Abstract
The head is the body part that is most exposed to radiofrequency radiation (RFR) during a mobile phone conversation. Therefore, it can be expected that brain cells will be positively or negatively affected by this physical agent. The purpose of this study was to investigate the effects of 900, 1800 and 2100 MHz on the interstitial space between cells in the right and left lobes of the brain. The study was carried out on 28 Wistar Albino rats, which were divided randomly into four groups (n: 7): sham control, 900 MHz, 1800 MHz and 2100 MHz exposure groups. The rats in the exposure groups were subjected to RFR for 3 h/day for one month. At the end of the last exposure, brains were immediately removed and prepared for electron microscopic examination. We determined the interstitial space (µm) between brain cells in the left and right lobes separately and compared them statistically. The results indicated that all three frequencies used in this study increased the interstitial space between cells in both brain lobes. The maximum effective frequencies were 1800 MHz for the right lobe and 2100 MHz for the left lobe. In conclusion, we observed that the RFR used in this study enhanced the interstitial space between cells in both lobes of the brain. Further studies are needed to confirm the results of this study, which we think will open different horizons.
Introduction
It is known that there are many mechanistic agents that affect brain cells, functions and regulations. For instance, it is suggested that the distance between brain cells increases during sleep, so that more fluid flows into the intercellular space, and in this way, it is possible for molecules such as amyloid-beta (Aβ) to move away from the environment [Citation1]. However, there are many other agents, including physical ones, that affect brain cell functions. For example, while talking on mobile phones, the relation between RFR and brain cells or intercellular distance is not yet clear enough. However, increasing or decreasing the interstitial space between brain cells when exposed to RFR is very important for brain and body health. The increase or decrease in the interstitial space between brain cells due to RFR exposure can open new horizons in terms of the cause or treatment of diseases. Because the most RFR-exposed area is the head when a person is talking on a mobile phone, one of the most controversial issues is the effects of RFR emitted from wireless communications on the brain. Considering that the frequencies used by communication service providers are different, the issue should be handled and evaluated accordingly. Therefore, it becomes important to know which frequency (900, 1800, and 2100 MHz) changes the interstitial space between brain cells.
In the study which is the source of the main idea of this research, Xie et al. showed that ‘natural sleep or anesthesia is associated with a 60% increase in interstitial space, resulting in a dramatic increase in the convective exchange of cerebrospinal fluid with interstitial fluid’ [Citation1]. The same group also suggested that ‘convective flows of interstitial fluid increase the rate of Aβ clearance during sleep and that the restorative function of sleep may be related to the removal of potentially neurotoxic waste products that accumulate in the waking central nervous system’. Suggesting that sleep plays an important role in the healthy functioning of the brain and even in the prevention of diseases such as Alzheimer’s paved the way for determining whether other factors have similar roles. When the subject is associated with brain health and therefore Alzheimer’s disease (AD), it is necessary to take a look at the studies on whether RFRs cause AD.
Arendash et al. claimed that ‘long-term radiofrequency radiation (RFR) exposure (918 MHz; 0.25 W/kg) reduced brain amyloid-beta (Aβ) deposition through Aβ anti-aggregation actions and increased brain temperature during exposure periods in AD mice’ [Citation2]. Similarly, Tsoy et al. also reported the potential of RFR to reduce Aβ and some oxidative parameters in the brain [Citation3], whereas, we previously did not find that 900 MHz RFR altered the Aβ level in the brain and contrarily observed that protein carbonyl level, which is an indicator of protein oxidation, increased in the brain of exposed rats [Citation4]. According to Arendash, ‘long-term transcranial electromagnetic treatment (TEMT), which differs from transcranial direct current stimulation (tDCS) and transcranial magnetic stimulation (TMS), has the potential to prevent and reverse both cognitive impairment and brain Aβ deposition, including improving cognitive performance’ [Citation5]. In a review published by Dasdag O et al. it is emphasized that different theses or opposing views are still valid in the relationship between RF electromagnetic fields and AD and many studies are needed to clarify the subject [Citation6]. As outlined in this review, many researchers suggest that some electromagnetic radiation has prepared the ground for AD, while others claim that RFR has a positive effect on it. As noted, the frequency, exposure time and other characteristics of RFRs should be naturally handled separately. This is because the effective range of RFRs may vary depending on the application time and other physical parameters mentioned here [Citation6].
