ABSTRACT
Objectives: Reactive oxygen species, which are implicated in the process of carcinogenesis, are also responsible for cell death during chemotherapy (CHT). Therefore, the aim of the study was to evaluate exhaled H2O2 levels in non-small cell lung cancer (NSCLC) patients before and after CHT.
Methods: Thirty patients (age 61.3 ± 9.3 years) with advanced NSCLC (stage IIIB–IV) and 15 age-matched healthy cigarette smokers were enrolled into the study. Patients received four cycles of cisplatin or carboplatin with vinorelbine every three weeks. Before and after the first, second, and fourth cycle, the concentration of H2O2 in exhaled breath condensate was measured with respect to treatment response.
Results: At the baseline, NSCLC patients exhaled 3.8 times more H2O2 than the control group (0.49 ± 0.14 vs. 0.13 ± 0.03 µmol/L, P < 0.05); this difference persisted throughout the study. CHT had no noticeable effect on exhaled H2O2 levels independent of the treatment response (partial remission vs. progressive disease). Pre- and post-CHT cycles of H2O2 levels generally correlated positively.
Discussion: The study demonstrated the occurrence of oxidative stress in the airways of advanced NSCLC patients. Exhaled H2O2 level was not affected by CHT and independent of treatment results and changes in the number of circulating neutrophils.
Introduction
Lung cancer is the most common malignancy worldwide and the leading cause of cancer-related death. Non-small cell lung cancer (NSCLC) comprises 85% of all lung cancer types. Unfortunately, only 15% of patients with NSCLC can undergo surgical treatment. Subsequently, the majority of patients are treated with chemotherapy (CHT) or a combined modality of CHT and radiotherapy with some receiving supportive care exclusively. An overproduction of reactive oxygen species (ROS) leading to oxidative stress has been implicated in the pathogenesis of different respiratory diseases such as chronic obstructive pulmonary disease and lung cancer. Cigarette smoke and inflammatory processes are the main risk factors for the development of lung cancer. Both aspects are also the most important sources of ROS involved in all three phases of cancer development: initiation, promotion, and progression [Citation1–3].
During the last decades, different oxidative stress markers including lipid peroxidation products (malondialdehyde, thiobarbituric acid reactive substances, and 8-isoprostanes), nitric oxide, and H2O2 have been explored not only in the blood, but also in exhaled breath condensate (EBC) and in lung cancer tissue [Citation4–7]. Exhaled H2O2 can originate from inflammatory cells (alveolar macrophages, polymorphonuclear leukocytes), type II pneumocytes, airway epithelial cells, and cancer tissue [Citation6,Citation7]. NSCLC patients were reported to have systemic [Citation8,Citation9] and oxidative stress in airways [Citation8] which can cause increased exhalation of H2O2. On the other hand, it is well known that the cytotoxic effect of cisplatin, one of the most effective anticancer agents widely used in the treatment of lung cancer involves free radical-induced reactions [Citation10]. However, data concerning the impact of CHT on oxidative stress in the NSCLC patients remain sparse and conflicting. While some studies found enhanced circulating oxidative stress markers in NSCLC patients treated with CHT [Citation11–14], in others an opposite trend was noted [Citation15].
Therefore, in this study we tested the hypotheses that: (A) patients with advanced NSCLC exhale more H2O2 and have higher plasma concentrations of lipid peroxidation products than age-matched apparently healthy cigarette smokers; (B) CHT (whole four cycles) enhances H2O2 exhalation and increases circulating levels of lipid peroxidation products in these patients. Finally, the associations between EBC H2O2 levels, CHT outcome, and the number of circulating neutrophils as well as plasma lipid peroxidation products were analyzed. The concentration of H2O2 in EBC was the primary endpoint while circulating levels of lipid peroxidation products were the secondary endpoint in this study.
