Abstract
Non-alcoholic fatty liver disease (NAFLD) is associated with cardiovascular disease (CVD) and both are prevalent in men with testosterone deficiency. Long-term effects of testosterone therapy (TTh) on NAFLD are not well studied. This observational, prospective, cumulative registry study assesses long-term effects of testosterone undecanoate (TU) on hepatic physiology and function in 505 hypogonadal men (T levels ≤350 ng/dL). Three hundred and twenty one men received TU 1000 mg/12 weeks for up to 12 years following an initial 6-week interval (T-group), while 184 who opted against TTh served as controls (C-group). T-group patients exhibited decreased fatty liver index (FLI, calculated according to Mayo Clinic guidelines) (83.6 ± 12.08 to 66.91 ± 19.38), γ-GT (39.31 ± 11.62 to 28.95 ± 7.57 U/L), bilirubin (1.64 ± 4.13 to 1.21 ± 1.89 mg/dL) and triglycerides (252.35 ± 90.99 to 213 ± 65.91 mg/dL) over 12 years. Waist circumference and body mass index were also reduced in the T-group (107.17 ± 9.64 to 100.34 ± 9.03 cm and 31.51 ± 4.32 to 29.03 ± 3.77 kg/m2). There were 25 deaths (7.8%) in the T-group of which 11 (44%) were cardiovascular related. In contrast, 28 patients (15.2%) died in C-group, and all deaths (100%) were attributed to CVD. These data suggest that long-term TTh improves hepatic steatosis and liver function in hypogonadal men. Improvements in liver function may have contributed to reduced CVD-related mortality.
Introduction
In recent decades, the prevalence of hepatic steatosis has increased substantially, reaching 10–24% in the overall global population, and is directly associated with the increased incidence of type 2 diabetes (T2D) and obesity that afflicts western societies [Citation1]. Hepatic steatosis is characterised by fat deposition in the liver and is considered the precursor to non-alcoholic fatty liver disease (NAFLD). As NAFLD progresses, liver inflammation and damage can occur ultimately leading to cirrhosis, liver failure and often hepatocarcinoma. Hepatic steatosis linearly correlates with incidence of T2D and metabolic syndrome (MetS), obesity and key features of these diseases including insulin resistance, hyperinsulinemia and dyslipidemia [Citation2–4]. NAFLD prevalence ranges from 50% to 75% in patients with T2D [Citation5–8] and from 80% to 90% in obese patients [Citation9–11]. Hepatic steatosis increases CVD risk and cardiovascular events in patients with T2D (odds ratio 1.84) [Citation12–15]. Indeed, with their shared aetiology hepatic steatosis and atherosclerosis are often considered tissue specific manifestations of the same cardiometabolic pathology [Citation16].
In addition to the characteristic symptoms of functional hypogonadism such as impaired libido, erectile dysfunction, fatigue, increased risk of depression and reduced quality of life (QoL) [Citation17], low testosterone is associated with T2D, MetS and is considered an independent cardiovascular risk factor [Citation18–20]. T2D is prevalent in men with low testosterone levels and increases the risk of cardiovascular disease (CVD) in this population [Citation21,Citation22]. Testosterone therapy (TTh), the primary treatment for alleviating symptoms of functional hypogonadism, has been reported to reduce insulin resistance, dyslipidemia and central adiposity, improve glycaemic control and consequently decrease cardiovascular risk in hypogonadal men with T2D and/or MetS [Citation23–28].
A limited number of studies have explored the relationship between hepatic steatosis and testosterone often with conflicting results. Some population-based studies report a correlation between low circulating serum testosterone levels and hepatic steatosis [Citation29–32], whereas others found no association [Citation33]. Hepatic steatosis, defined by sonographic criteria, has been demonstrated to be directly correlated with a total testosterone level lower than 14.2 nmol/L in men in a retrospective cohort study even after adjusting for confounding factors including age, BMI, smoking, diabetes, and visceral adipose tissue [Citation29]. Furthermore, it was reported that low total testosterone levels in healthy Korean men were inversely associated with NAFLD and this association persisted after controlling for the effect of visceral adiposity, insulin resistance and low grade inflammation, indicating an independent correlation [Citation30]. In obese men with sleep apnoea, 18 weeks of TTh led to improvements in insulin sensitivity and reduced liver fat content assessed by CT imaging despite the men not being initially selected for low testosterone [Citation34]. Supporting these clinical findings, several animal studies have also demonstrated beneficial effects of testosterone on hepatic steatosis. Hepatic lipid deposition is elevated in animal models of testosterone deficiency fed a high fat diet [Citation35–37] an effect that is abrogated following TTh [Citation36,Citation38]. The improvement in hepatic physiology as a result of TTh was accompanied by altered expression of important regulatory genes involved in hepatic lipid assembly and secretion [Citation36]. Moreover, TTh ameliorated hepatic steatosis and steatohepatitis by supressing endoplasmic reticulum stress, inhibiting macrovesicular lipid droplet formation and promoting very-low-density lipoprotein export in castrated male rats [Citation38].
Hypogonadism in men with hepatic steatosis is likely to be multifactorial. Patients with low testosterone levels often have comorbidities such as T2D, MetS and CVD which may contribute to hepatic steatosis and can ultimately perpetuate hypogonadism through the hypogonadal-obesity-adipocytokine axis [Citation39]. While experimental and clinical data suggest physiological testosterone, whether endogenous or administered, may prevent or ameliorate hepatic steatosis in males, few studies investigate the direct effects of long-term TTh on hepatic steatosis. The present long-term registry study investigates hepatic steatosis and parameters of liver function in hypogonadal men following 12-years of TTh to establish the role of testosterone in hepatic pathophysiology.