Another point that should not be ignored in studies investigating the effects of RFRs is what kind of effects occur in the right and left lobes of the brain. For this reason, it is also important to know whether the interstitial space between cells in the right and left lobes of the brain exposed to RFRs changes in similar or different ways.
The aim of this research was to contribute to the subject discussed above. Therefore, the purpose of this study was to investigate the effects of 900, 1800 and 2100 MHz RFRs on the interstitial space between cells of the rat brain. We also aimed to reveal whether there is a difference between the left and right lobes of the brain in terms of the parameters mentioned above.
Materials and methods
Ethics statement
This study was approved by the Dicle University Aimal Experiments Local Ethics Committee (DÜBAP-TIP.15.004).
Animals and RFR exposure
Twenty-eight Wistar Albino adult male rats were acquired from the Medical Science Application and Research Center of Dicle University. They were separated equally into four groups: sham control (n: 7), 900 MHz, 1800 MHz and 2100 MHz exposure groups (n: 7 for each experimental group), and kept on a 14/10 h light/dark schedule. The rats were confined in different Plexiglas carousels and exposed to the RFRs mentioned above by means of three signal generators. The carousel was surrounded with electromagnetic absorber material backed by metal to isolate outdoor electromagnetic fields from the test set-up during the study duration of 1 month. The experimental set-up is illustrated in [Citation7].
For the exposure groups, the rats were exposed to RFR for 3 h per day for 1 month. For the sham control group, the rats were placed in the carousel and the same procedure was applied to the rats, except that the generator was turned off, i.e. no RF signal was present. The antenna of the generator was placed at the center of the plexiglas carousel to provide ideal exposure conditions. The distance between the antenna and the heads of the rats was approximately 1 cm.
All rats were kept under identical conditions for one month with free access to food and water. At the end of one month, the rats were intraperitoneally administered a combination of 6 mg/kg of 2% xylazine hydrochloride (Rompun) and 75 mg/kg ketamine hydrochloride (Ketalar) for anesthesia. At the end of the study, left and right lobes were removed and prepared for electron microscopic examination to determine the distances between the cells and the percentages of cytoplasmic areas. To prevent observer bias, the researchers who performed all measurements, analysis and evaluations were blinded to the experimental groups. The distance between the brain cells and cytoplasmic spaces was evaluated by the method defined below.
Transmission electron microscopy (TEM)
For electron microscopic evaluation, after RFR exposure, temporal cortexes were dissected from each rat. Brain samples were fixed with 4% glutaraldehyde and then post-fixed with 2% osmium tetraoxide in 0.1 mol/L Sorensen’s phosphate buffer solution (pH 7.3). After dehydration through a graded series of ethanol, they were embedded in an epoxy resin (Araldite CY212, Agar Scientific Ltd, Stansted, UK). Semi-thin (1 mm) and ultra-thin (40–60 nm) sections were cut with an ultramicrotome (Leica ultracut, UCT, Leica MZ6, England). Semi-thin sections (1 µm) were stained with toluidine blue and examined with a light microscope (Olympus CX41). Afterwards, ultrathin sections (40–60 nm) were contrasted with uranyl acetate and lead citrate and were examined with a transmission electron microscope (Zeiss LEO 906E TEM, Oberkochen, Germany), as previously described [Citation8].
Measurement of interstitial space, cytoplasmic area and neuron area
Transmission electron micrographs were taken in the temporal cortex at a magnification of about 1000x for interstitial distance between neurons and about 4000x for neuron and cytoplasm area measurements, with an exposure time of 500 ms. Image processing and analysis were performed by analySIS software (Soft Imaging System GmbH, Leinfelden-Echterdingen, Germany). In each group, measurements were taken from at least 10 different areas of the left and right lobes, which were randomly chosen and the mean values were used for statistical analysis. We examined the interstitial space in the temporal cortex between two adjacent neuron cells. The distances between all pairs of neurons in electron micrographs were measured. Neuron cytoplasm areas were calculated by subtracting nucleus areas from the total neuron areas. The percentage of cytoplasmic area was calculated according to the formula: (Cytoplasm area/Neuron cell area) * 100.
