Abstract
Aims: Point-of-care electroencephalogram (POC-EEG) is an acute care bedside screening tool for the identification of nonconvulsive seizures (NCS) and nonconvulsive status epilepticus (NCSE). The objective of this narrative review is to describe the economic themes related to POC-EEG in the United States (US).
Materials and methods: We examined peer-reviewed, published manuscripts on the economic findings of POC-EEG for bedside use in US hospitals, which included those found through targeted searches on PubMed and Google Scholar. Conference abstracts, gray literature offerings, frank advertisements, white papers, and studies conducted outside the US were excluded.
Results: Twelve manuscripts were identified and reviewed; results were then grouped into four categories of economic evidence. First, POC-EEG usage was associated with clinical management amendments and antiseizure medication reductions. Second, POC-EEG was correlated with fewer unnecessary transfers to other facilities for monitoring and reduced hospital length of stay (LOS). Third, when identifying NCS or NCSE onsite, POC-EEG was associated with greater reimbursement in Medical Severity-Diagnosis Related Group coding. Fourth, POC-EEG may lower labor costs via decreasing after-hours requests to EEG technologists for conventional EEG (convEEG).
Limitations: We conducted a narrative review, not a systematic review. The studies were observational and utilized one rapid circumferential headband system, which limited generalizability of the findings and indicated publication bias. Some sample sizes were small and hospital characteristics may not represent all US hospitals. POC-EEG studies in pediatric populations were also lacking. Ultimately, further research is justified.
Conclusions: POC-EEG is a rapid screening tool for NCS and NCSE in critical care and emergency medicine with potential financial benefits through refining clinical management, reducing unnecessary patient transfers and hospital LOS, improving reimbursement, and mitigating burdens on healthcare staff and hospitals. Since POC-EEG has limitations (i.e. no video component and reduced montage), the studies asserted that it did not replace convEEG.
Introduction
From a clinical perspective, status epilepticus (SE) is a medical emergency comprised of prolonged seizure activity that can induce irreversible neuronal damage or deathCitation1–5. Estimates of the annual incidence of SE in the United States (US) are variable at 18Citation6 to 41Citation7 patients per 100,000 population. Median daily hospitalization costs for SE inpatient admissions in the US are approximated at $2,366 to $3,359, depending on SE refractorinessCitation8.
The American Clinical Neurophysiology SocietyCitation3 provides specific terminology defining SE in their guidelines. Electroclinical SE refers to SE involving both electroencephalogram (EEG) findings and clinical manifestations of seizures, which may include prominent motor activity leading to the classification of convulsive SE. Electrographic SE, in contrast, refers to SE that primarily involves EEG data without requiring clinical manifestations of seizuresCitation3. Nevertheless, since SE must be promptly addressedCitation1, a physician’s decision to treat a patient for SE may be empirical. This is particularly relevant for nonconvulsive seizures (NCS) and nonconvulsive SE (NCSE), as NCSE does not have the prominent motor activity characteristic of convulsive SECitation3,Citation9. Accordingly, clinical symptoms in patients with NCSE can be difficult to detect or even absent, obfuscating empirical treatment decisionsCitation2,Citation3,Citation10–12. Underlying causes of NCSE include brain injuryCitation13,Citation14, undifferentiated comatose statesCitation15, and systemic medical conditions, such as sepsisCitation16; therefore, physicians often require EEG to confirm NCSE in patients with impaired consciousness. Clinical acumen combined with EEG assessment is ultimately needed to corroborate an NCSE diagnosisCitation3,Citation10.
NCSE is a common medical condition in hospitalized patients, spurring clinical and financial burdens. In an observational cohort study across three years at a tertiary care center, nonconvulsive and subtle SE (with minimal clinical symptoms) accounted for 81% of SE episodesCitation17. Laccheo et al.Citation11 reported that the median length of stay (LOS) in the intensive care unit (ICU) was 3 days longer for neurological patients with than those without NCS or NCSE. The former group also had more than double the mortality rate of the latterCitation11. In the US, the median cost of SE-related admissions among adults is $15,595Citation18.
Conventional EEG for NCS and NCSE detection
Due to the challenges in the clinical identification of NCS and NCSE, together with the medicalCitation11,Citation12 and financial repercussionsCitation8,Citation18, accurate and objective means of facilitating NCSE diagnosis are imperative. Conventional 16-channel EEG (convEEG) using the International 10–20 electrode system remains the standard modality in detecting NCSE, as well as other forms of electroclinical and electrographic SE, and for informing treatment decisionsCitation3,Citation10,Citation19–21. The Neurocritical Care SocietyCitation2 states that continuous EEG initiation should occur within 1 h of clinical suspicion of SE, including NCSE. Based on these guidelinesCitation2, the American Clinical Neurophysiology SocietyCitation22 recommends that “critical care continuous EEG should be initiated as soon as possible when NCS are suspected, since prolonged NCS and NCSE are associated with higher morbidity and mortality and treatment is likely to be more effective earlier in the course.”
