M. B. , Rincon , M. , Vacek , P. , Hemenway , D. , Taatjes , D. J. , Lee , P. J. and Mossman , B. T. 2008 . Targeting the MEK1 cascade in lung epithelium inhibits proliferation and fibrogenesis by asbestos . Am. J. Respir. Cell Mol. Biol , 38 : 618 – 626 .
- Marsit , C. J. , Hasegawa , M. , Hirao , T. , Kim , D. H. , Aldape , K. , Hinds , P. W. , Wiencke , J. K. , Nelson , H. H. and Kelsey , K. T. 2004 . Loss of heterozygosity of chromosome 3p21 is associated with mutant TP53 and better patient survival in non-small-cell lung cancer . Cancer Res , 64 : 8702 – 8707 .
- Mast , R. W. , Hesterberg , T. W. , Glass , L. R. , McConnell , E. E. , Anderson , R. and Bernstein , D. M. 1994 . Chronic inhalation and biopersistence of refractory ceramic fiber in rats and hamsters . Environ. Health Perspect , 102 ( suppl. 5 ) : 207 – 209 .
- Mast , R. W. , McConnell , E. E. , Anderson , R. , Chevalier , J. , Kotin , P. , Bernstein , D. M. , Thevenaz , P. , Glass , L. R. , Miiller , W. C. and Hesterberg , T. W. 1995 . Studies on the chronic toxicity (inhalation) of four types of refractory ceramic fiber in male Fischer 344 rats . Inhal. Toxicol , 7 : 425 – 467 .
- Mattson , S. M. 1994 . Glass fiber dissolution in simulated lung fluid and measures needed to improve consistency and correspondence to in vivo dissolution . Environ. Health Perspect , 102 ( suppl. 5 ) : 87 – 90 .
- McClellan , R. O. , Miller , F. J. , Hesterberg , T. W. , Warheit , D. B. , Bunn , W. B. , Kane , A. B. , Lippmann , M. , Mast , R. W. , McConnell , E. E. and Reinhardt , C. F. 1992 . Approaches to evaluating the toxicity and carcinogenicity of man-made fibers: summary of a workshop held November 11–13, 1991, Durham, North Carolina . Regul. Toxicol. Pharmacol , 16 : 321 – 364 .
- McConnell , E. E. , Axten , C. , Hesterberg , T. W. , Chevalier , J. , Miiller , W. C. , Everitt , J. , Oberdorster , G. , Chase , G. R. , Thevenaz , P. and Kotin , P. 1999 . Studies on the inhalation toxicology of two fiberglasses and amosite asbestos in the Syrian golden hamster. Part II. Results of chronic exposure . Inhal. Toxicol , 11 : 785 – 835 .
- McConnell , J. E. , Armstrong , J. F. , Hodges , P. E. and Bard , J. B. 1995 . The mouse 14-3-3 epsilon isoform, a kinase regulator whose expression pattern is modulated in mesenchyme and neuronal differentiation . Dev. Biol , 169 : 218 – 228 .
- McDonald , J. C. and McDonald , A. D. 1987 . “ Epidemiology of asbestos-related lung cancer ” . In Asbestos-related malignancy , Edited by: Antman , K. and Aisner , J. 57 – 79 . Orlando, FL : Grune and Stratton .
- Miller , B. G. , Jones , A. D. , Searl , A. , Buchanan , D. , Cullen , R. T. , Soutar , C. A. , Davis , J. M. and Donaldson , K. 1999 . Influence of characteristics of inhaled fibres on development of tumours in the rat lung . Ann. Occup. Hyg , 43 : 167 – 179 .
- Mishra , A. , Liu , J. Y. , Brody , A. R. and Morris , G. F. 1997 . Inhaled asbestos fibers induce p53 expression in the rat lung . Am. J. Respir. Cell Mol. Biol , 16 : 479 – 485 .
