ABSTRACT
Phoenix sylvestris is an underutilized seasonal fruit in West Bengal, India. Methanol extract and extracts after alkaline hydrolysis of the mesocarp tissue of full-mature edible fruits of P. sylvestris were analyzed by GC-MS following a metabolomics approach. The fractions were tested for their antioxidant and inhibitory properties against the two key enzymes involved in diabetes, α-amylase, and α-glucosidase. Total 71 metabolites belonging to organic acids, amino acids, sugars, sugar alcohols, fatty acids, and phenols were identified in the methanol extract and in fractions after saponification. All the extracts and fractions showed high antioxidant, α-glucosidase, and α-amylase inhibitory activities. Sugars like raffinose (IC50 = 0.36 μM), sucrose (IC50 = 0.51 μM), trehalose (IC50 = 0.85 μM), and phenols like taxifolin (IC50 = 0.31 μM), benzoic acid (IC50 = 2.74 μM) inhibited only the enzyme α-amylase. Phenolic components which inhibited both the enzymes were caffeic acid (IC50 = 1.42 μM for α-amylase and IC50 = 1.8 μM for α-glucosidase), 3, 4-dihydroxy benzoic acid (IC50 = 0.23 μM for α-amylase and IC50 = 2.58 μM for α-glucosidase), and quinic acid (IC50 = 4.91 μM for α-amylase and IC50 = 4.95 μM for α-glucosidase). Ferulic acid (IC50 = 0.52 μM) and 4-hydroxycinnamic acid (IC50 = 0.23 μM) inhibited only α-glucosidase. This study suggested that the metabolites present in the fruit mesocarp tissue showed the potential antioxidant activity and properties to inhibit the enzymes α-amylase and α-glucosidase. Further in vivo study is to be carried out to prove the efficacy of the fruits.
Abbreviations: FM: crude methanol extract; SI: ethyl acetate extract after alkaline hydrolysis step I; SII: ethyl acetate extract after alkaline hydrolysis step II
Introduction
Phoenix sylvestris (L.) Roxb. (Arecaceae) is distributed in the South Asia from Pakistan to Myanmar across India, Nepal, Bhutan, and Bangladesh.[Citation1] The fruits are edible but of inferior quality to that of the commercially important fruits of P. dactylifera. Morphologically, P. sylvestris plants have solitary trunk with a spherical crown of long, green leaves with a few short spines at the base. Pinnae are numerous, linear ending in short points. The trunk is covered by persistent bases of petioles.[Citation2] Each fruit of P. sylvestris is about 2.5 cm long, orange yellow, rounded at the ends, and sweet in taste. Edible portion of the fruit consists of only the scaly epicarp and the fibrous mesocarp around the large seed. The plant usually grows wild and prefers moist alluvial soils. Natural regeneration takes place freely by seeds. But the naturally grown plants are also sometimes cultivated by medium category farmers and managed by landless farmers also mainly for sap production. The farmers practice their indigenous knowledge from planting through tapping for collection of sap [Citation3] which is used for production of jaggery or gur. The sap (nira) is consumed fresh or is sometimes fermented into a drink called “toddy.” P. sylvestris populations do not seem to undergo any selection based on individual qualities. From a genetic point of view, the cultivated populations therefore should have the same structure as wild ones.[Citation1]
Carotenoids, phenolic acids, flavonoids, sterols, and anthocyanins of the fruits of P. dactylifera are in report.[Citation4–Citation7] P. dactylifera fruits are nutritious and are reported to have antioxidant,[Citation4–Citation7] antimutagenic,[Citation8] antihepatotoxic,[Citation9] nephroprotective,[Citation10] immunostimulant,[Citation11] gonadotropic,[Citation12] anti-inflammatory,[Citation13] and antihyperlipidaemic [Citation14] properties. In contrast, P. sylvestris fruits are underutilized because of their thin flesh (60 percent of the whole fruit). Fruits are considered as restorative. They may be preserved as such or made into jellies and jams.[Citation2] Previously we reported high α – amylase and α – glucosidase and Angiotensin I-converting enzyme inhibitory properties of this fruit.[Citation15,Citation16] Report on detailed phytochemical and pharmacological analysis of the fruits of P. sylvestris is lacking. The objectives of the present work were to profile the metabolites of the mesocarp of P. sylvestris fruits and to identify the compounds responsible for inhibiting the key enzymes α-amylase and α-glucosidase involved in diabetes.