Patients and methods
Thirty patients (mean age 61.3 ± 9.3 years, 25 men, five women) with histologically or cytologically confirmed NSCLC (inoperable stage IIIB and IV), without prior CHT, were included into the study. All participants were current cigarette smokers with an Eastern Cooperative Oncology Group PS of 0 or 1 (). Fifteen age- and cigarette smoking habit-matched apparently healthy subjects served as the control group. All NSCLC patients and controls had a pre-bronchodilator FEV1/FVC ratio of >0.7. Prior to starting CHT, all patients underwent a physical examination, blood and urine chemistry, and a chest CT scan. An abdominal ultrasound and or CT scan were also performed. In patients suspected of brain and bone metastases, a head CT scan and/or MRI or bone scans were additionally administered. Blood and urine chemistry was also performed before each cycle of CHT. Patients were treated with cisplatin or carboplatin with vinorelbine every 3 weeks. Nineteen patients received cisplatin and vinorelbine (10 patients in stage IIIB and nine in stage IV) and 11 patients were treated with carboplatin and vinorelbine (seven in stage IIIB and four in stage IV). All patients received four cycles of CHT. Cisplatin 80 mg/m2 was administered over a 1-hour infusion, while carboplatin at an area under the curve at 5 mg/mL/min over a 30 min infusion was given on the first day of each CHT cycle. An intravenous bolus of vinorelbine 25 mg/m2 was added on the first and the eighth day of the cycle. Antiemetic therapy with 5-hydroxytryptamine-3 antagonist (ondansetron 24 mg) and corticosteroids (dexamethasone 8 mg) was provided before and during the first five days of each CHT cycle. Some CHT cycles were delayed by two weeks due to grade two neutropenia (second cycle for two patients and fourth cycle for three additional patients). Two weeks after the second and fourth round of treatment, chest CT scans were performed and responses to treatment were assessed using the Response Evaluation Criteria in Solid Tumors (RECIST) [Citation16]. In the cancer group, EBC was collected on six occasions (before and 24 hours after the first, second, and fourth CHT cycle), while in the control group EBC was collected on only one occasion. The EBC collection was executed between 8 and 10 a.m. The study protocol was approved by the Ethics Committee of the Medical University of Lodz (RNN/174/06/KE) and a written informed consent was obtained from all participants enrolled in the study.
Table 1. Characteristic of 30 patients with non-small cell lung cancer (NSCLC) and 15 apparently healthy controls.
Collection of exhaled breath condensate and hydrogen peroxide measurement
The collection of EBC was performed as previously described [Citation17,Citation18]. The collecting device was composed of a mouthpiece that provided a one-way valve along with a saliva trap connected through a 50 cm tube to a glass Liebig tube cooler (Labmed, Lodz, Poland cat no. 6010). The tube was cooled by ethanol pumped in a closed circuit and then kept at −9°C with a Multi Temp III (Pharmacia, Biotech). The liquid EBC was collected into a sterile plastic ice-covered tube mounted at the base of a Liebig tube cooler. Subjects were instructed to keep an invariable tidal breathing (respiratory rate ranged from 17 to 24 breaths/min) during a 20 min session of EBC collection. The subjects were given a nose-clip and were instructed to rinse their mouth with distilled water just before and at seven and 14 min of EBC collection to minimize the risk of EBC specimen contamination with saliva containing relatively high levels of H2O2. The use of a nose-clip during EBC collection prevented (A) inhalation of air through the nose along with the possibility of H2O2 containing aerosols from the nasal epithelium entering inhaled air, as well as (B) leakage of exhaled air through the nose. All condensate samples were tested for amylase activity (salivary contamination marker) as previously described [Citation18]; enzyme activity was not detected in any of the samples, which excluded contamination with saliva. At the end of the session, 3–5 mL aliquots of EBC were transferred to Eppendorf tubes and stored at −80°C for no more than seven days until the final H2O2 measurements were taken according to the Ruch method (fluorometric, homovanillic acid, and horseradish peroxidase method) [Citation19] with some modifications [Citation17]. The lower limit of H2O2 detection was 83 nmol/L. The intra-assay variability did not exceed 2% of a standard H2O2 solution ranging from 0.1 to 0.5 µmol/L. During pre-incubation of EBC specimens, EBC specimens mixed with equal volume of 1 µmol/L H2O2 standard solution or H2O2 standard solution alone (n = 4) with 20 U of catalase for 30 min at 37°C completely abolished the H2O2 signal.
Complete blood count was determined in the Diagnostic Laboratory of Medical University Teaching Hospital of Lodz. Serum concentration of thiobarbituric reactive substances (TBARs) as a quantitative estimation of lipid peroxidation products was measured as previously described [Citation13].