Methods
For this observational, prospective, cumulative registry study 505 elderly men (mean age: 61.4 ± 9.7 years) with symptoms of functional hypogonadism, where symptoms were defined as at least moderate symptoms on the Aging Males’ Symptoms scale, and a total testosterone (TT) ≤350 ng/dL (≤12.1 nmol/L) were identified for inclusion. Exclusion criteria included active prostrate or male breast cancer, indicated desire for paternity (especially in younger patients), allergy to ingredients in the testosterone formulation, acute heart disease within last 6 months or uncontrolled blood pressure problems. The study protocol conforms to the ethical guidelines of the 1975 Declaration of Helsinki as reflected in a priori approval by the German Ärztekammer (German medical association), and written informed consent was obtained from each patient included in the study and for receiving their treatment protocol. Three hundred and twenty one men received 1000 mg testosterone undecanoate (TU) parenterally every 12 weeks, following an initial 6-week interval, for up to 12 years (T-group). TTh was temporarily discontinued in 147 of these men after 5.5 years for 17 months (mean) due to reimbursement issues. TTh was re-established in these patients upon resolution of the issues. The 184 men who opted against TTh comprised the control group (C-group).
Total testosterone and SHGB were measured by enzyme-linked immunosorbent assay (Axym System, Abbott Germany. Threshold: 10–73 nmol/L). The effects of long-term TU on hepatic steatosis was assessed biannually by measuring fatty liver index (FLI), calculated based on waist circumference (WC), body mass index (BMI), triglyceride, and gamma-glutamyl-transferase (γ-GT) using the formula published by Bedogni G et al. [Citation40]. A FLI <30 rules out (negative likelihood ratio: 0. 2) and a FLI ≥60 indicates with a positive likelihood ratio of 4.3 that the patient has hepatic steatosis. Parameters of liver function, serum gamma-glutamyl transferase (γ-GT), aspartate transaminase (AST) and alanine transaminase (ALT) were assessed enzymatically on the Abbott Alinity c analytical system. Bilirubin was measured by Photometric Colour Test (Alinity C-Module Abbott).
Statistical analysis
The statistical analysis software Statistical Package for Social Sciences (SPSS) v.11 for Windows (SPSS Inc., Chicago, IL) was used for data processing and analysis. Data are expressed at each timepoint as mean group values with standard deviations. Comparison of clinical parameters between groups across the time points was done using a mixed-effects, repeated-measures model with period, group and their interaction as fixed effects. Analysis of variance (ANOVA) was used to compare continuous variables with significance accepted where p < 0.05.
Results
Patient baseline characteristics included in this registry are shown in . The mean baseline age for T-group consisting of 321 patients was 59 ± 9.5 years, with a mean follow-up of 8.3 ± 3.5 years. In the C-group (184 patients), the mean age was 66.1 ± 7.6 years, and mean follow-up of 5.5 ± 1.6 years. The FLI (p < 0.0001), bilirubin (p < 0.05), triglycerides (p < 0.0005), BMI (p < 0.0001) and WC (p < 0.0001) were significantly higher at baseline in the T-group compared to the C-group. For all subsequent comparisons over the study duration, data were only available for the T-group beyond 8-years.
Table 1. Baseline characteristics of Testosterone treatment group (T-group) and control group (C-group).
Men in the T-group had a significant elevation in TT levels following TTh at the 1-year follow-up (7.74 nmol/L to 16.11 nmol/L, p < 0.0001), which was sustained throughout the 8-year study period (15.98 nmol/L), compared to C-group patients (9.22 nmol/L at baseline to 9.24 nmol/L at 8-years) (). TT levels continued to increase beyond 8 years in the T-group with a mean concentration of 22.83 ± 2.34 nmol/L at 12 years (). SHBG was only measured in the T-group and declined from 36.68 ± 22.45 to 30.61 ± 13.85 at 8-years and then further to 29.91 ± 9.17 at 12 -years. Haematocrit increased in the T-group from 42.55 ± 3.94 to 45.96 ± 3.44 at 8-years and declined in the C-group from 45.01 ± 3.78 to 42.75 ± 1.52. Haemoglobin increased in the T-group from 14.14 ± 1.16 to 14.49 ± 0.98 and decreased in the C-group from 13.75 ± 1.16 to 13.61 ± 0.93.
Figure 1. Total testosterone (nmol/L) in 321 hypogonadal men on long-term treatment with testosterone undecanoate and 184 untreated hypogonadal controls. *p < 0.0001 between groups.
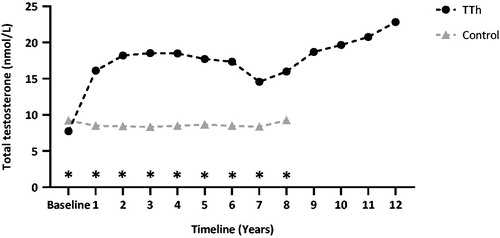
The FLI in the T-group decreased from 83.6 ± 12.08 at baseline to 66.91 ± 19.38 at 8-years (p < 0.001), compared to the C-group which increased from 68.67 ± 19.35 to 81.35 ± 16.91 (p < 0.001); with years 3–8 having a significantly lower FLI in the T-group compared to the C-group (p < 0.0001, ). The decline in the FLI continued in the T-group up to 12-years (59.13 ± 9.52).
Figure 2. The Fatty Liver Index (FLI) in 321 hypogonadal men on long-term treatment with testosterone undecanoate and 184 untreated hypogonadal controls. *p < 0.0001 between groups.
