ABSTRACT
Objectives: We tested the hypothesis that performing a rhythmic physical task accompanied by a cognitive task, such as multitask movement music therapy (MMT) involving repetitive rhythmic movement with a musical instrument (the Naruko clapper), may improve pre-frontal cortex (PFC) function and cognitive performance.
Method: Forty-five older adult participants with MCI (74.62 ± 5.05 years) participated in this randomized, controlled, single-blind intervention trial. 35 were assigned to the MMT group and 10 to the control STT group. Before and after the 12-week exercise program, we administered six physical function tests, the Frontal Assessment Battery (FAB), and measured relative oxyhemoglobin concentrations using 45-multichannel functional near-infrared spectroscopy as a reflection of hemodynamic responses in the PFC.
Results: We observed significant improvements in FAB scores only in the MMT group. Cerebral blood flow (CBF) in the PFC during the exercise was significantly increased in the MMT group compared with the STT group. The CBF increase was significantly correlated among various channels in the MMT group.
Conclusions: The MMT program appeared to stimulate the PFC and improve cognitive performance in our older adult participants with MCI, suggesting that the repetitive, rhythmic movements of MMT can activate the prefrontal area in older adults.
Trial registration: Clinical Trial Registry Numbers: R000026130, UMIN000022671 (2016/06/08) [(University Hospital Medical Information Network (UMIN) Center] retrospectively registered.
Background
Cognitive changes occur relatively slowly in healthy older adults. There is usually a 5–10-year period between the point at which cognitive testing detects cognitive decline unrelated to the normal aging process and the point at which cognitive impairment is sufficiently severe for a dementia diagnosis (Levy, Citation1994). Generally, individuals in the early stages of cognitive decline are diagnosed with mild cognitive impairment (MCI).
Palmer, Bäckman, Winblad, and Fratiglioni (Citation2008) compared individuals with and without amnestic MCI, a disorder that mainly involves memory impairment, observing that a higher proportion of individuals with amnestic MCI developed Alzheimer's disease (AD). Therefore, we believe that intervention at the stage of MCI, a prodromal condition, will prevent progression to dementia. We focused on execution function among cognitive functions.
Executive function is controlled by the prefrontal cortex (PFC), which communicates with many other brain regions (e.g. the cingulate gyrus, temporoparietal junction, and basal ganglia). A recent neuroimaging study of older adults with MCI reported that functional connectivity decreased between the medial PFC (mPFC) and other brain regions (e.g. the dorsolateral PFC [DLPFC] and cingulate gyrus) as the ability to recall personal experience became more ambiguous (Ries et al., Citation2012), highlighting the associations between PFC function, MCI, and memory impairment. Thus, the prevention of executive function disorders due to functional decline of the PFC may prevent the transition from MCI to Alzheimer's disease (AD).
Exercise therapy, a form of non-drug treatment, has received increasing attention because it has been found to stimulate the brain and thereby slow or stop the progression of dementia (Yoshitake et al. Citation1995). A report has shown that forced aerobic exercise for 45–60 min a day, four days a week for six months significantly improved the results of executive function tests in elderly people with MCI (Baker et al., Citation2010). With regard to exercise habits, the risk of developing dementia in older adults who engaged in high levels of physical activity more than three times per week was half that in older adults who did not exercise at all (Laurin, Verreault, Lindsay, MacPherson, & Rockwood, Citation2001). This indicates that the intensity and frequency of aerobic exercise plays an important role in the prevention of dementia. That study compared the effects of exercise therapy with those of occupational therapy; no music or musical instruments were involved.
However, it is difficult for older adults who are not good at exercise to voluntarily engage in such exercise four days a week for six months. In addition, it is difficult for older adults who generally do not exercise regularly to become accustomed to high levels of physical activity that exceed the speed of normal walking. For older adults who find exercise difficult, exercise interventions may cause dizziness, light-headedness, fatigue, hyperactivity, irritability, and tachycardia. Thus, using a currently available exercise intervention approach, we performed a specific intervention that facilitates regular exercise in elderly people who previously did not habitually exercise.
One solution to this issue may be movement music therapy (MMT), which has conventionally been used as a low-impact exercise intervention. Indeed, even older adults who do not exercise frequently are able to complete MMT sessions. MMT involves repetitive rhythmic patterns and synchronization with the vibratory stimulation of percussion instruments, and has been found to promote spontaneity, as well as verbal and emotional expression in older adults with dementia (Hanson, Gfeller, Woodworth, Swanson, & Garand, Citation1996). According to Bridenbaugh and Kressig (Citation2011), music therapy facilitates the development of neural circuits in the brain through the mimicry of gestures within the music via actual physical gestures. Furthermore, recognizing and repeating rhythmic elements in music through muscle exercises stimulates these neural circuits. In a study using synchronized finger tapping, Pecenka, Engel, and Keller (Citation2013) revealed an extensive brain network related to prediction during sensorimotor synchronization (SMS) with auditory pacing sequences containing tempo changes, similar to that observed in musical ensemble performances. SMS has been studied from the perspective of the organization of individual movement and the adjustment of various complex movements, and these processes are thought to involve a number of brain areas associated with SMS and learning (Zatorre & Halpern, Citation2005). Therefore, MMT that facilitates SMS is likely to stimulate a large number of brain regions.
