ABSTRACT
Fractures in the maxillofacial region are among the most frequent incidences in patients exposed to trauma. The aim of this study was to evaluate the effect of 2100 MHz electromagnetic radiation on the healing of mandibular fractures in rabbits. The study was carried out on 12 mature male New Zealand rabbits. After producing a left mandibular osteotomy, the fracture was repaired by using a plate screw. The rabbits were divided into two groups – control and experimental. The experimental group was exposed for 3 h daily to a 2100 MHz radiofrequency (RF) for 28 d. Biomechanical, histopathological and radiological tests were performed during the healing of the mandibular bone. According to the biomechanical tests, the energy absorption capacity, toughness and maximum strength of the mandibular bone were increased compared to the control group (6.84 vs. 2.57, 0.135 vs. 0.041 and 22.21 vs. 10.32, respectively). No significant differences between the two groups were found during the radiological assessment. However, the histopathological examination revealed that the fracture healing score was higher in the experimental group when compared to the control group (8.0 vs. 2.75, respectively). In conclusion, the results of this study showed that a 2100 MHz RF radiation positively affected fracture healing.
Introduction
The healing of a fracture is a complex biological process that comprises several overlapping steps and is managed by a number of mechanisms. Maxillofacial injury occurs in approximately 5%–33% of patients which have experienced severe trauma.[Citation1,Citation2] It was also reported that the most frequent fractures seen in maxillofacial trauma were multiple bone fractures including the mandible, maxilla and zygoma.[Citation3] In the same study, open reduction and miniplate screw fixation were performed in the majority of the fractures.[Citation3] Since the bones in the maxillofacial region define the facial appearance, deformities of the appearance and functional problems may develop following significant fractures of this region. Delayed union or nonunion have been reported in approximately 5%–10% of the patients with fracture development.[Citation4,Citation5] This complication may result in long-term physical therapy procedures, repeating surgical interventions and disabilities. Therefore, the healing of a fracture in this particular area is of great importance.
Many local and systemic factors affect the fracture healing either positively or negatively. Diabetes, anaemia, malnutrition, as well as smoking and alcohol intake impact the bone healing negatively.[Citation6] While some antibiotics, corticosteroids and anticoagulants are agents that adversely affect bone healing, calcium sulphate, bone morphogenetic proteins, regular vitamin D intake, parathyroid hormone and oestrogen are some of the agents that improve bone healing.[Citation6–8] Other factors that influence bone healing are electrical and mechanical stimulation, pulsed mode ultrasound and radiofrequency (RF) irradiation.[Citation9–12]
Electromagnetic fields (EMFs) are an important external factor that has an effect on bone metabolism and fracture healing. First, it was shown that a low-frequency pulsed EMF facilitated bone formation.[Citation13] After this observation, many studies have been undertaken on this effect.[Citation14–17] Positive and negative effects, as well as lack of effects of EMFs at various frequencies and intensities have been reported on fracture healing in various studies.[Citation13,Citation14,Citation16,Citation18] RF radiation is one of the nonionizing parts of the electromagnetic spectrum. Sources of RF radiation include mobile phones, base stations, TV and radio antennas. Mobiles phones, despite being used for voice communication at first, have become part of wireless internet and communication technologies, which are indispensable elements of daily life. Mobile phones with 3G features and their accompanying base stations often utilize 1710–2170 MHz frequency band. Accompanied by the increase in mobile phone use, the duration of exposure to the RF radiation emitted by these phones is progressively increasing.[Citation19,Citation20] The maxillofacial region is one of the leading locations of exposure to RF radiation since cellular phones are often operated close to the ear. There are no published studies evaluating the effects of 2100 MHz radiation on the healing of fractures in the maxillofacial region. Therefore, the aim of this study was to evaluate the effect of 2100 MHz RF radiation on the healing of fractures in rabbit mandibular osteotomy.
Materials and methods
The experiments were performed on 12 mature male New Zealand rabbits weighing 2.5–3.3 kg (mean: 2.9 kg) obtained from the Medical Science Application and Research Center of Dicle University. Experimental protocols were approved by the local ethics committee (number of the ethics committee permission: 2012-49). A left mandibular osteotomy was performed on all rabbits. Subsequently, the rabbits were randomly divided into two groups with six rabbits in each group. The rabbits in each respective group were alternated within their group, and the staff who performed histological stainings and the one who carried out laboratory measurements did not know which sample belonged to which group. The experiment protocol ensured blindness in the laboratory experiments.