Statistical analysis
The results were expressed as a mean (SD). The Analysis of Variance (ANOVA) for repeated observations or Friedman’s ANOVA was applied for the assessment of changes in variables during CHT depending on the data distribution tested with Shapiro–Wilk’s W test. In case of a statistically significant ANOVA, the post hoc analyses were performed with Scheffe’s test or a post hoc analysis for Friedman’s ANOVA (multiple comparisons at two different time points). The Mann–Whitney U test was used to compare EBC H2O2 levels of NSCLC patients and controls. The correlations between variables were determined using Pearson’s r and Spearman’s ρ according to data distribution. A P-value < 0.05 was considered significant.
Results
All patients completed the study. Fifteen patients, according to the RECIST criteria, achieved partial remission (PR) (11 in stage IIIB and four in stage IV) after the second cycle of CHT, whereas the remaining patients (six patients in stage IIIB and nine in stage IV) demonstrated stable disease (SD). None of the studied NSCLC patients achieved complete remission in response to two cycles of CHT. At the end of study (after four cycles of CHT), patients who previously achieved PR demonstrated the same clinical response at the completion of treatment. However, at the end of CHT, out of the 15 NSCLC patients with SD (at the halfway point of treatment), one patient (stage IIIB) achieved PR and 10 patients (three in stage IIIB and seven in stage IV) were classified as progressive disease (PD). No patient had brain and bone metastases. There were significant changes in WBC and neutrophil count in NSCLC patients during the course of treatment. The WBC count (×103/µL) was the lowest (P < 0.001) before the second cycle (7.27 ± 2.91) in comparison to the values measured before the first (9.85 ± 3.80) and the fourth cycle (8.85 ± 3.41). The number of neutrophils (×103/µL) exhibited a similar behavior: 7.14 ± 3.59, 4.60 ± 2.49, and 6.04 ± 3.21 before the first, second, and fourth cycle, respectively (P < 0.05).
EBC H2O2 levels in patients with NSCLC
The concentration of H2O2 in the EBC of NSCLC patients was persistently higher than in healthy controls (). Before the first CHT cycle, NSCLC patients had an EBC H2O2 level 3.8-times higher than controls. Similarly, the H2O2 concentrations noted in the EBC of NSCLC patients at all subsequent occasions (after the first, before and after the second and fourth cycle of CHT) were approximately 4.1-, 4.0-, 4.2-, 3.5-, and 4.0-times higher than in healthy patients, respectively (). However, no significant changes in exhaled H2O2 over the study period were noted in the whole group of the NSCLC patients. This suggests that CHT has no effect on H2O2 exhalation. Similarly, analysis of EBC H2O2 levels in three subgroups of the NSCLC patients (classified accordingly to the final result of treatment as PR, SD, and PD) revealed no significant effect of CHT cycles (except for a rise in H2O2 after the fourth cycle in the PR subgroup) on this variable (). Moreover, no differences between-subgroups in H2O2 exhalation were noted at anytime ().
Figure 1. EBC H2O2 concentrations in NSCLC patients before and after the first, second, and the fourth cycle of CHT in comparison to the control group. *P < 0.05 vs. control group.
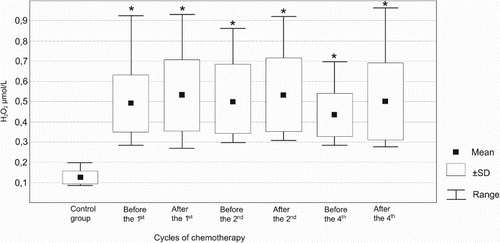
Table 2. EBC H2O2 concentrations in patients with PR, SD, and PD during CHT.
Serum TBARS levels in patients with NSCLC
The serum concentration of TBARS in the whole group of NSCLC patients at baseline (before the first CHT course) was 7.4-times higher than that in healthy controls (4.02 ± 3.54 µmol/L vs. 0.54 ± 0.25 µmol/L, P < 0.05). Relatedly, circulating TBARS observed in NSCLC patients at all subsequent occasions (after the first, before and after the second and fourth cycle of CHT) were about 8.6-, 6.7-, 9.2-, 5.7-, and 7.7-times higher than those in the healthy subjects, respectively. Although, post-CHT TBARS tended to be higher than the corresponding pre-CHT, these differences did not reach statistical significance (). Similar results were found for PR, SD, and PD subgroups (data not shown).