- Monchaux , G. , Bignon , J. , Jaurand , M. C. , Lafuma , J. , Sebastien , P. , Masse , R. , Hirsch , A. and Goni , J. 1981 . Mesotheliomas in rats following inoculation with acid-leached chrysotile asbestos and other mineral fibres . Carcinogenesis , 2 : 229 – 236 .
- Morgan , A. , Black , A. , Evans , N. , Holmes , A. and Pritchard , J. N. 1980 . Deposition of sized glass fibres in the respiratory tract of the rat . Ann. Occup. Hyg , 23 : 353 – 366 .
- Morgan , A. and Holmes , A. 1984 . “ The deposition of MMMF in the respiratory tract of the rat, their subsequent clearance, solubility in vivo and protein coating ” . In Biological effects of man-made mineral fibers , Vol. 2 , 1 – 17 . Copenhagen : World Health Organization (WHO) .
- Morrow , P. E. 1988 . Possible mechanisms to explain dust overloading of the lungs . Fundam. Appl. Toxicol , 10 : 369 – 384 .
- Mossman , B. 2003 . “ Cell signaling and proliferation by asbestos ” . In Asbestos Mechanisms of Toxicity Workshop , Chicago : U.S. Environmental Protection Agency .
- Mossman , B. T. 1993 . Mechanisms of asbestos carcinogenesis and toxicity: the amphibole hypothesis revisited . Br. J. Ind. Med , 50 : 673 – 676 .
- Mossman , B. T. , Bignon , J. , Corn , M. , Seaton , A. and Gee , J. B. 1990 . Asbestos: Scientific developments and implications for public policy . Science , 247 : 294 – 301 .
- Mossman , B. T. , Borm , P. J. , Castranova , V. , Costa , D. L. , Donaldson , K. and Kleeberger , S. R. 2007 . Mechanisms of action of inhaled fibers, particles and nanoparticles in lung and cardiovascular diseases . Part. Fibre Toxicol , 4 : 4
- Mossman , B. T. , Cameron , G. S. and Yotti , L. P. 1985 . Cocarcinogenic and tumor promoting properties of asbestos and other minerals in tracheobronchial epithelium . Carcinogen. Compr. Surv , 8 : 217 – 238 .
- Mossman , B. T. and Churg , A. 1998 . Mechanisms in the pathogenesis of asbestosis and silicosis . Am. J. Respir. Crit. Care Med , 157 : 1666 – 1680 .
- Mossman , B. T. , Craighead , J. E. and MacPherson , B. V. 1980 . Asbestos-induced epithelial changes in organ cultures of hamster trachea: Inhibition by retinyl methyl ether . Science , 207 : 311 – 313 .
- Mossman , B. T. and Gee , J. B. 1989 . Asbestos-related diseases . N. Engl. J. Med , 320 : 1721 – 1730 .
- Mossman , B. T. , Lounsbury , K. M. and Reddy , S. P. 2006 . Oxidants and signaling by mitogen-activated protein kinases in lung epithelium . Am. J. Respir. Cell Mol. Biol , 34 : 666 – 669 .
- Mossman , B. T. and Sesko , A. M. 1990 . In vitro assays to predict the pathogenicity of mineral fibers . Toxicology , 60 : 53 – 61 .
- Muhle , H. , Bellman , B. and Heinrich , U. 1988 . Overloading of lung clearance during chronic exposure of experimental animals to particles . Ann. Occup. Hyg , 32 : 141 – 147 .
- Muhle , H. , Pott , F. , Bellmann , B. , Takenaka , S. and Ziem , U. 1987 . Inhalation and injection experiments in rats to test the carcinogenicity of MMMF . Ann. Occup. Hyg , 31 : 755 – 764 .
- Musselman , R. P. , Miiller , W. C. , Eastes , W. , Hadley , J. G. , Kamstrup , O. , Thevenaz , P. and Hesterberg , T. W. 1994 . Biopersistences of man-made vitreous fibers and crocidolite fibers in rat lungs following short-term exposures . Environ. Health Perspect , 102 ( suppl. 5 ) : 139 – 143 .