Data analysis
The results are expressed as the mean values with standard deviation (±SD). Statistical significance was determined at a level of p < 0.05. The differences between the groups were analyzed with one-way analysis of variance (ANOVA) and the Holm-Sidak post-hoc test. When the normality test failed, Kruskal–Wallis one-way ANOVA on ranks, followed by Dunn’s method for pairwise multiple comparison, was performed.
Results
Our results showed that the interstitial space between the cells in the right brain lobe of rats exposed to 900, 1800 and 2100 MHz frequency RFRs increased compared to the sham control group ( and ). We also observed that the effective frequency which increased the the interstitial space the most was 1800 MHz, whereas the effective frequency that increased the interstitial space the least was 900 MHz.
Figure 2. Interstitial space between neurons in right lobes of rats in all groups. RFR groups compared with respect to C-RB group respectively. *p = 0.017, **p < 0.001, ***p < 0.001. C-RB: Sham control-right brain lobes, 900 MHz-RB: 900 MHz-right brain lobes, 1800 MHz-RB: 1800 MHz-right brain lobes, 2100 MHz-RB: 2100 MHz-right brain lobes.
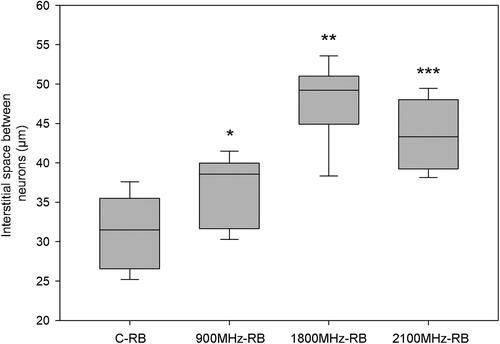
We similarly observed that all three frequencies increased the interstitial space between the cells in the left lobe ( and ). The most effective frequency to increase the interstitial space between the cells was 2100 MHz, and 900 MHz was the frequency that least increased it. On the other hand, we did not observe any alterations in the area of cytoplasm (%) in any of the RFR exposure groups.
Figure 3. Interstitial space between neurons in left lobes of rats in all groups. RFR groups compared with respect to C-LB group respectively. *p < 0.001, **p = 0.002, ***p < 0.001. C-LB: Sham control-left brain lobes, 900 MHz-LB: 900 MHz- left brain lobes, 1800 MHz-LB: 1800 MHz- left brain lobes, 2100 MHz-LB: 2100 MHz- left brain lobes.
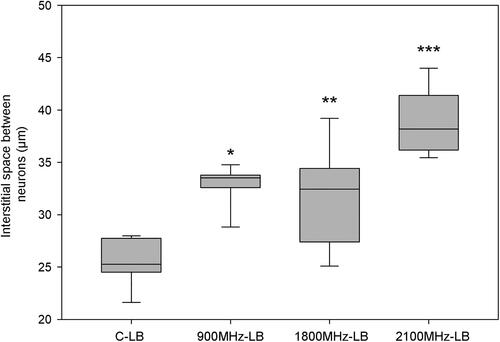
Interestingly, there was difference in the interstitial space between the neurons in the right and left brain lobe of the sham control group (). The interstitial space between the cells in right lobe of the sham control group (C-RB) was about 30 μm, while the space between the cells in the left lobe of the sham control group (C-LB) was about 25 μm. Similarly, when the interstitial spaces between the neurons in the RFR groups were compared separately, significant differences were observed between the right and left lobes in all groups (). In all RFR frequencies, the interstitial spaces between neurons in the right brain lobe were higher than those in the left brain lobe.
Figure 4. Comparison of the groups for the right and left brain lobes in terms of interstitial space between neurons. Each group was compared for the right and left brain lobes. *p = 0.012, **p = 0.029, ***p < 0.001, ****p = 0.026. C-RB: Sham control-right brain lobes, C-LB: Sham control-left brain lobes, 900 MHz-RB: 900 MHz-right brain lobes, 900 MHz-LB: 900 MHz- left brain lobes, 1800 MHz-RB: 1800 MHz-right brain lobes, 1800 MHz-LB: 1800 MHz- left brain lobes, 2100 MHz-RB: 2100 MHz-right brain lobes, 2100 MHz-LB: 2100 MHz- left brain.