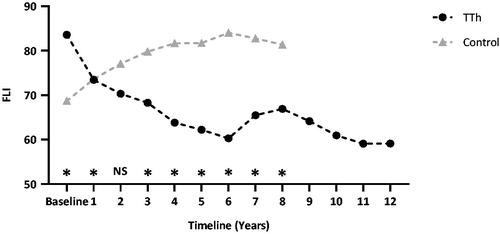
γ-GT significantly decreased in the T-group from 39.31 ± 11.62 at baseline to 28.95 ± 7.57U/L at 8-years (p < 0.0001). By 12 years γ-GT in the T-group had decreased further to 24.65 ± 3.67. Whereas it increased from 37.79 ± 29.55 at baseline to 39.5 ± 26.71U/L at 8-years in the C-group (p < 0.005; ) demonstrating a between group difference from year 1 to year 8 (p ≤ 0.005). A non-significant decline in bilirubin levels was observed in T-group patients from 1.64 ± 4.13 to 1.21 ± 1.89 mg/dL (p = 0.1716), C-group patients remained unchanged (1.04 ± 7.08 to 1.12 ± 1.96 mg/dL; p > 0.05, ). Although T-group had significantly elevated bilirubin levels at baseline (p < 0.05) by the 8 year point they were reduced to similar levels to those in C-group. At 12 years in the T-group, bilirubin was 0.9 ± 0.15 which was still not statistically significant vs. baseline. Additionally, AST levels remained unchanged versus baseline at 8 years for both groups and at 12 years for T-group (). Fluctuations occurred throughout with statistically significant increased at 2 (p < 0.0005), 3 (p < 0.0001), 4 (p < 0.0005), and 7 years (p < 0.01), then a significant decrease at 9 (p < 0.0001) and 10 years (p < 0.001) but at no other time point for the T-group, while AST significantly increased at 2 (p < 0.0005) and 4 years (p < 0.0005) for the C-group but not at any other point in time. Between group differences in AST were only demonstrable at 1 (p < 0.0001), 2 (p < 0.005) and 4 years (p < 0.005) with the T-group having lower levels, but these differences were not sustained. ALT levels declined slightly for both patient-groups but not significantly so at the end of the study period (). In the T-group, ALT was significantly increased versus baseline at 2 (p < 0.005), 3 (p < 0.0001), 4 (p < 0.0005), and 5 years (p < 0.05) and decreased at 8 (p < 0.05), 9 (p < 0.0001) and 10 years (p < 0.0005), while C-group had an increase statistically significant versus baseline only at 3 years (p < 0.05). Between group differences showed a T-group ALT level significantly lower than the C-group only at year 1 (p < 0.005).
Figure 3. γ-GT (A) and bilirubin levels (B) 321 hypogonadal men on long-term treatment with testosterone undecanoate and 184 untreated hypogonadal controls. Significance indicated between groups. *p < 0.0001.
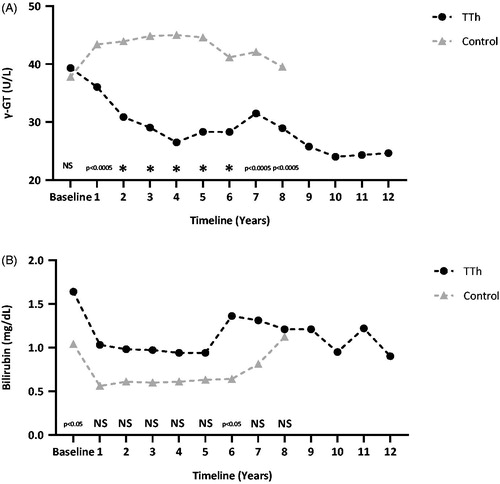
Figure 4. AST (A) and ALT (B) levels in 321 hypogonadal men on long-term treatment with testosterone undecanoate and 184 untreated hypogonadal controls. *p < 0.0001 between groups.
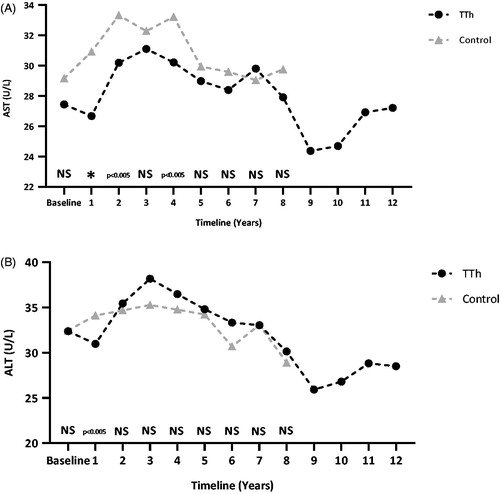
Triglycerides decreased from 252.35 ± 90.99 at baseline to 213 ± 65.91 mg/dL at 8-years in the T-group and increased from 196 ± 91.31 at baseline to 244.55 ± 61.39 mg/dL at 8-years in the C-group after 8 years; although a significant difference between the groups was reached after the first year and continued up to 8 years (p < 0.0001, ). Triglyceride levels continued to decline in the T-group up to 12-years (175.01 ± 37.31, p < 0.0001 vs. baseline).
Figure 5. Triglyceride levels in 321 hypogonadal men on long-term treatment with testosterone undecanoate and 184 untreated hypogonadal controls. *p < 0.0001 between groups.
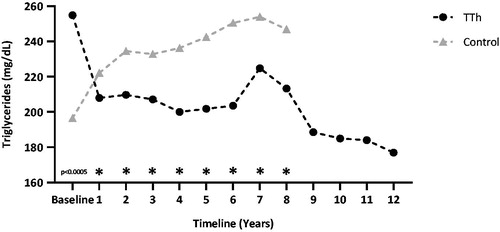
BMI decreased from 31.51 ± 4.32 at baseline to 29.03 ± 3.77 kg/m2 at 8-years in the T-group and increased from 29.2 ± 3.22 at baseline to 30.68 ± 3.99 kg/m2 at 8-years in C-group (p < 0.01, ). There was a significant difference of BMI between the groups after 3 years and this trend continued up to 8 years. Furthermore, BMI continued to decline in the T-group up to 12-years (27.57 ± 2.11, p < 0.0001 vs. baseline). WC was reduced in the T-group from 107.17 ± 9.64 at baseline to 100.34 ± 9.03 cm at 8-years and increased from 99.8 ± 9.13 at baseline to 104.65 ± 8.25 cm at 8-years in the C-group (p < 0.05, ). Similarly to BMI, WC continued to decrease significantly in the T-group compared to the C-group after 3 years of TTh. WC also continued to decline in the T-group up to 12-years (93.56 ± 3.05, p < 0.0001 vs. baseline).