Materials and methods
Collection of plant material
Three kilograms of orange-coloured fruits of Phoenix sylvestris at their full-mature stage, which on further ripening turns reddish brown after postharvest storage at room temperature, were collected from a single plant growing in Pichaboni village of East Midnapur district, West Bengal, India during summer in the month of May, 2012 (voucher no. 33213a) when the fruits ripen. Fruits were separated from the fruiting spadices and stored at −20ºC prior to analysis.
Reagents
PNPG (p-nitrophenyl α-D-glucopyranoside), α-Glucosidase (e.g., microorganism), α-Amylase (e.g., porcine pancreas) from Sisco Research Laboratories Pvt. Ltd., India, DNSA (3, 5-di-nitro salicylic acid), starch from Sigma, USA, Adonitol, Methoxamine hydrochloride from Sigma (St. Louis, MO), N-methyl-N-trimethylsilyltrifluoroacetamide (MSTFA) with 1% trimethylchlorosilane (TMCS), Methyl Octanoate (C8), Methyl Decanoate (C10), Methyl Laurate (C12), Myristic Acid Methyl Ester (C14), Methyl Palmitate (C16), Stearic Acid Methyl Ester (C18), Methyl Arachidate (C20), Methyl Behenate (C22), Methyl Tetracosanoate (C24), Methyl Hexacosanoate (C26) from SIGMA ALDRICH were purchased. All the other reagents used for sample preparation were of analytical grade, and all the solvents used for GC/MS were of HPLC grade.
Extraction of metabolites from P. sylvestris fruit mesocarp
Mesocarpic tissue (120 g) was separated manually from the seeds and the epicarp of the mature P. sylvestris fruit. The mesocarp tissue was extracted modifying the method of Vera et al. [Citation17] Crushed separated pieces were soaked in 700 ml of 50% methanol (MeOH) overnight at low temperature (4ºC) in freezer, after wrapping the whole content with black paper to protect it from light and to reduce the browning reaction. The methanol extract was evaporated to dryness under reduced pressure in a rotary evaporator to obtain the crude extract (FM). The residual mesocarp tissue (38 g), after extraction with MeOH, was rinsed repeatedly with distilled water until and unless the tissue became lighter in colour. Then it was dried in oven at 50ºC for 24 h. The dried tissue (11 g) was subjected to sequential alkaline hydrolysis under progressively more vigorous condition.[Citation18,Citation19] It was extracted with 550 ml of 0.1 (M) NaOH for 24 h at room temperature in the dark (Step I). The suspension was filtered, and the residue was further extracted with 550 ml of 2 (M) NaOH under the same condition (Step II).
Each of the extracts obtained after step I and step II of alkaline hydrolysis was collected separately after filtering the solution. It was then acidified with concentrated HCl till the pH reached 2.0. The acidified solution was extracted with ethyl acetate (x3) in a separating funnel, and the organic phase was collected. The combined organic phase was evaporated to dryness under vacuum at 30ºC in a rotary evaporator. Thus, the extracts obtained were crude SI and SII from fractions after step I and step II of hydrolysis, respectively.
Preparation of sample for identification of metabolites of P. sylvestris fruits
FM (30 mg) was dissolved in MeOH: water (1:1). SI and SII (10 mg each) were dissolved in 2.5 ml MeOH. Adonitol (20 µl of 0.2 mg/ml solution) was added as internal standard. Each of the sample solutions was distributed into Eppendorf tubes (3 × 50 µl) and evaporated to dryness. The residue was re-dissolved in 10 μl of methoxyamine hydrochloride (20 mg/ml in Pyridine) and subsequently shaken for 90 min at 30ºC. Then 90 μl of N-methyl-N-trimethylsilyltrifluoroacetamide (MSTFA) with 1% trimethylchlorosilane (TMCS) was added, and the mixture was shaken at 37ºC for 30 min for trimethylsilylation of acidic protons to increase volatility of metabolites. Fatty Acid Methyl Esters (FAME) markers [a mixture of internal Retention Index (RI) markers was prepared using fatty acid methyl esters of C8, C10, C12, C14, C16, C18, C20, C22, C24, and C26 linear chain length, dissolved in chloroform (HPLC) at a concentration of 0.8 mg/ml (C8–C16) and 0.4 mg/ml (C18–C26)] was added.[Citation20]
Analytical methods
GC-MS analysis
GC-MS analysis was carried out following the method of Kind et al. [Citation20] after modification.[Citation21] HP-5MS capillary column (Agilent J & W; GC Columns (USA) (length 30 m plus Duraguard 10 m, diameter 0.25 mm narrow bore, film 0.25 μm) was used. Injection was made in sandwich mode with fast plunger speed without viscosity delay or dwell time. The analysis was performed under the following oven temperature programme: oven ramp 60°C (1 min hold) to 325ºC at 10ºC/min; 10 min hold before cool-down, 37.5 min run time. The injection temperature was set at 250ºC, the MS transfer line at 290ºC, and the ion source at 230ºC. Helium was used as the carrier gas at a constant flow rate of 0.723 ml/min (carrier linear velocity 31.141 cm/sec). Samples (1 μl) were injected via the split mode (Split ratio 1:5) onto the GC column. Prior to analysis, the method was calibrated with the FAME standards available with the Fiehn GC /MS Metabolomics library (2008) (Agilent Chem Station, Agilent Technologies Inc., Wilmington, USA). Identification of the metabolites was carried out by comparing the fragmentation patterns of the mass spectra and retention times (Rt) with entries of mass spectra and retention time in Agilent Fiehn Metabolomics library using Agilent retention time locking (RTL) method. Fiehn retention indices (RI) were also compared. Automated mass spectral deconvolution and identification system (AMDIS) was used to deconvolute GC-MS results and to identify chromatographic peaks.
