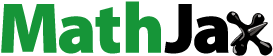
ABSTRACT
Excessive soil moisture (ESM) is a critical factor limiting the productivity of buckwheat (Fagopyrum esculentum Moench) because ESM inhibits its germination. This study investigated the effects of pericarp and seed respiration on reduced buckwheat germination caused by submergence. Seeds were submerged prior to germination testing under aerobic conditions. The effects of days of submergence treatment and pericarp removal on germination percentage were significant. The germination percentage in the 3-day submergence treatment was low in seeds with pericarp (P+) but was significantly improved in seeds without pericarp (P−). Pericarp removal also improved the germination percentage of non-submerged seeds. Pericarp thickness and pericarp/seed dry weight ratio (PWR) were not significantly correlated with the germination percentage in 3-day submerged P+. However, both were significantly negatively correlated with the germination percentage in 1-day submerged P+. Seed respiration rate in water, measured on seeds not submerged before measurement, was significantly higher in P− than in P+; that of P− increased with time, while that of P+ showed a decreasing trend with time beyond the first 24 h. Seed respiration rate during the first 24 h was significantly negatively correlated with germination percentage in 3-day submerged P+. A negative influence of pericarp thickness and PWR on the seed respiration rate of P+ during the first 24 h was suggested. Suppressed seed respiration by the pericarp is probably a cause of inhibited buckwheat germination under ESM conditions.
Introduction
In Japan, buckwheat (Fagopyrum esculentum Moench) is often cultivated in upland fields converted from paddy fields that are poor in drainage (MAFF, Citation2019). However, it is one of the upland crops most susceptible to excessive soil moisture (ESM) injury. This poses the problem of poor growth, resulting in reduced yields. Nishimaki (Citation1983) reported that buckwheat was the most susceptible to ESM injury among seven upland crops. Björkman (Citation2001) also reported that it was more susceptible to ESM injury than rye and snap bean. The influence of ESM conditions on buckwheat growth varies with the growth stage. ESM injury in buckwheat is more severe when exposed to ESM conditions earlier in the growth stage, and the most severe injury occurs at the germination stage (Nishimaki, Citation1983; Sakata & Ohsawa, Citation2005; Sugimoto & Sato, Citation2000; Takemae, Citation1986). The germination growth stage is the most susceptible and vulnerable (Sakata & Ohsawa, Citation2005), and the transition stage from the vegetative to the reproductive growth stage is also susceptible and affects growth and yield (Koyama et al., Citation2019). Differences in germination under ESM conditions among cultivars have been reported by Murayama et al. (Citation2004) and Sakata and Ohsawa (Citation2006).
Takeshima et al. (Citation2023) reported that raised-bed planting increased buckwheat yield in Japan by improving the soil water conditions and enhancing both vegetative and reproductive growth. However, the reported yield increase was not attributed to improved seedling establishment. To improve buckwheat productivity in Japan, in addition to improving field drainage, breeding cultivars tolerant to ESM conditions is important. Since cultivar differences in germination under ESM conditions have been reported, breeding cultivars tolerant to ESM conditions is expected. To establish an effective breeding system for buckwheat cultivars tolerant to ESM conditions, developing effective selection methods based on the analysis of factors involved in reduced germination under ESM conditions is indispensable.
Michiyama et al. (Citation2013) and Kudo and Sone (Citation2017) observed that aeration of water in which buckwheat seeds were soaked improved germination under submerged conditions. Mizushima (Citation2015), Kudo and Sone (Citation2017), and Sasaki et al. (Citation2021) reported that coating buckwheat seeds with calcium peroxide (CaO2) improved the seedling emergence percentage under ESM conditions. Aeration of water increases the dissolved oxygen (DO) concentration, and CaO2 generates oxygen through a chemical reaction with water. Aeration and CaO2 seem to have the same effect of increasing the oxygen supply to the seeds. Sekinuma et al. (Citation2015) demonstrated that pericarp removal improved the germination and seedling emergence of buckwheat under excess water conditions. This seems to be due to the increased oxygen permeability through the seed coat tissue, resulting in increased oxygen supply to the embryo. Although poor germination growth of buckwheat is thought to be caused by a suppressed oxygen supply to the embryo and the resulting decline in seed respiration, seed respiration of buckwheat under submerged conditions has not been reported in detail. The relationship between cultivar differences in submergence tolerance and seed respiration under submerged conditions remains unclear.
This study aimed to obtain useful information for breeding buckwheat cultivars tolerant to germination under submerged conditions by analyzing the involvement of the pericarp in reduced germination under submerged conditions. To this end, we investigated the relationship between pericarp characteristics, such as thickness, and germination under submerged conditions. We also compared seed respiration rates in water between seeds with and without pericarp removal.