Figure 6. BMI (A) and waist circumference (B) of 321 hypogonadal men on long-term treatment with testosterone undecanoate and 184 untreated hypogonadal controls. *p < 0.0001 between groups.
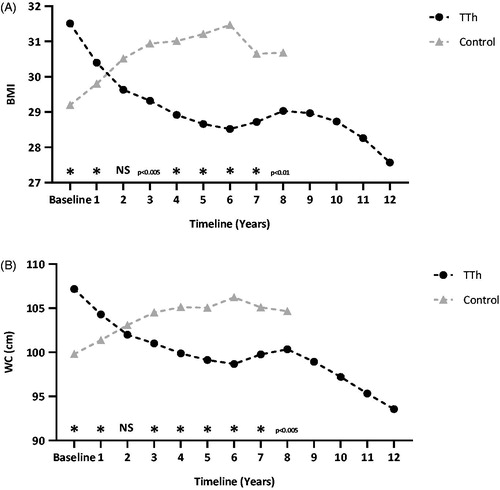
Twenty-seven deaths (14.7%) were recorded in the C-group, all of which were attributed to CVD including myocardial infarction (13, 48%), stroke (7, 27%), heart failure (3, 11%), aortic aneurysm (2, 7%) and lung embolism (2, 7%) (). In contrast, significantly less recorded deaths of 25 (7.8%, p = 0.0351) were noted in the T-group, of which a lower proportion of 11 deaths (44%, p = 0.001) were due to CVD made up of myocardial infarction (5, 20%), stroke (2, 8%), heart failure (2, 8%), aortic aneurysm (1, 4%) and lung embolism (1, 4%) ().
Table 2. Adverse events observed in testosterone treatment group (T-group) and control group (C-group).
Discussion
Low testosterone levels in men and the resulting hypogonadism correlates with components of MetS and T2D and associated cardiovascular risk [Citation3,Citation4,Citation41–43]. Furthermore, liver steatosis is indicative of metabolic dysfunction and may be considered the hepatic manifestation of MetS common in T2D and increasing CVD risk. Hepatic lipid deposition is independently associated with low testosterone levels in men [Citation31]. TTh is widely reported to improve CVD risk and components of MetS and T2D [Citation44–48], yet the effects of testosterone on hepatic steatosis are not fully understood. In this observational, prospective, registry study, we demonstrate for the first time the beneficial effects of long-term TTh in hypogonadal men on clinical measures of hepatic steatosis and liver function with an associated reduction in cardiovascular death.
The majority of studies investigating hypogonadism in the context of liver function have observed a link between low testosterone and NAFLD. In agreement with the present study, Barbonetti et al. [Citation31] reported an independent association between NAFLD and low testosterone levels in male patients with chronic spinal cord injury. Although, the patients included in the study had an increased prevalence of metabolic and lifestyle-related risk factors for NAFLD (such as reduced physical activity, higher BMI and increased insulin resistance and triglyceride levels), within this population it was demonstrated that patients with fatty liver, assessed by sonographic criteria, had reduced total (261.6 ± 159.5 ng/dL) and free testosterone levels (77.4 ± 51.7 pg/ml). The prevalence of NAFLD in men with low testosterone was 85%. Although the relationship was more pronounced in men exhibiting higher BMI and increased insulin resistance, triglycerides and γ-GT levels the association still remained after adjusting for these confounders. Only one, albeit large-scale, population-based cross-sectional study reports an association between low serum testosterone concentrations and hepatic steatosis in men [Citation29]. Two smaller studies report contrasting results in men with hepatic steatosis with one identifying low serum concentrations of testosterone in their patients and the other reporting no significant differences in testosterone levels compared to healthy men [Citation32,Citation33].
Interventional studies investigating TTh in hypogonadal patients have primarily focussed on sexual function restoration and improvements in metabolic parameters such as obesity, insulin resistance, glycaemic control and lean muscle mass rather than on parameters of hepatic steatosis and liver function. Improvements in metabolic parameters including insulin resistance and reduced liver fat are seen following 18 weeks of TTh in obese men with sleep apnea, although no effect on overall body weight was demonstrated [Citation34]. Conversely, results from two randomized controlled trials in elderly men with low testosterone levels reported no effect of 6 months of TTh on hepatic fat content as assessed by magnetic resonance imaging (MRI) or magnetic resonance spectroscopy (MRS) [Citation49]. Similarly, a randomized placebo-controlled trial of 44 hypogonadal men with T2D reported unchanged hepatic fat content assessed by MRI following 6 months of TTh [Citation50]. These previous studies were relatively short-term in treatment length and had smaller cohorts compared to the current study, where we report the rapid and continuous reduction in the FLI following TTh. This is the first study to suggest that long-term TTh reduces lipid accumulation in the liver and maintains improvements in liver function up to 12 years.
