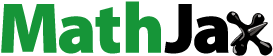
Abstract
Background
Janus kinase-2 (JAK2) inhibitors are now being tried in basic research and clinical practice in prostate cancer (PCa). However, the causal relationship between JAK2 and PCa has not been uniformly described. Here, we examined the cause-effect relation between JAK2 and PCa.
Methods
Two-sample Mendelian randomization (MR) analysis of genetic variation data of JAK2, PCa from IEU OpenGWAS Project was performed by inverse variance weighted, MR-Egger, and weighted median. Cochran’s Q heterogeneity test and MR-Egger multiplicity analysis were performed to normalize the MR analysis results to reduce the effect of bias on the results.
Results
Five instrumental variables were identified for further MR analysis. Specifically, combining the inverse variance-weighted (OR: 1.0009, 95% CI: 1.0001–1.0015, p = 0.02) and weighted median (OR: 1.0009, 95% CI: 1.0000–1.0017, p = 0.03). Sensitivity analysis showed that there was no heterogeneity (p = 0.448) and horizontal multiplicity (p = 0.770) among the instrumental variables.
Conclusions
We found JAK2 was associated with the development of PCa and was a risk factor for PCa, which might be instructive for the use of JAK2 inhibitors in PCa patients.
Introduction
Prostate cancer (PCa) is the next most common type of male cancer, affecting millions of men worldwide, mainly in high human development index (HDI) regions [Citation1,Citation2]. It is estimated that there were approximately 1,276,000 cases of PCa and 359,000 deaths from PCa worldwide in 2018 [Citation3]. The growth of PCa cells depends on androgen, so androgen deprivation therapy (ADT) is an effective treatment strategy widely used in clinical practice [Citation4]. The use of some drugs, immunotherapy, and surgical treatment also plays an important role in the treatment of PCa [Citation5–7].
Janus kinase-2 (JAK2) is a non-receptor protein, tyrosine kinase, a family member of Janus kinase (JAKs), which comprises JAK1-3 and TYK2 [Citation8]. While JAK1/2 signaling is implicated in the etiology of several cancers, JAK1/3 signaling is involved in the pathogenesis of inflammatory disorders [Citation9]. It is reported that JAK2 was regulated by highly expressed miR-375 and was selected as a hub gene in the protein interaction network in PCa [Citation10]. JAK2 was reported to be the significant tyrosine kinase of signal transducer and transcriptional activator 5 (STAT5), associated with high histological grade and high Gleason score in human PCa [Citation11]. Meanwhile, suppression of JAK2 expression is effective in PCa intervention studies [Citation12,Citation13].
In theories probing cause and disease, whether based on experimental studies of cellular lineages or population-based cohort epidemiological trials, the observed associations are not accurate causal inferences. Chance associations (random errors), spurious associations (selection, measurement bias), and indirect associations (confounding bias) may all disguise the truthful search for cause and effect. Mendelian randomization (MR) is a new method, which simulates randomized controlled trials (RCTs) at the genetic level by taking genetic variation as a tool variable [Citation14]. In contrast, MR jumps out of the dilemma of biological experiments or population cohort tests seeking to prove causality, based on the principle of random assignment of parental alleles at conception with the help of instrumental variables, cleverly avoiding environmental, conditional, bias, and ethical restrictions on truth-seeking. Moreover, compared with RCT, MR method has the advantages of saving money, time, and human resources, while avoiding the interference of confounding factors as much as possible [Citation15]. Currently, there is increasing research on single nucleotide polymorphism (SNP) in prostate diseases [Citation16,Citation17], which provided a foundation for MR analysis. Therefore, we mined publicly available databases to confirm the causal relationship between JAK2 and PCa utilizing an MR control analysis of two-sample MR control analysis.