- Nelson , H. H. , Christiani , D. C. , Wiencke , J. K. , Mark , E. J. , Wain , J. C. and Kelsey , K. T. 1999 . k-ras mutation and occupational asbestos exposure in lung adenocarcinoma: Asbestos-related cancer without asbestosis . Cancer Res , 59 : 4570 – 4573 .
- Nelson , H. H. , Wiencke , J. K. , Gunn , L. , Wain , J. C. , Christiani , D. C. and Kelsey , K. T. 1998 . Chromosome 3p14 alterations in lung cancer: Evidence that FHIT exon deletion is a target of tobacco carcinogens and asbestos . Cancer Res , 58 : 1804 – 1807 .
- National Institute of Occupational Safety and Health . 2011 . Current intelligence bulletin 62. Asbestos fibers and other elongated mineral particles: State of the science and roadmap for research , Washington, DC : National Institute of Occupational Safety and Health .
- Nymark , P. , Kettunen , E. , Aavikko , M. , Ruosaari , S. , Kuosma , E. , Vanhala , E. , Salmenkivi , K. , Pirinen , R. , Karjalainen , A. , Knuutila , S. , Wikman , H. and Anttila , S. 2009 . Molecular alterations at 9q33.1 and polyploidy in asbestos-related lung cancer . Clin. Cancer Res , 15 : 468 – 475 .
- Nymark , P. , Wikman , H. , Hienonen-Kempas , T. and Anttila , S. 2008 . Molecular and genetic changes in asbestos-related lung cancer . Cancer Lett , 265 : 1 – 15 .
- Nymark , P. , Wikman , H. , Ruosaari , S. , Hollmen , J. , Vanhala , E. , Karjalainen , A. , Anttila , S. and Knuutila , S. 2006 . Identification of specific gene copy number changes in asbestos-related lung cancer . Cancer Res , 66 : 5737 – 5743 .
- Oberdorster , G. 1995a . Lung particle overload: Implications for occupational exposures to particles . Regul. Toxicol. Pharmacol , 21 : 123 – 135 .
- Oberdorster , G. 1995b . The NTP talc inhalation study: A critical appraisal focused on lung particle overload . Regul. Toxicol. Pharmacol , 21 : 233 – 241 .
- Oberdorster , G. 2000 . Determinants of the pathogenicity of man-made vitreous fibers (MMVF) . Int. Arch. Occup. Environ. Health , 73 ( suppl ) : S60 – S68 .
- Oberdorster , G. , Ferin , J. , Finkelstein , J. , Soderholm , S. and Gelein , R. 1990 . “ Mechanistic studies on particle-induced acute and chronic lung injury ” . In Aerosols: Science, industry, health and environment , Edited by: Masuda , S. and Takahashi , K. Vol. 2 , 1229 – 1233 . Oxford, , UK : Pergamon Press .
- Oberdorster , G. , Maynard , A. , Donaldson , K. , Castranova , V. , Fitzpatrick , J. , Ausman , K. , Carter , J. , Karn , B. , Kreyling , W. , Lai , D. , Olin , S. , Monteiro-Riviere , N. , Warheit , D. and Yang , H. 2005 . Principles for characterizing the potential human health effects from exposure to nanomaterials: Elements of a screening strategy . Part. Fibre Toxicol , 2 : 8
- Palekar , L. D. , Eyre , J. F. , Most , B. M. and Coffin , D. L. 1987 . Metaphase and anaphase analysis of V79 cells exposed to erionite, UICC chrysotile and UICC crocidolite . Carcinogenesis , 8 : 553 – 560 .