Based on the previous studies mentioned above, we developed an MMT program for older adults using the Naruko clapper, a type of percussion instrument resembling wooden castanets that was originally used to scare birds in rice fields. In this multitask MMT intervention, participants mimic the movements of an instructor to the beat of music while using the Naruko clapper. Subjects were required to shake the Naruko clapper instrument and singing a song at the time with the music, and simultaneously follow the body movement of a trainer. This low-impact exercise simultaneously requires high levels of cognitive and motor function (Shimizu et al., Citation2013).
Therefore, we hypothesized that the multitask MMT intervention would improve PFC activity, and physical and cognitive function. To test our hypothesis in older adults with MCI, we compared the effects of MTT and STT on the following: (1) physical function post-intervention, (2) frontal lobe or cognitive function post-intervention, and (3) functional connectivity between brain regions post-intervention. We compared the effects of the multitask MMT intervention with those of a single-training task (STT) control intervention.
To visualize the stimulation of the prefrontal area, we used near-infrared spectroscopy (NIRS). Although magnetic resonance imaging (MRI) is frequently used as a non-invasive functional neuroimaging technique, it is extremely difficult to visualize brain function during exercise using MRI because the MRI apparatus restrains the head. In recent years, NIRS has received increasing attention as a functional neuroimaging modality characterized by non-invasiveness and high mobility. NIRS quantifies hemoglobin concentrations in brain tissues to measure blood and oxygen metabolism. Lee, Folley, Gore, and Park (Citation2008) reported similar cortical function measured with NIRS and functional MRI during working memory tasks. If found to be successful, the MMT intervention may represent a method for effectively stimulating brain activity in older adults with MCI, an early manifestation of dementia.
Methods
Participants
We recruited individuals who voluntarily attended a prevention of dementia care class in 2014 provided by the municipality of Eiheiji in Fukui Prefecture, Japan. Participants completed a preventive care checklist that focused on memory impairment, constructed with reference to the definition of MCI given by Petersen (Citation2004) and Petersen et al (Citation1999). We invited 45 volunteers who checked ≥ 1 item on the memory impairment checklist in the health check section () to attend a meeting at a venue close to their home. At this meeting, they were assigned to either the MMT group or STT control group, and given a detailed explanation about the purpose, method, publication, and risks of the study from the researchers (). The MMT group participated in a multitask MMT program consisting of exercise therapy with music and use of the Naruko clapper. The STT group participated in exercise sessions that involved the same movements as those in the MMT group. However, in these sessions, the movements were counted out by the instructor (single task) without accompanying background music or the Naruko clapper.
Table 1. The cognitive function checklist in the health check section of the preventive care checklist.
This randomized controlled trial was conducted at three public health centers located in Eiheiji. The staff members assessing the outcomes were blinded to the intervention received. All participants gave written informed consent after receiving an explanation of the study and before commencing the trial. Written informed consent was obtained from all participants in accordance with the research protocol approved by the ethics committee of the Ishikawa Prefectural Nursing University (KANDAI#338).
Intervention protocol
In the MMT intervention group, each MMT session started with a 20 min warm-up period including about 5 min of light exercise completed in synchronization with background music. The next 15 min involved exercises with background music that aimed to strengthen leg muscles for fall prevention. This was followed by 30 min of MMT during which the participants used the Naruko clapper to accompany ‘Yosakoi Icchorai Ondo,’ a song familiar to residents of Fukui Prefecture. The song was played for 4 min, followed by ‘March of 365 steps’ (3 min), ‘Hakodatenohito’ (3 min), ‘Palm in the sun’ (3 min), ‘Kiyoshi's Zundoko’ (4 min), etc. Each session, we selected 2 songs and repeated them for 30 min.
These songs were repeated three times per one song, two songs per a session. It included an explanation of each move, times to wipe sweat and to water supply between song and song for 2 min. describes the songs and times. The session ended with relaxation exercises, as well as deep breathing and massage to accompanying background music.
Table 2. Schedule of the MMT exercise program using the Naruko clapper and the control exercise program.
In the STT control group, an instructor guided the participants through the same exercises performed in the MMT group by giving counts only, without accompanying background music or use of the Naruko clapper (). Both interventions were conducted once a week for 12 weeks.
Assessment measures
Demographics and physical indicators were administered in the pre- and post-interventions. In the 1st and 12th sessions, cognitive tests were performed.
Demographics
Participants completed a self-report questionnaire that assessed household status, chief medical complaint, medical and family history, and presence/absence of a primary physician.
Physical indicators
Physical function tests
We assessed physical function using measures of systolic blood pressure (SBP), diastolic blood pressure (DBP), body weight (BW), body balance (standing on one leg with eyes open), flexibility (sit-and-reach test), gait (maximum walking speed), muscle endurance (30 s Chair Stand Test: CS-30), muscle strength (grip strength in both hands), and functional mobility (timed Up & Go test).