Surgical procedure
Ketamine (Ketalar; Pfizer, Istanbul, Turkey) in a dose of 35 mg/kg and xylazine in a dose of 3 mg/kg (Rompun; Bayer, Istanbul, Turkey) were injected intramuscularly for a general anaesthesia of the rabbits. Following the clipping of the hair in the submandibular region, the animal was placed in supine position, asepsis was provided and the animals were covered with sterile sheets. Two millilitres of articaine with 1:200,000 epinephrine (Ultracain-DS; Hoechst Marion Roussel, Istanbul, Turkey) were injected supraperiosteally to achieve anaesthesia and haemostasis. The mandibular corpus was exposed with a left submandibular incision. Straight, titanium, four-hole miniplate (Trimed®, Ucmed Medical, Ankara, Turkey) was fixed to the mandible using four miniscrews (2 mm × 7 mm) prior to the osteotomy. Subsequently, the miniplate and the screws were removed and an osteotomy was performed at a location of 2 mm anterior to the mental foramen using a fissured burr (2 mm diameter). A 2 mm gap was created between the bone fragments and then the fragments were fixed again using the miniplate screw. Soft tissues were sutured in layers using absorbable sutures (Vicryl; Ethicon, Brussels, Belgium). On the 28th postoperative day, all animals were sacrificed with an intravenous injection of 100 mg/kg pentobarbital sodium (Pental; IE Ulagay, Istanbul, Turkey). The complete mandibles were obtained and split at the midline with the use of a scalpel. Consequently, a fractured and a nonfractured hemi-mandible were obtained from each animal. Miniscrews and miniplates were removed from the fractured hemi-mandible.
Postoperative care
Antibiotics (Cefazolin 25 mg/kg; Cefamezine; Eczacıbaşı, Istanbul, Turkey) and analgesics (Tramadol 1 mg/kg; Contramal; Abdi İbrahim, Istanbul, Turkey) were injected intramuscularly at the end of the operation and the injections were repeated twice daily for the following four postoperative days. Rabbits were placed in different cages in the same environment. Animals were fed with normal diet postoperatively. In addition to the food and water intakes, the weight of the animals was measured and recorded daily.
Experimental design
A signal generator (Everest Comp., Adapazarı, Turkey), which could produce identical 3G GSM signal waveform at 2100 MHz, was used in the study to expose the rabbits to radiation. A typical mobile phone antenna with an omnidirectional radiation pattern in free space was connected to the generator. The peak transmitted power for the 3G modulated signal was 250 mW (24 dBm). The rabbits were confined in Plexiglas carousels and the carousels were evenly distributed around the antenna. The generator antenna was placed at the centre of the Plexiglas carousels to provide ideal exposure conditions. The distance of the antenna from the head of the rabbits was 1 cm. The experimental set-up is illustrated in a) and 1(b). The groups were formed by using 12 rabbits (6 for the experimental group and 6 for the control group). The rabbits from the first group were exposed to RF radiation for 3 h per day (7 d a week) for 4 weeks. For the control group, the rabbits were placed in the carousels and the same procedure was applied (3 h per day, 7 d a week for 4 weeks), except that the generator was turned off, i.e. no RF signal was present. All rabbits were kept under identical conditions for 4 weeks with free access to food and water.