- Panduri , V. , Surapureddi , S. , Soberanes , S. , Weitzman , S. A. , Chandel , N. and Kamp , D. W. 2006 . P53 mediates amosite asbestos-induced alveolar epithelial cell mitochondria-regulated apoptosis . Am. J. Respir. Cell Mol. Biol , 34 : 443 – 452 .
- Perdue , T. D. and Brody , A. R. 1994 . Distribution of transforming growth factor-beta 1, fibronectin, and smooth muscle actin in asbestos-induced pulmonary fibrosis in rats . J. Histochem. Cytochem , 42 : 1061 – 1070 .
- Pfeifer , G. P. and Rauch , T. A. 2009 . DNA methylation patterns in lung carcinomas . Semin. Cancer Biol , 19 : 181 – 187 .
- Pinkerton , K. E. , Brody , A. R. , McLaurin , D. A. , Adkins , B. Jr. , O'Connor , R. W. , Pratt , P. C. and Crapo , J. D. 1983 . Characterization of three types of chrysotile asbestos after aerosolization . Environ. Res , 31 : 32 – 53 .
- Pociask , D. A. , Sime , P. J. and Brody , A. R. 2004 . Asbestos-derived reactive oxygen species activate TGF-beta1 . Lab. Invest , 84 : 1013 – 1023 .
- Pundsack , F. L. 1955 . The properties of asbestos. I. The colloidal and surface chemistry of chrysotile . J. Phys. Chem , 59 : 892 – 895 .
- Pylkkanen , L. , Wolff , H. , Stjernvall , T. , Tuominen , P. , Sioris , T. , Karjalainen , A. , Anttila , S. and Husgafvel-Pursiainen , K. 2002 . Reduced Fhit protein expression and loss of heterozygosity at FHIT gene in tumours from smoking and asbestos-exposed lung cancer patients . Int. J. Oncol , 20 : 285 – 290 .
- Quinlan , T. R. , BeruBe , K. A. , Hacker , M. P. , Taatjes , D. J. , Timblin , C. R. , Goldberg , J. , Kimberley , P. , O'Shaughnessy , P. , Hemenway , D. , Torino , J. , Jimenez , L. A. and Mossman , B. T. 1998 . Mechanisms of asbestos-induced nitric oxide production by rat alveolar macrophages in inhalation and in vitro models . Free Radical Biol Med , 24 : 778 – 788 .
- Quinlan , T. R. , BeruBe , K. A. , Marsh , J. P. , Janssen , Y. M. , Taishi , P. , Leslie , K. O. , Hemenway , D. , O'Shaughnessy , P. T. , Vacek , P. and Mossman , B. T. 1995 . Patterns of inflammation, cell proliferation, and related gene expression in lung after inhalation of chrysotile asbestos . Am. J. Pathol , 147 : 728 – 739 .
- Quinlan , T. R. , Marsh , J. P. , Janssen , Y. M. , Leslie , K. O. , Hemenway , D. , Vacek , P. and Mossman , B. T. 1994 . Dose-responsive increases in pulmonary fibrosis after inhalation of asbestos . Am. J. Respir. Crit. Care Med , 150 : 200 – 206 .
- Quinn , M. T. , Ammons , M. C. and Deleo , F. R. 2006 . The expanding role of NADPH oxidases in health and disease: no longer just agents of death and destruction . Clin. Sci. (Lond.) , 111 : 1 – 20 .
- Rice , A. B. , Moomaw , C. R. , Morgan , D. L. and Bonner , J. C. 1999 . Specific inhibitors of platelet-derived growth factor or epidermal growth factor receptor tyrosine kinase reduce pulmonary fibrosis in rats . Am. J. Pathol , 155 : 213 – 221 .
- Richardson , D. B. 2009 . Lung cancer in chrysotile asbestos workers: Analyses based on the two-stage clonal expansion model . Cancer Causes Control , 20 : 917 – 923 .
- Robledo , R. and Mossman , B. 1999 . Cellular and molecular mechanisms of asbestos-induced fibrosis . J. Cell Physiol , 180 : 158 – 166 .