Materials and methods
Acquisition of instrumental variables for exposure factors (JAK2) and outcome factors (PCa)
The human proteome is a key source of therapeutic targets, and genetic relation studies of the plasma proteome enable systematic examination of the causal effects of variation in plasma protein levels [Citation18]. A genome-wide test of 2,994 plasma protein levels in 3,301 individuals of European ancestry with 10.6 million attributed autosomal variants was performed, including plasma protein levels of JAK2. The GWAS data have adjusted for covariates such as age and gender [Citation19]. The PCa data were from the Neale lab analysis of UK Biobank phenotypes (http://www.nealelab.is/blog/2017/7/19/rapid-gwas-of-thousands-of-phenotypes-for-337000-samples-in-the-uk-biobank) and were included as an outcome factor. The research comprised 334,664 healthy controls and 2,495 PCa patients in total. The best situation in the MR analysis process is that the exposure and outcome samples do not overlap at all, but this is almost unavoidable in the usual analysis. The sample overlap rate can be used to determine the bias caused by weak instrumental variables, thereby balancing the bias elsewhere [Citation20]. In this study, we assumed that the samples completely overlap is the sample overlap rate.
Selection of instrumental variables for PCa associated with JAK2 levels
To obtain valid instrumental variables in the MR study, we imposed the following restrictions: (1) the selected IVs were closely related to exposure factors but not outcome factors (p < 5 × 10−8, r2 < 0.001, LD = 10,000 kb). (2) The selected IVs were not related to exposure factors or confounders of outcome factors (p > 1 × 10−5). (3) The IVs acted on the outcome only through exposure factors. PhenoScanner (http://www.phenoscanner.medschl.cam.ac.uk) was used for identification of confound factors (p value: 1 × 10−5, r2: 0.8, build: 37).
In MR research, instruments are frequently poorly associated with the exposure, which biases and inflates impact estimates of type I errors [Citation21]. Estimates from studies utilizing IVs are skewed in finite samples even when the essential underlying assumptions are valid [Citation22]. To prevent bias in the direction of the combined observation relationship between phenotype and outcome [Citation23], we calculated the strength-of-relationship statistic F between IVs and phenotype with the following equation:
where N represents the exposure database’s sample size. K is the number of SNPs, indicating the extent to which the instrumental variable explains the exposure correlated with the other parameters.
In the formula, MAF refers to the frequency of minor alleles, β is the size of the SNP effect on exposure, and SE denotes the standard deviation.
In general, SNPs with F greater than 10 are considered effective in avoiding bias due to weak instrumental variables, although higher thresholds are less likely to cause bias. In addition, to ensure that the above hypotheses were met, we tested whether the association information of the five SNPs was associated with potential risk factors for PCa through the PhenoScanner platform.
Sensitivity analysis
Sensitivity analysis has proven essential in MR research to find hidden pleiotropy and heterogeneity; otherwise, MR estimations may be seriously compromised [Citation2]. Since the sensitivity analysis has proven essential in MR research to find hidden pleiotropy and heterogeneity; otherwise, MR estimations may be seriously compromised under a weaker assumption [Citation24], it plays a vital role in testing heterogeneity and pleiotropy analysis. Within the framework of the InSIDE (Instrument Strength Independent of Direct Effect) hypothesis, the interception test of the MR-Egger analysis makes it possible to assess the validity of the hypothesis of the instrumental variables, with a non-zero intercept indicating bias in the inverse variance weighted (IVW) estimates and that IVW may act on the outcome through other phenotypic means [Citation24]. In addition, MR Egger and IVW method was applied in the heterogeneity test along with Cochran’s Q, which reacts to the variability between the instrumental variables. Afterward, the effect of missing each instrumental variable on the causality of the remaining instrumental variables was tested by excluding SNPs. The larger the difference before and after the exclusion, the higher the sensitivity of the test. The experimental procedure for this study is reflected in .
Statistics
Based on the different assumptions of horizontal pleiotropy, this study used IVW, MR-Egger, and weighted median for two-sample MR. The three methods complement each other to illustrate the joint investigation of the causal relationship of JAK2 on PCa. IVW was considered as the primary method for MR analysis because it applies a meta-analysis of Wald ratio estimates for all SNPs. Weighted median assumes no more than half of SNPs were invalid and it can provide relative causal estimates when heterogeneity exists. MR-Egger allowed intercepts exist and thus it applies to the case where pleiotropy exists. The above analyses were analyzed and visualized in R v.4.1.3, and the related R packages include “MRPRESSO”, “TwoSampleMR”, and its dependent extensions.