Despite the urgency intrinsic to detecting and treating NCS and NCSE, implementation of convEEG is resource-heavy and time-consuming; in academic hospitals, the median delay for convEEG is nearly 4 h longCitation23. The reasons are manifold. First, EEG technologists must operate convEEG, but staffing and EEG equipment availability can be expensive and scarce. Since it is unknown when or where SE will occur in a hospital, both EEG technologists and convEEG may already be occupied. This complicates triaging, for when EEG supply is needed to assess the severity of a patient’s condition, it may be unprocurable. Further, inpatient EEG requests may stack such that convEEG in use for active monitoring must be disconnected, packed, and transported to patients in other departments. When the EEG arrives at bedside, additional time is expended in setup and troubleshooting by the EEG technologist for proper signal acquisition. Finally, only once a neurologist or neurology subspecialist interprets the EEG data, can the study be resultedCitation24,Citation25.
Each of these issues compounds the time to diagnosis and delays proper care of NCSE. Standard business hoursCitation26 of EEG coverage from 8:00am–4:00pm or 9:00am–5:00pm, Monday through Friday in some hospitals only comprises about 24% of the entire week, yet again, seizure occurrence is not confined to weekday workday hours. The demand for inpatient EEG is unpredictable and on-call services may in turn be constrained. A NCSE patient could experience several seizures before receiving convEEG evaluation given lengthy delays, which exist even among academic hospitals with onsite EEG technologists during and outside business hoursCitation23–25,Citation27. Postponement of convEEG assessment and SE treatment to hours later, the next workday, or even longer may jeopardize patient healthCitation23,Citation27,Citation28. Although continuous EEG has been linked with more seizure detection and alterations to antiseizure treatment, some evidence has indicated that it may not benefit patient outcomes as compared with routine EEGCitation29. Hospitalization charges may also rise with the use of continuous EEGCitation30.
In the absence of timely EEG assessment, healthcare providers relying on incomplete information (i.e. clinical evaluation only) are at risk for overtreating in some cases and administering inadequate treatment in others. Delays in convEEG for a suspected actively-seizing patient may precipitate empirical treatment; thus, some patients may be erroneously treated for NCSE based on clinical manifestations aloneCitation25,Citation31. Benzodiazepines, which are first-line antiseizure medications (ASMs) to abort seizures, can have substantial side effectsCitation2 if prescribed to patients without NCSE or other seizures. Spatola et al.Citation32 found that excess benzodiazepine administration was associated with an increased use of orotracheal intubation, and subsequently, extended hospital LOS. In a clinical trial (ESETT) of rapid treatment of seizure without the benefit of EEG monitoring, researchers similarly noted that the lack of improvement in some patients may have been due to the sedative nature of ASMs prescribed to those who might not have been in SE. These patients therefore may have incorrectly received anti-epileptic treatmentCitation31.
Moreover, an analysis of data from ESETT and from another clinical trial on prehospital benzodiazepine administration (RAMPART)Citation33 revealed that about 8% of patients had prolonged psychogenic nonepileptic seizures, even though they had been treated for SE. Over 20% of these inappropriately treated patients required an ICU stay and almost 30% experienced adverse eventsCitation34. High rates of benzodiazepine administration to patients with dissociative (i.e. nonepileptic) seizures have been observed in a different study at a university hospital as wellCitation35. With the accuracy of diagnosing video recordings of epileptic versus psychogenic nonepileptic seizures ranging from about 44% to 58% among non-neurology healthcare staffCitation36, the importance of EEG monitoring to minimize overmedication of benzodiazepines is clear. Lastly, in addition to the detrimental impact on patient health, overtreatment in general drains resources, with approximately $75.7 to $101.2 billion wasted per year from overtreatment or low-value care in the overall US healthcare systemCitation37.
Without prompt confirmation of seizures via EEG, patients could conversely receive insufficient treatmentCitation25. Benzodiazepine underdosing is prevalent and may lower seizure recovery ratesCitation38,Citation39. ASMs are less effective when prescribed late into prolonged seizure and protracted SE is linked to worse health outcomesCitation28; early detection of SE is again vital. Delays may be particularly inimical for patients who are then prescribed suboptimal benzodiazepine dosesCitation38, though these findings on treatment dosing and timing may be less suited for electrographic seizures, focal SE, and NCSE. Moreover, inpatient hospital admission costs are inversely proportional to treatment success. Compared with the costs associated with non-refractory SE, those of refractory SE and super-refractory SE are two and five times greater, respectivelyCitation18. Like overtreatment, inadequate treatment of SE may have financial consequences as well.
Point-of-care EEG for NCS and NCSE detection
Over the past years, new high-quality EEG devices have been developed and introduced to hospitals for patients at risk for SE, especially NCSECitation21,Citation23,Citation24,Citation40,Citation41. The purpose of these technologies is to facilitate faster bedside detection without mandating technologist involvement. This narrative review defines such devices as point-of-care EEGs (POC-EEGs). With fewer electrodes than convEEG, POC-EEG serves as a screening test for NCS and NCSECitation21. The speed of POC-EEG encapsulates its value; its setup time has been reported as about 5 to 6 minCitation23,Citation42,Citation43, with an approximate time from order and time from arrival at the emergency department (ED) or ICU, respectively, to initiation of 23Citation43 and 96Citation41 minutes. The median duration of POC-EEG monitoring is about 2 hCitation44, which is distinct from that of routine EEG, with its recording time of around 0.3–0.5 hCitation45.