Specific absorption rate (SAR) simulations
In the experimental set-up, the EMF values were measured with an electric-field probe EMR 300 (NARDA, Pfullingen, Germany) while the transmitter was operating to validate the intended transmit power. Then, the measured values were used in the EMF solver to find the field distribution inside the rabbit. Simulations were performed using CST Microwave Studio, which is a 3D EMF solver based on the finite integration technique (FIT).[Citation21] The FIT is similar to the well-known finite-difference time domain technique, but it utilizes discretization on general nonorthogonal grids using the integral form of Maxwell's equations rather than differential forms. It is claimed by CST that charge and energy conservation inherent to Maxwell's equations are preserved with FIT so that very stable numerical results in time domain can be obtained.[Citation21] Voxel (volumetric pixel) model of a rabbit was created by using the typical dimensions of a rabbit used in the experiments. Usually, detailed voxel models exist for humans and rats, but an electromagnetic rabbit model is almost nonexistent. The inner organs and tissue characteristics were inspired from those of a rat, which has a detailed voxel model. The rabbit model is shown in , where almost all of the inner organs (brain, intestine, heart, skin, muscle and bones) and nose, mouth and eyes were modelled. The titanium implant was modelled as a perfect electric conductor because the conductivity of titanium is large. The simulation model consisted of an electric field and SAR distribution inside and around the rabbit. The IEEE C95.3 standard was used for SAR averaging. Simulated electric field values were consistent with the measured electric field data that were obtained with the field probe. The 1-gram and 10-gram SAR values, when averaged over voxel size, were provided in for the chin with metal plate, the opposite side of the chin and the upper region of the nose. The maximum SAR values are also shown in .
Figure 2. Simulation set-up. (a) Rabbit model; (b) simulated rabbits; (c) cross-sectional view; (d) SAR distribution at 2100 MHz (10 g).
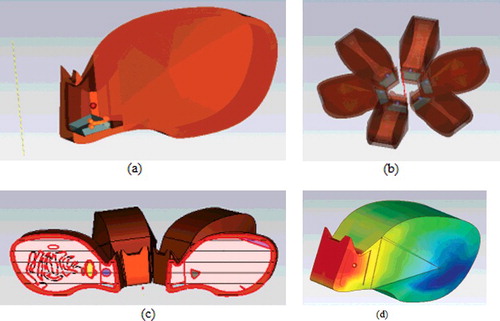
Table 1. SAR values at 2100 MHz.
Radiological density analysis
The mandibles of the rabbits in the experimental group were evaluated with extremity computed tomography (CT) (16-detector Toshiba Activion V3.00; 2010). The acquired images were evaluated together by a radiology expert on the extremity CT at the work station (Philips Extended Brilliance Workspace Philips Medical Systems, Best, the Netherlands). The images were first evaluated in axial sections. Then, 3D reformat views were obtained and sagittal and coronal sections were created and used when necessary. The thickness of the sections was 1 mm; 120 kV and 300 mA were used. Hounsfield unit (HU) measurements were performed in the bone window. The measurements were obtained by placing the region of interest on the area of the fracture in left mandibles. Measurements of the right mandibles were obtained in the same localization.
After the radiological measurements were performed, the hemi-mandibles with surrounding muscle tissue were stored in gauze pads soaked with normal saline at –20 °C until the time of biomechanical analysis.
Biomechanical analysis
Geometrical measurements and analyses
Geometrical measurements were performed on a computerized tomography equipment (Toshiba Aquilion 64 Slice CT) in the Department of Radiology, Mersin University. In the geometrical analysis, endosteal and periosteal dimensions and cortical cross sections were measured (mm) along the fracture line (at the level of the third molar: from 2.5 to 3 mm) in mediolateral and anteroposterior directions. The results were evaluated using Vitrea software (V 2.0, Minnetonka, MN, USA). The readings were repeated 10 times and the mean values of the parameters were calculated and used.