Results
Five SNPs (rs6809081, rs3775298, rs1801020, rs72840032, and rs704) loci affected the development of JAK2 levels in the organism. After calculation of instrumental variables and strength of phenotypic relationships, these SNPs with statistics F and effect allele frequencies were greater than 10 and 0.01, respectively: rs6809081 (sitting height, height, or pre-cancer cells cervix), rs3775298 (deep venous thrombosis, blood clot in the leg, pulmonary embolism, etc.), rs1801020 (factor XII antigen, activated partial thromboplastin time, interleukin 16 levels, etc.), rs72840032 (age-related macular degeneration), and rs704 (osteoprotegerin levels, age-related macular degeneration, height), whose diseases and traits shown by these IVs were not related to the mainstream considered risk factors for PCa (smoking, alcohol consumption, body mass index) [Citation2].
Five instrumental variables were preliminarily confirmed and IVW result showed a positive relationship between JAK2 and PCa (OR: 1.001, 95% CI: 1.000–1.002, p = 0.017). The weighted median method also supported this causal relationship (OR. 1.001, 95% CI: 1.000–1.002, p = 0.035) ( and ).
Figure 2. Forest plot of MR analysis of plasma protein JAK2 with PCa by IVW, weighted median, and MR Egger methods.

Figure 3. Scatter plot of MR analysis of plasma protein JAK2 with PCa by inverse variance weighted, weighted median, and MR Egger methods.
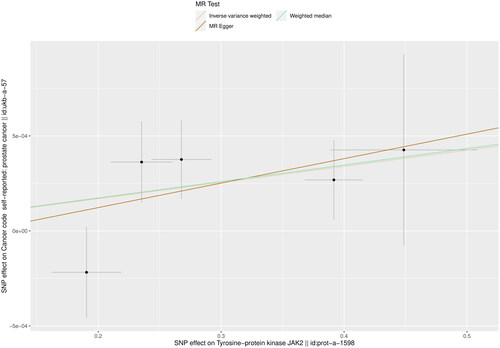
To verify that the selected IVs were not correlated with the outcome factors and followed the basic assumption of MR, the Egger-intercept operation suggested that the IVs met the three main assumptions with no horizontal multiplicity (p = 0.770). In addition, Cochran’s Q test (MR Egger Cochran’s Q, p = 0.311; inverse variance weighted Cochran’s Q p = 0.448) also confirmed the absence of concordance (). In addition, the visualization of the leave-out sensitivity analysis indicated that eliminating one SNP did not significantly affect the overall results ().
Discussion
Based on the GWAS database exploring the association between JAK2 and PCa, our findings suggested that JAK2 was a risk factor of PCa.
Lack of specific, sensitive targets often results in the excessive treatment of PCa, leading to the development of PCa [Citation25]. For example, a recent review indicates that there is no significant difference in the targets of some targeted therapies between the high and low scoring groups of PCa [Citation26]. Notably, as a critical link in numerous signaling, JAK2 and upstream and downstream signaling molecules are active in the molecular biology and pathology of PCa. For instance, CXCR7 caused castration-resistant prostate cancer (CRPC) progression through the JAK2/STAT1 signaling cascade [Citation27], C-kit increased PCa cell migration capacity and clonogenic properties through the JAK2/STAT1 pathway [Citation28], and androgen receptor (AR) and combined inhibition of JAK2/STAT1 affects DNA damage repair and apoptosis in PCa cells [Citation27]. In addition, high expression of Src homology region 2-containing protein tyrosine phosphatase 2 (SHP2) increases T cell activation and PD-1L expression in PCa cell lines [Citation29]. Blocking STAT1 phosphorylation with a JAK2 inhibitor (AG490) eliminates the influence of SHP2 on PD-L1 induction [Citation29], which is overexpressed on the surface of prostate tumor cells, mediates tumor immune escape and inhibits the immune response to exert a pro-cancer effect [Citation30]. JAK2 inhibitors can also regulate the proliferation of PCa cancer cells by regulating CDK4 kinase [Citation31,Citation32].