Materials and methods
Plant materials
Seven buckwheat cultivars, Shinano-natsusoba (SN), Harunoibuki (HI), Kitawasesoba (KW), Nanbusoba (NS), Shinano No. 1 (S1), Hokkai No. 3 (H3), and Shinshu-oosoba (SO), were used. The ecotypes of the used cultivars are shown in .
Table 1. Cultivars used in this study, and their 1000-seed weight, ecotypes, and moisture contents.
Seed preparation
Seeds with damaged pericarps and those without pericarps were carefully excluded before conducting the following experiments. Buckwheat seeds are triangular in shape, but some are biangular or quadrangular; the latter two were not excluded. After selecting seeds, they were put in zippered plastic bags to keep their moisture content constant and stored in a refrigerator at 5°C or lower. Pericarps of some of the stored seeds were removed using a hand-huller designed for hulling a small number of rice seeds (Huller for one panicle; FUJIWARA SCIENTIFIC Co. Ltd., Tokyo, Japan), and seeds without damaged testae were re-stored in the same refrigerator. Hereafter, seeds with and without pericarps are referred to as P+ and P− seeds, or simply P+ and P−, respectively. The fresh weights of 100 P+ seeds of each cultivar were measured before dissection and division into pericarps and P− seeds, followed by measurement of the fresh weights of pericarps and P− seeds for each cultivar. After drying the pericarps and P− seeds at 80°C for 48 h, the dry weights of each were measured, and the seed moisture content was determined. Seed moisture content ranged from 11.1% to 14.2% (). The pericarp/seed dry weight ratio (PWR) was determined as [pericarp dry weight]/[whole seed dry weight].
Seed submergence treatment and germination test
Fifty seeds of each cultivar were submerged in pure water using a plastic container (2.5 cm radius and 6.0 cm deep) with a screw lid and were placed in an incubator kept at 25°C in the dark. The pure water amount was 30 times that of the fresh seed weight. During seed submergence, the lid was closed, the water was not replaced, and a stainless-steel wire mesh was placed over the seeds wrapped in a paper towel (Pure leaf M210; Ozu Corporation, Tokyo, Japan) to prevent the seeds from floating (). The days of submergence were 0, 1, and 3 in P+ and 0 and 3 in P–. In the 0-day submergence, no seed submergence was applied. The treatments were applied in triplicate without seed sterilization. For P−, 1-day submergence was omitted because the preliminary experiment showed a notable improvement in germination even with a 3-day submergence. After the submergence, 50 seeds of each cultivar were sown in a plastic box (7.5 × 10.5 cm and 2.0 cm deep) lined with two sheets of the above-mentioned paper towels. The boxes were covered with lids, and water was added as needed to keep the paper towels moist and the germination conditions aerobic. Each cultivar was germinated in triplicate at 25°C in the dark, and the seeds were considered germinated when the tip of a radicle broke through the pericarp and appeared on the outside. The radicle length was at least 1 mm. The number of germinated seeds was recorded 4 days after sowing, and the germination percentage was determined for each box based on 50 seeds. In the following, for example, the germination percentage in seeds exposed to 3 days of submergence prior to the germination test is referred to as the germination percentage after 3-day submergence.
Figure 1. Submergence treatment prior to germination tests. During the treatment, the lid was closed. A stainless-steel wire mesh was placed over the seeds to prevent them from floating.
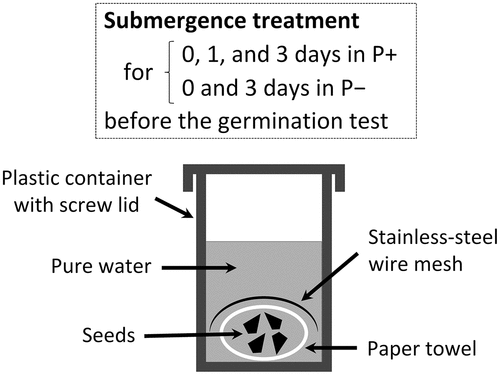
To clarify the effect of pericarp removal on germination percentage, an index of how much the germination percentage of 3-day submerged seeds decreased compared to 0-day submerged seeds (G% reduction) was defined as follows.
Measurement of pericarp thickness
One sheet of pericarp was peeled off per seed regardless of the seed shape, triangular, biangular, or quadrangular. It was cut perpendicular to the basal apex direction at the midpoint of its length in the basal apex direction. The pericarp thickness was measured at A and B using a digital caliper (CD-10CPX; Mitsutoyo Co. Ltd., Kanagawa, Japan) (). The thicknesses were almost constant along the basal apex direction in both parts. The pericarp thicknesses measured at A and B are referred to as pericarp thickness A and pericarp thickness B, respectively. Measurements were made on nine pericarp samples for each cultivar, treated as nine replicates.