Table 3. Serum concentrations of TBARS in 30 NSCLC patients during CHT.
Correlations between measured variables at different time points of CHT
In NSCLC patients (n = 30), exhaled H2O2 did not significantly correlate (P > 0.05) with the neutrophil count before the first, second, and fourth cycle, r = 0.06, −0.02, and 0.20, respectively. Likewise, no correlation was found between EBC H2O2 levels and WBC count at corresponding time-points (data not shown). There were no significant associations between exhaled H2O2 and serum TBARS in the whole NSCLC group. The correlation coefficient (r) between EBC H2O2 and serum TBARS ranged between −0.12 and 0.18 (P > 0.05) for all studied time-points (before and after the first, second, and fourth cycle). Comparable results were found in the PD and PR subgroups (data not shown). In the whole NSCLC group, baseline (before the first cycle) EBC H2O2 levels significantly (P < 0.05) correlated with those after the first cycle (r = 0.46), before the second (r = 0.51), and after the second cycle (r = 0.38). Similarly, there were significant correlations between exhaled H2O2 concentrations after the first cycle as well as the concentrations noted after the second (r = 0.69) and after the fourth cycle (r = 0.46). In the subgroup of the NSCLC patients with PD, a significant correlation (P < 0.05) was noted for exhaled H2O2 after the first and second cycle (r = 0.66, n = 10) as well as for those after the second and fourth cycle (r = 0.69, n = 10). In patients who achieved PR (n = 16), the following correlations (P < 0.05) were observed: r = 0.66 for H2O2 before and after the first cycle, r = 0.52 for H2O2 before the first and the second cycle, r = 0.69 for H2O2 after the first and the second cycle and r = 0.72 for H2O2 after the second and the fourth cycle, respectively.
Discussion
We found that the NSCLC patients exhaled more H2O2 than age- and cigarette smoking habit-matched apparently healthy controls and this exhalation did not change in response to CHT. Thus, increased H2O2 exhalation by the NSCLC patients in comparison to the control group cannot be attributed to the cigarette smoking. Two factors seem to be responsible for this difference, direct ROS production via cancer cells and the inflammatory response in the tumor microenvironment. Cancer cells commonly have increased intracellular levels of H2O2 [Citation20]. Several tumor cell lines, including those in lung cancer, constitutively produced large amounts of H2O2 in vitro [Citation21]. In addition, ex vivo homogenates of cancer tissue (NSCLC and small-cell lung cancer – SCLC) released more H2O2 and contained more products of lipid peroxidation (Schiff bases and TBARS) than uninvolved lung parenchyma [Citation7].
The inflammatory response presented in the lung cancer microenvironment involving leukocytes and especially tumor-associated macrophage infiltration can be an additional important source of ROS including H2O2 in airways and subsequently in EBC. At the time of diagnosis, NSCLC patients already have increased circulatory levels of C-reactive protein [Citation22], pro-inflammatory and pro-oxidant cytokines (e.g. TNF, IL1, IL-6) [Citation23]. Furthermore, the markers of inflammation and oxidative damage to DNA were also elevated in the normal bronchial epithelium of patients with lung cancer. Thus, while typical inflammation is a self-limiting process [Citation8], cancer-related inflammation persists and can reflexively stimulate ROS overproduction and promote tumor cells growth [Citation24].