Non-neurologist physicians, nurses, and other allied health professionals can deploy POC-EEG, as its operation is independent of EEG technologists and only basic training is necessary for applicationCitation25,Citation42. Although POC-EEG application is non-specialized, neurologists or neurology subspecialists are still responsible for the interpretation of recordings. Since the incorporation of POC-EEG in various hospital settings, its impact on workflow and outcomes has been illustratedCitation44,Citation46,Citation47. POC-EEG, however, neither antiquates nor supplants convEEGCitation48–50, nor does it replace the role of EEG technologists or neurologists. ConvEEG underpins patient diagnosis and treatment strategyCitation10,Citation19,Citation20. The utility of POC-EEG is in time-sensitive ED and ICU cases, including ruling out NCSECitation48–50. Continuous EEG, when available, should ideally be acquired for patients with NCS or NCSE detected on POC-EEG to ensure ongoing evaluation and long-term treatment planning.
Since POC-EEG utilization has grown in the last few years among large tertiary and academic hospitals, as well as in community hospitals, device performance has been investigatedCitation48,Citation51,Citation52. Research on the economic impacts of POC-EEG, nonetheless, is relatively sparse. The aim of this narrative review is to summarize the literature on the economic themes related to POC-EEG use for the bedside detection of NCS and NCSE in US hospital patients.
Methods
Research related to economic themes for POC-EEG evaluation of hospital patients in the US was identified. The evidence base consisted of articles provided by the study funder and those found through targeted searches on PubMed and Google Scholar. The relevant search terms are listed in Table S1. As this was a narrative review, Preferred Reporting Items for Systematic Reviews and Meta-Analyses (PRISMA) or other formal systematic review protocols were not utilized.
Studies were restricted to peer-reviewed publications involving US hospitals, written in English, and conducted with hospital patients at bedside; these studies had to feature economic or potential economic themes associated with POC-EEG. Economic or potential economic themes considered were budgetary implications, cost-benefits, cost-savings, healthcare resource utilization (including staffing), reimbursement, and other monetary-related data (such as LOS), all of which could be actual, possible, or theoretical. Studies about clinical management modifications associated with POC-EEG utilization that could lead or relate to any of these themes were also considered. Adhering to these same guidelines, pertinent references within relevant publications were assessed for applicability.
Conference abstracts, gray literature offerings, frank advertisements, white papers, and studies conducted outside the US were ineligible for this narrative review. Further excluded studies were those describing POC-EEG accuracy, feasibility, performance, or use that did not feature any implicit or explicit economic findings, such as research that tested the sensitivity and/or specificity of POC-EEG in seizure detection. summarizes study selection.
Figure 1. Overview of study design.
ConvEEG, Conventional electroencephalogram; EEG, Electroencephalogram; POC-EEG, Point-of-care electroencephalogram; US, United States.
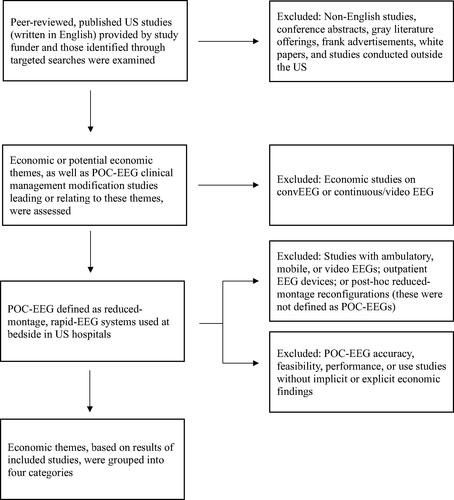
POC-EEGs were defined as reduced-montage, rapid-EEG systems utilized at bedside in US hospitals. Ambulatory, mobile, or video EEG devices, as well as EEG devices in the outpatient setting, were not regarded as POC-EEGs for this narrative review, nor were post-hoc full-montage to reduced-montage reconfigurations. These systems and others have been examined elsewhere, along with POC-EEGs in other countriesCitation48,Citation51–Citation53. Economic studies on convEEG or continuous/video EEG were additionally excluded.
Results
Twelve publications on the economic themes associated with POC-EEG were identified and cited in the results of this narrative review. These studies were published from 2018 to 2023; most were single center. All investigated a single rapid circumferential headband system. provides a more detailed overview of the literature presented. After examination of the economic themes presented in this included research, the study results were organized into four categories of patient care-driven economic evidence for POC-EEG: refining clinical management, reducing unnecessary patient transfers and hospital LOS, improving reimbursement, and mitigating burdens on healthcare staff and hospitals.
Table 1. Summary of included studies.
Refining clinical management
Findings in eight studies indicated that POC-EEG could help fine-tune clinical management, which has possible economic implications. Overall, the trend was that POC-EEG supported physicians’ ability to rule out seizures, prompting decreased administration or planned administration of unnecessary ASMs.
The multicenter prospective observational DECIDE trial was the pivotal study that demonstrated POC-EEG’s utility in influencing clinical management among physicians. It was conducted at five academic hospitals across the US. Study researchers distributed the same questionnaire to physicians before and after POC-EEG use to quantify treatment plan changes for patients with altered mental status. Due to evaluation with POC-EEG, physicians modified their diagnostic suspicion for seizures and adjusted treatment decisions in approximately 40% (n = 72/179) and 20% (n = 36/179) of cases, respectively. They were also less likely to intensify treatment with ASMs following utilization of POC-EEG in almost 13% (n = 23/179) of patientsCitation23.