The effect of TTh on hepatic steatosis could also be mediated via improvements in the various components of MetS and T2D, as outlined previously [Citation39,Citation48]. The negative correlation of hepatic steatosis with MetS and T2D components such as obesity, insulin resistance and dyslipidemia, observed in previous studies further supports this hypothesis [Citation6,Citation51,Citation52]. Furthermore, the improvement in BMI and WC alongside the reduction in triglycerides in the T-group suggests metabolic parameters were improved compared to the C-group. TTh has been shown to reduce BMI and WC in hypogonadal men regardless of testosterone formulation and administration route [Citation53]. This collective continuous improvement of metabolic parameters following TTh highlights the beneficial effects of long-term TTh on body composition and triglycerides that may improve the degree of liver steatosis in hypogonadal men and contribute to cardiometabolic health.
Compelling evidence of a direct impact of testosterone upon liver physiology has been obtained from animal models. A greater degree of hepatic steatosis and inflammation was observed in hepatic androgen receptor (AR) knock-out mice [Citation54], 5α-reductase type 1 knock-out mice [Citation55] and testicular feminized (Tfm) mice which have non-functional AR and low circulating levels of testosterone [Citation36]. Similarly, severe androgen deficiency induced by surgical orchiectomy in rodents is associated with the development of hepatic steatosis in male mice and rats fed a high-fat diet, and TTh can significantly reduce the degree of hepatic lipid deposition in these testosterone deficient animals [Citation36,Citation37]. Mechanistically, TTh has been implicated in reducing expression of Acaca, Fasn and Srebf1 genes involved in hepatic lipid assembly and secretion [Citation35,Citation36,Citation56], which may therefore contribute to ectopic lipid deposition. It has also been hypothesized that androgens could modulate liver fatty acid β-oxidation and de novo lipid synthesis via regulation of SREBF-1c, a key regulator of fatty acid synthesis, and its primary target gene SCD1 that catalyses the rate-limiting step in the synthesis monounsaturated fatty acids [Citation54]. In further support of this hypothesis, dihydrotestosterone treatment in orchidectomized rats was associated with decreased lipid accumulation, potentially by decreasing fatty acid and cholesterol synthesis and increasing β-oxidation [Citation57]. Nevertheless, mechanistic findings have only arisen from animal studies, and their validity to the relationship between low testosterone levels and hepatic steatosis in humans remains to be established from studies assessing biopsy samples in TTh clinical trials.
Improved liver function in the T-group as evidenced by reduced γ-GT and bilirubin levels, suggests improvement of metabolic parameters. γ-GT is a glutathione catalase protein, the major thiol antioxidant. Elevated serum γ-GT is a marker of oxidative stress and is associated with the presence of hepatic steatosis [Citation58]. Elevated serum γ-GT correlates with the presence of CVD and has been identified in atheromatous plaques where it plays a role in LDL oxidation, platelet aggregation, apoptosis and influences plaque rupture [Citation59,Citation60]. Large epidemiological studies suggest an association between elevated γ-GT activity with CVD and CVD-related mortality [Citation60–62]. Furthermore, a study investigating the effects of TTh on 225 hypogonadal men with MetS found that AST and ALT levels decline over 5 years [Citation63]. The current study observed moderately increased levels of ALT, exceeding those of AST at baseline in both groups, the typically observed biochemical pattern in hepatic steatosis. However, we report no significant change following TTh, and levels of AST and ALT were within the normal range (8–48 and 7–55 U/L, respectively) throughout the study for both groups. Studies have reported that an elevated AST/ALT ratio is significantly associated with increased risk of developing CVD in men [Citation64,Citation65]; however another study did not observe this association [Citation66] and indicate that γ-GT is a superior liver function biomarker associated with CVD [Citation60]. Parameters of liver function and steatosis are influenced by alcohol consumption, and while there was no indication of changes in alcohol intake of patients between the groups, the present study did not specifically assess alcohol consumption and should therefore be considered a potential confounding factor and limitation of the observations.
Finally, the reduction in CVD related deaths in the T-group agrees with previous reports of improved cardiovascular health and reduced mortality in hypogonadal men that receive TTh [Citation67,Citation68]. It is not certain whether the reduction in CVD related deaths was due to the reduction in hepatic steatosis and improved function. Indeed, patients with NAFLD possess a high risk of developing CVD with shared pathogenic aetiology, and improvement in hepatic steatosis concomitantly decreases cardiovascular risk [Citation16]. Furthermore, long-term TTh has been shown to have favourable effects on multiple organ systems [Citation69]. The improvement in body composition and metabolic parameters in the present study did correspond with improved hepatic steatosis, feasibly reducing number of deaths attributed to CVD in the T-group. Although no significant differences in age were reported between the groups, death rates were not corrected for age and therefore we acknowledge that mortality data should be interpreted with caution.
This observational study is not without inherent limitations. As the current study is not a randomised controlled trial with a placebo arm, it does not allow direct comparison of testosterone versus non-treatment in matched patients, limiting the scope of interpretation. The primary focus of the study was to assess the long-term effectiveness and safety of TU injections in comparison to a voluntarily untreated hypogonadal control group rather than liver steatosis and function. At baseline, several parameters relating to liver function (FLI, bilirubin, triglycerides, BMI and WC) were significantly higher in the T-group compared to the C-group which may indicate a selection bias where patients undergoing treatment have the most pre-existing disease. However, it is notable that men in the T-group were not healthier than the controls as this would represent a bias in terms of cardiovascular events and mortality. This study does present clinically meaningful data of a large patient cohort with long-term follow-up-period of up to 12-years and demonstrates effects that justify further investigation and prospective randomised placebo controlled studies to further delineate the relationship between testosterone and hepatic steatosis. As indicated in the methods, several patients discontinued TTh for a temporary period during the course of follow-up due to reimbursement issues. This interruption in treatment may explain some of the trend reversals demonstrated in treated patients for some parameters, particularly between 6 and 8 years. This is also demonstrable in the total testosterone levels achieved in the treatment group, although the group mean remained in the physiological range. This additionally may have reduced the inter-group differences in liver function and associated parameters. Indeed, we have previously shown that TTh withdrawal results in a loss of beneficial effects on several cardiometabolic risk factors in hypogonadal men with treatment reinstatement restoring the positive effects [Citation70,Citation71]. Finally, the current study used measures of total testosterone to indicate androgen status. While SHBG was measured in patients receiving TTh it was not analysed in control patients due to financial reasons and this not being an randomised clinical trial, therefore comparative measures of free and bioavailable testosterone were not assessed. This allows SHBG as a potential confounder in both testosterone action and as a metabolic factor that may influence study outcomes.