Besides cancer-related chronic inflammation, CHT itself can enhance tissue generation of ROS. Cisplatin- and carboplatin-induced ROS generation as well as suppression of antioxidant enzymes may be one of the mechanisms of anti-cancer activity and also the culprit of adverse events during CHT [Citation11,Citation25–27]. Elevated plasma levels of lipid peroxidation products, nitric oxide, and increased urinary excretion of 8-isoprostane were found in NSCLC and SCLC patients in response to cisplatin-based combination CHT [Citation11–14]. Therefore, one may suspect that lung cancer CHT may induce airway oxidative stress and subsequently increase H2O2 exhalation due to the pro-oxidant activity of platinum-based agents and vinorelbine. As a result, the positive response to CHT in reducing tumor size and cancer-related inflammation would decrease the concentration of H2O2 in the EBC of NSCLC patients. Nonetheless, the results of our study show elevated and stable H2O2 exhalation in NSCLC patients throughout CHT, which does not match this assumption. This is in line with the results monitoring serum TBARS which were elevated and did not change significantly in NSCLC patients over the study period. These results clearly show that patients with advanced lung cancer had distinct systemic and airway oxidative stress. The source of enhanced H2O2 exhalation and elevated serum TBARS in NSCLC patients appears to be different since no correlation was observed between these two variables at any of the six time-points. Dexamethasone and ondansetron (used for antiemetic treatment) inhibited the production of ROS by human neutrophils likely via the attenuation of a calcium intracellular signal [Citation28,Citation29]. In addition, dexamethasone inhibited the pro-oxidant and pro-inflammatory cytokines (e.g. TNF-α) release from alveolar macrophages isolated from resected lungs due to NSCLC [Citation30]. Such factors may have counteracted the pro-oxidant action of CHT and thus be responsible for the relatively stable levels of H2O2 exhalation in the NSCLC patients during CHT. Moreover, the significant correlations between baseline EBC H2O2 levels (before and after the first cycle) and those noted before and after the second and fourth cycle suggest no changes in the individual pattern of H2O2 exhalation in the NSCLC patients in response to CHT. These associations were also observed in the PR and PD subgroups of NSCLC patients. It indicates that patients with a high exhalation of H2O2 (within group distribution) at the study entry (before CHT) tended to have high EBC H2O2 levels after CHT independently of cancer progression or PR. Similarly, NSCLC patients with low H2O2 levels before CHT tended to have such concentrations of H2O2 in EBC over the whole study period. This suggests that individual endogenic oxidative processes could be the major determinants of exhaled H2O2 in NSCLC patients. On the other hand, the relative stability of the individual pattern of H2O2 exhalation may result from the counteracting activities of CHT and antiemetic therapy. Nonetheless, the action of other factors (such as the strong pro-oxidant effect of cigarette smoking and cancer-related inflammation) cannot be excluded. Confirmation of such an assumption would entail further studies directed at patients not receiving antiemetic therapy and those desisted from smoking for at least several months prior to the onset of observation. It was also suggested that CHT-induced suppression of the WBC count and the number of circulating neutrophils may have specifically led to the decreased EBC H2O2 levels in NSCLC patients [Citation31]. In the case of our patients, the mean neutrophil count was 1.6 and 1.3 times lower before the second cycle than it was before the first and fourth cycles. Despite this, the exhaled H2O2 did not change significantly. Moreover, no correlations between exhaled H2O2, neutrophils, and WBC count were noted at any-time point. Therefore, neutrophils are not likely the main source of exhaled H2O2 in our patients. The rise of exhaled H2O2 after the fourth CHT cycle in PR patients is in contrast to the results observed for the whole NSCLC group and PD and SD subgroups. Moreover, PR patients tended to have higher (albeit without statistical significance) EBC H2O2 levels after the first and second CHT cycle. Free radicals may be involved in the formation of platinum (cisplatin, carboplatin) – DNA adducts and antineoplastic effects [Citation32]. These drugs can also be activated by ROS to form adducts with proteins and subsequent cytostatic effects [Citation32]. Therefore, one may suppose that in patients with PR these aforementioned processes had higher intensity and resulted in tumor suppression and increased H2O2 exhalation in response to the fourth CHT cycle. The monitoring of only one exhaled marker of oxidative stress (exhaled H2O2) is an important limitation in our study. It cannot be excluded that simultaneous measurements of other oxidative stress markers in the EBC (e.g. malondialdehyde, isoprostanes, and nitric oxide) of the NSCLC patients would better describe changes in the intensity of airway peroxidative processes in relation to CHT and treatment results.
In conclusion, the present study demonstrated that patients with advanced NSCLC exhaled increased amounts of H2O2 and had elevated circulating TBARS, which suggests the occurrence of a distinct airway and systemic oxidative stress. This was not affected by CHT and was independent of treatment results and changes in the number of circulating neutrophils. Consequently, further studies are necessary to establish the clinical utility of this method in predicting a response to CHT.