Body balance: in the one-leg standing test with eyes open (Briggs, Gossman, Birch, Drews, & Shaddeau, Citation1989), participants were instructed to stand with their hands on their waist, raise an arbitrarily chosen foot approximately 20 cm from the floor, and keep their balance. We recorded the time taken to loss of balance (maximum: 120 s). Loss of balance was defined as shifting the sole of the non-lifted foot from its original position, removing the hands from the waist, or touching the floor with any body part except for the pivot leg. The test was repeated twice, with the higher value recorded for analysis.
Flexibility: in the sit-and-reach test (Jones, Rikli, Max, & Noffal, Citation1998), participants were instructed to sit on the floor with their legs stretched out straight in front of them with their knees locked. They were asked to reach forward as far as possible with their hands placed on top of a digital sit-and-reach instrument box (T.K.K.5112; Takei Scientific Instruments Co., Ltd., Niigata, Japan), and the distance they could reach was recorded.
Gait: maximum walking speed, defined as the time taken to walk 10 m in a straight line after being instructed to walk as quickly as possible, was recorded with a walking measurement instrument equipped with a stopwatch function (T.K.K.5801; Takei Scientific Instruments Co., Ltd). The shorter time of two trials was recorded for analysis.
Muscle endurance: we assessed muscle strength in the lower extremities with the CS-30 (Jones, Rikli, & Beam, Citation1999), a safe and effective test for older adults. The test began with participants seated on a chair with their back straight, feet flat on the floor approximately a shoulder width apart, and arms crossed against the chest. At the ‘go’ signal, they rose to a full standing position (body erect and straight) before returning to the original sitting position. They were instructed to complete as many full stands as possible within 30 s. The score was the total number of stands executed.
Muscle strength: to assess grip strength in both hands, the participants held digital handgrip dynamometers (T.K.K.5401; Takei Scientific Instruments Co., Ltd.) in each hand at the sides of the body and squeezed the devices as hard as they could during expiration. They were instructed not to move their hands or touch the dynamometer to their body. The values for each hand were analyzed.
Functional mobility: in the timed Up & Go test (Podsiadlo & Richardson, Citation1991), we measured the time taken for participants to stand up from a chair, walk 3 m, turn around, walk back to the chair, and sit down again. They were instructed to walk in a straight line at a comfortable and safe pace.
Cognitive tests
Frontal Assessment Battery (FAB)
The FAB assesses frontal lobe function using 6 tasks that are associated with the prefrontal region: similarities (conceptualization); lexical fluency (mental flexibility); motor series (motor programming); conflicting instructions (sensitivity to interference); Go–No Go (inhibitory control); and prehension behavior (environmental autonomy) (Benton, Citation1968; Dubois, Slachevsky, Litvan, & Pillon, Citation2000). The total score for the six tests is 18 points (each test score is 3 points perfect). The FAB is frequently used in clinical situations as it is easy to conduct, takes <15 min to complete, and produces easily interpreted results because of the small score range.
CBF measurement
We performed functional neuroimaging with NIRS (fNIRS). We used a functional near-infrared spectroscope (LABNIRS, Shimadzu Co., Kyoto, Japan) to irradiate near-infrared wavelengths of 780, 805, and 830 nm through the scalp. We measured CBF (task-related changes in the concentration of oxygenated hemoglobin (oxy-Hb) in mMmm) pre- and post-intervention in the MMT and STT groups. In each intervention group, we measured CBF for 120 s starting 30 s pre-intervention, throughout the intervention (which lasted 60 s), and until 30 s post-intervention. This enabled us to capture changes in the integral values of oxy-Hb concentration, which correlates with changes in neural activity in the brain ().
Figure 2. Measurement methods conducted during intervention (intervention and control groups were separated).
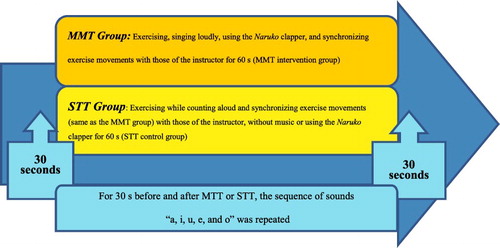
During the 30-s pre and post-intervention periods, participants were instructed by researchers, who stood next to the participants, to repeatedly pronounce Japanese kana sounds ‘a, i, u, e, o’ without moving their body, to keep their gaze fixed on a cross in front of them, and to not think of anything. Subsequently, this baseline brain activity (associated with the pronunciation of sounds without meaning or body movement [twice for 30 s each] and expressed as the integral oxy-Hb value) could be subtracted from the intervention-related brain activity (Kameyama et al., Citation2006; Suto, Fukuda, Ito, Uehara, & Mikuni, Citation2004).
Study period
The study was performed over a 14-week (including pre- and post-test, intervention performed for 12 weeks, ) period from July to October 2014. Participant characteristics, physical indicators, and FAB score at each of the three study venues were collected at sessions 1 and 12. CBF was also measured at sessions 1 and 12 ().
Data analysis
Demographics, physical performance, and frontal lobe function
At baseline, we compared participant characteristics between MMT and STT groups with the Student's t-test, while physical indicators and FAB scores pre- and post-intervention for each group were compared with paired t-tests. Then, MTT and STT groups’ physical indicators, FAB scores differences of pre-and post-intervention were compared with one-way ANOVAs. Adjustments for multiple testing were made using Bonferroni's correction.