Measurements of bone biomechanics and analysis
Following the completion of geometrical measurements, biomechanical measurements and analyses of the left mandibles were performed. A mechanical test was performed on the bones after soaking them in 0.9% saline at a room temperature for 30 min. Since the measured and estimated parameters were affected by the temperature of the test medium, the temperature was held at 22 °C–24 °C. The bones were separated from the muscle tissue and the length of the mandibles (length between the incisor and gonion) were measured using a digital calliper (TESA, Renens, Switzerland). The bones were placed in the test equipment in a mediolateral direction with the buccal side up, after the distance between the bearings points was measured with the digital calliper. The level of the third molar on the mandibular mid-shaft was defined as the pressure point. The three-point bending test was performed on the bones by using MAY TPBM 2113 test equipment (Commat, Ankara, Turkey) (). The bending load was applied at a speed of 1 mm/s. The signals occurring as a result of the applied bending pressure were recorded using a 12 Bit analog-to-digital (A/D) converter with 1000 samples/s sensitivity. The strain rate in the three-points bending system was applied for 0.064 s. Only the bones fractured at the mandibular mid-shaft (third molar) were included in the study; the rest of the bones were excluded. Load-deformation curves were drawn and ultimate force (N), ultimate displacement (mm), energy absorption capacity (mj) and stiffness (N/mm) were obtained directly. These parameters were evaluated using LoggerPro software (V 3.8.3, Vernier Software & Technology, Orlando, FL, USA). The ultimate force is the amount of force observed at the moment of fracture. Ultimate displacement is the amount of deformation in the bone developed until the moment of fracture. Energy absorption capacity is the energy that the bone stored until the fracture occurred. Stiffness reflects the resistance of the bone against the applied force. In addition to the above parameters, maximum stress (MPa), maximum strain (mm/mm), elastic modulus (GPa) and toughness (MPa) values were calculated using a stress–strain curve. The maximum stress is defined as the maximum endurance. The maximum strain is the strain value observed at the moment of the fracture of the bone. Elastic modulus reflects the intrinsic toughness of the tissue and demonstrates the amount of deformation that can be applied to the bone against a stretch without inducing permanent damage. The toughness reflects the amount of energy required to fracture the bone.
Histopathological analyses
For the histopathological assessment (Nikon, Eclipse 80i microscope, Japan), all fractured mandibles were fixed in 10% (v/v) buffered formalin solution (MKS Marmara Kimya, İstanbul, Turkey) for a period of 2 weeks. After fixation, the mandibles were decalcified in 10% (v/v) nitric acid (MKS Marmara Kimya, İstanbul, Turkey). The samples were then embedded in paraffin blocks and after 3–4 micron thick sections were taken, they were stained with haematoxylin and eosin. For the evaluation of fracture healing, the histopathological improvement scale was used,[Citation22] in which scoring is conducted over 10 points (). The slides were evaluated by one pathologist to ensure standardization. In bilateral group comparisons, a decrease in the score was assigned as a negative effect on fracture healing, whereas an increase was evaluated as a positive effect.
Table 2. Histopathological scoring for the evaluation of fracture healing.
Statistical analyses
The data were summarized by mean, standard deviation, minimum and maximum values. Shapiro–Wilk test was used to control normal distribution. Then, independent t-test was used to compare control and RF exposed groups for geometrical properties of the bone and biomechanical measurements. Mann–Whitney U-test was used to analyse histopathological and radiological data. Significant differences (two-tailed p-values) less than 0.05 were considered as statistically significant.
Results and discussions
The rabbits were observed to lose approximately 10% of their weight during the early postoperative periods. All rabbits reached their previous weights at the end of the study. None of the rabbits were lost during the postoperative period.
Radiological analysis
The results of the statistical analyses of the data obtained by computerized tomography examination are shown in . No statistically significant differences were found between the left and right mandibles’ results and no differences (%) were found between the left and right mandibles’ values in the experimental and control groups (p > 0.05).
Table 3. Radiological comparison of the left and right mandibles between the experimental and control groups.
Geometrical and biomechanical analyses
Geometrical properties of the mandibular bones in this study are demonstrated in . According to the obtained results, no statistically significant differences were found between the cross-section areas (mm2) of the right and left mandibular bones. No statistically significant differences were found between the length (mm) and cross-section parameters of the left mandibular bones (p > 0.05). The results of the statistical analysis of the parameters obtained from the biomechanical test are described in . When the left mandibles of the control and experimental groups were compared, no statistically significant differences were found in terms of stiffness, ultimate force, ultimate displacement, elastic modulus and maximum strain values between the groups (p > 0.05), whereas statistically significant differences were found between the energy absorption capacity, toughness and maximum stress values in the two groups (p < 0.05). Energy absorption capacity (62.4%), toughness (71.9%) and maximum stress (53.5%) values increased in the experimental group when compared to the control group.
Table 4. Geometric properties of the left mandible bone in the control and experimental groups.
Table 5. Comparison of biomechanical parameters of mandibular bones with osteotomy between the two groups.
Histopathological analysis
Statistical comparisons of the histopathological score values are demonstrated in . There was a statistically significant increase in the scores of the experimental group when compared to the scores of the control group (p < 0.05) ().