- Robledo , R. F. , Buder-Hoffmann , S. A. , Cummins , A. B. , Walsh , E. S. , Taatjes , D. J. and Mossman , B. T. 2000 . Increased phosphorylated extracellular signal-regulated kinase immunoreactivity associated with proliferative and morphologic lung alterations after chrysotile asbestos inhalation in mice . Am. J. Pathol , 156 : 1307 – 1316 .
- Roggli , V. L. and Brody , A. R. 1984 . Changes in numbers and dimensions of chrysotile asbestos fibers in lungs of rats following short-term exposure . Exp. Lung Res , 7 : 133 – 147 .
- Roggli , V. L. , George , M. H. and Brody , A. R. 1987 . Clearance and dimensional changes of crocidolite asbestos fibers isolated from lungs of rats following short-term exposure . Environ. Res , 42 : 94 – 105 .
- Roggli , V. L. and Vollmer , R. T. 2008 . Twenty-five years of fiber analysis: What have we learned? . Hum. Pathol , 39 : 307 – 315 .
- Rom , W. N. 1998 . Assessment of activation, differentiation, and carcinogenesis of lung cells by quantitative competitive RT-PCR . Am. J. Respir. Cell Mol. Biol , 19 : 3 – 5 .
- Rothman , K. J. 1976 . The estimation of synergy or antagonism . Am. J. Epidemiol , 103 : 506 – 511 .
- Ruosaari , S. , Hienonen-Kempas , T. , Puustinen , A. , Sarhadi , V. K. , Hollmen , J. , Knuutila , S. , Saharinen , J. , Wikman , H. and Anttila , S. 2008 . Pathways affected by asbestos exposure in normal and tumour tissue of lung cancer patients . BMC Med. Genom , 1 : 55
- Russi , M. and Cone , J. 1994 . Textbook of clinical, occupational and environmental medicine , Edited by: Chan-Yeung , M. , Rosenstock , L. and Cullen , M. R. 543 – 554 . Philadelphia : W. B. Saunders .
- Sabo-Attwood , T. , Ramos-Nino , M. , Bond , J. , Butnor , K. J. , Heintz , N. , Gruber , A. D. , Steele , C. , Taatjes , D. J. , Vacek , P. and Mossman , B. T. 2005 . Gene expression profiles reveal increased mClca3 (Gob5) expression and mucin production in a murine model of asbestos-induced fibrogenesis . Am. J. Pathol , 167 : 1243 – 1256 .
- Saracci , R. 1987 . The interactions of tobacco smoking and other agents in cancer etiology . Epidemiol Rev , 9 : 175 – 193 .
- Schlesinger , R. B. , Concato , J. and Lippmann , M. 1983 . “ Particle deposition during exhalation: A study in replicate casts of the human upper tracheobronchial tree ” . In Aerosols in the mining and industrial work environments: Fundamentals and status , Edited by: Marple , V. A. and Liu , B. Y. H. Vol. 1 , 165 – 176 . Ann Arbor, MI : Ann Arbor Science .
- Schlesinger , R. B. and Lippmann , M. 1978 . Selective particle deposition and bronchogenic carcinoma . Environ. Res , 15 : 424 – 431 .
- Scholze , H. and Conradt , R. 1987 . An in vitro study of the chemical durability of siliceous fibres . Ann. Occup. Hyg , 31 : 683
- Selikoff , I. J. , Hammond , E. C. and Churg , J. 1968 . Asbestos exposure, smoking, and neoplasia . J. Am. Med. Assoc , 204 : 106 – 112 .
- Shukla , A. , Timblin , C. , BeruBe , K. , Gordon , T. , McKinney , W. , Driscoll , K. , Vacek , P. and Mossman , B. T. 2000 . Inhaled particulate matter causes expression of nuclear factor (NF)-kappaB-related genes and oxidant-dependent NF-kappaB activation in vitro . Am. J. Respir. Cell Mol. Biol , 23 : 182 – 187 .