Disclaimer statements
Contributors Krawczyk A: collecting data, analysing data, interpreting data, obtaining ethics approval. Nowak D: conceiving the study, writing the article in part, revising the article. Nowak P: analysing data, interpreting data. Padula G: analysing data, interpreting data. Kwiatkowska S: designing the study, writing the article, revising the article.
Funding None.
Conflict of interest The authors declare that they have no conflict of interest.
Ethics approval The study protocol was approved by the Ethics Committee of Medical University of Lodz.
Acknowledgment
The authors thank Dr Jeffrey de Graft-Johnson (Heart and Vascular Institute of North Florida, USA) for the revision of the English language.
References
- Ziech D, Franco R, Pappa A, et al. Reactive Oxygen Species (ROS)––Induced genetic and epigenetic alterations in human carcinogenesis. Mutat Res. 2011;711:167–173. doi: 10.1016/j.mrfmmm.2011.02.015
- Dizdaroglu M. Oxidatively induced DNA damage: mechanisms, repair and disease. Cancer Lett. 2012;327:26–47. doi: 10.1016/j.canlet.2012.01.016
- Dizdaroglu M, Jaruga P. Mechanisms of free radical-induced damage to DNA. Free Radic Res. 2012;46:382–419. doi: 10.3109/10715762.2011.653969
- Lee W, Thomas PS. Oxidative stress in COPD and its measurement through exhaled breath condensate. Clin Transl Sci. 2009;2:150–155. doi: 10.1111/j.1752-8062.2009.00093.x
- Chan HP, Lewis C, Thomas PS. Exhaled breath analysis: novel approach for early detection of lung cancer. Lung Cancer. 2009;63:164–168. doi: 10.1016/j.lungcan.2008.05.020
- Stolarek R, Bialasiewicz P, Krol M, et al. Breath analysis of hydrogen peroxide as a diagnostic tool. Clin Chim Acta. 2010;411:1849–1861. doi: 10.1016/j.cca.2010.08.031
- Zieba M, Suwalski M, Kwiatkowska S, et al. Comparison of hydrogen peroxide generation and the content of lipid peroxidation products in lung cancer tissue and pulmonary parenchyma. Respir Med. 2000;94:800–805. doi: 10.1053/rmed.2000.0825
- Barreiro E, Fermoselle C, Mateu-Jimenez M, et al. Oxidative stress and inflammation in the normal airways and blood of patients with lung cancer and COPD. Free Radic Biol Med. 2013;65:859–871. doi: 10.1016/j.freeradbiomed.2013.08.006
- Wakabayashi T, Kawashima T, Matsuzawa Y. Evaluation of reactive oxygen metabolites in patients with non-small cell lung cancer after chemotherapy. Multidiscip Respir Med. 2014;9:44. doi: 10.1186/2049-6958-9-44
- Marullo R, Werner E, Degtyareva N, et al. Cisplatin induces a mitochondrial-ROS response that contributes to cytotoxicity depending on mitochondrial redox status and bioenergetic functions. PLoS One. 2013;8:e81162. doi: 10.1371/journal.pone.0081162
- Srivastava AN, Gupta A, Srivastava S, et al. Cisplatin combination chemotherapy induces oxidative stress in advance non small cell lung cancer patients. Asian Pac J Cancer Prev. 2010;11:465–471.
- Johns NP, Johns JR. Assessment of 8-isoprostane (8-isoPGF2α) in urine of non-small cell lung cancer (NSCLC) patients undergoing chemotherapy. Asian Pacific J Cancer Prev. 2012;13:775–780. doi: 10.7314/APJCP.2012.13.3.775
- Nowak D, Janczak M. Effect of chemotherapy on serum end-products of lipid peroxidation in patients with small cell lung cancer: association with treatment results. Respir Med. 2006;100:157–166. doi: 10.1016/j.rmed.2005.04.002
- Gupta A, Srivastava S, Prasad R, et al. Oxidative stress in non-small cell lung cancer patients after chemotherapy: association with treatment response. Respirology. 2010;15:349–356. doi: 10.1111/j.1440-1843.2009.01703.x
- Stathopoulos D, Loukides S, Syrigos K. 8-Isoprostane in exhaled breath condensate of patients with non-small cell lung cancer: the effect of chemotherapy. Anticancer Res. 2014;34:5143–5145.