Findings similar to those in the DECIDE trialCitation23 have been repeated in other prospective studies in various US hospitals, further corroborating that POC-EEG can play a role in improving clinical management. In Stanford University Hospital (SUH) ICUs, Hobbs et al.Citation43 showed that POC-EEG usage after initial assessment spurred physicians to amend treatment in 40% (n = 14/35) of suspected NCSE cases, precipitating treatment with significantly fewer ASMs (p = 0.01). Additionally, POC-EEG evaluation may have averted intubation of a patient scheduled for further medical careCitation43. At a California community hospital ICU, POC-EEG for suspected seizures helped enable de-escalation of antiseizure treatment in 40% (n = 4/10) of patientsCitation42. A study at a Maryland teaching hospital likewise found that negative POC-EEG evaluations decreased overtreatment with ASMs in 90% (n = 9/10) of patients concerning for SECitation50. Furthermore, at the EDs of Stanford Health Care (SHC) in California and Episcopal Hospital (EH) in Pennsylvania, POC-EEG impacted clinical management in over half (53%, n = 20/38) of suspected NCSE cases at the two hospitals. Compared with treating only on clinical suspicion, EH emergency physicians, who were auditorily interpreting sonified POC-EEG data, prescribed fewer ASMs in 43% (n = 6/14) of their cases after POC-EEG monitoring. (On-call neurologist visual inspection verified the diagnosesCitation54.)
Two retrospective studies of POC-EEG also emphasized this economic theme of refining clinical management. Kurup et al.Citation55 performed a chart review of 100 patients assessed with POC-EEG for any reason at SUH ED and ICU. For the 19 (19%) patients presenting with highly epileptiform or generalized/focal seizures on POC-EEG, 84% (n = 16/19) received or continued to receive ASMs, in contrast to 51% (n = 41/81) of those presenting with normal or nonepileptic encephalopathic patterns (NL/SL) on POC-EEG. ASM initiation or continuation was significantly less probable for patients with NL/SL after POC-EEG, compared with patients for whom seizures were not ruled out after POC-EEG (Χ2 = 7.086, p = 0.0078)Citation55. Kozak et al.Citation44 analyzed POC-EEG data over the span of one year from 157 ED patients at Providence Mission Hospital Mission Viejo, a community hospital in California. POC-EEG evaluation resulted in discontinuation of ASMs in 49 (53%) of the 93 patients who had received ASMs prior to POC-EEG. Of the 88 patients treated with ASMs after POC-EEG assessment, 44 (50%) patients had received ASMs based on POC-EEG findings. Altogether, clinical management amendments were significantly correlated with POC-EEG findings (p < 0.001)Citation44.
To determine financial implications from POC-EEG’s influence on clinical management, Ney et al.Citation25 developed a decision-analytic model from extracted data of the DECIDE trialCitation23 and other sources, such as the 2017 National Inpatient Sample datasetCitation56. The model examined the costs and benefits of treating patients with suspected seizures based on POC-EEG versus treating based only on clinical assessment. For each POC-EEG use, the authors determined an average savings of $3,971, given the projected 51% decrease in parenteral ASM treatments and intubations, along with the shorter ICU and hospital LOS. These conclusions, including the monetary savings, were theoretical, since they were derived from a model, not from a randomized clinical trial or prospective studyCitation25.
Reducing unnecessary patient transfers and hospital LOS
Five studies illustrated that POC-EEG can reduce both unneeded patient transfers to other sites for EEG assessment and extended hospital LOS. In conjunction with refining clinical management, decreasing unnecessary patient transfers and LOS may retrench hospital expenses.
Madill et al.Citation57 examined the introduction of POC-EEG at SHC ValleyCare, a community hospital in California. Before the introduction of POC-EEG, patients arriving outside business hours with suspected NCS were transferred to SUH for convEEG or were evaluated during the next business day. The authors found 33 cases of suspected seizure in sixteen months that would have met criteria for transfer before POC-EEG implementation. With POC-EEG, 2 (6%, n = 2/33) of these patients were transferred to SUH for EEG. Based on an average cost of $1,274 per patient for inter-facility transfer via ambulanceCitation58, the researchers calculated savings of over $39,000 just for ground transportation during the study period from December 2018 to the end of March 2020Citation57.
In a prospective cohort study of POC-EEG in a New Jersey community hospital setting, a mean of 2 (n = 22 across 11 months) patients each month were transferred offsite for emergent EEG prior to POC-EEG introduction. Over the ten months since using POC-EEG, these two hospitals experienced a 45% reduction in mean transfer rate (1.1 transfers per month) for emergent EEG. Ward et al.Citation46 calculated a net loss of about $3,463 for each historically transferred patient ($4,037, the transfer reimbursement, subtracted from $7,500, the transfer cost). For each non-transferred patient evaluated with POC-EEG, POC-EEG was attributed with a total gain of almost $14,000, according to the approximate avoided transfer cost ($3,463) combined with the mean amount collected ($11,161) and acknowledging the headband cost ($688). Ultimately, the savings from POC-EEG from reducing unnecessary transfers were projected at over $37,000 for the year ([$3,463.11/patient transfer × mean of 2 patient transfers/month × 12 months] – [$3,463.11/patient transfer × mean of 1.1 patient transfers/month × 12 months] = $37,401.59). Further cost offsets could occur if interfacility transfers were by air ambulance, due to remoteness or medical urgency, in which averted transfers could save over $40,000 in transportation costs per patientCitation46.