In conclusion, this study indicates that long-term TTh does not worsen liver function, but on the contrary is associated with a possible improvement and highlights the potential beneficial effects of long-term TTh on liver steatosis in hypogonadal men. Consequently, as hepatic steatosis is a cardiometabolic risk factor, this study also suggests this improvement may reduce CVD-related mortality in these patients. Large randomized and placebo-controlled trials are necessary to elucidate the impact of TTh on hepatic function and steatosis in association with cardiovascular risk in hypogonadal men.
Acknowledgements
Open Access funding provided by the Qatar National Library. Editorial support for this manuscript was provided by Astra-Health, www.astra-health.co.uk
Disclosure statement
No financial support has been made to research or to prepare this article. A.Y. has received partial compensation for data entry and occasional honoraria from Bayer AG, Ferring Pharmaceuticals and GSK. F.S. is a consultant to Bayer AG. D.M.K. has received research grants and speaker honoraria from Bayer AG and funding to attend conferences from Bayer AG and Novo Nordisk. No other conflicts of interest to report.
References
- Angulo P. Nonalcoholic fatty liver disease. N Engl J Med. 2002;346(16):1221–1231.
- Mohr BA, Bhasin S, Link CL, et al. The effect of changes in adiposity on testosterone levels in older men: longitudinal results from the Massachusetts Male Aging Study. Eur J Endocrinol. 2006;155(3):443–452.
- Derby CA, Zilber S, Brambilla D, et al. Body mass index, waist circumference and waist to hip ratio and change in sex steroid hormones: the Massachusetts Male Ageing Study. Clin Endocrinol (Oxf). 2006;65(1):125–131.
- Kawano H. The relationship between testosterone and metabolic syndrome. Hypertens Res. 2010;33(6):537–538.
- Roden M. Mechanisms of Disease: hepatic steatosis in type 2 diabetes-pathogenesis and clinical relevance. Nat Clin Pract Endocrinol Metab. 2006;2(6):335–348.
- Medina J, Fernández-Salazar LI, García-Buey L, et al. Approach to the pathogenesis and treatment of nonalcoholic steatohepatitis. Diabetes Care. 2004;27(8):2057–2066.
- Bedogni G, Miglioli L, Masutti F, et al. Incidence and natural course of fatty liver in the general population: the Dionysos study. Hepatology. 2007;46(5):1387–1391.
- Ballestri S, Zona S, Targher G, et al. Nonalcoholic fatty liver disease is associated with an almost twofold increased risk of incident type 2 diabetes and metabolic syndrome. Evidence from a systematic review and meta-analysis. J Gastroenterol Hepatol. 2016;31(5):936–944.
- Farrell GC, Larter CZ. Nonalcoholic fatty liver disease: from steatosis to cirrhosis. Hepatology. 2006;43(2 Suppl 1):S99–S112.
- Bellentani S, Scaglioni F, Marino M, et al. Epidemiology of non-alcoholic fatty liver disease. Dig Dis. 2010;28(1):155–161.
- Lazo M, Clark JM. The epidemiology of nonalcoholic fatty liver disease: a global perspective. Semin Liver Dis. 2008;28(4):339–350.
- Targher G, Bertolini L, Padovani R, et al. Increased prevalence of cardiovascular disease in type 2 diabetic patients with non-alcoholic fatty liver disease. Diabet Med. 2006;23(4):403–409.
- Targher G, Bertolini L, Poli F, et al. Nonalcoholic fatty liver disease and risk of future cardiovascular events among type 2 diabetic patients. Diabetes. 2005;54(12):3541–3546.
- Targher G, Bertolini L, Rodella S, et al. Nonalcoholic fatty liver disease is independently associated with an increased incidence of cardiovascular events in type 2 diabetic patients. Diabetes Care. 2007;30(8):2119–2121.
- Targher G, Day CP, Bonora E. Risk of cardiovascular disease in patients with nonalcoholic fatty liver disease. N Engl J Med. 2010;363(14):1341–1350.
- Wójcik-Cichy K, Koślińska-Berkan E, Piekarska A. The influence of NAFLD on the risk of atherosclerosis and cardiovascular diseases. Clin Exp Hepatol. 2018;4(1):1–6.
- Almehmadi Y, Yassin AA, Nettleship JE, et al. Testosterone replacement therapy improves the health-related quality of life of men diagnosed with late-onset hypogonadism. Arab J Urol. 2016;14(1):31–36.
- Jones TH. Testosterone deficiency: a risk factor for cardiovascular disease? Trends Endocrinol Metab. 2010;21(8):496–503.
- Jones TH. Effects of testosterone on Type 2 diabetes and components of the metabolic syndrome. J Diabetes. 2010;2(3):146–156.
- Traish AM, Guay A, Feeley R, et al. The dark side of testosterone deficiency: I. Metabolic syndrome and erectile dysfunction. J Androl. 2009;30(1):10–22.
- Daka B, Langer RD, Larsson CA, et al. Low concentrations of serum testosterone predict acute myocardial infarction in men with type 2 diabetes mellitus. BMC Endocr Disord. 2015;15:35–35.