Figure 2. Parts of the pericarp where the thickness was measured. The lower part of the figure is a cross-section of a seed at mid-seed height. The upper part of the figure is an enlarged view of a pericarp cross-section. For each seed, a sheet of pericarp bounded by two seed ridges was peeled off and the thicknesses at A (the central part of a pericarp sheet where a vascular bundle is located) and B (the central part between A and the seed ridge) were measured using a digital caliper.
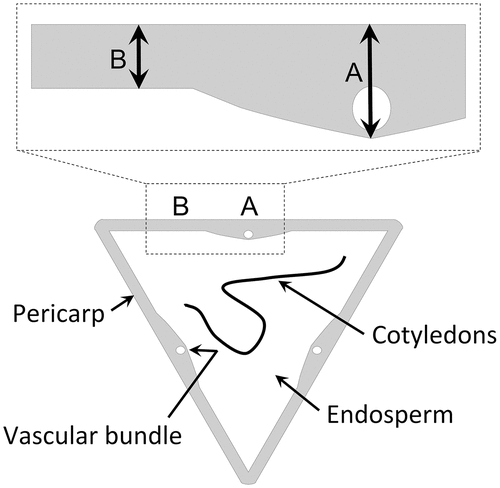
Measurement of the seed respiration rate of P+
Fresh weights of 50 P+ seeds of each cultivar were measured before sterilization with 10% sodium hypochlorite solution for 1 min, followed by rinsing with pure water for 1 min. The sterilized seeds were held in a stainless-steel wire mesh case (spherical tea strainer, diameter 37 mm) and soaked in 2 L of pure water in a plastic cup (top diameter 15.8 cm, bottom diameter 12.5 cm, height 18.0 cm) at 25°C in the dark. The DO concentration of the water was measured to determine the seed respiration rate following Sekinuma et al. (Citation2015) using an optical DO probe (MM4-DDO; TOA DKK Co. Ltd., Tokyo, Japan) connected to a portable multi-water quality meter (MM-42DP; TOA DKK Co. Ltd., Tokyo, Japan). During the measurement, the water surface was covered with approximately 125 mL of liquid paraffin to a depth of approximately 5 mm to suppress oxygen diffusion into the water, and the water was stirred continuously with a stirrer. DO concentrations during the 3-day measurement period initially ranged from 8.42 to 8.25 ppm and then from 6.20 to 4.43 ppm, varying by cultivar. Data were recorded by a data logger (VR-71; T&D Corp., Nagano, Japan) every 1 min for 3 days. Oxygen consumption was determined from the decrease in DO concentration every 12 h, and seed respiration rate of P+ on a seed dry weight basis (RP+) was obtained as shown below. Measurements were triplicated for each cultivar.
where ΔO2 is the decrease in oxygen (mg) in a 12-h period, Δt is 12 (h), and W is the seed dry weight (g). Seed dry weight was determined using the previously determined seed moisture content.
Measurement of the seed respiration rate of P−
Seed sterilization, seed soaking cup, water amount, temperature conditions, and DO concentration measurements were the same as those described for P+, except as follows. Since the DO concentration decreased rapidly to nearly 0 ppm when P− seeds were soaked, the DO concentration for P− was adjusted as follows. First, 2 L of continuously aerated pure water was prepared in a plastic cup of the same size but different from the one in which the seeds were soaked. Then, the cups were connected by tubing pumps (SNM-23AS; AS ONE Co. Ltd., Osaka, Japan) to allow water circulation between them. The pumps were triggered by a homemade relay device to control the DO concentration. When the DO concentration fell below 4.5 ppm, the pumps circulated water to raise it to 6.5 ppm, and when it exceeded 6.5 ppm, the pumps stopped circulating. The DO concentration was controlled to be within this range because, in the case of P+, the DO concentration did not fall below approximately 4.5 ppm during a 3-day measurement period. DO concentrations during the measurement period were initially 8.58‒8.26 ppm, then decreased and remained within a range of 6.69‒6.38 ppm to 4.63‒4.49 ppm, each varying by cultivar.
The DO concentration and whether the pumps were on or off were recorded every 1 min using the same data logger as for P+. During the measurement period, increases in DO concentration that occurred while the pumps were on and decreases in DO concentration that occurred while the pumps were off were repeated. On average for all cultivars, the pumps ran 1.12 times and 52.92 min per 12 h. Oxygen consumption was determined from the total decrease in DO concentration while the pumps were off during each 12-h period. Oxygen consumption was divided by the total time the pumps were off during the 12-h period to obtain the seed respiration rate of P− (RP−), as follows. Measurements were triplicated for each cultivar.
where ΔO2 is the decrease in oxygen (mg) in a 12-h period, Δt is 12 minus [total hours the pumps were on during the 12-h period] (i.e. the total time in hours that the pumps were off during the 12-h period) (h), and W is the seed dry weight (g) before pericarp removal. W was calculated using the previously determined seed moisture content and PWR.