Despite these potential financial benefits, the price of POC-EEG must also be considered. The authors of the previous research elaborated that POC-EEG would have to preclude about 0.7 transfers per month, or 8.6 patients annually, to offset the multi-hospital system cost, including disposables, of the POC-EEG ($119,700 per year) in their studyCitation46.
Along with fewer transfers, some of the research demonstrated that POC-EEG usage and abbreviated length of hospitalization were linked. Kozak et al.Citation44, in their retrospective study at a California community hospital ED, found a positive association between ED (p = 0.04) and hospital LOS (p < 0.001) and the time from patients’ first ED evaluation to the initiation of POC-EEG. In another retrospective study at a different community hospital in northern California, hospital days were also fewer for patients receiving POC-EEG. Among 62 patients assessed with POC-EEG, Eberhard and BeckermanCitation47 found a median LOS decrease of 3 days (4 versus 7 days before and after POC-EEG introduction at the hospital, respectively), yet this was not significant (p = 0.058).
Ney et al.Citation25 reported that shorter LOS (0.4 and 1.2 days fewer for ICU and hospital LOS, respectively) contributed to the estimated average savings ($3,971 per patient) with POC-EEG utilization in the decision-analytic model based on DECIDE trial findingsCitation23. LOS for false-positive suspected seizure was the main factor in total cost variance, since these patients received ASMs, intubations, and ventilations that could have been avoided with more informed treatment decisionsCitation25. Similarly, Eberhard and BeckermanCitation47 noted potential cost reductions due to decreases in LOS with POC-EEG usage in their aforementioned study at the northern California community hospital. From an extrapolation of their six-month findings across one year, they postulated that fewer hospitalization days and ED discharges with POC-EEG implementation would be linked with nearly $740,000 in annual savings. The costs of equipment were incorporated in the financial analysisCitation47. Again, however, these two models by Ney et al.Citation25 and Eberhard and BeckermanCitation47 were derived from calculations of real-world data, not the results of a priori clinical trial analyses.
Improving reimbursement
Combined with the other categories of economic impact, securing additional reimbursement for NCS or NCSE patients via POC-EEG may support hospital finances, as shown in four of the studies identified for this narrative review. Medical Severity-Diagnosis Related Group (MS-DRG) with baseline DRG, as well as elevated reimbursement categories relating to complication or comorbidity (CC) or major complication or comorbidity (MCC) assignment, plays a role in optimizing hospital reimbursement with POC-EEG.
As Madill et al.Citation57 explained, transferring hospitals may forfeit a proportion of reimbursement within the Medicare Part A MS-DRG to the hospitals that receive and treat the transferred patientCitation59. In the 2023 study examining patient transfers at the New Jersey community hospital setting, the approximate mean amount collected ($11,161) for a non-transferred patient assessed with POC-EEG was greater than that of a historically transferred patient ($4,037) in the control group. As described above, POC-EEG would have to preclude the transfer of 8.6 patients annually to offset its multi-hospital system cost ($119,700/year ÷ $13,936.44/patient = 8.59 patients/year), which accounted for the reimbursement differences for transferred versus non-transferred patientsCitation46.
Regarding MS-DRG appropriate medical severity coding, a retrospective cohort study analyzed over 350 POC-EEG recordings from patients at six US academic and community hospitals. In total, epileptiform abnormalities or seizures were detected in 33% (n = 141/353) of casesCitation40. If the primary diagnosis for these cases were non-seizure, then POC-EEG could have enabled hospitals to code for the additional CC/MCC DRGs, thus bolstering reimbursement.
Indeed, Eberhard and BeckermanCitation47 evinced that use of POC-EEG was associated with higher MS-DRG coding (“with CC/MCC” DRGs) in their retrospective study on POC-EEG implementation at the northern California hospital. Within the six-month period after POC-EEG was introduced, POC-EEG detected seizures in 15 patients, 10 (67%) of whom had a primary diagnosis of non-seizure. Subsequently, 1 (10%, n = 1/10) patient had an MS-DRG “with MCC,” while the other 9 (90%, n = 9/10) patients coded for MS-DRG “with CC.” The authors projected a total gain of almost $146,000 for their hospital from MS-DRG reimbursements for one year of POC-EEG usage. This value, combined with the nearly $740,000 in annual savings from fewer hospitalization days and ED discharges, and including the additional equipment costs (over $200,000), would lead to a positive yearly income (about $683,000) for this hospital ([$145,580 + $737,818] – $200,240 = $683,158)Citation47. When considering the impact of both transfer savingsCitation46,Citation57 and the reimbursements associated with POC-EEGCitation47, the overall annual financial impact of POC-EEG may be greater, even as operating costs are applied.