- Hackett G. Type 2 diabetes and testosterone therapy. World J Mens Health. 2019;37(1):31–44.
- Boyanov MA, Boneva Z, Christov VG. Testosterone supplementation in men with type 2 diabetes, visceral obesity and partial androgen deficiency. The Aging Male. 2003;6(1):1–7.
- Kapoor D, Goodwin E, Channer KS, et al. Testosterone replacement therapy improves insulin resistance, glycaemic control, visceral adiposity and hypercholesterolaemia in hypogonadal men with type 2 diabetes. Eur J Endocrinol. 2006;154(6):899–906.
- Heufelder AE, Saad F, Bunck MC, et al. Fifty-two-week treatment with diet and exercise plus transdermal testosterone reverses the metabolic syndrome and improves glycemic control in men with newly diagnosed type 2 diabetes and subnormal plasma testosterone. J Androl. 2009;30(6):726–733.
- Cornoldi A, Caminiti G, Marazzi G, et al. Effects of chronic testosterone administration on myocardial ischemia, lipid metabolism and insulin resistance in elderly male diabetic patients with coronary artery disease. Int J Cardiol. 2010;142(1):50–55.
- Kalinchenko SY, Tishova YA, Mskhalaya GJ, et al. Effects of testosterone supplementation on markers of the metabolic syndrome and inflammation in hypogonadal men with the metabolic syndrome: the double-blinded placebo-controlled Moscow study. Clin Endocrinol. 2010;73(5):602–612.
- Jones TH, Arver S, Behre HM; TIMES2 Investigators, et al. Testosterone replacement in hypogonadal men with type 2 diabetes and/or metabolic syndrome (the TIMES2 study). Diabetes Care. 2011;34(4):828–837.
- Völzke H, Aumann N, Krebs A, et al. Hepatic steatosis is associated with low serum testosterone and high serum DHEAS levels in men. Int J Androl. 2010;33(1):45–53.
- Kim S, Kwon H, Park JH, et al. A low level of serum total testosterone is independently associated with nonalcoholic fatty liver disease. BMC Gastroenterol. 2012;12:69.
- Barbonetti A, Caterina Vassallo MR, Cotugno M, et al. Low testosterone and non-alcoholic fatty liver disease: evidence for their independent association in men with chronic spinal cord injury. J Spinal Cord Med. 2016;39(4):443–449.
- Kley HK, Nieschlag E, Wiegelmann W, et al. Steroid hormones and their binding in plasma of male patients with fatty liver, chronic hepatitis and liver cirrhosis. Acta Endocrinol (Copenh). 1975;79(2):275–285.
- Myking O, Aakvaag A, Digranes Ø. Androgen-oestrogen imbalance in men with chronic alcoholism and fatty liver. Alcohol Alcohol. 1987;22(1):7–15.
- Hoyos CM, Yee BJ, Phillips CL, et al. Body compositional and cardiometabolic effects of testosterone therapy in obese men with severe obstructive sleep apnoea: a randomised placebo-controlled trial. Eur J Endocrinol. 2012;167(4):531–541.
- Senmaru T, Fukui M, Okada H, et al. Testosterone deficiency induces markedly decreased serum triglycerides, increased small dense LDL, and hepatic steatosis mediated by dysregulation of lipid assembly and secretion in mice fed a high-fat diet. Metabolism. 2013;62(6):851–860.
- Kelly DM, Nettleship JE, Akhtar S, et al. Testosterone suppresses the expression of regulatory enzymes of fatty acid synthesis and protects against hepatic steatosis in cholesterol-fed androgen deficient mice. Life Sci. 2014;109(2):95–103.
- Nikolaenko L, Jia Y, Wang C, et al. Testosterone replacement ameliorates nonalcoholic fatty liver disease in castrated male rats. Endocrinology. 2014;155(2):417–428.
- Jia Y, Yee JK, Wang C, et al. Testosterone protects high-fat/low-carbohydrate diet-induced nonalcoholic fatty liver disease in castrated male rats mainly via modulating endoplasmic reticulum stress. Am J Physiol Endocrinol Metab. 2018;314(4):E366–E376.
- Kelly DM, Jones TH. Testosterone: a metabolic hormone in health and disease. J Endocrinol. 2013;217(3):R25–45.
- Bedogni G, Bellentani S, Miglioli L, et al. The Fatty Liver Index: a simple and accurate predictor of hepatic steatosis in the general population. BMC Gastroenterol. 2006;6(1):33.
- Boden WE, Miller MG, McBride R, et al. Testosterone concentrations and risk of cardiovascular events in androgen-deficient men with atherosclerotic cardiovascular disease. Am Heart J. 2020;224:65–76.
- Wang C, Jackson G, Jones TH, et al. Low testosterone associated with obesity and the metabolic syndrome contributes to sexual dysfunction and cardiovascular disease risk in men with type 2 diabetes. Diabetes Care. 2011;34(7):1669–1675.
- Morgunov LY, Denisova IA, Rozhkova TI, et al. Hypogonadism and its treatment following ischaemic stroke in men with type 2 diabetes mellitus. Aging Male. 2020;23(1):71–80.
- Groti K, Žuran I, Antonič B, et al. The impact of testosterone replacement therapy on glycemic control, vascular function, and components of the metabolic syndrome in obese hypogonadal men with type 2 diabetes. The Aging Male. 2018;21(3):158–169.
- Salman M, Yassin DJ, Shoukfeh H, et al. Early weight loss predicts the reduction of obesity in men with erectile dysfunction and hypogonadism undergoing long-term testosterone replacement therapy. The Aging Male. 2017;20(1):45–48.