- Therasse P, Arbuck SG, Eisenhauer EA, et al. New guidelines to evaluate the response to treatment in solid tumors. European Organization for Research and Treatment of Cancer, National Cancer Institute of the United States, National Cancer Institute of Canada. J Natl Cancer Inst. 2000;92:205–216. doi: 10.1093/jnci/92.3.205
- Kwiatkowska S, Szkudlarek U, Łuczyńska M, et al. Elevated exhalation of hydrogen peroxide and circulating IL-18 in patients with pulmonary tuberculosis. Respir Med. 2007;101:574–580. doi: 10.1016/j.rmed.2006.06.015
- Kordiak J, Szemraj J, Hamara K, et al. Complete surgical resection of lung tumor decreases exhalation of mutated KRAS oncogene. Respir Med. 2012;106:1293–1300. doi: 10.1016/j.rmed.2012.06.019
- Ruch W, Cooper PH, Baggiolini M. Assay of H2O2 production by macrophages and neutrophils with homovanillic acid and horse-radish peroxidase. J Immunol Methods. 1983;63:347–357. doi: 10.1016/S0022-1759(83)80008-8
- López-Lázaro M. Dual role of hydrogen peroxide in cancer: possible relevance to cancer chemoprevention and therapy. Cancer Lett. 2007;252:1–8. doi: 10.1016/j.canlet.2006.10.029
- Szatrowski TP, Nathan CF. Production of large amounts of hydrogen peroxide by human tumor cells. Cancer Res. 1991;51:794–798.
- Scott HR, McMillan DC, Crilly A, et al. The relationship between weight loss and interleukin 6 in non-small-cell lung cancer. Br J Cancer. 1996;73:1560–1562. doi: 10.1038/bjc.1996.294
- Jungraithmayr W, Frings C, Zissel G, et al. Inflammatory markers in exhaled breath condensate following lung resection for bronchial carcinoma. Respirology. 2008;13:1022–1027.
- Quatromoni JG, Eruslanov E. Tumor-associated macrophages: function, phenotype, and link to prognosis in human lung cancer. Am J Transl Res. 2012;4:376–389.
- Ognjanović BI, Djordjević NZ, Matić MM, et al. Lipid peroxidative damage on cisplatin exposure and alterations in antioxidant defense system in rat kidneys: a possible protective effect of selenium. Int J Mol Sci. 2012;13:1790–1803. doi: 10.3390/ijms13021790
- Carozzi VA, Marmiroli P, Cavaletti G. The role of oxidative stress and anti-oxidant treatment in platinum-induced peripheral neurotoxicity. Curr Cancer Drug Targets. 2010;10:670–682. doi: 10.2174/156800910793605820
- Demkow U, Stelmaszczyk-Emmel A. Cardiotoxicity of cisplatin-based chemotherapy in advanced non-small cell lung cancer patients. Respir Physiol Neurobiol. 2013;187:64–67. doi: 10.1016/j.resp.2013.03.013
- Mikawa K, Akamatsu H, Nishina K, et al. Effects of ondansetron, granisetron, ramosetron, and azasetron on human neutrophil functions. Gynecol Obstet Invest. 2002;53:93–99. doi: 10.1159/000053001
- Coates TD, Wolach B, Tzeng DY, et al. The mechanism of action of the antiinflammatory agents dexamethasone and Auranofin in human polymorphonuclear leukocytes. Blood. 1983;62:1070–1077.
- Higham A, Lea S, Ray D, et al. Corticosteroid effects on COPD alveolar macrophages: dependency on cell culture methodology. J Immunol Methods. 2014;405:144–153. doi: 10.1016/j.jim.2014.02.003
- Wewel AR, Crusius JA, Gatzemeier U, et al. Time course of exhaled hydrogen peroxide and nitric oxide during chemotherapy. Eur Respir J. 2006;27:1033–1039.
- Fong CW. Platinum anti-cancer drugs: free radical mechanism of Pt-DNA adduct formation and anti-neoplastic effect. Free Radic Biol Med. 2016;95:216–229. doi: 10.1016/j.freeradbiomed.2016.03.006