Also, as a signaling molecule downstream of JAK2, unlike STAT1 hypophosphorylation, STAT3 is constitutively activated in PCa cells [Citation29,Citation33]. Jak kinase activates the nuclear transcription factor STAT3, which also functions as a cytoplasmic signaling protein in response to the phosphorylation of specific tyrosine residues at the carboxyl terminus of cytokines [Citation34–36]. Similarly, JAK2/STAT3 assumes a top-down role in IL-6 signaling to PCa. IL-6/JAK2/STAT3 signaling increases WASF3 gene expression. It promotes PCa cell invasion and metastasis and inhibition of IL-6/JAK2/STAT3 signaling restores the effects on PCa cells in response to STING agonists, which facilitates cGAS-cGAMP-STING-mediated tumor rejection and immune infiltration, and IL-6/JAK2/STAT3 upregulates IGF-1Ec and PEc (Ec peptide) secretion for oncogenic and tumor repair effects [Citation37–39]. TGF-β1 decreases the transcription of JAK2, thus affecting how IL-6 activates STAT3 in prostatic hyperplasia cells [Citation40].
STAT3 controls the expression of mitochondrial apoptosis-related proteins in the nucleus, including Bcl-2, Mcl-1, and cIAP2, etc. Streptomycin B (Sch B) inhibits JAK2/STAT3/Bcl-2, promotes the collapse of mitochondrial membrane potential expression, induces oxidative stress, and leads to apoptosis in prostate tumor cells [Citation41]. On the contrary, the dissociation of BcL-2 can reduce cell apoptosis and increase prostate size [Citation42]. JAK1, JAK2, STAT3, and Src are all significantly activated by low-density lipoproteins in prostate cells [Citation43]. Benzo(a)pyrene (environmental carcinogen) enhances proliferation and mutagenesis activity via the JAK2-STAT3 pathway in PC-3 cells [Citation44]. In addition to the effects of JAK2/STAT3 signaling pathway inhibitors on PCa described above, some natural chemical drugs play essential roles in interfering with PCa and CRPC, typically cucurbitacin E [Citation45].
JAK2 and STAT3 also play essential roles in CRPC. Abnormal activation of JAK2/STAT3/MCL-1 and NF-κB is the leading cause of CRPC development [Citation46]. Inhibition of the JAK2-STAT3 signaling pathway can effectively reduce PD-L1 expression, reverse the inhibition of NKG2D, and activate NK cells against hypoxia-induced CRPC cells, providing a new idea for immunotherapy of CRPC [Citation47]. PD-L1 expression may be reduced if the JAK2/STAT3 pathway is used. It enhances the immune response and PD-L1 transduction inactivation within the tumor, facilitating anticancer activity [Citation48]. In conclusion, PCa frequently exhibits the requirement for active STAT3 for survival [Citation49]. JAK2/STAT3 signaling pathway plays a crucial role in tumorigenesis and malignant tumors, so it is a promising anti-cancer target [Citation48].