- Shukla , A. , Ramos-Nino , M. and Mossman , B. 2003a . Cell signaling and transcription factor activation by asbestos in lung injury and disease . Int. J. Biochem. Cell Biol , 35 : 1198 – 1209 .
- Shukla , A. , Gulumian , M. , Hei , T. K. , Kamp , D. , Rahman , Q. and Mossman , B. T. 2003b . Multiple roles of oxidants in the pathogenesis of asbestos-induced diseases . Free Radical Biol. Med , 34 : 1117 – 1129 .
- Shukla , A. , Flanders , T. , Lounsbury , K. M. and Mossman , B. T. 2004a . The gamma-glutamylcysteine synthetase and glutathione regulate asbestos-induced expression of activator protein-1 family members and activity . Cancer Res , 64 : 7780 – 7786 .
- Shukla , A. , Vacek , P. and Mossman , B. T. 2004b . Dose response relationships in expression of biomarkers of cell proliferation in in vitro assays and inhalation experiments . Nonlinearity Biol. Toxicol. Med , 2 : 117 – 128 .
- Shukla , A. , Barrett , T. F. , Nakayama , K. I. , Nakayama , K. , Mossman , B. T. and Lounsbury , K. M. 2006 . Transcriptional up-regulation of MMP12 and MMP13 by asbestos occurs via a PKC delta-dependent pathway in murine lung . FASEB J , 20 : 997 – 999 .
- Shukla , A. , MacPherson , M. B. , Hillegass , J. , Ramos-Nino , M. E. , Alexeeva , V. , Vacek , P. M. , Bond , J. P. , Pass , H. I. , Steele , C. and Mossman , B. T. 2009 . Alterations in gene expression in human mesothelial cells correlate with mineral pathogenicity . Am. J. Respir. Cell Mol. Biol , 41 : 114 – 123 .
- Soutar , C. A. , Simon , G. and Turner-Warwick , M. 1974 . The radiology of asbestos-induced disease of the lungs . Br. J. Dis. Chest , 68 : 235 – 252 .
- Stanton , M. F. , Layard , M. , Tegeris , A. , Miller , E. , May , M. , Morgan , E. and Smith , A. 1981 . Relation of particle dimension to carcinogenicity in amphibole asbestoses and other fibrous minerals . JNCI , 67 : 965 – 975 .
- Stöber , F. W. S. , Flachsbart , F. H. S. and Hochrainer , F. D. D. 1970 . Der aerodynamische durchmesser von latexaggregaten und asbestfasern . Staub-Reinhalt. Luft , 30 : 277 – 285 .
- Su , W. C. and Cheng , Y. S. 2006 . Fiber deposition pattern in two human respiratory tract replicas . Inhal. Toxicol , 18 : 749 – 760 .
- Sullivan , D. E. , Ferris , M. , Pociask , D. and Brody , A. R. 2005 . Tumor necrosis factor-alpha induces transforming growth factor-beta1 expression in lung fibroblasts through the extracellular signal-regulated kinase pathway . Am. J. Respir. Cell Mol. Biol , 32 : 342 – 349 .
- Sullivan , D. E. , Ferris , M. , Pociask , D. and Brody , A. R. 2008 . The latent form of TGFbeta(1) is induced by TNFalpha through an ERK specific pathway and is activated by asbestos-derived reactive oxygen species in vitro and in vivo . J. Immunotoxicol , 5 : 145 – 149 .
- Sullivan , D. E. , Ferris , M. , Nguyen , H. , Abboud , E. and Brody , A. R. 2009 . TNF-alpha induces TGF-beta1 expression in lung fibroblasts at the transcriptional level via AP-1 activation . J. Cell Mol. Med , 13 : 1866 – 1876 .