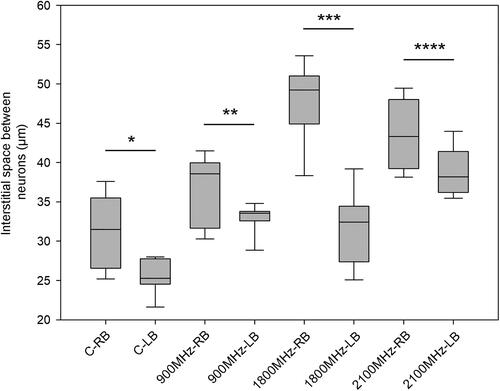
When the results were evaluated in terms of the most effective frequency that increased the interstitial space between cells, it was also observed that the effective frequency (1800 MHz) in the right lobe increased the interstitial space (approx. 16 µm) to a greater extent than the effective frequency (2100 MHz) did in the left lobe (approx. 11 µm).
The area of cytoplasm (%) revealed no significant differences between the sham control group and RFR groups in the percentage of cytoplasm areas in the right and left lobe ( and ). Similarly, when the percentages of cytoplasm areas in the groups were compared separately, no significant differences were observed between the right and left brain lobes in all groups ().
Figure 5. Area of cytoplasm (%) of neurons in the right brain lobes of rats in all groups. RFR groups compared with respect to the C-RB group, respectively. The differences between the groups are non-significant (C-RB vs. 900 MHz-RB p = 0.757; C-RB vs, 1800 MHz-RB p = 0.211; C-RB vs. 2100 MHz-RB p = 0.108). C-RB: Sham control-right brain lobes, 900 MHz-RB: 900 MHz-right brain lobes, 1800 MHz-RB: 1800 MHz-right brain lobes, 2100 MHz-RB: 2100 MHz-right brain lobes.
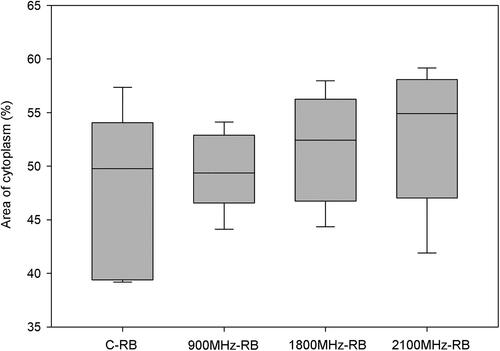
Figure 6. Area of cytoplasm (%) of neurons in the left brain lobes of rats in all groups. RFR groups compared with respect to the C-LB group, respectively. The differences between the groups are non-significant (C-LB vs. 900 MHz-LB p = 0.180; C-LB vs. 1800 MHz-LB p = 0.747; C-LB vs. 2100 MHz-LB p = 0.757). C-LB: Sham control-left brain lobes, 900 MHz-LB: 900 MHz- left brain lobes, 1800 MHz-LB: 1800 MHz- left brain lobes, 2100 MHz-LB: 2100 MHz- left brain lobes.
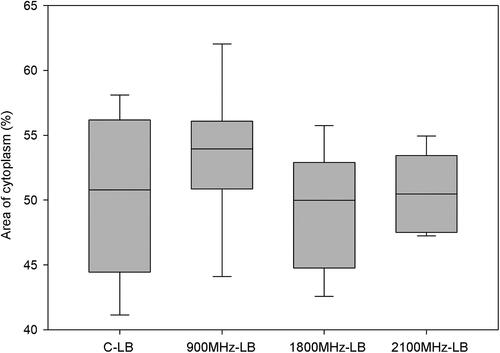
Figure 7. Comparison of the groups for the right and left brain lobes in terms of area of cytoplasm (%) of neurons. Each group was compared for the right and left brain lobe (C-RB vs. C-LB p = 0.475; 900 MHz-RB vs. 900 MHz-LB p = 0.055; 1800 MHz-RB vs. 1800 MHz-LB p = 0.320; 2100 MHz-RB vs. 2100 MHz-LB p = 0.145). C-RB: Sham control-right brain lobes, C-LB: Sham control-left brain lobes, 900 MHz-RB: 900 MHz-right brain lobes, 900 MHz-LB: 900 MHz- left brain lobes, 1800 MHz-RB: 1800 MHz-right brain lobes, 1800 MHz-LB: 1800 MHz- left brain lobes, 2100 MHz-RB: 2100 MHz-right brain lobes, 2100 MHz-LB: 2100 MHz- left brain lobes.