Table 6. Histopathological comparison of the fracture lines in the mandibular bones with osteotomy in experimental and control groups.
Figure 4. (a) Histopathological images of the samples from a rabbit in the control group with bone healing score 2 and (b) a rabbit in the experimental group with bone healing score 8.
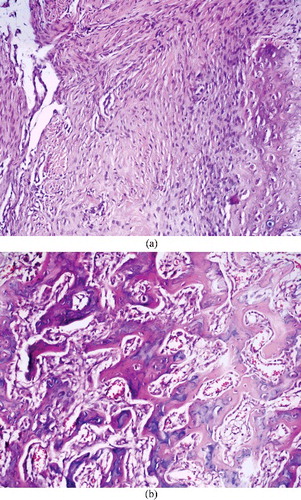
There have been numerous studies published on the effects of EMFs on bone tissue and fracture healing using various low-to-moderate frequencies, various power levels and waveforms, often with different results.[Citation23–25] However, there are few studies on the effects of EMF radiated from mobile phones on bone and fracture healing.[Citation26,Citation27] The effects of 900 MHz EMF (30 min a day, 5 d a week for 8 weeks) were evaluated on fracture healing in a rat tibia fracture model,[Citation26] and it was found that the fracture healing in the experimental group was worse in terms of radiology, histology and mechanics compared to the control group. In another study, the effects of a 1800 MHz EMF (30 min a day, 5 d a week for 8 weeks) on fracture healing in a rat tibia fracture model was also studied.[Citation27] No statistically significant differences were seen between the groups in terms of bone healing and it was reported that 1800 MHz mobile phone-like EMF had no effect on bone healing. These two studies were performed on long bones. However, very few studies have focused on the effects of mobile phone frequencies, particularly 2100 MHz, on tissues.[Citation28,Citation29] In a study performed by using 1900–2100 MHz frequency band, it was claimed that mobile phone exposure induced p53 gene and Bcl-2 gene expression in cerebral tissue, which might have affected apoptosis and triggered cerebral carcinogenesis in rats.[Citation28] Dasdag et al. [Citation29] stated that the final score for apoptosis, total antioxidant capacity and catalase in rat brain might be altered by 900 MHz RF radiation. However, Yılmaz et al. [Citation30] reported that RF radiation emitted from 900 MHz cellular phones did not alter antiapoptotic bcl-2 protein in the brain and testes of rats. In a different study, it was reported that 1800 and 2100 MHz EMF radiation had no effect on exfoliated bladder cells in rats.[Citation31] However, 3G mobile phone radiation at 2100 MHz was found to induce many histopathological changes in parotid gland of rats, but no nuclear changes were observed.[Citation32] There are no previously published studies in the literature on the effects of a 2100 MHz EMF on fracture healing in the maxillofacial region. In this respect, this study evaluated the effects of EMF at 2100 MHz on bone fracture healing.
One of the most important methods for the evaluation of fracture healing in bone tissue is the analysis of the biomechanical test results. The ultimate force, ultimate displacement, stiffness and energy absorption capacity are important parameters for bone biomechanics. These parameters reflect the extrinsic structural properties of the bone tissue. In this study, the energy absorption capacity was statistically significantly higher in the group exposed to EMF compared to the control group as a result of the biomechanical test. An increase in the energy absorption capacity demonstrated that a 2100 MHz mobile phone-like EMF increases the bone strength and thus positively affects the bone healing. Maximum stress, maximum strain, elastic modulus and toughness are the other important parameters reflecting bone endurance and intrinsic properties by biomechanical tests. These parameters are strongly associated with the collagen component of the bone.[Citation33,Citation34] In biomechanical evaluation, combinational changes between maximum stress, maximum strain, elastic modulus and toughness indicate various problems, including increased or decreased bone mineralization and/or collagen degradation.[Citation35] While the maximum strain was constant in the studies that we performed, toughness and maximum stress increased within statistical significance. This increase demonstrated that a 2100 MHz GSM-like EMF increased the bone strength by affecting the collagen integrity.