- Sussman , R. G. , Cohen , B. S. and Lippmann , M. 1991a . Asbestos fiber deposition in a human tracheobronchial cast. I. Experimental . Inhal. Toxicol , 3 : 145 – 160 .
- Sussman , R. G. , Cohen , B. S. and Lippmann , M. 1991b . Asbestos fiber deposition in a human tracheobronchial cast. II. Empirical model . Inhal. Toxicol , 3 : 161 – 179 .
- Tanaka , S. , Choe , N. , Hemenway , D. R. , Zhu , S. , Matalon , S. and Kagan , E. 1998 . Asbestos inhalation induces reactive nitrogen species and nitrotyrosine formation in the lungs and pleura of the rat . J. Clin. Invest , 102 : 445 – 454 .
- Thomas , G. , Ando , T. , Verma , K. and Kagan , E. 1994 . Asbestos fibers and interferon-gamma up-regulate nitric oxide production in rat alveolar macrophages . Am. J. Respir. Cell Mol. Biol , 11 : 707 – 715 .
- Timbrell , V. 1972 . “ An aerosol spectrometer and its applications ” . In Assessment of airborne particles , Edited by: Mercer , T. T. , Morrow , P. E. and Stober , W. 290 – 330 . Springfield, IL : Thomas .
- Timbrell , V. 1982 . Deposition and retention of fibres in the human lung . Ann. Occup. Hyg , 26 : 347 – 369 .
- Tran , C. L. , Jones , A. D. , Cullen , R. T. and Donaldson , K. 1997 . Overloading of clearance of particles and fibres . Ann. Occup. Hyg , 41 : 23 – 243 .
- U.S. Environmental Protection Agency . 2001 . Combined chronic toxicity/carcinogenicity testing of respirable fibrous particle, Final test guideline , Washington, DC : U.S. Environmental Protection Agency, Office of Program Management and Evaluation, Office of Pollution Prevention and Toxics (7401) .
- U.S. Environmental Protection Agency . 2003 . Report on the Peer Consultation Workshop to discuss a proposed protocol to assess asbestos-related risk , Washington, DC : U.S. Environmental Protection Agency, Office of Solid Waste and Emergency Response .
- Vainio , H. and Boffetta , P. 1994 . Mechanisms of the combined effect of asbestos and smoking in the etiology of lung cancer . Scand. J. Work Environ. Health , 20 : 235 – 242 .
- Vincent , J. H. 2005 . Health-related aerosol measurement: A review of existing sampling criteria and proposals for new ones . J. Environ. Monit , 7 : 1037 – 1053 .
- Vincent , J. H. , Johnston , A. M. , Jones , A. D. , Bolton , R. E. and Addison , J. 1985 . Kinetics of deposition and clearance of inhaled mineral dusts during chronic exposure . Br. J. Ind. Med , 42 : 707 – 715 .
- Vorwald , A. J. , Durkan , T. M. and Pratt , P. C. 1951 . Experimental studies of asbestosis . AMA Arch. Ind. Hyg. Occup. Med , 3 : 1 – 43 .
- Vu , V. , Barrett , J. C. , Roycroft , J. , Schuman , L. , Dankovic , D. , Bbaro , P. , Martonen , T. , Pepelko , W. and Lai , D. 1996 . Chronic inhalation toxicity and carcinogenicity testing of respirable fibrous particles. Workshop report . Regul. Toxicol. Pharmacol , 24 : 202 – 212 .
- Wagner , J. C. , Berry , G. , Skidmore , J. W. and Timbrell , V. 1974 . The effects of the inhalation of asbestos in rats . Br. J. Cancer , 29 : 252 – 269 .
- Wagner , J. C. and Skidmore , J. W. 1965 . Asbestos dust deposition and retention in rats . Ann. NY Acad. Sci , 132 : 77 – 86 .
- Walker , N. J. and Bucher , J. R. 2009 . A 21st century paradigm for evaluating the health hazards of nanoscale materials? . Toxicol. Sci , 110 : 251 – 254 .