The value obtained was considered as RP− for each 12-h period, assuming that the seed respiration rate with the pumps on was the same as with the pumps off.
Statistical analysis
BellCurve for Excel ver. 4.04 (Social Survey Research Information Co., Ltd., Tokyo) was used for statistical data analyses (ANOVA, Tukey-Kramer test, t-test, and regression analysis). Arcsine transformation was applied before performing a three-way ANOVA for the germination percentage and a two-way ANOVA for G% reduction.
Results
Germination percentage
Germination percentages are shown in . In 0-day submerged (non-submerged) seeds, regardless of P+ or P−, they ranged from 86.7% to 100%, varying among cultivars. In P+, 1-day submergence resulted in germination percentages ranging from 36.7% in H3, which was significantly lower than other cultivars, followed by 66.7% in SN, which was significantly lower than S1 and HI, to 92.7% in S1, showing that H3 and SN were more susceptible to submergence than other cultivars. Three-day submergence in P+ resulted in germination percentages ranging from 4.0% to 21.3%, while in P− they were maintained at 74.0% to 95.3% even with 3-day submergence.
Table 2. Germination percentages of P+ and P−. P+ and P− are seeds without and with pericarp removal. Germination was tested under aerobic conditions on seeds exposed to different days of submergence before testing. In the 0-day submergence, the seeds were not submerged.
The results of a three-way ANOVA (cultivar (C), pericarp removal (P), and submergence days (S)) () showed that the main effects of C, P, and S were all significant at the 0.1% level. The C×P, C×S, and P×S interactions were also significant at the 1%, 5%, and 0.1% levels, respectively. Because the three-way interaction C×P×S was significant at the 5% level, the simple interaction test, i.e. the test of the interaction between two factors at each level of the third factor, was performed using two-way ANOVA. The simple-simple main effect, i.e. the main effect of the third factor in each combination of the levels of two factors, was also tested (Tukey-Kramer test). These tests were performed to better understand the effect of each of C, P, and S within specific conditions defined by the other factors.
When comparing among cultivars, in P+/0-day-submergence, SN was the lowest and was significantly lower than HI, S1, SO, and NS. In P+/1-day-submergence, H3 was the lowest, with significant differences from all other cultivars, followed by SN, with significant differences from S1 and HI. In P+/3-day-submergence, no significant difference was found among cultivars. In P−/0-day-submergence, SN was the lowest, with significant differences from all other cultivars. In P−/3-day-submergence, SN was the lowest but was significantly lower than only H3.
When comparing P+ and P− within the same days of submergence, in 0-day submergence, P− was significantly superior to P+ only in KW, and in 3-day submergence, P− was significantly superior to P+ in all cultivars.
When comparing days of submergence within P+ or P−, in P+, 3-day submergence significantly reduced germination percentage in all cultivars, and in P−, 3-day submergence significantly reduced germination percentage except for H3 and SN.
While 3-day submergence severely reduced germination percentage regardless of cultivar in P+, pericarp removal effectively suppressed the decrease in germination percentage after 3-day submergence in all cultivars with no significant difference between cultivars and no significant interaction between pericarp treatment and cultivar ().
Table 3. G% reduction, which is an index of how much the germination percentage of 3-day submerged seeds decreased compared to 0-day submerged seeds.
Relationship between pericarp thickness or PWR and germination percentage of P+
shows the cultivar differences in pericarp thickness and PWR. Pericarp thicknesses A and B were 0.07‒0.13 mm and 0.05‒0.10 mm, respectively. PWRs ranged from 0.167 to 0.297. H3, SN, and SO had relatively thicker pericarp or larger PWR among the cultivars. Pericarp thicknesses A and B were significantly different in all cultivars. They were correlated (r = 0.972) at the 0.1% significance level and also correlated with PWR at the 1% (r = 0.923) and 0.1% (r = 0.970) significance levels, respectively.
Table 4. Pericarp thickness and PWR. PWR is the ratio of pericarp dry weight to whole seed dry weight.
shows the correlation between pericarp characteristics and germination percentages of P+. When all cultivars were grouped, pericarp thickness and PWR did not correlate with germination percentage after 0- or 3-day submergence, whereas they were significantly negatively correlated with germination percentage after 1-day submergence. Among all cultivars, the germination percentage of P+ in SN was the lowest when 0-day submerged and the highest when 3-day submerged (). Also, the G% reduction in SN was the lowest in P+ and the highest in P− among all cultivars (). These results suggested that SN responded differently to submergence than the other cultivars. Therefore, the correlation was analyzed excluding SN. The analysis strengthened the correlations; they were significant between pericarp characteristics and germination percentage after 0- and 1-day submergence.