α-Glucosidase inhibitory activity
α-Glucosidase inhibitory activity was measured following the modified methods of Kwon et al. [Citation22] α-Glucosidase (e.g., microorganism) solution (0.006%) was prepared in 0.02 M phosphate buffer (pH 6.3). In each test, 0.034 ml of aqueous extract of fruit, 0.11 ml of Phosphate Buffer (pH 6.3), and 0.034 ml of enzyme were mixed. After 1 h of incubation at 25°C, 0.17 ml of p-nitrophenyl-α-D-glucopyranoside was added. Again the reaction mixtures were incubated at 30°C for 30 min. The enzyme reaction was stopped by adding 0.5 ml of 1(M) Na2CO3 solution. Blank set consisted of 0.034 ml of buffer instead of enzyme. Individual blanks were prepared for correcting the background absorbance. The control set contained 0.034 ml of distilled water instead of fruit extract. The Optical Density was measured at 405 nm spectrophotometrically. The percentage inhibition of α-glucosidase inhibitory activity by plant extract was calculated using the formula [(Ao-Ae)/Ao] x100 (Ao = Absorbance without extract; Ae = absorbance with extract).
α-Amylase inhibitory activity
α-Amylase inhibitory activity was measured following the modified method of Bernfeld [Citation23] as mentioned in Das et al. [Citation21] The reaction mixture consisted of 0.025 ml of aqueous extract of fruit and 0.05 ml of enzyme (ex. porcine pancreas) (0.2 ml of 0.003 % solution) dissolved in 0.02 M phosphate buffer (pH 6.9). After incubation at 37°C for 20 min, 0.025 ml of starch was added, and the mixture was again incubated at 37°C for 3 min. Then 0.05 ml of DNSA was added, and the tube was well plugged and heated at 100°C in water bath for 5 min. Distilled water (1 ml) was added after cooling, and then the absorbance was measured at 540 nm spectrophotometrically. The percentage inhibition of α-amylase activity by plant extract was calculated as [(Ao-Ae)/Ao] x100 (Ao = Absorbance without extract; Ae = absorbance with extract).
Determination of antioxidant activity
DPPH radical scavenging activity
The antioxidant activity of the extracts on the basis of scavenging the stable 1, 1-diphenyl-2-picrylhydrazyl (DPPH) free radical was determined following the method described by Braca et al. [Citation24] Aqueous /methanolic extract (0.1 ml) was added to 3 ml of 0.004% MeOH solution of DPPH. Absorbance at 517 nm was determined after 30 min, and the percentage inhibition activity was calculated as [(Ao-Ae)/Ao] x 100 (Ao = Absorbance without extract; Ae = absorbance with extract).
Superoxide radical (O2−.) scavenging activity
Superoxide radical scavenging activity was measured in the riboflavin-light-nitro blue tetrazolium (NBT) system [Citation25] as mentioned before. [Citation26] Each 3 ml reaction mixture contained 50 mM phosphate buffer (pH 7.8), 13 mM methionine, 2 μM riboflavin, 100 μM EDTA, NBT (75 μM), and 1 ml sample solution. The production of blue formazan was followed by monitoring the increase in absorbance at 560 nm after 10 min illumination from a fluorescent lamp.