- Wang , X. , Christiani , D. C. , Wiencke , J. K. , Fischbein , M. , Xu , X. , Cheng , T. J. , Mark , E. , Wain , J. C. and Kelsey , K. T. 1995 . Mutations in the p53 gene in lung cancer are associated with cigarette smoking and asbestos exposure . Cancer Epidemiol Biomarkers Prev , 4 : 543 – 548 .
- Warheit , D. B. and Hartsky , M. A. 1990 . Species comparisons of proximal alveolar deposition patterns of inhaled particulates . Exp. Lung Res , 16 : 83 – 99 .
- Warheit , D. B. , Hill , L. H. , George , G. and Brody , A. R. 1986 . Time course of chemotactic factor generation and the corresponding macrophage response to asbestos inhalation . Am. Rev. Respir. Dis , 134 : 128 – 133 .
- Weiss , W. 1984 . Cigarette smoke, asbestos, and small irregular opacities . Am. Rev. Respir. Dis , 130 : 293 – 301 .
- Weiss , W. 2000 . Asbestosis and lobar site of lung cancer . Occup. Environ. Med , 57 : 358 – 360 .
- Wikman , H. , Ruosaari , S. , Nymark , P. , Sarhadi , V. K. , Saharinen , J. , Vanhala , E. , Karjalainen , A. , Hollmen , J. , Knuutila , S. and Anttila , S. 2007 . Gene expression and copy number profiling suggests the importance of allelic imbalance in 19p in asbestos-associated lung cancer . Oncogene , 26 : 4730 – 4737 .
- Woodworth , C. D. , Mossman , B. T. and Craighead , J. E. 1983 . Induction of squamous metaplasia in organ cultures of hamster trachea by naturally occurring and synthetic fibers . Cancer Res , 43 : 4906 – 4912 .
- World Health Organization . 1988 . “ 77 Man-made mineral fibres ” . In Environmental Health Criteria , Geneva, , Switzerland : World Health Organization .
- World Health Organization . 1998 . “ Chrysotile asbestos ” . In Environmental Health Criteria Series , Geneva, , Switzerland : World Health Organization .
- Yarborough , C. M. 2006 . Chrysotile as a cause of mesothelioma: An assessment based on epidemiology . Crit. Rev. Toxicol , 36 : 165 – 187 .
- Yarborough , C. M. 2007 . The risk of mesothelioma from exposure to chrysotile asbestos . Curr. Opin. Pulmonary Med , 13 : 334 – 338 .
- Yuan , Z. , Taatjes , D. J. , Mossman , B. T. and Heintz , N. H. 2004 . The duration of nuclear extracellular signal-regulated kinase 1 and 2 signaling during cell cycle reentry distinguishes proliferation from apoptosis in response to asbestos . Cancer Res , 64 : 6530 – 6536 .
- Zanella , C. L. , Posada , J. , Tritton , T. R. and Mossman , B. T. 1996 . Asbestos causes stimulation of the extracellular signal-regulated kinase 1 mitogen-activated protein kinase cascade after phosphorylation of the epidermal growth factor receptor . Cancer Res , 56 : 5334 – 5338 .
- Zeidler-Erdely , P. C. , Calhoun , W. J. , Ameredes , B. T. , Clark , M. P. , Deye , G. J. , Baron , P. , Jones , W. , Blake , T. and Castranova , V. 2006 . In vitro cytotoxicity of Manville Code 100 glass fibers: Effect of fiber length on human alveolar macrophages . Part. Fibre Toxicol , 3 : 5
- Zhang , P. , Wang , Y. Z. , Kagan , E. and Bonner , J. C. 2000 . Peroxynitrite targets the epidermal growth factor receptor, Raf-1, and MEK independently to activate MAPK . J. Biol. Chem , 275 : 22479 – 22486 .