Osteoblasts are one of the most important cells on which the studies of the EMF on fracture healing are conducted. Pulsed EMF was demonstrated to increase the proliferation and differentiation in osteoblasts in numerous studies.[Citation36,Citation37] On the contrary, in an in vitro study, it was reported that 50 Hz 1.8 mT and 3.6 mT sinusoidal EMF inhibited the osteoblast proliferation and increased osteoblast differentiation and mineralization.[Citation38] In another in vitro study, it was reported that 50 Hz EMF at 0.8 mT increased the intracellular free calcium (Ca2+) level in osteoblasts.[Citation39] Intracellular free calcium has a role as a second messenger in the cell and plays a part in the regulation of many physiological functions. An increase in the intracellular Ca2+ is associated with the differentiation and maturation of the osteoblasts and is reported to increase the osteogenic activity.[Citation38] Our biomechanical test results also indicated a similar improved bone healing by activating osteoblasts when rabbits were exposed to 2100 MHz EMF.
The fracture performed in this study was repaired by using a titanium alloy plate screw. Numerical SAR simulations of human head with metallic plate were performed before,[Citation40] and metal plate dimensions were one third of the applied RF wavelength, which resulted in resonance peaks at the absorbed electric field. In our study, the dimensions of the implant were much smaller than the RF exposure wavelength, which, in turn, did not result in resonance peaks in electric field distribution. The interaction of the EMF with the used plate is an important issue and it has been reported that different results might occur with different electromagnetic wave types (pulsed vs. continuous). In some studies, various RF exposures have been reported to result in burns in the tissues secondary to the increased temperature on the implanted metals.[Citation41] It was reported that 900 and 1800 MHz plane waves can result in temperature rises around the implant with less than 0.25 °C and 0.10 °C, respectively.[Citation42] On the other hand, compared to stainless steel, titanium alloy, which is frequently preferred in medical implants, have low magnetic permeability and electric conductivity. Therefore, lesser increases in temperature have been reported secondary to RF applications around implants with titanium alloy.[Citation43,Citation44] This condition suggests that titanium and titanium alloy plate screws may not develop tissue damage with the effect of increased temperature. We also have not observed any tissue damages or tissue burns in the surrounding tissue of the titanium plate when exposed to 2100 MHz RF radiation.
In this study, there were no statistical differences between the experimental and control groups in the HU measurements but the within group variability (20%–40%) did not permit us to form reliable conclusions. In addition, all animals were sacrificed on the 28th postoperative day and tests were performed to evaluate the fracture healing. The 28th postoperative day is within the period in which callus tissue is still immature. The fact that no differences were found in the HU between the groups, whereas there were statistically significant differences between the groups in the biomechanical and histopathological examinations, suggested that measurement of HU on the 28th postoperative day might be inadequate for the evaluation of fracture healing or a more detailed analysis using micro-CT was needed.
To the best of our knowledge, this is the first study investigating the effects of 2100 MHz electromagnetic radiation exposure on mandibular fracture healing with assessments on radiological, histopathological and biomechanical parameters in detail.
Conclusions
This study showed that a 2100 MHz EMF positively affects fracture healing, according to the biomechanical test results and the obtained histopathological parameters. The 2100 MHz EMF radiation is widely used in various applications, like in wireless and mobile communications. Therefore, the effects of the RF radiation on different human tissues need further investigations.
Disclosure statement
No potential conflict of interest was reported by the authors.
Additional information
Funding
References
- Shahim FN, Cameron P, McNeil JJ. Maxillofacial trauma in major trauma patients. Aust Dent J. 2006;51(3):225–230.
- Ghodke MH, Bhoyar SC, Shah SV. Prevalence of mandibular fractures reported at C.S.M.S.S Dental College, Aurangabad from February 2008 to September 2009. J Int Soc Prev Community Dent. 2013;3(2):51–58.
- Singh V, Malkunje L, Mohammad S, et al. The maxillofacial injuries: a study. Natl J Maxillofac Surg. 2012;3(2):166–171.
- Einhorn TA. Enhancement of fracture-healing. J Bone Joint Surg Am. 1995;77(6):940–956.
- Lai CH, Chuang CC, Li JK, et al. Effects of ultrasound on osteotomy healing in a rabbit fracture model. Ultrasound Med Biol. 2011;37(10):1635–1643.
- Tarantino U, Cerocchi I, Celi M, et al. Pharmacological agents and bone healing. Clin Cases Miner Bone Metab. 2009;6(2):144–148.