Total antioxidant capacity
The assay is based on the reduction of Mo (VI) to Mo (V) by the extract and subsequent formation of a green phosphate/Mo (V) complex at acid pH.[Citation27] The reaction mixture consisted of 0.1 ml sample solution and 1 ml of reagent solution (Phosphate buffer, sulphuric acid, and ammonium heptamolybdate in a ratio of 4:3:3). Total antioxidant capacity (TAC) was measured as equivalent to ascorbic acid.
Statistical analysis
All the experiments were performed thrice. Mathematical calculations like means and standard deviations were calculated from replicas within the experiments, and analyses have been done using Microsoft Excel 2007. In GC/MS data analysis, the relative response ratios of all the metabolites, which are routinely described for comparison, were calculated after normalizing the peak areas of the compounds by extract dry weight and by the peak area of the internal standard adonitol.[Citation28] The metabolite profile was statistically analyzed by Tukey method to compare between groups (FM, SI, SII).
Results and discussion
Profiling metabolites from P. sylvestris mesocarp tissue
Metabolites present in the methanol extract (FM) of the fruit mesocarp as well as in the extracts after alkaline hydrolysis (SI and SII) were profiled using GC/MS analysis. Total 71 metabolites (15 organic acids, 8 amino acids, 5 sugars, 7 sugar alcohols, 13 fatty acids, 20 phenolic compounds, and 3 other components) were identified from the fruit mesocarp (). FM consisted mainly of the organic acids, amino acids, sugars, sugar alcohols, some fatty acids, and a few phenolic compounds. SI fraction was rich in different fatty acids and phenolic compounds. SII fraction was rich in phenolic compounds. FM contained all the sugars. The sugar alcohols, except glycerol, 1-hexadecanol, and xylitol, were mainly present in that fraction. 4-Guanidinobutyric acid, gluconic acid, lauric acid, palmitic acid, and stearic acid were detected in FM fraction. Palmitic acid and stearic acid are the fatty acids present in high amount in SI. FM contains only two phenolic compounds (e.g., 2-amino-3-methoxy benzoic acid and catechin). SI and SII were rich in phenolic constituents. Major components of these fractions were caffeic acid, 3,4- dihydroxybenzoic acid (protocatechuic acid), 3, 4-dihydroxy phenyl acetic acid, ferulic acid, 4-hydroxybenzoic acid, 4-hydroxycinnamic acid, 4-hydroxy 3-methoxy benzoic acid, 2-hydroxy-2-napthoic acid, piceatannol, quinic acid, shikimic acid, and taxifolin.
Table 1. GC-MS identified metabolites in P. sylvestris mesocarp tissue.
A few fatty acids were present in the free form which could be detected in FM. After mild saponification, the fatty acids combined in complex molecules were made free. That is why higher amounts of fatty acids could be detected in SI fraction. Higher level of glycerol, after saponification, was detected in SI. This is probably due to hydrolysis of lipids and liberation of this sugar alcohol. A larger number of phenolic compounds were detected in SI and SII after saponification due to liberation of cell wall bound phenolics.
α-Glucosidase and α-amylase inhibitory activities
α-Glucosidase and α-amylase inhibitory activities in the three extracts FM, SI, and SII were measured. All the fractions inhibited the carbohydrate digesting enzymes in a dose dependent manner. IC50 values (the concentrations required for inhibition of the enzyme activities by 50%) of the three different extract fractions were compared in . The SII fraction showed the highest α-glucosidase (IC50 = 3.18 µg/ml) and α-amylase (IC50 value = 140.4 µg/ml) inhibitory activities. SI fraction showed very high α-glucosidase inhibition (IC50 value = 21.62 µg/ml) but no α-amylase inhibition in the concentration range within which the experiments were carried out. On the other hand, the FM fraction showed lowest α-glucosidase and α-amylase inhibition activities. IC50 values for α-glucosidase and α-amylase inhibitions were 134.21 µg/ml and 306.14 µg/ml, respectively, in the FM.
Figure 1. Comparison of the glycosidase inhibition by three fractions isolated from mesocarp tissue of P. sylvestris fruits.