CBF measurements
Channel locations. With no corresponding MRI images, probabilistic regression can be used to register fNIRS channel locations onto standard brain coordinates developed by the Montreal Neurological Institute (MNI) in line with the International 10–20 system (Singh, Okamoto, Dan, Jurcak, & Dan, Citation2005). In fNIRS with a 3D digitizer, a flexible adjustable surface holder (Shimadzu Co.) is placed in the frontal process zone (FPZ) to direct a light-transmitting probe 7 (T7) to that zone (). We then used Statistical Parametric Mapping for Near-infrared Spectroscopy software (NIRS-SPM) to convert the channel locations into MNI coordinates projected onto the brain surface ().
CBF alterations
We measured differences scores for task-related changes in oxy-Hb concentration before and after the MMT and STT interventions. Prior to analyzing the data with a multivariate analysis of variance (MANOVA with Bonferroni correction), channels were divided into three groups based on the MNI coordinates in seven channels each in the left and right temporal lobes, and 15 channels in the prefrontal lobe.
Intervention-related activation of a network of interacting brain regions
We analyzed pre- and post-intervention oxy-Hb concentration (in several brain regions at 45 channels) difference scores using Pearson's correlation coefficients in the MMT and STT groups. The data were then used to extract and compare networks of brain regions activated during each intervention. Statistical analysis was performed using SPSS 21.0 J for Windows (IBM, Tokyo, Japan), with significance set at p < .05.
Results
Participant characteristics
Of the 45 participants who consented to the study, 39 (mean age: 74.64 ± 4.82 years) completed both pre- and post-intervention assessments (), with 30 participants in the MMT group (mean age: 74.90 ± 4.29 years) and 9 in the STT group (mean age: 73.33 ± 7.31 years).
Table 3. Participant characteristics.
As shown in , we observed no significant differences in age, physical indicators, or FAB scores between the MMT and STT groups, and no significant differences in intervention program attendance between the groups. We thought that the SD of the age of the control group was likely larger due to the smaller ‘n.’ In any case, the range of age between the two groups was nearly the same, so it is unlikely that the result would have a large influence. Furthermore, we found no significant differences in CBF before for any of the 45 channels (MANOVA).
Table 4. Results of physical function tests at baseline in the MMT and STT groups.
Physical function pre- and post-intervention
We compared pre- and post-intervention results for the physical function tests (body balance, flexibility, gait, muscle endurance, muscle strength, and functional mobility) in each intervention group (). In the MMT group, we observed a significant improvement in the four areas of flexibility, functional mobility, gait, and muscle endurance after intervention. The STT group showed no significant differences.
Table 5. Results of physical function tests before and after interventions in each group (p < .05).
To assess the effects of the MMT intervention, we compared pre- and post-intervention differences in each group using one-way ANOVA. While the MMT group maintained their body balance score, the STT group showed a significantly decreased score.
FAB score and bilateral frontal lobe activation pre- and post-intervention
Changes in FAB scores indicated a significant improvement in the MMT group but not in the STT group after the intervention, although there were no significant between-group differences in FAB score improvements in the presence/absence of the MMT intervention (ANOVA) ().
Moreover, CBF was significantly higher in the MMT group at channels 22 and 24 compared with that in the STT group. According to the manufacturer's data for NIRS-SPM, activity at channels 22 and 24 reflects activity in the mPFC (Brodmann area 10; BA10) ( and ). The MMT group also had significantly higher activation in six of seven locations in the left brain, four of seven in the right brain, and all 15 regions in the forehead compared with the STT group (–).
Table 6. Pre- and post-intervention results for the six subsets of the Frontal Assessment Battery in each group (p < .05).
Table 7. Pre- and post-intervention differences in integral value (oxy-HbTable Footnotea) at channels in the left temporal region, i.e. task-related changes in the integral values of oxy-Hb concentrations (p < .05)
Table 8. Pre- and post-intervention differences in integral value (oxy-HbTable Footnotea) at channels in the right temporal region (p < .05).
Table 9. Pre- and post-intervention differences in integral value (oxy-HbTable Footnotea) at channels in the frontal temporal region (p < .05).
Temporal and prefrontal activation
We compared correlations in CBF values between individual channels in the bilateral PFC between the MMT and STT groups (Pearson's correlation coefficient). Channels that were significantly different are connected by a line in and .
Figure 7. Pre- and post-intervention brain blood flow and correlations between channels in the MMT group (p < .05).
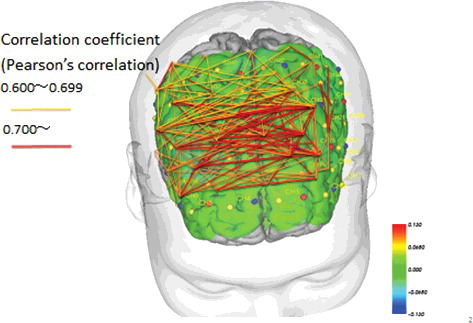
Figure 8. Pre- and post-intervention brain blood flow and correlations between channels in the STT group (p < .05).