Table 5. Correlation coefficients between pericarp characteristics and germination percentage of P+ after submergence. Germination was tested on seeds exposed to 0- (no submergence), 1-, and 3-day submergence before the test. P+ is seeds without pericarp removal. PWR is the ratio of pericarp dry weight to whole seed dry weight.
Seed respiration rate
The RP+ () during the first 24 h remained almost constant in SN, HI, and NS, while it decreased in other cultivars and tended to decrease further in all cultivars thereafter. In contrast, the RP− ( and ) tended to increase; it was 1.7‒3.6 times higher during the first 24 h, 2.5‒4.4 times higher during 24‒48 h, and 7.1‒13.0 times higher beyond 48 h compared to RP+. RP− was significantly higher than RP+ in all cultivars and all periods, except in SN during 12–24 h ().
Figure 3. Changes with time in the respiration rate of P+ and P− obtained for seeds not submerged before respiration measurement. P+ and P− are seeds without and with pericarp removal.
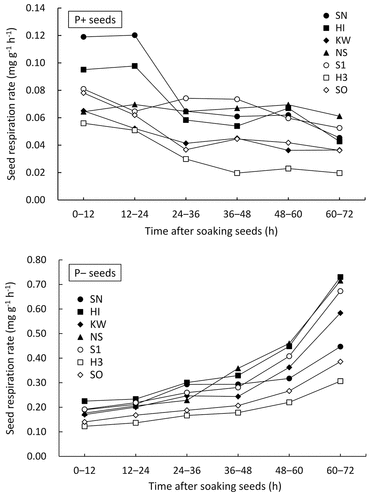
Table 6. Comparison of the seed respiration rate of P+ (RP+) and P− (RP−) at each seed soaking period. P+ and P− are seeds without and with pericarp removal.
Relationship between seed respiration rate and germination percentage
The correlation between RP+ and the germination percentage of P+ was examined to clarify the effect of seed respiration rate under submerged conditions on the germination percentage of P+. Correlation coefficients between RP+ up to 24 h or 72 h and 1- or 3-day submergence are shown in . For 1-day submerged seeds, the correlation was not significant when all cultivars were grouped, but it was significant and positive when SN was excluded. For 3-day submerged seeds, a significant positive correlation was found only between the initial (0‒24 h) RP+ and the germination percentages. In contrast, no significant correlation was found for RP− regardless of the days of submergence (data not shown).
Table 7. Correlation coefficients between RP+ at each seed soaking period and germination percentages of P+ under aerobic conditions after exposure to 1- or 3-day submergence. P+ is seeds without pericarp removal.
Relationship between pericarp thickness, PWR, and seed respiration rate
Correlations between pericarp thickness or PWR and the RP+ in 0‒24 h were negative (not significant at the 10% level) and were negative and significant at the 10% level when SN was excluded (Supplementary Figure).
Discussions
Effect of pericarp removal on germination percentage
In spinach, pericarp removal has been reported to improve germination percentage under excess moisture conditions (Heydecker & Orphanos, Citation1968). Sekinuma et al. (Citation2015) reported that pericarp removal improved the seedling emergence of 3-day submerged S1. However, it remains unknown whether pericarp removal is effective in other buckwheat cultivars. Our study used cultivars that differed in their ecotypes and 1000-seed weight, and demonstrated that pericarp removal significantly improved the germination percentage after 3-day submergence in all cultivars (). Pericarp removal was effective regardless of the days of submergence, and no significant difference in effect was found between cultivars (). Since the pericarp mechanically inhibits germination in a plant of the Polygonaceae (Metzger, Citation1992), to which buckwheat belongs, the possible role of the buckwheat pericarp as a mechanical barrier to germination needs to be investigated.
When the seeds were recorded as germinated, the mean radicle length across cultivars was longer for P+ than for P− in 0-day submergence (data not shown). On the other hand, it was shorter for P+ than for P− in 3-day submergence. This may have some influence on the germination percentages of P+ and P−, but the difference between them was so significant and clear () that there is no doubt about the germination-promoting effect of pericarp removal and the importance of the role of pericarp in the germination of submerged seeds. Furthermore, pericarp removal showed efficacy even in the most submergence-susceptible cultivar H3, suggesting that pericarp removal may notably improve buckwheat germination under ESM conditions.
Effect of pericarp removal on seed respiration rate
In this study, DO concentrations were kept similar for both RP+ and RP− measurements to allow for meaningful comparisons. In P−, respiration rates could not be measured while the water circulation was on because the DO concentration increased during this period. However, it is unreasonable to assume that the respiration rate during this period is lower than when the water circulation was off. Therefore, it is reasonable to assume that RP− was not overestimated, at least during the first 24 h when no roots appeared, and the result that RP– significantly exceeded RP+ is unaffected. The higher RP− than RP+ beyond 24 h may be partly due to root respiration.