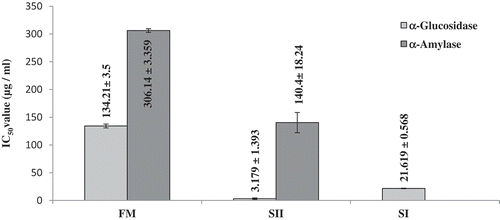
The activities of some of the metabolites detected from the FM, SI, and SII fractions were studied against the enzymes α-glucosidase and α-amylase. Those metabolites were three sugars (raffinose, sucrose, and trehalose) and seven phenolic components (caffeic acid, 3, 4-dihydroxy benzoic acid, 4-hydroxybenzoic acid, ferulic acid, 4-hydroxycinnamic acid, taxifolin, and quinic acid). The sugars inhibited only the enzyme α-amylase. Highest activity was observed in raffinose. All the phenolic compounds, except ferulic acid and 4-hydroxycinnamic acid, inhibited the enzyme α-amylase. Ferulic acid and 4-hydroxycinnamic acid inhibited only α-glucosidase. The phenolic metabolites, except benzoic acid and taxifolin, inhibited α-glucosidase. IC50 value for α-amylase inhibitory activity was lowest in 3, 4-dihydroxy benzoic acid indicating highest activity of this metabolite than the others. Other metabolites showed α-amylase inhibitory activities in the following decreasing order (e.g., taxifolin, caffeic acid, benzoic acid, quinic acid). Highest α-glucosidase inhibition was noticed with hydroxycinnamic acid followed by ferulic acid, caffeic acid, 3,4-dihydroxy benzoic acid, and quinic acid ().
Table 2. Glycosidase inhibitory properties of metabolites detected in P. sylvestris mesocarp.
Fatty acids have previously been reported to have such inhibitory properties against the enzymes α-glucosidase and α-amylase. Oleic acid, linoleic acid, and palmitic acid[Citation29,Citation30] were reported to have potent glucosidase inhibitory properties. The phenolic metabolites showed glycosidase inhibitory properties during the present study. Catechin was previously reported to have anti-amylase and anti-glucosidase properties.[Citation31] Higher glycosidase inhibitory properties of SI and SII fractions are probably due to the presence of higher concentrations of fatty acids and phenolic compounds in those fractions than that of the methanol soluble fraction.
Inhibition of carbohydrate digesting enzymes α-amylase and α-glucosidase decrease post-prandial hyperglycemia or raised blood sugar related to type 2 diabetes.[Citation22] Synthetic α-glucosidase inhibitor voglibose has side effects on the small intestine.[Citation32] So search for antidiabetic potential of plants and phytochemicals has received attention for the treatment of such disease with many eventual complications. Antidiabetic potential of many edible plants,[Citation33–Citation36] and grains [Citation37,Citation38] are in report. Fruit extracts and their metabolites are also reported to inhibit α-amylase and α-glucosidase.[Citation15,Citation21,Citation39] This study showed the antidiabetic potential of P. sylvestris fruits and the sugar and phenolic metabolites through inhibition of the carbohydrate digesting enzymes.
Antioxidant activities
Methanol extract and extracts after saponification scavenged DPPH radical in dose dependent manner, IC50 values being 0.14 mg/ml ± 0.001, 0.27 mg/ml ± 0.003 and 0.22 mg/ml ± 0.001 for FM, SI, and SII, respectively. The extracts also scavenged superoxide radical in concentration dependent manner. The IC50 values of FM, SI, and SII were 0.55 mg/ml ± 0.02, 0.45 mg/ml ± 0.002, and 4.44 mg/ml ± 0.038, respectively, for scavenging superoxide radical. Methanol extract showed highest ascorbic acid equivalent total antioxidant capacity (). Antioxidant activities of some of the phenolic compounds identified in the extracts were also measured (). Ferulic acid, caffeic acid, 4-hydroxycinnamic acid, and 3,4-dihydroxybenzoic acid scavenged superoxide radical which initiates generation of harmful reactive oxygen species. The antioxidant activities of different extracts of P. sylvestris fruit mesocarp were due to the presence of these phenolic constituents. Reactive oxygen species induce oxidative damage of biomolecules and accelerate different oxidative stress induced diseases[Citation40–Citation43] including diabetes. Antioxidant properties of edible plants, leafy vegetables, and flowers were reported earlier from the laboratory.[Citation44–Citation46] Now we report antioxidant potential of metabolites present in P. sylvestris fruit mesocarp tissue. Indigenous fruits have antioxidant properties.[Citation47,Citation48] This study suggested the potential health beneficial effect of P. sylvestris fruit through prevention of formation of free radicals. The underutilized fruits of P. sylvestris could be used as a food ingredient because of the important biological activities determined during the present study. Increased consumption would help improve the economic conditions of the farmers in the rural areas.