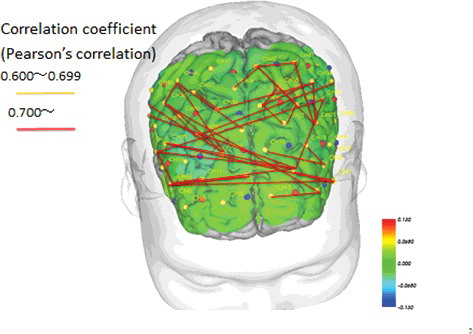
The results showed that in the MMT group only, there were similar changes in CBF after the intervention in several areas across the brain, ranging from the bilateral temporal lobe to the DLPFC and PFC (). One channel in the MMT group was correlated with a number of other channels, acting like a hub. However, in the STT group, we observed comparatively fewer correlations between channels. These findings suggest minimal correlation between the DLPFC and mPFC ( and ).
Discussion
Improved physical function
In this study, we found significant post-intervention improvements in four categories of physical function among individuals in the MMT intervention group, indicating that this intervention improved physical function. However, there were no significant differences in improvement compared with the STT control intervention that were specifically related to the presence/absence of the MMT intervention. This result may be due to both interventions involving exercise, such that both interventions have similar effects on physical function. Future studies could include comparative analyses that measure change in physical function with control groups that do not receive any exercise intervention.
In addition, our result revealed a significant difference in post-intervention body balance between the MMT and STT groups. This significant difference may have resulted because of a post-intervention decrease in the control group and an upward trend in the MMT group. Body balance is an important physical function for older adults, especially with respect to outdoor activities, and is thought to be stronger in older adults who are active (DiPietro, Citation1996). In the present study, body balance was maintained in the MMT group.
The MMT intervention used in this study required movement and cognitive function at the same time, specifically those involved in performing repetitive rhythmic movements in synchrony with musical rhythms while playing the Naruko clapper and following the movements of an instructor (Priest, Karageorghis, & Sharp, Citation2004). It has been reported that walking in a rhythmic manner engages different brain areas to those involved in finely controlled irregular or non-rhythmic movements (Hayden, Clair, Johnson, & Otto, Citation2009). As such, performing repetitive rhythmic movements may decrease the working load in specific brain areas and free up cognitive capacity (Hayden et al., Citation2009). Music may be a useful means of promoting rhythmic activity in exercise. While this is outside the results of the present study, it is supported by a host of previous research (Clair 1996; Reid et al., Citation2014; Satoh et al., Citation2017; Tabei et al., Citation2017).
FAB scores and frontal lobe activation
The FAB assesses cognitive function in the frontal lobe. In this study, we found a significant improvement in FAB test scores after the MMT intervention. However, we found no significant difference in FAB scores between the two groups that was due to the presence/absence of the MMT intervention. Future studies should further examine relationships between exercise interventions and cognitive function in larger numbers of participants.
In this study, we observed a significant post-intervention increase in mPFC activation in the MMT group but not in the STT group. Schecklmann, Ehlis, Plichta, and Fallgatter (Citation2008) conducted a verbal fluency task for 3 weeks with healthy older adults, observing that individuals not engaged in any physical or other cognitive activities during the study period had reduced CBF after 3 weeks. MMT constitutes a multitask exercise program; subjects were required to shake the Naruko clapper instrument and singing a song at the time with the music, and simultaneously follow the body movement of a trainer. As subjects in the MMT group were required to pay attention to the timing of the Naruko throughout the exercise, MMT may have involved a higher level of cognitive performance than the control intervention involving a single physical task (Shimizu et al., Citation2013). Thus, the combination of rhythmic movement with cognitive functioning in our specific MMT intervention may have been necessary to activate the prefrontal area to the extent observed in the present study. As mentioned, we observed decreased CBF (concentration of oxy-Hb) after 3 weeks without MMT, suggesting that increased CBF following the MMT intervention may have resulted from brain activation.
We also observed increased CBF after the MMT intervention, particularly in the mPFC (channels 22 and 24; ). Given the connectivity that exists between the mPFC and various brain regions (e.g. the motor cortex, cingulate gyrus, and basal ganglia), our findings suggest that interventions simultaneously requiring both physical and cognitive functions, such as our MMT program, activate many brain regions. Therefore, it is logical that increased activity was observed in the mPFC, which integrates such functions.
We observed significant improvements in motor programming in the MMT group after the intervention (p = 0.021; r = 0.414). The fist-edge palm task (FEPT) is part of the motor series (motor programming) of the FAB. FEPT is a motor programming task first described by Luria in 1966 (Dubois et al., Citation2000). Originally, the test was used to evaluate motor dysfunction, but it has since been used to assess frontal lobe function, particularly to screen for executive function, sequential components, imitation suppression, and physical function (Duncan, Citation1997; Haaland, Elsinger, Mayer, Durgerian, & Rao, Citation2004). The FEPT assesses motor programming and planning, and the frontal lobe is considered to be responsible for such functions (Dubois et al., Citation2000). Recent studies have revealed the importance of the motor cortex for the implementation of complex motor sequences, which are subsequently integrated in the parietal lobe (Bengtsson et al., Citation2009; Rizzolatti, Fadiga, Gallese, & Fogassi, Citation1996).