- Canguven O, Talib RA, El Ansari W, et al. Testosterone therapy has positive effects on anthropometric measures, metabolic syndrome components (obesity, lipid profile, Diabetes Mellitus control), blood indices, liver enzymes, and prostate health indicators in elderly hypogonadal men. Andrologia. 2017;49(10):e12768.
- Saad F, Yassin A, Doros G, et al. Effects of long-term treatment with testosterone on weight and waist size in 411 hypogonadal men with obesity classes I-III: observational data from two registry studies. Int J Obes (Lond). 2016;40(1):162–170.
- Yassin AA, Nettleship J, Almehmadi Y, et al. Effects of continuous long-term testosterone therapy (TTh) on anthropometric, endocrine and metabolic parameters for up to 10 years in 115 hypogonadal elderly men: real-life experience from an observational registry study. Andrologia. 2016;48(7):793–799.
- Magnussen LV, Andersen PE, Diaz A, et al. MR spectroscopy of hepatic fat and adiponectin and leptin levels during testosterone therapy in type 2 diabetes: a randomized, double-blinded, placebo-controlled trial. Eur J Endocrinol. 2017;177(2):157–168.
- Dhindsa S, Ghanim H, Batra M, et al. Insulin resistance and inflammation in hypogonadotropic hypogonadism and their reduction after testosterone replacement in men with type 2 diabetes. Dia Care. 2016;39(1):82–91.
- Hamaguchi M, Kojima T, Itoh Y, et al. The severity of ultrasonographic findings in nonalcoholic fatty liver disease reflects the metabolic syndrome and visceral fat accumulation. Am J Gastroenterol. 2007;102(12):2708–2715.
- Kotronen A, Yki-Järvinen H. Fatty liver: a novel component of the metabolic syndrome. Arterioscler Thromb Vasc Biol. 2008;28(1):27–38.
- Kelly DM, Jones TH. Testosterone and obesity. Obes Rev. 2015;16(7):581–606.
- Lin HY, Yu IC, Wang RS, et al. Increased hepatic steatosis and insulin resistance in mice lacking hepatic androgen receptor. Hepatology. 2008;47(6):1924–1935.
- Dowman JK, Hopkins LJ, Reynolds GM, et al. Loss of 5α-reductase type 1 accelerates the development of hepatic steatosis but protects against hepatocellular carcinoma in male mice. Endocrinology. 2013;154(12):4536–4547.
- Kelly DM, Akhtar S, Sellers DJ, et al. Testosterone differentially regulates targets of lipid and glucose metabolism in liver, muscle and adipose tissues of the testicular feminised mouse. Endocrine. 2016;54(2):504–515.
- Zhang H, Liu Y, Wang L, et al. Differential effects of estrogen/androgen on the prevention of nonalcoholic fatty liver disease in the male rat. J Lipid Res. 2013;54(2):345–357.
- Westerbacka J, Cornér A, Tiikkainen M, et al. Women and men have similar amounts of liver and intra-abdominal fat, despite more subcutaneous fat in women: implications for sex differences in markers of cardiovascular risk. Diabetologia. 2004;47(8):1360–1369.
- Emdin M, Pompella A, Paolicchi A. Gamma-glutamyltransferase, atherosclerosis, and cardiovascular disease: triggering oxidative stress within the plaque. Circulation. 2005;112(14):2078–2080.
- Mason JE, Starke RD, Van Kirk JE. Gamma-glutamyl transferase: a novel cardiovascular risk biomarker. Prev Cardiol. 2010;13(1):36–41.
- Ndrepepa G, Kastrati A. Gamma-glutamyl transferase and cardiovascular disease. Ann Transl Med. 2016;4(24):481–481.
- Lee DS, Evans JC, Robins SJ, et al. Gamma glutamyl transferase and metabolic syndrome, cardiovascular disease, and mortality risk: the Framingham Heart Study. ATVB. 2007;27(1):127–133.
- Traish AM, Haider A, Doros G, et al. Long-term testosterone therapy in hypogonadal men ameliorates elements of the metabolic syndrome: an observational, long-term registry study. Int J Clin Pract. 2014;68(3):314–329.
- Weng SF, Kai J, Guha IN, et al. The value of aspartate aminotransferase and alanine aminotransferase in cardiovascular disease risk assessment. Open Heart. 2015;2(1):e000272
- Yokoyama M, Watanabe T, Otaki Y, et al. Association of the aspartate aminotransferase to alanine aminotransferase ratio with BNP level and cardiovascular mortality in the general population: the yamagata study 10-year follow-up. Dis Markers. 2016;2016:4857917.
- Choi KM, Han K, Park S, et al. Implication of liver enzymes on incident cardiovascular diseases and mortality: a nationwide population-based cohort study. Sci Rep. 2018;8(1):3764–3764.
- Wallis CJ, Lo K, Lee Y, et al. Survival and cardiovascular events in men treated with testosterone replacement therapy: an intention-to-treat observational cohort study. Lancet Diabetes & Endocrinol. 2016;4(6):498–506.
- Jones TH, Saad F. The effects of testosterone on risk factors for, and the mediators of, the atherosclerotic process. Atherosclerosis. 2009;207(2):318–327.
- Yassin A, Almehmadi Y, Alwani M, et al. Long-term testosterone therapy improves renal function in men with hypogonadism: a real-life prospective controlled registry. J Clin Nephrol Res. 2020;7(1):1095.
- Saad F, Yassin A, Almehmadi Y, et al. Effects of long-term testosterone replacement therapy, with a temporary intermission, on glycemic control of nine hypogonadal men with type 1 diabetes mellitus - a series of case reports. Aging Male. 2015;18(3):164–168.
- Yassin A, Nettleship JE, Talib RA, et al. Effects of testosterone replacement therapy withdrawal and re-treatment in hypogonadal elderly men upon obesity, voiding function and prostate safety parameters. Aging Male. 2016;19(1):64–69.