Mitigating burdens on healthcare staff and hospitals
Lastly, several studies suggested that the adoption of POC-EEG could mitigate burdens on healthcare staff and hospitals. For 38% (n = 15/40) of control cases with convEEG assessment from the earlier study at the Maryland teaching hospital by LaMonteCitation50, EEG technologists were requested after normal business hours. However, none of the patients evaluated with POC-EEG required EEG technologist support outside workday hours, representing a marked diminution in after-hours EEG technologist labor (p = 0.02)Citation50.
Further, of 118 POC-EEG cases cited by Madill et al.Citation57, only 31% (n = 37/118) overlapped with SHC ValleyCare’s typical business hours; this included 1 (10%, n = 1/10) seizure case. Kozak et al.Citation44 noted that just under half (45%, n = 71/157) of POC-EEG evaluations were conducted during business hours in the community hospital ED setting, consisting of 14 (64%) of 22 seizure cases. In these studies, the capacity for after-hours convEEG was either limitedCitation44 or nonexistentCitation57, which may encumber EEG technologists and incur overtime or other additional costs when occurringCitation44.
Healthcare staff, including during COVID-19 isolation, have also positively commented on the impact of POC-EEGCitation50, such as its user-friendlinessCitation23,Citation42,Citation43, and this is crucial for non-specialist operation. Although more causal proof is necessary, POC-EEG may alleviate scheduling and work burdens where both labor (EEG technologists) and equipment (convEEG) are scarce.
Discussion
POC-EEGs are rapid screening tests for NCS and NCSE in critical care and emergency medicineCitation21,Citation48; they can confirm or rule out NCS or NCSE diagnoses when convEEG technology is constrained. This narrative review examined the economic value of POC-EEG, a resource that has been implemented in hospitals across the US in recent years to expedite patient care in the detection of NCS and NCSECitation48,Citation51,Citation52. Key patient care-driven economic categories were refining clinical management, reducing unnecessary patient transfers and hospital LOS, improving reimbursement, and mitigating burdens on healthcare staff and hospitals.
Utilization of unnecessary anti-epileptic treatments and procedures is costly and can lengthen hospitalization stay for patients who must recover from excess benzodiazepines and ventilationsCitation25,Citation32. POC-EEG can fine-tune clinical management via quickly screening for NCS and NCSE, particularly when convEEG and EEG technologists are not readily availableCitation23,Citation25,Citation42–44,Citation50,Citation54,Citation55. This in turn could precipitate cost-savingsCitation25. Moreover, POC-EEG has shown economic value in eliminating the need for patient transfers, which can be expensive, through enabling physicians to assess for NCS and NCSE on-siteCitation46,Citation57. Transfers can additionally generate anxiety for patients and their family members, squander healthcare resources, and encumber society through inflating Medicare and/or Medicaid costs for federally insured patientsCitation60,Citation61.
Prolonged LOS can be harmful for patients, including those with NCS or NCSE and those incorrectly treated for it, as well as to the healthcare systemCitation32. Since POC-EEG assessment can affect clinical management, it may curb overtreatment and shorten hospitalization stayCitation25,Citation44,Citation47, thereby paring expenses and improving clinical outcomes. POC-EEG has the capacity to bolster reimbursement through avoiding unnecessary transfers and triggering higher Medical Severity DRG-related billing codesCitation46,Citation47,Citation57. The latter is notable because the average additional reimbursement for an MS-DRG “with MCC” is about $7,000 for conditions linked frequently with NCSECitation22,Citation62. Finally, POC-EEG adoption could lead to savings in EEG technologist labor expenditures for staffing convEEG requests outside business hoursCitation44,Citation50,Citation57. Overtime and lengthy working hours have been correlated with burnout across various fields of medicine, in conjunction with health risks, such as ischemic heart disease, motor vehicle accidents, stroke, workplace injury, and deathCitation63–65. Of course, neurologist or neurology subspecialists must still be present outside weekday workday hours, including overnight shifts, to interpret POC-EEG recordings.
POC-EEG tradeoffs
Although convEEG systems have their own costs, both upfront and annualizedCitation66, they differ from those of POC-EEG and depend on specific hospital infrastructure. It however must be reiterated that POC-EEG does not replace convEEG, the work of EEG technologists, or the neurology review of EEG recordings, nor does it render them obsoleteCitation48–50. POC-EEG indeed has limitations in comparison with convEEG. Overall, convEEG via the International 10–20 electrode system provides a more comprehensive evaluation of electrographic activity in patients than does POC-EEG with its fewer electrodesCitation21,Citation49,Citation50. The rapid circumferential headband system mentioned in this narrative review, for example, does not place electrodes in the midline or parasagittal regions of the brain. Consequently, it may not detect midline or parasagittal seizures, which have a prevalence of about 1%, or other epileptic abnormalities in these areasCitation43,Citation49.