In the MMT, in addition to sensorimotor exercise participants must make accurate but transient adjustments to interpersonal behavior as they predict and mimic the movements of the instructor. This SMS task (requiring two tasks to be completed simultaneously) requires a high level of prediction (predicting the timing of the instructor's movements), and is therefore more difficult than simply dancing to music. As such, our MMT intervention may contribute more to brain activation compared with the STT intervention, which involves performing a single task. Because MMT requires SMS, our observed improvement in motor sequence tasks after the MMT intervention may imply neural interactions within the network comprising the premotor cortex, prefrontal association area, anterior cerebellum, supplementary motor area (SMA), and basal ganglia (Zatorre & Halpern, Citation2005). It may also suggest that MMT interventions facilitate improvements in motor programming.
In studies of interventions that affect brain activation, exercise has been shown to effectively activate the prefrontal lobe (Hasson, Ghazanfar, Galantucci, Garrod, & Keysers, Citation2012; Lindenberger, Li, Gruber, & Müller, Citation2009; Pecenka et al., Citation2013). However, no previous studies have shown that, compared with single-task exercise interventions, a 3-month multitask exercise intervention requiring rhythmic movement with use of a musical instrument led to increased cognitive and physical function in the frontal cortex. Therefore, this study is the first to report an association between 3 months of continuous and repetitive MMT and improved CBF in the premotor cortex and prefrontal association area.
Intervention-related activation of a network of interacting brain regions
Our analysis of altered CBF in individual channels during MMT and STT revealed that CBF changes were positively correlated between many channels in the MMT intervention group. This indicates that the MMT intervention increased functional connectivity in prefrontal areas more than the STT intervention in individuals with MCI. That the MMT was continuous and repetitive may have been an important factor in the observed increased activation of brain neural networks. Specifically, we observed a significant post-intervention increase in mPFC (at the positions of 14, 15, 16, 22, 24, and 25 channels) activation in the MMT group but not in the STT group. Functional brain imaging studies have revealed altered activation in the frontal and temporal cortices associated with working memory in patients with MCI. In patients with MCI compared with healthy controls, there was significantly decreased activation in the left frontal, right superior frontal, and left temporal lobes (Ogawa, Kotani, & Jimbo, Citation2014). Our results showed increased CBF and activity in various brain regions after MMT. This is a meaningful result for elderly with MCI who have decreased CBF blood flow.
When comparing MMT and STT, it is important to consider that CBF is much higher when an individual is attempting to synchronize their movements with those of another person (Hasson et al., Citation2012). Accordingly, synchronizing one's movements with those of another person while predicting that person's feelings or movements serves to further activate neural connectivities in the brain, compared with moving to predictable movements while counting. Similar findings have been reported for activation of brain neural networks in SMS tasks (Zatorre & Halpern, Citation2005). Imaging studies of brain function designed to measure timing in exercise have revealed links between several cortical and subcortical regions, including the cerebellum and basal ganglia, as well as the supplementary motor area (Zatorre & Halpern, Citation2005). The present data also support such findings.
There are several important limitations to this study. There were fewer participants in the control STT group than in the MMT group. Initially, we planned to balance the sizes of the intervention and control groups. However, following a request from the municipality that implemented this study, the size of the intervention group was increased. In addition, the size of the control group was decreased because the families of participants in the control group were required to transport the participants to the elderly care centers and were not able to do so (–) (Faul, Erdfelder, Lang, & Buchner, Citation2007; Field & Hole, Citation2003).
In addition, it would be useful to examine a third group of participants who did not undergo any intervention during the study period. Finally, a longer-term study would be beneficial to examine which intervention effects are maintained and for how long.
Conclusions
In this 3-month intervention study, consisting of weekly 60 min sessions, physical function (body balance) was maintained in older adults with MCI by an MMT using the Naruko clapper but not by a simple STT exercise program. MMT was shown to increase FAB scores and activate the mPFC, which in turn resulted in improved functional connectivity in the PFC. These findings suggest that the prefrontal area can be activated in individuals with MCI by repetitive, rhythmic movements in MMT, thereby improving executive function associated with this region. Therefore, our MMT program appears to be an effective intervention for enhancing neural activation in older adults with MCI.
Cognitive function declines with MCI. However, by activating neural networks related to cognitive function with interventions such as MMT, it may be possible to slow this decline.
Acknowledgments
We thank all staff at the Eiheiji Regional Comprehensive Support Center and all the research participants from Eiheiji Town in Fukui Prefecture who partook in this study.
Disclosure statement
The authors declare that they have no competing interests.