As another activating molecule downstream of JAK2, the activation of STAT5 in human PCa is mainly associated with high histological grade, and the current studies on JAK2/STAT5 are primarily focused on high-grade PCa, CRPC, and metastatic PCa [Citation11]. Under a normal physiological state, an AR regulates prostate development, maturation, and maintenance of functional operation [Citation50]. In PCa cells, androgen hyperactivation leads to the uncontrolled proliferation of tumor cells and the AR mediates the critical survival and growth of PCa [Citation51]. It is considered a key target for anti-cancer [Citation50]. Accordingly, ADT by inhibiting AR-driven PCa growth has been applied in the treatment of metastatic and high-risk PCa [Citation12]. As the disease progresses, despite castrating serum levels of androgens, however, genetic alterations, steroid metabolism, and signaling still contribute to the reactivation of AR, leading to lethal CRPC [Citation52]. The resistance of CRPC to second-generation anti-androgen therapy such as enzalutamide (ENZ) is developing rapidly [Citation12,Citation53]. The JAK2-Stat5a/b signaling pathway not only plays an essential role in fostering castrate-resistant PC development through both AR-dependent and AR-independent mechanisms, but also induces ENZ-resistant PC growth in preclinical PC models [Citation12,Citation13,Citation54]. Combined, the relationship between JAK2, Src, and STAT1/3/5 suggests that if the STAT family of signaling molecules is inhibited by the inhibition of Src, complete inhibition of its upstream genes may be required, and JAK2 is essential but not exclusive. According to our analysis above, there may be more than just JAK2 and Src. It is worth considering whether inhibition of JAK2 represents complete inhibition of STAT1/3/5 affecting PCa. It is also noteworthy that some studies of JAK2/STAT signaling molecules are primarily based on PCa cell lines. However, different cell lines have other effects on PCa, and the interventions.
We are the first to solve the causal relationship between JAK2 and PCa through MR research, effectively avoiding confusion, bias, and reverse causal relationship, and the cost is lower. The sample overlap rate of the study will not exceed 0.98%, indicating that if the weak IVs bias has little effect on the results. In addition, our findings may guide the use of JAK inhibitors in PCa. Our research still has some limitations. First of all, as PCa usually only occurs in males, GWAS data on exposure factors cannot only obtain JAK2 levels in males. However, the exposure data adjusted the gender during sequencing [Citation19], and we excluded the SNP located on the sex chromosome, so the gender interference can be almost ignored. Second, due to the limitation of data, we only studied the relationship between JAK2 and PCa in the JAK family. Third, our study only reveals the causal relationship between JAK2 and PCa in the European population, which needs to be further extended to other ethnic groups.
Conclusions
A two-sample MR analysis combined with existing studies confirmed a significant effect of JAK2 on PCa. Clinical development and practice of JAK2 inhibitors on PCa may require comprehensive consideration of the upstream and downstream signaling molecular relationships of JAK2, especially Src and PD-1/PD-1L signaling molecules.
Author contributions
Yujia Xi and Shengxiao Zhang designed the research ideas for this project. Yujia Xi and Ran Zhang wrote the main content of the full text. Rui Wen and Sijia Hou supplemented the group descriptions in the results and discussion sections. Qirui Dong summarized and collated the result data information, and Shengxiao Zhang proofread and reviewed the full-text information and provided constructive comments for reference.
Acknowledgements
Thanks to the authors and organizations who provided GWAS data of JAK2 and prostate cancer.
Disclosure statement
All authors declared no potential conflicts of interest.
Data availability statement
All data can be found in the IEU OpenGWAS Project database (https://gwas.mrcieu.ac.uk/).
Additional information
Funding
References
- Rebello RJ, Oing C, Knudsen KE, et al. Prostate cancer. Nat Rev Dis Primers. 2021;7(1):9. doi: 10.1038/s41572-020-00243-0.
- Chen X, Kong J, Diao X, et al. Depression, and prostate cancer risk: a Mendelian randomization study. Cancer Med. 2020;9(23):9160–9167. doi: 10.1002/cam4.3493.
- Culp MB, Soerjomataram I, Efstathiou JA, et al. Recent global patterns in prostate cancer incidence and mortality rates. Eur Urol. 2020;77(1):38–52. doi: 10.1016/j.eururo.2019.08.005.
- Guan X, Polesso F, Wang C, et al. Androgen receptor activity in T cells limits checkpoint blockade efficacy. Nature. 2022;606(7915):791–796. doi: 10.1038/s41586-022-04522-6.
- Teo MY, Rathkopf DE, Kantoff P. Treatment of advanced prostate cancer. Annu Rev Med. 2019;70(1):479–499. doi: 10.1146/annurev-med-051517-011947.
- Sebesta EM, Anderson CB. The surgical management of prostate cancer. Semin Oncol. 2017;44(5):347–357. doi: 10.1053/j.seminoncol.2018.01.003.