Further, convEEG has the potential to be used with concomitant video, as endorsed by the American Clinical Neurophysiology SocietyCitation22 to aid physicians in patient care, whereas POC-EEG does not. Signal artifacts have in turn been more frequent in POC-EEG than convEEG evaluation; this may obfuscate interpretation of electrographic dataCitation41. POC-EEG also has a lower sampling rate (250 Hz) than convEEG (200–1,000 Hz)Citation46, thus limiting analysis in the high frequency domain. Ease of POC-EEG application by a range of healthcare providersCitation23,Citation42,Citation43 for rapid assessment of patients, as previously described, may counter some of these limitations. Similarly, when integrated with an automated algorithm, POC-EEG can be used to continuously monitor and alert bedside providers of suspected NCS or NCSE within minutes, whereas convEEG requires human monitoring at an infrequent rateCitation40. Ultimately, when utilized as a screening tool and monitor for NCS or NCSE in critical care and emergency medicine, POC-EEG complements but does not supplant convEEGCitation48–50.
Limitations
This narrative review was based on targeted searches, as depicted in Table S1 and . It was not a systematic review conducted under PRISMA guidelines and should not be held to the same reporting standards. The studies identified in this narrative review were largely local or regional in geographic scope. As such, the findings may not be generalizable to all hospitals in the US or inpatient medical centers in non-US settings, like those in low-income countries. Additionally, it is unknown whether the financial gains of POC-EEG would be applicable to pediatric patients because the research in this narrative review was almost exclusively based on adult patients.
Sample sizes in some of the included studies were also modest, with two having only 10 patients receiving POC-EEGCitation42,Citation50. In the study by Kozak et al.Citation44, neither ED nor hospital LOS was significantly associated with the time to first ASM treatment after POC-EEG utilization. Further, Ney et al.Citation25 primarily sourced clinical parameters from the DECIDE trialCitation23 for their model; its generalizability outside academic centers is uncertainCitation25. The twelve included studies were observational, not randomized controlled trials, which exposes them to systemic biases in how the data were collected and reportedCitation67, particularly as the findings were in favor of POC-EEG.
All studies presented here investigated a single rapid circumferential headband system; the evidence on this device may not translate to other POC-EEGs. In the absence of a systematic review or meta-analytic framework, formal testing for publication bias, such as Begg’sCitation68 and Egger’s testsCitation69, are not available. On average, quality of the cohort studies cited within the results was fair (Table S2) according to the Newcastle-Ottawa scaleCitation70; in general, nonetheless, assessment of these publications was not well suited to this scale given their aims and design. Examining the disclosure statements of the twelve cited publications also revealed that the majority of study authors received payment or support from the manufacturer of that POC-EEG, who funded this narrative review. Similarly, negative economic outcomes linked with this particular POC-EEG were not identified.
Future directions
We have endeavored to survey pertinent available evidence for POC-EEG, yet a systematic review would likely expand this narrative review. The research on clinical management was the most robust, but overall, more studies analyzing this topic and these evidence categories are imperative, especially those with greater sample sizes, more geographic variety, and the resulting outcomes of clinical decisions. Assessing the long-term financial consequences of NCS and NCSE accrued over time, along with the influence of POC-EEG on reimbursement through Medicare Part A MS-DRGsCitation59, would be valuable as well. Since POC-EEGs have been implemented in other countriesCitation48,Citation53, it would furthermore be interesting to analyze whether POC-EEG utilization is more widespread around the globe and which unique challenges have arisen from it.
More broadly, point-of-care testing expands past POC-EEG utilization in the detection of NCS or NCSE in US hospital patients. Medical point-of-care devices, such as cardiac monitorsCitation71, point-of-care ultrasoundCitation72, and pupilometersCitation73, have been developed for multiple indications. Some devices, like cardiac monitors, are more widespread than POC-EEG, for individuals can use them at their own discretionCitation71. Others must be actuated by qualified personnel and are restricted to inpatient units or hospitalsCitation72,Citation73. The goal of all these point-of-care technologies, nevertheless, mirrors that of POC-EEG; they aim to quickly and specifically assess certain aspects of patient health so that precise treatment is initiated soonerCitation74. Like with POC-EEG, the utility and finances of these devices compared with conventional devices should continue to be explored, both in and outside the US.
Conclusions
POC-EEG can refine clinical management of hospitalized patients with suspected seizures, reduce unnecessary patient transfers and hospital LOS, improve reimbursement, and mitigate burdens on healthcare staff and hospitals, all of which are accompanied with potential economic benefits. As an adjunct to convEEG, POC-EEG is an expeditious screening device for identifying NCS or NCSE in critical care and emergency medicineCitation48–50 with the promise of financial advantages over standard care.
Transparency
Declaration of financial/other interests
AG is a paid consultant for Ceribell, Inc. MEW is a paid employee of Costello Medical Consulting, Inc., who was contracted by Ceribell, Inc. to provide medical writing assistance. JPN is a paid consultant for Ceribell, Inc., an advisor for Forsquare Consulting, and has consulted for Potrero, Inc. and Briova RX.
Author contributions
AG, MEW, and JPN had substantial contributions to conception, design, analysis, and interpretation of the data, drafted the paper or reviewed it critically for intellectual content, had final approval of the version to be published, and agreed to be accountable for all aspects of the work.
Reviewer disclosures
A reviewer on this manuscript has disclosed that they have received funds for travel and conference attendance, as well as meals, from Ceribell. Peer reviewers on this manuscript have no other relevant financial relationships or otherwise to disclose.
Previous presentations
None.