- Capone A, Orgiano F, Pianu F, et al. Orthopaedic surgeons' strategies in pharmacological treatment of fragility fractures. Clin Cases Miner Bone Metab. 2014;11(2):105–109.
- Pountos I, Georgouli T, Blokhuis TJ, et al. Pharmacological agents and impairment of fracture healing: what is the evidence? Injury. 2008;39(4):384–394.
- Hronik-Tupaj M, Rice WL, Cronin-Golomb M, et al. Osteoblastic differentiation and stress response of human mesenchymal stem cells exposed to alternating current electric fields. Biomed Eng Online. 2011;10:9. doi:10.1186/1475-925X-10-9
- Qaddoumi N, Al-Nashash H, Sediq AB, et al. Towards an assessment of bone fracture healing using pulsed mode ultrasound. Technol Health Care. 2011;19(4):261–269.
- Teven CM, Greives M, Natale RB, et al. Differentiation of osteoprogenitor cells is induced by high-frequency pulsed electromagnetic fields. J Craniofac Surg. 2012;23(2):586–593.
- Liu Y, Wei X, Kuang Y, et al. Ultrasound treatment for accelerating fracture healing of the distal radius. A control study. Acta Cir Bras. 2014;29(11):765–770.
- Bassett CA, Pawluk RJ, Pilla AA. Augmentation of bone repair by inductively coupled electromagnetic fields. Science. 1974;184(4136):575–577.
- De Haas WG, Lazarovici MA, Morrison DM. The effect of low frequency magnetic fields on the healing of the osteotomized rabbit radius. Clin Orthop Relat Res. 1979;145:245–251.
- Fredericks DC, Piehl DJ, Baker JT, et al. Effects of pulsed electromagnetic field stimulation on distraction osteogenesis in the rabbit tibial leg lengthening model. J Pediatr Orthop. 2003;23(4):478–483.
- Inoue N, Ohnishi I, Chen D, et al. Effect of pulsed electromagnetic fields (PEMF) on late-phase osteotomy gap healing in a canine tibial model. J Orthop Res. 2002;20(5):1106–1114.
- Shi HF, Xiong J, Chen YX, et al. Early application of pulsed electromagnetic field in the treatment of postoperative delayed union of long-bone fractures: a prospective randomized controlled study. BMC Musculoskelet Disord. 2013;14:35. doi:10.1186/1471-2474-14-35
- Marino AA, Cullen JM, Reichmanis M, et al. Fracutre healing in rats exposed to extremely low-frequency electric fields. Clin Orthop Relat Res. 1979;145:239–244.
- Atay T, Aksoy BA, Aydogan NH, et al. Effect of electromagnetic field induced by radio frequency waves at 900 to 1800 MHz on bone mineral density of iliac bone wings. J Craniofac Surg. 2009;20(5):1556–1560.
- Dasdag S, Akdag MZ, Kizil G, et al. Effect of 900 MHz radio frequency radiation on beta amyloid protein, protein carbonyl, and malondialdehyde in the brain. Electromagn Biol Med. 2012;31(1):67–74.
- Marklein R. Ultrasonic wave and transducer modelling with the finite integration technique (FIT). In: Yuhas DE, Schneider SC, editors. Proceedings of the IEEE International Ultrasonic Symposium; 2002 Oct 8--11; Munich. Piscataway (NJ): Institute of Electrical and Electronic Engineers; 2002.
- Huo MH, Troiano NW, Pelker RR, et al. The influence of ibuprofen on fracture repair: biomechanical, biochemical, histologic, and histomorphometric parameters in rats. J Orthop Res. 1991;9(3):383–390.
- Gurgul S, Erdal N, Yilmaz SN, et al. Deterioration of bone quality by long-term magnetic field with extremely low frequency in rats. Bone. 2008;42(1):74–80.
- Akdag MZ, Dasdag S, Erdal N, et al. The effect of long-term extremely low-frequency magnetic field on geometric and biomechanical properties of rats' bone. Electromagn Biol Med. 2010;29(1–2):9–18.
- Fredericks DC, Nepola JV, Baker JT, et al. Effects of pulsed electromagnetic fields on bone healing in a rabbit tibial osteotomy model. J Orthop Trauma. 2000;14(2):93–100.