Additional information
Funding
References
- Baker, L. D., Frank, L. L., Foster-Schubert, K., Green, P. S., Wilkinson, C. W., McTiernan, A., … Craft, S. (2010). Effects of aerobic exercise on mild cognitive impairment: A controlled trial. Archives of Neurology, 67(1), 71–79. doi:10.1001/archneurol.2009.307
- Bengtsson, S. L., Ullén, F., Ehrsson, H. H., Hashimoto, T., Kito, T., Naito, E., … Sadato, N. (2009). Listening to rhythms activates motor and premotor cortices. Cortex, 45(1), 62–71. doi:10.1016/j.cortex.2008.07.002
- Benton, A. L. (1968). Differential behavioral effects in frontal lobe disease. Neuropsychologia, 6(1), 53–60. doi:10.1016/0028-3932(68)90038-9
- Bridenbaugh, S. A., & Kressig, R. W. (2011). Laboratory review: The role of gait analysis in seniors' mobility and fall prevention. Gerontology, 57, 256–264. doi:10.1159/000322194
- Briggs, R. C., Gossman, M. R., Birch, R., Drews, J. E., & Shaddeau, S. A. (1989). Balance performance among noninstitutionalized elderly women. Physical Therapy, 69, 748–756.
- Clair, A. A. (1996). Therapeutic uses of music with older adults; in music in physical exercise, pp. 151–167. Maryland: Health Professions Press.
- DiPietro, L. (1996). The epidemiology of physical activity and physical function in older people. Medicine and Science in Sports and Exercise, 28, 596–600. doi:10.1249/00005768-199605000-00010
- Dubois, B., Slachevsky, A., Litvan, I., & Pillon, B. (2000). The FAB: A frontal assessment battery at bedside. Neurology, 55, 1621–1626. doi:10.1212/WNL.55.11.1621
- Duncan, R. (1997). The clinical use of SPECT in focal epilepsy. Epilepsia, 38(S10), 39–41. doi:10.1111/j.1528-1157.1997.tb00091.x
- Faul, F., Erdfelder, E., Lang, A.-G., & Buchner, A. (2007). G*Power 3. A flexible statistical power analysis program for the social, behavioral, and biomedical sciences. Behavior Research Methods, 39, 175–191.
- Field, A., & Hole, G. (2003). How to design and report experiments. London: Sage Publications.
- Haaland, K. Y., Elsinger, C. L., Mayer, A. R., Durgerian, S., & Rao, S. M. (2004). Motor sequence complexity and performing hand produce differential patterns of hemispheric lateralization. Journal of Cognitive Neuroscience, 16, 621–636. doi:10.1162/089892904323057344
- Hanson, N., Gfeller, K., Woodworth, G., Swanson, E. A., & Garand, L. (1996). A comparison of the effectiveness of differing types and difficulty of music activities in programming for older adults with Alzheimer's disease and related disorders. Journal of Music Therapy, 33(2), 93–123. doi:10.1093/jmt/33.2.93
- Hasson, U., Ghazanfar, A. A., Galantucci, B., Garrod, S., & Keysers, C. (2012). Brain-to-brain coupling: A mechanism for creating and sharing a social world. Trends in Cognitive Sciences, 16(2), 114–121. doi:10.1016/j.tics.2011.12.007
- Hayden, R., Clair, A. A., Johnson, G., & Otto, D. (2009). The effect of rhythmic auditory stimulation (RAS) on physical therapy outcomes for patients in gait training following stroke: A feasibility study. International Journal of Neuroscience, 119, 2183–2195. doi:10.3109/00207450903152609
- Jones, C. J., Rikli, R. E., & Beam, W. C. (1999). A 30-s chair-stand test as a measure of lower body strength in community-residing older adults. Research Quarterly for Exercise and Sport, 70(2), 113–119. doi:10.1080/02701367.1999.10608028
- Jones, C. J., Rikli, R. E., Max, J., & Noffal, G. (1998). The reliability and validity of a chair sit-and-reach test as a measure of hamstring flexibility in older adults. Research Quarterly for Exercise and Sport, 69, 338–343. doi:10.1080/02701367.1998.10607708
- Kameyama, M., Fukuda, M., Yamagishi, Y., Sato, T., Uehara, T., Ito, M., … Mikuni, M. (2006). Frontal lobe function in bipolar disorder: A multichannel near-infrared spectroscopy study. NeuroImage, 29(1), 172–184. doi:10.1016/j.neuroimage.2005.07.025
- Laurin, D., Verreault, R., Lindsay, J., MacPherson, K., & Rockwood, K. (2001). Physical activity and risk of cognitive impairment and dementia in elderly persons. Archives of Neurology, 58, 498–504. doi:10.1001/archneur.58.3.498
- Lee, J., Folley, B. S., Gore, J., & Park, S. (2008). Origins of spatial working memory deficits in schizophrenia: An event-related fMRI and near-infrared spectroscopy study. PLoS One, 3, e1760. doi:10.1371/journal.pone.0001760
- Levy, R. (1994). Aging-associated cognitive decline. Working Party of the International Psychogeriatric Association in collaboration with the World Health Organization. International Psychogeriatrics, 6(1), 63–68. doi:10.1017/S1041610294001626
- Lindenberger, U., Li, S. C., Gruber, W., & Müller, V. (2009). Brains swinging in concert: Cortical phase synchronization while playing guitar. BMC Neuroscience, 10, 22. doi:10.1186/1471-2202-10-22
- Ogawa, Y., Kotani, K., & Jimbo, Y. (2014). Relationship between working memory performance and neural activation measured using near-infrared spectroscopy. Brain and Behavior, 4(4), 544–551. doi:10.1002/brb3.238
- Palmer, K., Bäckman, L., Winblad, B., & Fratiglioni, L. (2008). Mild cognitive impairment in the general population: Occurrence and progression to Alzheimer disease. The American Journal of Geriatric Psychiatry, 16, 603–611. doi:10.1097/JGP.0b013e3181753a64
- Pecenka, N., Engel, A., & Keller, P. E. (2013). Neural correlates of auditory temporal predictions during sensorimotor synchronization. Frontiers in Human Neuroscience, 7, 380. doi:10.3389/fnhum.2013.00380
- Petersen, R. C. (2004). Mild cognitive impairment as a diagnostic entity. Journal of Internal Medicine, 256(3), 183–194. doi:10.1111/j.1365-2796.2004.01388.x
- Petersen, R. C., Smith, G. E., Waring, S. C., Ivnik, R. J., Tangalos, E. G., & Kokmen, E. (1999). Mild cognitive impairment: Clinical characterization and outcome. Archives of Neurology, 56, 303–308. doi:10.1001/archneur.56.3.303
- Podsiadlo, D., & Richardson, S. (1991). The timed “Up & Go”: A test of basic functional mobility for frail elderly persons. Journal of the American Geriatrics Society, 39, 142–148.