- Bilusic M, Madan RA, Gulley JL. Immunotherapy of prostate cancer: facts and hopes. Clin Cancer Res. 2017;23(22):6764–6770. doi: 10.1158/1078-0432.CCR-17-0019.
- Hubbard SR. Mechanistic insights into regulation of JAK2 tyrosine kinase. Front Endocrinol. 2017;8:361. doi: 10.3389/fendo.2017.00361.
- Roskoski RJ. Janus kinase (JAK) inhibitors in the treatment of inflammatory and neoplastic diseases. Pharmacol Res. 2016;111:784–803. doi: 10.1016/j.phrs.2016.07.038.
- He S, Shi J, Mao J, et al. The expression of miR-375 in prostate cancer: a study based on GEO, TCGA data and bioinformatics analysis. Pathol Res Pract. 2019;215(6):152375. doi: 10.1016/j.prp.2019.03.004.
- Li H, Ahonen TJ, Alanen K, et al. Activation of signal transducer and activator of transcription 5 in human prostate cancer is associated with high histological grade. Cancer Res. 2004;64(14):4774–4782. doi: 10.1158/0008-5472.CAN-03-3499.
- Beinhoff P, Sabharwal L, Udhane V, et al. Second-generation Jak2 inhibitors for advanced prostate cancer: are we ready for clinical development? Cancers. 2021;13(20):5204. doi: 10.3390/cancers13205204.
- Udhane V, Maranto C, Hoang DT, et al. Enzalutamide-induced feed-forward signaling loop promotes therapy-resistant prostate cancer growth providing an exploitable molecular target for Jak2 inhibitors. Mol Cancer Ther. 2020;19(1):231–246. doi: 10.1158/1535-7163.MCT-19-0508.
- Bowden J, Holmes MV. Meta-analysis and Mendelian randomization: a review. Res Synth Methods. 2019;10(4):486–496. doi: 10.1002/jrsm.1346.
- Birney E. Mendelian randomization. Cold Spring Harb Perspect Med. 2022;12(4):a041302. doi: 10.1101/cshperspect.a041302.
- Qian X, Xu D, Liu H, et al. Genetic variants in 5p13.2 and 7q21.1 are associated with treatment for benign prostatic hyperplasia with the alpha-adrenergic receptor antagonist. Aging Male. 2017;20(4):250–256. doi: 10.1080/13685538.2017.1358261.
- Spisak S, Tisza V, Nuzzo PV, et al. A biallelic multiple nucleotide length polymorphism explains functional causality at 5p15.33 prostate cancer risk locus. Nat Commun. 2023;14(1):5118. doi: 10.1038/s41467-023-40616-z.
- Zheng J, Haberland V, Baird D, et al. Phenome-wide Mendelian randomization mapping the influence of the plasma proteome on complex diseases. Nat Genet. 2020;52(10):1122–1131. doi: 10.1038/s41588-020-0682-6.
- Sun BB, Maranville JC, Peters JE, et al. Genomic atlas of the human plasma proteome. Nature. 2018;558(7708):73–79. doi: 10.1038/s41586-018-0175-2.
- Burgess S, Davies NM, Thompson SG. Bias due to participant overlap in two-sample Mendelian randomization. Genet Epidemiol. 2016;40(7):597–608. doi: 10.1002/gepi.21998.
- Wang S, Kang H. Weak-instrument robust tests in two-sample summary-data Mendelian randomization. Biometrics. 2022;78(4):1699–1713. doi: 10.1111/biom.13524.
- Burgess S, Thompson SG. Bias in causal estimates from Mendelian randomization studies with weak instruments. Stat Med. 2011;30(11):1312–1323. doi: 10.1002/sim.4197.
- Burgess S, Thompson SG, CRP CHD Genetics Collaboration. Avoiding bias from weak instruments in Mendelian randomization studies. Int J Epidemiol. 2011;40(3):755–764. doi: 10.1093/ije/dyr036.