Magnée et al. (Citation2020) reported low germination percentages for spinach seeds with thicker pericarp at normal O2 levels. However, higher O2 levels resulted in higher germination percentages. They suggested that the low O2 permeability of the pericarp resulted in limited O2 supply to the embryo, leading to suppressed germination under excessive moisture conditions. Živković et al. (Citation2021) compared the buckwheat germination of P+ and P− after soaking them for 8 h, with a 15-min period out of the water every hour, and reported that the germination percentages exceeded 95% in both P+ and P−. They also reported that the seed weight losses and root growth increases were significantly greater in P−, probably because of improved metabolism due to the increased permeability of oxygen and carbon dioxide caused by pericarp removal. These reports suggest that pericarp removal improves respiratory metabolism in seeds exposed to submergence.
According to , the effect of pericarp removal on seed respiration (RP−/RP+ ratio) during the first 24 h was large in KW, NS, and S1 and was small in SN and SO. These results suggest that the pericarp exerts stronger suppression on seed respiration in the former cultivars than the latter.
Cause of poor germination under submerged conditions
Remarkable differences in seed respiration rates and germination percentages between P+ and P− were found in this study. Previous studies have shown that increasing the O2 supply to the embryo, using techniques such as aeration during water soaking (Kudo & Sone, Citation2017; Michiyama et al., Citation2013; Sasaki et al., Citation2021) or seed coating with CaO2 (Kudo & Sone, Citation2017; Mizushima, Citation2015; Sasaki et al., Citation2021) to release O2 upon contact with water, leads to better germination. Thus, suppressed seed respiration is the most likely cause of inhibited buckwheat germination due to submergence.
As days of submergence increased, germination percentages decreased in P+ and slightly decreased in P− (). Germination in 1- or 3-day submerged P+ appeared to be influenced by seed respiration rate during the first 24 h (). The RP− was 1.7‒3.6 times higher than RP+ even during 0–24 h when no roots appeared (). Pericarp removal increased seed respiration rates during the early stage of seed soaking, probably resulting in less damage to embryo physiology, which in turn resulted in accelerated seed respiration rates during the later stage of seed soaking ( and ), allowing normal germination to proceed. Seed respiration rate generally increases after seed imbibition (Bewley & Black, Citation1994). Since the RP+ did not increase beyond 24 h ( and ), soaking P+ for 24 h likely damages respiration physiology, depending on the cultivar. The germination percentage after 1-day submergence in P+ varied from 36.7% to 92.7% among cultivars (). The greatly reduced germination percentage after 3-day submergence in P+ (4.0% to 21.3%) is presumably due to the prolonged low seed respiration beyond 24 h, which aggravated germination physiology. No correlation between seed respiration rates and germination percentages was observed in P− (data not shown), probably because the sufficiently high seed respiration rates did not result in an inhibitory factor of germination.
Relationship between pericarp characteristics and germination percentage or seed respiration rate
Except for SN, thicker pericarp and larger PWR were suggested to be inhibitory factors of germination of 0- and 1-day submerged P+ (). SN had a medium thick pericarp and large PWR among the cultivars tested () but also had the highest RP+ during the first 24 h ( and ). The relationship between pericarp characteristics and seed respiration rate in SN seemed to be different from that in other cultivars. The germination percentages of 3-day submerged P+ and pericarp characteristics were not correlated, probably because the germination percentages were extremely reduced.
While the effect of pericarp removal on promoted seed respiration rate was evident regardless of cultivar ( and ), the negative correlation between pericarp characteristics and RP+ up to 24 h was not clear when all cultivars were grouped. However, as mentioned earlier, there appeared to be a difference in the effect of the pericarp on seed respiration rate between cultivar groups. In addition, the RP+/RP− ratio up to 24 h tended to decrease with increasing pericarp thickness and PWR within each cultivar group with a thicker pericarp and larger PWR and that with a thinner pericarp and smaller PWR (data not shown). It is suggested that a thicker pericarp and larger PWR have a suppressing effect on seed respiration rate, but the effect did not appear to be uniform among cultivars. Some factors other than pericarp thickness and PWR, possibly substances contained in the pericarp as discussed below, also seem to play a role in the effect of pericarp on seed respiration rate.
Chemical factors related to suppressed seed respiration rate
Côme and Tissaoui (Citation1972) suggested that polyphenols in apple seed tissue cause the inhibition of O2 permeability. Polyphenols are antioxidant substances present in buckwheat pericarp (Holasova et al., Citation2002; Watanabe, Citation1998; Watanabe et al., Citation1997). Therefore, buckwheat pericarp may reduce seed respiration rate by chemically inhibiting O2 permeation.