- Priest, D. L., Karageorghis, C. I., & Sharp, N. C. (2004). The characteristics and effects of motivational music in exercise settings: The possible influence of gender, age, frequency of attendance, and time of attendance. The Journal of Sports Medicine and Physical Fitness, 44, 77–86.
- Reid, K. F., Pasha, E., Doros, G., Clark, D. J., Patten, C., Phillips, E. M., … Fielding, R. A. (2014). Longitudinal decline of lower extremity muscle power in healthy and mobility-limited older adults: Influence of muscle mass, strength, composition, neuromuscular activation and single fiber contractile properties. European Journal of Applied Physiology, 114(1), 29–39.
- Ries, M. L., McLaren, D. G., Bendlin, B. B., Guofanxu, Rowley, H. A., Birn, R., … Johnson, S. C. (2012). Medial prefrontal functional connectivity–relation to memory self-appraisal accuracy in older adults with and without memory disorders. Neuropsychologia, 50, 603–611. doi:10.1016/j.neuropsychologia.2011.12.014
- Rizzolatti, G., Fadiga, L., Gallese, V., & Fogassi, L. (1996). Premotor cortex and the recognition of motor actions. Brain Research. Cognitive Brain Research, 3(2), 131–141. doi:10.1016/0926-6410(95)00038-0
- Satoh, M., Ogawa, J. I., Tokita, T., Nakaguchi, N., Nakao, K., Kida, H., & Tomimoto, H. (2017). Physical exercise with music maintains activities of daily living in patients with dementia: Mihama-Kiho project part 21. Journal of Alzheimers Disease, 57(1), 85–96.
- Schecklmann, M., Ehlis, A. C., Plichta, M. M., & Fallgatter, A. J. (2008). Functional near-infrared spectroscopy: A long-term reliable tool for measuring brain activity during verbal fluency. NeuroImage, 43(1), 147–155. doi:10.1016/j.neuroimage.2008.06.032
- Shimizu, N., Umemura, T., Hirai, T., Tamura, T., Sato, K., & Kusaka, Y. (2013). Effects of movement music therapy with the Naruko clapper on psychological, physical and physiological indices among elderly females: A randomized controlled trial. Gerontology, 59, 355–367. doi:10.1159/000346763
- Singh, A. K., Okamoto, M., Dan, H., Jurcak, V., & Dan, I. (2005). Spatial registration of multichannel multi-subject fNIRS data to MNI space without MRI. NeuroImage, 27, 842–851. doi:10.1016/j.neuroimage.2005.05.019
- Suto, T., Fukuda, M., Ito, M., Uehara, T., & Mikuni, M. (2004). Multichannel near-infrared spectroscopy in depression and schizophrenia: Cognitive brain activation study. Biological Psychiatry, 55, 501–511. doi:10.1016/j.biopsych.2003.09.008
- Tabei, K. I., Satoh, M., Ogawa, J. I., Tokita, T., Nakaguchi, N., Nakao, K., … Tomimoto, H. (2017). Physical exercise with music reduces gray and white matter loss in the frontal cortex of elderly people: The Mihama-Kiho scan project. Front Aging Neuroscience, 7(9), 174. doi:10.3389/fnagi.2017.00174 eCollection 2017.
- Yoshitake, T., Kiyohara, Y., Kato, I., Ohmura, T., Iwamoto, H., Nakayama, K., … Fujishima, M. (1995). Incidence and risk factors of vascular dementia and Alzheimer's disease in a defined elderly Japanese population: the Hisayama study. Neurology, 45, 1161–1168. doi:10.1212/WNL.45.6.1161
- Zatorre, R. J., & Halpern, A. R. (2005). Mental concerts: Musical imagery and auditory cortex. Neuron, 47(1), 9–12. doi:10.1016/j.neuron.2005.06.013