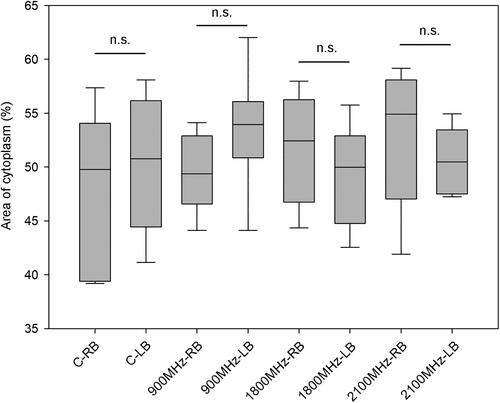
Figure 8. Representative TEM images of the right brain temporal cortex. lobes. Sham control group (A), 900 MHz exposure group (B), 1800 MHz exposure group (C), 2100 MHz exposure group (D). Scale bars = 10 µm (A, B, C, D).
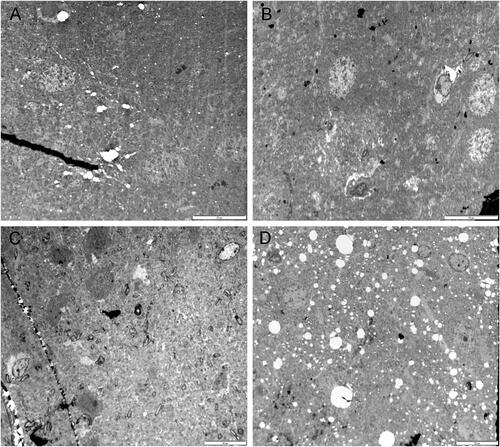
Figure 9. Representative TEM images of the left brain temporal cortex. Sham control group (A), 900 MHz exposure group (B), 1800 MHz exposure group (C), 2100 MHz exposure group (D). Scale bars = A:5 µm (A); 10 µm (B, C, D).
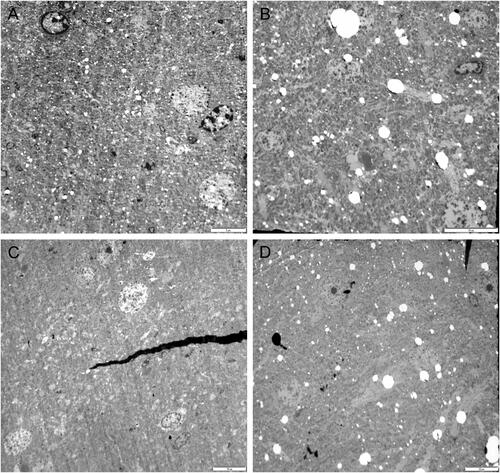
Additionally, when we measured the neuron and cytoplasm areas in the right and left brain, the neuron and cytoplasm areas in the RFR groups decreased compared to the sham control groups, but these decrements were not statistically significant ( and ). The results on the cytoplasm and neuron cell areas also indicated that these three RF frequencies have the potential to create shrinkage in the left and right lob brain cells.
Table 1. Right brain neuron and cytoplasm areas of the groups.
Table 2. Left brain neuron and cytoplasm areas.