- Burgess S, Thompson SG. Interpreting findings from Mendelian randomization using the MR-Egger method. Eur J Epidemiol. 2017;32(5):377–389. doi: 10.1007/s10654-017-0255-x.
- Kong P, Zhang L, Zhang Z, et al. Emerging proteins in CRPC: functional roles and clinical implications. Front Oncol. 2022;12:873876. doi: 10.3389/fonc.2022.873876.
- Bahcivan A, Gamsizkan M, Kantarcioglu CS, et al. KRAS, BRAF, PIK3CA mutation frequency of radical prostatectomy samples and review of the literature. Aging Male. 2020;23(5):1627–1641. doi: 10.1080/13685538.2021.1901274.
- Luo Y, Yang X, Basourakos SP, et al. Enzalutamide-resistant progression of castration-resistant prostate cancer is driven via the JAK2/STAT1-dependent pathway. Front Mol Biosci. 2021;8:652443. doi: 10.3389/fmolb.2021.652443.
- Guo JH, Zhou J, Zhao Y, et al. Normal peripheral prostate stromal cells stimulate prostate cancer development: roles of the c-kit signal. Am J Transl Res. 2015;7(3):502–512.
- Liu Z, Zhao Y, Fang J, et al. SHP2 negatively regulates HLA-ABC and PD-L1 expression via STAT1 phosphorylation in prostate cancer cells. Oncotarget. 2017;8(32):53518–53530. doi: 10.18632/oncotarget.18591.
- Sun Z, Yin S, Zhao C, et al. Inhibition of PD-L1-mediated tumor-promoting signaling is involved in the anti-cancer activity of beta-tocotrienol. Biochem Biophys Res Commun. 2022;617(Pt 2):33–40. doi: 10.1016/j.bbrc.2022.05.082.
- Li X, Yang T, Hu M, et al. Synthesis and biological evaluation of 6-(pyrimidin-4-yl)-1H-pyrazolo[4,3-b]pyridine derivatives as novel dual FLT3/CDK4 inhibitors. Bioorg Chem. 2022;121:105669. doi: 10.1016/j.bioorg.2022.105669.
- Fu X, Wang D, Shu T, et al. LncRNA NR2F2-AS1 positively regulates CDK4 to promote cancer cell proliferation in prostate carcinoma. Aging Male. 2020;23(5):1073–1079. doi: 10.1080/13685538.2019.1670157.
- Agarwal C, Tyagi A, Kaur M, et al. Silibinin inhibits constitutive activation of Stat3, and causes caspase activation and apoptotic death of human prostate carcinoma DU145 cells. Carcinogenesis. 2007;28(7):1463–1470. doi: 10.1093/carcin/bgm042.
- Levy DE, Darnell JJ. Stats: transcriptional control and biological impact. Nat Rev Mol Cell Biol. 2002;3(9):651–662. doi: 10.1038/nrm909.
- Bromberg J, Wang TC. Inflammation and cancer: IL-6 and STAT3 complete the link. Cancer Cell. 2009;15(2):79–80. doi: 10.1016/j.ccr.2009.01.009.
- Abdulghani J, Allen JE, Dicker DT, et al. Sorafenib sensitizes solid tumors to Apo2L/TRAIL and Apo2L/TRAIL receptor agonist antibodies by the Jak2-Stat3-Mcl1 axis. PLOS One. 2013;8(9):e75414. doi: 10.1371/journal.pone.0075414.
- Teng Y, Ghoshal P, Ngoka L, et al. Critical role of the WASF3 gene in JAK2/STAT3 regulation of cancer cell motility. Carcinogenesis. 2013;34(9):1994–1999. doi: 10.1093/carcin/bgt167.
- Suter MA, Tan NY, Thiam CH, et al. cGAS-STING cytosolic DNA sensing pathway is suppressed by JAK2-STAT3 in tumor cells. Sci Rep. 2021;11(1):7243. doi: 10.1038/s41598-021-86644-x.
- Armakolas A, Dimakakos A, Loukogiannaki C, et al. IL-6 is associated to IGF-1Ec upregulation and Ec peptide secretion, from prostate tumors. Mol Med. 2018;24(1):6. doi: 10.1186/s10020-018-0003-z.