Table 3. Antioxidant properties of P. sylvestris mesocarp extracts and metabolites.
Conclusions
The present study demonstrates the potential antioxidant, α-glucosidase, and α-amylase inhibitory activities of P. sylvestris fruit mesocarp due to the presence of the phenolic compounds and sugars detected in the mesocarp tissue. This in vitro study showed that the sugars (e.g., raffinose, sucrose, and trehalose) inhibited α-amylase. Caffeic acid, 3,4-dihydroxy benzoic acid, and quinic acid inhibited both the carbohydrate digesting enzymes. Benzoic acid and taxifolin inhibited only α-amylase. Ferulic acid and 4-hydroxycinnamic acid inhibited only α-glucosidase. The phenolic compounds were also responsible for the antioxidant properties. So it can be concluded that P. sylvestris fruits have potential health beneficial properties.
Acknowledgements
The authors are grateful to the date palm farmer of Midnapur district, West Bengal, India, for supplying the ample amount of fruits. SD acknowledges financial assistance from University Grant Commission, India (Faculty Development Programme – Teacher Fellowship Award). Financial support from DST (FIST) is also acknowledged.
References
- Newton, C.; Gros-Balthazard, M.; Ivorra, S.; Paradis, L.; Pintaud, J.; Terral, J. Phoenix dactylifera and P. sylvestris in Northwestern India: A Glimpse of Their Complex Relationships. Palms 2013, 51, 37–50.
- Anonymous. A Dictionary of Indian Raw Materials and Industrial Products. Publication and Information Directorate, CSIR, New Delhi. The Wealth of India 1969, 8, 25–26.
- Chowdhury, MSH; Halim, MA.; Muhammed, N.; Haque, F.; Koiko, M. Traditional Utilization of Wild Date Palm (Phoenix sylvestris) in Rural Bangladesh: An Approach to Sustainable Biodiversity Management. Journal of Forestry Research 2008, 19, 245–251.
- Al-Farsi, M.; Alasalvar, C.; Morris, A.; Baron, M.; Shahidi, F. Comparison of Antioxidant Activity, Anthocyanins, Carotenoids and Phenolics of Three Native Fresh and Sun-dried Date (Phoenix dactylifera L.) Varieties Grown in Oman. Journal of Agricultural and Food Chemistry 2005, 53,7592–7599.
- Boudries, H.; Kefalas, P.; Hornero-Mendez, D. Carotenoid Composition of Algerian Date Varieties at Different Edible Maturation Stages. Food Chemistry 2007, 101, 1372–1377.
- Mansouri, A.; Embarek, G.; Kokkalou, E.; Kefalas, P. Phenolic Profile and Antioxidant Activity of the Algerian Ripe Date Palm Fruit (Phoenix dactylifera). Food Chemistry 2005, 89,411–420.
- Baliga, MS.; Baliga, BRV.; Kandathil, S.; Bhat, HP.; Vyalil, PK. Review of the Chemistry and Pharmacology of the Date Fruits (Phoenix dactylifera L.). Food Research International 2011, 44, 1812–1822.
- Vayalil, PK. Antioxidant and Antimutagenic Properties of Aqueous Extract of Date Fruit (Phoenix dactylifera L. Arecaceae). Journal of Agricultural and Food Chemistry 2002, 50, 610–617.
- Al Qarawi, AA.; Abdel-Rahman, H.; Ali, BH.; Mousa, H.M.; El-Mougy, SA. Protective Effect of Extracts from Dates (Phoenix dactylifera L.) on Carbon Tetrachloride-Induced Hepatotoxicity in Rats. The International Journal of Applied Research in Veterinary Medicine 2004, 3, 176–180.
- Al Qarawi, AA.; Abdel-Rahman, H.; Mousa, HM.; El-Mougy, SA. Nephroprotective Action of Phoenix Dactylifera in Gentamicin-induced Nephrotoxicity. Pharmaceutical Biology 2008, 46, 227–230.
- Puri, A.; Sahai, R.; Singh, KL.; Saxena, RP.; Tandon, JS.; Saxena, KC. Immunostimulant Activity of Dry Fruits and Plant Materials Used in Indian Traditional Medical System for Mothers and Childbirth and Individuals. Journal of Ethnopharmacology 2000, 71, 89–92.
- Ali, BH.; Bashir, AK.; Al Hadrami, G. Reproductive Hormonal Status of Rats Treated with Date Pits. Food Chemistry 1999, 66, 437–441.