Sekinuma et al. (Citation2015) compared the seedling emergence of pericarp-removed seeds soaked together with or without the removed pericarp in S1. They found that the final seedling emergence percentage did not differ considerably, but the former seeds delayed the emergence. This suggests that buckwheat pericarp contains substances that inhibit germination. Buckwheat pericarp is rich in rutin (Watanabe et al., Citation1997), which is a polyphenol (Holasova et al., Citation2002; Watanabe, Citation1998). Golisz et al. (Citation2007) reported that buckwheat polyphenols, such as rutin, inhibited root and hypocotyl growth of lettuce seedlings. Polyphenols, such as rutin, contained in buckwheat pericarp may have inhibitory effects on its germination.
Further studies are needed to clarify why the pericarp suppresses the respiration of submerged buckwheat seeds. They should focus on obtaining more accurate pericarp thickness using image analysis, investigating the compactness of the pericarp and the airtight structure of its ridge parts of the pericarp, and evaluating the chemicals contained in the pericarp, such as antioxidants and germination inhibitors. A detailed comparison and analysis of the cultivar groups that appeared to differ in the effect of the pericarp on seed respiration rates, as mentioned above, along with other cultivars from the above perspectives, will help clarify what factors in the pericarp and how they suppress seed respiration or germination under submerged conditions. This would provide critical information for improving buckwheat germination under ESM conditions by enhancing seed respiration.
Conclusions
Pericarp removal significantly improved germination after 3-day submergence in all cultivars, with no significant difference in effect among cultivars. Suppressed seed respiration rate was revealed as the primary cause of reduced germination under submergence, as RP+ was low during the first 24 h and decreased thereafter, while RP− was significantly higher than RP+ during 3-day submergence. Negative correlations of pericarp thickness, PWR, and RP+ during the first 24 h with the germination percentage of P+ were suggested. These results suggested that a smaller pericarp thickness and PWR may favor buckwheat germination under ESM conditions. However, since the relationship between pericarp characteristics and seed respiration rate did not appear to be uniform among cultivars, further studies are needed to clarify how the pericarp suppresses seed respiration, which will help the efficient breeding of buckwheat tolerant to ESM conditions.
Author contributions
MH conceived and designed the basic part of the experiments, and AH designed the practical part. AH performed the experiments. AH and MH analyzed the data. AH drafted the manuscript, and MH revised and finalized the manuscript. All the authors have read and approved the final manuscript.
Supplemental Material
Download MS Excel (30.9 KB)Acknowledgments
We thank Dr. Asana Matsuura, Associate Professor of Shinshu University, for her general comments and helpful suggestions. We also thank Ms. Natsuki Niwa, a graduate student at Shinshu University, for her assistance with the measurements of seed respiration rate.
Disclosure statement
No potential conflict of interest was reported by the author(s).
Supplementary material
Supplemental data for this article can be accessed online at https://doi.org/10.1080/1343943X.2024.2322772
References
- Bewley, J. D., & Black, M. (1994). Seeds: Physiology of development and germination (2nd ed.). Plenum Press. https://doi.org/10.1007/978-1-4899-1002-8
- Björkman, T. (2001). Causes of poor stand establishment after heavy rains. Advances in Buckwheat Research: Proceedings of the 8th International Symposium on Buckwheat, 134–137.
- Côme, D., & Tissaoui, T. (1972). Interrelated effects of imbibition, temperature and oxygen on seed germination. In W. Heydecker (Ed.), Seed ecology (pp. 157–168). Butterworth-Heinemann.
- Golisz, A., Lata, B., Gawronski, S. W., & Fujii, Y. (2007). Specific and total activities of the allelochemicals identified in buckwheat. Weed Biology and Management, 7(3), 164–171. https://doi.org/10.1111/j.1445-6664.2007.00252.x
- Heydecker, W., & Orphanos, P. I. (1968). The effect of excess moisture on the germination of Spinacia oleracea L. Planta, 83(3), 237–247. https://doi.org/10.1007/BF00385333
- Holasova, M., Fiedlerova, V., Smrcinova, H., Orsak, M., Lachman, J., & Vavreinova, S. (2002). Buckwheat—the source of antioxidant activity in functional foods. Food Research International, 35(2–3), 207–211. https://doi.org/10.1016/S0963-9969(01)00185-5
- Koyama, T., Suenaga, M., & Takashima, R. (2019). Growth and yield response of common buckwheat (fagopyrum esculentum Moench) to waterlogging at different vegetative stages. Plant Production Science, 22(4), 456–464. https://doi.org/10.1080/1343943X.2019.1670682
- Kudo, T., & Sone, C. (2017). Effects of the temperature on the germination of common buckwheat under submerged condition. Abstract of the 243rd Meeting of CSSJ, 177. https://doi.org/10.14829/jcsproc.243.0_177. Japanese with English title.