- Starsichova A, Lincova E, Pernicova Z, et al. TGF-beta1 suppresses IL-6-induced STAT3 activation through regulation of Jak2 expression in prostate epithelial cells. Cell Signal. 2010;22(11):1734–1744. doi: 10.1016/j.cellsig.2010.06.014.
- Nasser MI, Han T, Adlat S, et al. Inhibitory effects of schisandrin B on human prostate cancer cells. Oncol Rep. 2019;41(1):677–685.
- Sreenivasulu K, Nandeesha H, Dorairajan LN, et al. Over expression of PI3K-AkT reduces apoptosis and increases prostate size in benign prostatic hyperplasia. Aging Male. 2020;23(5):440–446. doi: 10.1080/13685538.2018.1519014.
- Jung YY, Ko JH, Um JY, et al. LDL cholesterol promotes the proliferation of prostate and pancreatic cancer cells by activating the STAT3 pathway. J Cell Physiol. 2021;236(7):5253–5264. doi: 10.1002/jcp.30229.
- Gao M, Li H, Dang F, et al. Induction of proliferative and mutagenic activity by benzo(a)pyrene in PC-3 cells via JAK2/STAT3 pathway. Mutat Res. 2020;821:111720. doi: 10.1016/j.mrfmmm.2020.111720.
- Dong Y, Lu B, Zhang X, et al. Cucurbitacin E, a tetracyclic triterpenes compound from Chinese medicine, inhibits tumor angiogenesis through VEGFR2-mediated Jak2-STAT3 signaling pathway. Carcinogenesis. 2010;31(12):2097–2104. doi: 10.1093/carcin/bgq167.
- Bao X, Zhu J, Ren C, et al. Beta-elemonic acid inhibits growth and triggers apoptosis in human castration-resistant prostate cancer cells through the suppression of JAK2/STAT3/MCL-1 and NF-kB signal pathways. Chem Biol Interact. 2021;342:109477. doi: 10.1016/j.cbi.2021.109477.
- Xu LJ, Ma Q, Zhu J, et al. Combined inhibition of JAK1,2/Stat3PDL1 signaling pathway suppresses the immune escape of castration-resistant prostate cancer to NK cells in hypoxia. Mol Med Rep. 2018;17(6):8111–8120.
- Liu P, Zhao L, Xu X, et al. N6-substituted adenosine analogues, a novel class of JAK2 inhibitors, potently block STAT3 signaling in human cancer cells. Cancer Lett. 2014;354(1):43–57. doi: 10.1016/j.canlet.2014.07.043.
- Shodeinde AL, Barton BE. Potential use of STAT3 inhibitors in targeted prostate cancer therapy: future prospects. Onco Targets Ther. 2012;5:119–125.
- Green SM, Mostaghel EA, Nelson PS. Androgen action and metabolism in prostate cancer. Mol Cell Endocrinol. 2012;360(1–2):3–13. doi: 10.1016/j.mce.2011.09.046.
- Culig Z, Santer FR. Androgen receptor signaling in prostate cancer. Cancer Metastasis Rev. 2014;33(2–3):413–427. doi: 10.1007/s10555-013-9474-0.
- Shafi AA, Yen AE, Weigel NL. Androgen receptors in hormone-dependent and castration-resistant prostate cancer. Pharmacol Ther. 2013;140(3):223–238. doi: 10.1016/j.pharmthera.2013.07.003.
- Qian S, Xia J, Liu H, et al. Integrative transcriptome analysis identifies genes and pathways associated with enzalutamide resistance of prostate cancer. Aging Male. 2018;21(4):231–237. doi: 10.1080/13685538.2018.1424129.
- Hoang DT, Iczkowski KA, Kilari D, et al. Androgen receptor-dependent and -independent mechanisms driving prostate cancer progression: opportunities for therapeutic targeting from multiple angles. Oncotarget. 2017;8(2):3724–3745. doi: 10.18632/oncotarget.12554.