Discussion
One of the inspirations for planning this study was that ‘Natural sleep or anesthesia produces a 60% increase in interstitial space’ reported by Xie et al. [Citation1]. They reported a dramatic increase in the convective exchange of cerebrospinal fluid with interstitial fluid and also suggested that convective flows of interstitial fluid increase the rate of Aβ clearance during sleep. Therefore, they emphasized that the therapeutic role of sleep may be related to the removal of neurotoxic waste products accumulated in the waking central nervous system. If this evidence is confirmed, increasing or decreasing the interstitial space between brain cells could make significant contributions to the treatment of brain-related diseases. Another piece of research that inspired our study was the work of Arendash et al. [Citation2]. They reported that long-term EMFs with a frequency of 918 MHz and a SAR value of 0.25 W/kg reduced brain Aβ accumulation through Aβ anti-aggregation actions and increased brain temperature during exposure times in mice with Alzheimer’s disease.
Thus, we investigated the effects of exposure to 900 MHz RFR to investigate whether long-term RFR has the potential to change the amount of Aβ in the brain, but we observed that RFR did not change the Aβ level. Another result we obtained in our previous study was that long-term 900 MHz RFR (2 h/day; 7 days/week; for 10 months) increased the protein carbonyl level in the brain [Citation4]. Our results did not confirm the findings of Arendash et al. and the fact that long-term RFR increased protein oxidation in the brain led us to different studies. This showed that RFR can have both positive and negative effects at the same time, and this could have important implications. This also suggested that RFR applications could have double-edged effects.
In 2019, Tsoy et al. suggested that RFRs reduce Aβ [Citation3]. The fact that there is a relationship between Aβ deposition and Alzheimer’s disease prompted us to examine and review the subject further [Citation6]. We observed that the relationship between RFR exposure and AD is still a controversial issue, and we found that gaining insight into that issue would be of great interest to the scientific community. Some of the researchers still suggested that electromagnetic fields might predispose to AD, while others suggested that they might play a role in treating AD [Citation6]. The idea that RFRs might increase the interstitial space between brain cells and therefore allow more incoming intercellular fluid to scavenge Aβ molecules needed further investigation. Therefore, in this study, we aimed to find out whether RFRs with frequencies of 900, 1800 and 2100 MHz could result in increased interstitial space.
We observed electron microscopically that all three frequencies increased the interstitial space in this study ( and ). As an original approach in our study, we investigated the interstitial space not only in one lobe of the brain but also in both the right and left lobes. An increase in fluid was detected in the interstitial space in RFR groups in a previous study performed on 900 MHz RFR on rats [Citation8]. Our finding of an increase in interstitial space in this study appears to be consistent with the previous findings of an increase in fluid in the interstitial space. It was interesting that all three frequency RFRs used in this study increased the interstitial spaces, but also in the sham control group the interstitial spaces in the right and left lobes of the brain were different from each other. To the best of our knowledge, there are no previous reports that the interstitial spaces between the cells of the two lobes of a normal brain are different from each other. Addressing the accuracy of this observation can be evaluated in terms of relevant research. Also there was a similar trend in the RFR groups in the right and left lobes of the brain.
One of the parameters we investigated in this study is the area of cytoplasm (%) of brain cells in both lobes. We observed that all three frequencies did not change the area of cytoplasm (%) of brain cells ( and ). To test the accuracy of our results, we additionally determined the neuron and cytoplasm areas in both lobes ( and ). We observed that the neuron cell areas in the right and left lobes decreased compared to the sham control group, but the difference was non-significant (p > 0.05). The fact that the space between neuron cells in the right and left lobes increased in all three frequencies compared to the sham control group supports the idea that there is shrinkage in the cells. Although the reduction in cell areas was not statistically significant, it indicates mild shrinkage in the cells. The most important result we obtained in this study is that all three RFRs used in this study could increase the interstitial space ( and ). On the other hand, more comprehensive studies are needed to reveal whether there is actually shrinkage in the cells and the accuracy of our claim. The decrease in neuron cell area alone may not be sufficient to explain the increase in the interstitial space, and it may be necessary to examine hollow structures such as vessels and ventricles in subsequent studies. Observing that the RFRs we used in this study increase the interstitial space and the idea of gaining a different perspective in the treatment of some brain diseases may raise hopes. However, it should be kept in mind that many studies have shown that that RFRs can cause different problems in the brain, especially tumors [Citation7–17].
Conclusions
In this study, we observed that exposure of rats to 900, 1800 and 2100 MHz frequency RFRs resulted in increased interstitial space in the right and left lobes of the brain. This result may contribute to the treatment of some brain-related diseases, but we should not forget the research results that show RFRs can cause brain tumors. Due to the limited number of studies on the subject, further and more detailed new studies are needed for risk assessment and clarification of the observed effects.