- Aslan A, Atay T, Gulle K, et al. Effect of 900MHz electromagnetic fields emitted from cellular phones on fracture healing: an experimental study on rats. Acta Orthop Traumatol Turc. 2013;47(4):273–280.
- Aslan A, Kirdemir V, Kocak A, et al. Influence of 1800 MHz GSM-like electromagnetic radiation exposure on fracture healing. Arch Med Res. 2014;45(2):125–131.
- Yilmaz A, Yilmaz N, Serarslan Y, et al. The effects of mobile phones on apoptosis in cerebral tissue: an experimental study on rats. Eur Rev Med Pharmacol Sci. 2014;18(7):992–1000.
- Dasdag S, Akdag MZ, Ulukaya E, et al. Effect of mobile phone exposure on apoptotic glial cells and status of oxidative stress in rat brain. Electromagn Biol Med. 2009;28(4):342–354.
- Yilmaz F, Dasdag S, Akdag MZ, et al. Whole-body exposure of radiation emitted from 900 MHz mobile phones does not seem to affect the levels of anti-apoptotic bcl-2 protein. Electromagn Biol Med. 2008;27(1):65–72.
- Gurbuz N, Sirav B, Colbay M, et al. No genotoxic effect in exfoliated bladder cells of rat under the exposure of 1800 and 2100 MHz radio frequency radiation. Electromagn Biol Med. 2014;33(4):296–301.
- Aydogan F, Unlu I, Aydin E, et al. The effect of 2100 MHz radiofrequency radiation of a 3G mobile phone on the parotid gland of rats. Am J Otolaryngol. 2015;36(1):39–46.
- Reddy GK, Stehno-Bittel L, Hamade S, et al. The biomechanical integrity of bone in experimental diabetes. Diabetes Res Clin Pract. 2001;54(1):1–8.
- Turner CH. Biomechanics of bone: determinants of skeletal fragility and bone quality. Osteoporos Int. 2002;13(2):97–104.
- Burr DB. The contribution of the organic matrix to bone's material properties. Bone. 2002;31(1):8–11.
- Barnaba S, Papalia R, Ruzzini L, et al. Effect of pulsed electromagnetic fields on human osteoblast cultures. Physiother Res Int. 2013;18(2):109–114.
- Jansen JH, van der Jagt OP, Punt BJ, et al. Stimulation of osteogenic differentiation in human osteoprogenitor cells by pulsed electromagnetic fields: an in vitro study. BMC Musculoskelet Disord. 2010;11:188. doi:10.1186/1471-2474-11-188
- Zhou J, Ming LG, Ge BF, et al. Effects of 50 Hz sinusoidal electromagnetic fields of different intensities on proliferation, differentiation and mineralization potentials of rat osteoblasts. Bone. 2011;49(4):753–761.
- Zhang X, Liu X, Pan L, et al. Magnetic fields at extremely low-frequency (50 Hz, 0.8 mT) can induce the uptake of intracellular calcium levels in osteoblasts. Biochem Biophys Res Commun. 2010;396(3):662–666.
- McIntosh RL, Anderson V, McKenzie RJ. A numerical evaluation of SAR distribution and temperature changes around a metallic plate in the head of a RF exposed worker. Bioelectromagnetics. 2005;26(5):377–388.
- Ye D, Xu Y, Zhang H, et al. Effects of low-dose microwave on healing of fractures with titanium alloy internal fixation: an experimental study in a rabbit model. PLOS One. 2013;8(9):e75756.
- Mattei E, Calcagnini G, Censi F, et al. Radiofrequency dosimetry in subjects implanted with metallic straight wires: a numerical study. Conf Proc IEEE Eng Med Biol Soc. 2008;2008:4387–4390.
- Huidobro C, Larson B, Mynderse S, et al. Characterizing Prostiva RF treatments of the prostate for BPH with gadolinium-enhanced MRI. Sci World J. 2009;9:10–16.
- Muranaka H, Horiguchi T, Ueda Y, et al. Evaluation of RF heating on hip joint implant in phantom during MRI examinations. Nihon Hoshasen Gijutsu Gakkai Zasshi. 2010;66(7):725–733.