- MAFF. (2019, April 10). Planted area and production of buckwheat in 2018. Retrieved from https://www.e-stat.go.jp/dbview?sid=0001796455
- Magnée, K. J. H., Scholten, O. E., Postma, J., Lammerts van Bueren, E. T., & Groot, S. P. C. (2020). Sensitivity of spinach seed germination to moisture is driven by oxygen availability and influenced by seed size and pericarp. Seed Science and Technology, 48(1), 117–131. https://doi.org/10.15258/sst.2020.48.1.13
- Metzger, J. D. (1992). Physiological basis of achene dormancy in Polygonum convolvulus (Polygonaceae). American Journal of Botany, 79(8), 882–886. https://doi.org/10.1002/j.1537-2197.1992.tb13669.x
- Michiyama, H., Yoshimura, K., Kondo, A., & Hirano, T. (2013). Effect of excess of soil moisture immediately after seeding on germination, growth and yield of buckwheat. Abstract of the 235rd Meeting of CSSJ, 108–109. https://doi.org/10.14829/jcsproc.235.0_108. Japanese with English title.
- Mizushima, S. (2015). Stabilization of emergence by coating with CaO2 for buckwheat seeds in flooding condition. The Hokuriku Crop Science, 50, 57–60. https://doi.org/10.19016/hokurikucs.50.0_57. Japanese with English title.
- Murayama, S., Suyama, Y., & Yanokuchi, Y. (2004). Varietal difference of pre-germination flooding tolerance in buckwheat. Proceedings of the 9th International Symposium on Buckwheat, Prague 2004, 143–145.
- Nishimaki, K. (1983). Present status of buckwheat cultivation and its technical problems. Nougyo Oyobi Engei, 58(1), 140–146. Japanese.
- Sakata, K., & Ohsawa, R. (2005). Effect of flooding stress on the seedling emergence and the growth of common buckwheat. Japanese Journal of Crop Science, 74(1), 23–29. https://doi.org/10.1626/jcs.74.23. Japanese with English title and abstract.
- Sakata, K., & Ohsawa, R. (2006). Varietal differences of flood tolerance during germination and selection of the tolerant lines in common buckwheat. Plant Production Science, 9(4), 395–400. https://doi.org/10.1626/pps.9.395
- Sasaki, F., Yoshida, R., Ishikawa, T., Kato, F., & Kurauchi, N. (2021). Effects of calcium peroxide coating on moisture damage and suitability for mechanical sowing of common buckwheat. Japanese Journal of Farm Work Research. 56(3), 171–177. https://doi.org/10.4035/jsfwr.56.171. Japanese with English title and abstract.
- Sekinuma, M., Kato, F., Sasaki, F., Takeda, S., Inoue, N., & Kasuga, S. (2015). Effects of fruit skin and water temperature during soaking before germination on the emergence rates of common buckwheat. Bulletin Shinshu University Alpine Field Center, 13, 113–118.
- Sugimoto, H., & Sato, T. (2000). Effects of excessive soil moisture at different growth stages on seed yield of summer buckwheat. Japanese Journal of Crop Science, 69(2), 189–193. https://doi.org/10.1626/jcs.69.189. Japanese with English title and abstract.
- Takemae, A. (1986). Saving and stable high yielding culture in buckwheat. Nougyo Oyobi Engei, 61(11), 51–56. Japanese.
- Takeshima, R., Murakami, S., Fujiwara, Y., Nakano, K., Fuchiyama, R., Hara, T., Shima, T., & Koyama, T. (2023). Subsurface drainage and raised-bed planting reduce excess water stress and increase yield in common buckwheat (fagopyrum esculentum Moench). Field Crops Research, 297, 108935. https://doi.org/10.1016/j.fcr.2023.108935
- Watanabe, M. (1998). Catechins as antioxidants from buckwheat (fagopyrum esculentum Moench) groats. Journal of Agricultural and Food Chemistry, 46(3), 839–845. https://doi.org/10.1021/jf9707546
- Watanabe, M., Ohshita, Y., & Tsushida, T. (1997). Antioxidant compounds from buckwheat (fagopyrum esculentum Moench) hulls. Journal of Agricultural and Food Chemistry, 45(4), 1039–1044. https://doi.org/10.1021/jf9605557
- Živković, A., Polak, T., Cigić, B., & Požrl, T. (2021). Germinated buckwheat: Effects of dehulling on phenolics profile and antioxidant activity of buckwheat seeds. Foods, 10(4), 740. https://doi.org/10.3390/foods10040740