Disclosure statement
No potential conflict of interest was reported by the authors.
Additional information
Funding
References
- Xie L, Kang H, Xu Q, et al. Sleep drives metabolite clearance from the adult brain. Science. 2013;342(6156):373–377.
- Arendash GW, Sanchez-Ramos J, Mori T, et al. Electromagnetic field treatment protects against and reverses cognitive impairment in alzheimer’s disease mice. J Alzheimers Dis. 2010;19(1):191–210.
- Tsoy A, Saliev T, Abzhanova E, et al. The effects of mobile phone radiofrequency electromagnetic fields on β-amyloid-induced oxidative stress in human and rat primary astrocytes. Neuroscience. 2019;408:46–57.
- Dasdag S, Akdag MZ, Kizil G, et al. Effect of 900 MHz radio frequency radiation on beta amyloid protein, protein carbonyl, and malondialdehyde in the brain. Electromagn Biol Med. 2012;31(1):67–74.
- Arendash GW. Transcranial electromagnetic treatment against alzheimer’s disease: why it has the potential to trump alzheimer’s disease drug development. J Alzheimers Dis. 2012;32(2):243–266.
- Dasdag O, Adalier N, Dasdag S. Electromagnetic radiation and alzheimer’s disease. Biotechnol Biotechnol Equip. 2020;34(1):1087–1094.
- Dasdag S, Akdag MZ, Erdal ME, et al. Long term and excessive use of 900 MHz radiofrequency radiation alter microRNA expression in brain. Int J Radiat Biol. 2015;91(4):306–311.
- Er H, Basaranlar G, Ozen S, et al. The effects of acute and chronic exposure to 900 MHz radiofrequency radiation on auditory brainstem response in adult rats. Electromagn Biol Med. 2020;39(4):374–386.
- Dasdag S, Akdag MZ, Ulukaya E, et al. Effects of mobile phone exposure on apoptotic glial cells and status of oxidative stress in rat brain. Electromagn Biol Med. 2009;28(4):342–354.
- Dasdag S, Akdag MZ, Erdal ME, et al. Effects of 2.4 GHz radiofrequency radiation emitted from Wi-Fi equipment On microRNA expression In brain tissue. Int J Radiat Biol. 2015;91(7):555–561.
- Me Alkis ME, Bilgin HM, Akpolat V, et al. Effect of 900-, 1800-, and 2100-MHz radiofrequency radiation on DNA and oxidative stress in brain. Electromagn Biol Med. 2019;38(1):32–47.
- Dasdag S, Erdal ME, Erdal N, et al. 900 MHz Radiofrequency radiation has potential to increase the expression of rno-miR-145-p in brain. J Int Dent Med Res. 2019;12(4):1652–1165.
- Dasdag S, Akdag MZ, Bashan M, et al. Role of 2.4 GHz radiofrequency radiation emitted from Wi-Fi on some miRNA and faty acids composition in brain. Electromagn Biol Med. 2022;41(3):281–292.
- Bektas H, Algul S, Altindag F, et al. Effects of 3.5 GHz radiofrequency radiation on ghrelin, nesfatin-1, and irisin level in diabetic and healthy brains. J Chem Neuroanat. 2022;126:102168.
- Tan B, Canturk Tan F, Yalcin B, et al. Changes in the histopathology and in the proteins related to the MAPK pathway in the brains of rats exposed to pre and postnatal radiofrequency radiation over four generations. J Chem Neuroanat. 2022;126:102187.
- Hardell L, Moskowitz JM. A critical analysis of the MOBI-Kids study of wireless phone use in childhood and adolescence and brain tumor risk. Rev Environ Health. 2022. Early Access, Indexed2022-05-25. https://doi.org/10.1515/reveh-2022-0040
- Pareja-Peña F, Burgos-Molina AM, Sendra-Portero F, et al. Evidences of the (400 MHz–3 GHz) radiofrequency electromagnetic field influence on brain tumor induction. Int J Environ Health Res. 2022;32(1):121–130.