- Doha, MA.; Al-Okbi, SY. In Vivo Evaluation of Antioxidant and Anti-inflammatory Activity of Different Extracts of Date Fruits in Adjuvant Arthritis. Polish Journal of Food and Nutrition Sciences 2004, 13, 397–402.
- Salah, A.; Al-Maiman. Effect of Date Palm (Phoenix dactylifera) Seed Fibres on Plasma Lipids in Rats. Journal of King Saud University 2005, 17, 117–123.
- Das, S.; Das, S.; De, B. In Vitro Inhibition of Key Enzymes Related to Diabetes by the Aqueous Extracts of Some Fruits of West Bengal, India. Current Nutrition and Food Science 2012, 8, 19–24
- Das, S.; De, B. Evaluation of Angiotensin I-Converting Enzyme Inhibitory Potential of Some Underutilized Indigenous Fruits of West Bengal using in Vitro Model. Fruits 2013, 68, 499–506.
- Vera, VL.; Tatyana, AG.; Elena, VY.; Natalya, IR.; Alfiya, V.; Alexander, U.; Jack, MW. Cold Alkali can Extract Phenolic Acids that are Ether Linked to Cell Wall Components in Dicotyledonous Plants (buckwheat, soybean and flax). Phytochemistry 1999, 50, 395–400.
- Dey, G.; Chakraborty, M.; Mitra, A. Profiling C6-C3 and C6-C1 Phenolic Metabolites in Cocos nucifera. Journal of Plant Physiology 2005, 162, 375–381.
- Parr, AJ.; Waldron, KW.; Ng, A.; Parker, ML. The Wall-bound Phenolics of Chinese Water Chestnut (Eleocharis dulcis). Journal of the Science of Food and Agriculture 1996, 71, 501–507.
- Kind, T.; Wohlgemuth, G.; Lee, DY.; Lu, Y.; Palazoglu, M.; Sevini, S.; Fiehn, O. FiehnLib – Mass Spectral and Retention Index Libraries for Metabolomics Based on Quadrupole and Time-of-flight Gas Chromatography/Mass Spectrometry. Analytical Chemistry 2009, 81, 10038–10048.
- Das, S.; Dutta, M.; Choudhury, K.; De, B. Metabolomic and Chemometric Study of Achras sapota L. Fruit Extracts for Identification of Metabolites Contributing to the Inhibition of α-amylase and α-glucosidase. European Food Research and Technology 2016, 242, 733–743.
- Kwon, YI.; Apostolidis, E.; Shetty, K. In vitro Studies of Eggplant (Solanum melongena) Phenolics as Inhibitors of Key Enzymes Relevant for Type 2 Diabetes and Hypertension. Bioresource Technology 2008, 99, 2981–2988.
- Bernfeld, P. Amylase, αand β. Meth Enzymol 1955, 1,149–158.
- Braca, A.; Nunziatina, De T.; Lorenzo, Di B.; Cosimo, P.; Mateo, P.; Ivano, M. Antioxidant Principles from Bauhinia terapotensis. Journal of Natural Product 2001, 64, 892–895.
- Beauchamp, C.; Fridovich, I. Superoxide Dismutase: Improved Assays and an Assay Applicable to Acrylamide Gels. Analytical Biochemistry 1971, 44, 276–287.
- Dasgupta, N.; De, B. Antioxidant Activity of Piper betle L. Leaf Extract in Vitro. Food Chemistry 2004, 88, 219–224.
- Prieto, P.; Pineda, M.; Aguilar, M. Spectrophotometric Quantitation of Antioxidant Capacity Through the Formation of a Phosphomolybdenum Complex: Specific Application to the Determination of Vitamin E. Analytical Biochemistry 1999, 269, 337–341.
- Oms-Oliu, G.; Hertog, MLA.; Van de Poel, TMB.; Ampofo-Asiama, J.; Geeraerd, AH.; Nicolaï, BM. Metabolic Characterization of Tomato Fruit During Preharvest Development, Ripening, and Postharvest Shelf-life. Postharvest Biology and Technology 2011, 62, 7–16.
- Su, C-H.; Hsu, C-H.; Ng, LT. Inhibitory Potential of Fatty Acids on Key Enzymes Related to Type 2 Diabetes. Biofactors 2013, 39, 415–421.
- Chen, L.; Kang, Y.; Suh, J. Roasting Processed Oriental Melon (Cucumis melo L. Var. makuwa Makino) Seed Influenced the Triglyceride Profile and the Inhibitory Potential Against Key Enzymes Relevant for Hyperglycemia. Food Research International 2014, 56, 236–242.
- Ray, S.; Samanta, T.; Mitra, A.; De, B. Effect of Extracts and Components of Black Tea on the Activity of β-glucuronidase, Lipase, α-amylase, α-glucosidase: An in Vitro Study. Current Nutrition and Food Science 2014, 10, 181–186.
- Murai, A.; Iwamura, K.; Takada, M.; Ogawa, K.; Usui, T.; Okumura, J. Control of Postprandial Hyperglycaemia by Galactosylmaltobiolactone and Its Novel Anti-amylase Effect in Mice. Life Science 2002, 71, 1405–1415.
- Adisakwattana, S.; Jiphimai, P.; Prutanopajai, P.; Chanathong, B.; Suwimol, S.; Ariyapitipan, T. Evaluation of α-glucosidase, α-amylase and Protein Glycation Inhibitory Activities of Edible Plants. International Journal of Food Sciences and Nutrition 2010, 61, 295–305.
- Mai, TT.; Thu, NN.; Tien, PG.; Van Chuyen, N. Alpha-glucosidase Inhibitory and Antioxidant Activities of Vietnamese Edible Plants and Theie Relationships with Polyphenol Contents. Journal of Nutritional Science and Vitaminology 2007, 53, 267–276.
- Liu, R.; Xu, B. Inhibitory Effects of Phenolics and Saponins from Commonly Consumed Food Legumes in China Against Digestive Enzymes Pancreatic Lipase and α-glycosidase. International Journal of Food Properties 2015, 18, 2246–2255.
- Nickavar, B.; Yousefian, N. Inhibitory Effects of Six Allium Species on α-amylase Enzyme Activity. Plant Foods for Human Nutrition 2011, 66, 143–148.
- Shobana, S.; Sreerama, YN.; Malleshi, NG. Composition and Enzyme Inhibitory Properties of Finger Millet (Elusine coracana L.) Seed Coat Phenolics: Mode of Inhibition of α-glucosidase and Pancreatic Amylase. Food Chemistry 2009, 115, 1268–1273.
- Das, S.; De, B. Glycosidase Inhibitory Properties of Different Varieties of Rice Grains fron India and Cyaniding-3-glucoside. Nutrition and Food Science 2012, 42, 428–433.
- Li, P.; Lin, Y.; Lu, W.; Hu, J.; Huang, D. In Vitro Hypoglycemic Activity of the Phenolic Compounds in Longan Fruit (Dimocarpus longan var. Fen Ke) Shell Against α-Glucosidase and β-galactosidase. International Journal of Food Properties 2016, 19, 1786–1797.
- Kellog, EW.; Fridovich, I.; Superoxide, Hydrogen Peroxide, and Singlet Oxygen in Lipid Peroxidation by Xanthine Oxidase System. Journal of Biological Chemistry 1975, 250, 8812–8817.
- Wiseman, H.; Halliwell, B. Damage to DNA by Reactive Oxygen and Nitrogen Species: Role of Inflammatory Disease and Progression to Cancer. Biochemical Journal 1996, 313, 17–29.
- Aimes, BN. Dietary Carcinogens and Anticarcinogens: Oxygen Radicals and Degenerative Diseases. Science 1983, 221, 1256–1264.
- Stadtman, ER. Protein Oxidation and Aging. Science 1992, 257, 1220–1224.
- Dasgupta, N.; De, B. Antioxidant Activity of Piper betle L. Leaf Extract in Vitro. Food Chemistry 2004, 88, 219–224.
- Dasgupta, N.; De, B. Antioxidant Activity of Some Leafy Vegetables of India: A Comparative Study, Food Chemistry 2007, 101, 471–474.
- Banerjee, A.; De, B. Comparative Study of Antioxidant Activity of the Food Flowers of West Bengal, India. International Journal of Food Properties 2013, 16, 193–204.
- Mahayothee, B.; Koomyart, I.; Khuwijitjaru, P.; Siriwongwilaichat, P.; Nagle, M.; Muuler, J. Phenolic Compounds, Antioxidant Activity, and Medium Chain Fatty Acids Profiles of Coconut Water And Meat at Different Maturity Stages. International Journal of Food Properties 2016, 19, 2041–2051.
- Banerjee, A.; Dasgupta, N.; De, B. In Vitro Study of Antioxidant Activity of Syzygium Cumini Fruit. Food Chemistry 2005, 90, 727–733.