Supplemental Material
Download MS Word (35.5 KB)Supplemental Material
Download MS Word (35.3 KB)Acknowledgements
The authors would like to thank the teams at Ceribell, Inc. and Costello Medical Consulting, Inc. for their guidance and writing assistance.
Additional information
Funding
References
- Wylie T, Sandhu DS, Murr N. Status epilepticus [Internet]. Treasure Island (Florida): StatPearls Publishing; 2023 [cited 2023 September 22]. Available from: https://www.ncbi.nlm.nih.gov/books/NBK430686/.
- Brophy GM, Bell R, Claassen J, et al. Guidelines for the evaluation and management of status epilepticus. Neurocrit Care. 2012;17(1):3–23. doi: 10.1007/s12028-012-9695-z.
- Hirsch LJ, Fong MWK, Leitinger M, et al. American Clinical Neurophysiology Society’s standardized critical care EEG terminology: 2021 version. J Clin Neurophysiol. 2021; 38(1):1–29. doi: 10.1097/WNP.0000000000000806.
- Wasterlain CG, Fujikawa DG, Penix L, et al. Pathophysiological mechanisms of brain damage from status epilepticus. Epilepsia. 1993;34 Suppl 1(s1):S37–S53. doi: 10.1111/j.1528-1157.1993.tb05905.x.
- Trinka E, Rainer LJ, Granbichler CA, et al. Mortality, and life expectancy in epilepsy and status epilepticus—current trends and future aspects. Front Epidemiol. 2023;3:1081757. doi: 10.3389/fepid.2023.1081757.
- Hesdorffer DC, Logroscino G, Cascino G, et al. Incidence of status epilepticus in Rochester, Minnesota, 1965–1984. Neurology. 1998; 50(3):735–741. doi: 10.1212/wnl.50.3.735.
- DeLorenzo RJ, Hauser WA, Towne AR, et al. A prospective, population-based epidemiologic study of status epilepticus in Richmond, Virginia. Neurology. 1996;46(4):1029–1035. doi: 10.1212/wnl.46.4.1029.
- Guterman EL, Betjemann JP, Aimetti A, et al. Association between treatment progression, disease refractoriness, and burden of illness among hospitalized patients with status epilepticus. JAMA Neurol. 2021;78(5):588–595. doi: 10.1001/jamaneurol.2021.0520.
- Trinka E, Cock H, Hesdorffer D, et al. A definition and classification of status epilepticus–report of the ILAE task force on classification of status epilepticus. Epilepsia. 2015; 56(10):1515–1523. doi: 10.1111/epi.13121.
- Beniczky S, Hirsch LJ, Kaplan PW, et al. Unified EEG terminology and criteria for nonconvulsive status epilepticus. Epilepsia. 2013; 54 Suppl 6(s6):28–29. doi: 10.1111/epi.12270.
- Laccheo I, Sonmezturk H, Bhatt AB, et al. Non-convulsive status epilepticus and non-convulsive seizures in neurological ICU patients. Neurocrit Care. 2015; 22(2):202–211. doi: 10.1007/s12028-014-0070-0.
- Rittenberger JC, Popescu A, Brenner RP, et al. Frequency and timing of nonconvulsive status epilepticus in comatose post-cardiac arrest subjects treated with hypothermia. Neurocrit Care. 2012; 16(1):114–122. doi: 10.1007/s12028-011-9565-0.
- Vespa PM, Nuwer MR, Nenov V, et al. Increased incidence and impact of nonconvulsive and convulsive seizures after traumatic brain injury as detected by continuous electroencephalographic monitoring. J Neurosurg. 1999; 91(5):750–760. doi: 10.3171/jns.1999.91.5.0750.
- Octaviana F, Harisman J, Wiratman W, et al. Altered mental status in moderate-severe traumatic brain injury in Indonesia: the clinical manifestation and EEG features of non-convulsive status epilepticus. Heliyon. 2021; 7(9):e08067. doi: 10.1016/j.heliyon.2021.e08067.
- Holtkamp M, Meierkord H. Nonconvulsive status epilepticus: a diagnostic and therapeutic challenge in the intensive care setting. Ther Adv Neurol Disord. 2011; 4(3):169–181. doi: 10.1177/1756285611403826.
- Alessandri F, Badenes R, Bilotta F. Seizures and sepsis: a narrative review. J Clin Med. 2021; 10(5):1041. doi: 10.3390/jcm10051041.
- Rudin D, Grize L, Schindler C, et al. High prevalence of nonconvulsive and subtle status epilepticus in an ICU of a tertiary care center: a three-year observational cohort study. Epilepsy Res. 2011; 96(1–2):140–150. doi: 10.1016/j.eplepsyres.2011.05.018.
- Sánchez Fernández I, Amengual-Gual M, Barcia Aguilar C, et al. Estimating the cost of status epilepticus admissions in the United States of America using ICD-10 codes. Seizure. 2019; Oct71:295–303. doi: 10.1016/j.seizure.2019.09.001.
- Smith SJ. EEG in the diagnosis, classification, and management of patients with epilepsy. J Neurol Neurosurg Psychiatry. 2005; 76 Suppl 2(Suppl 2):ii2–7. doi: 10.1136/jnnp.2005.069245.
- Kilbride RD, Costello DJ, Chiappa KH. How seizure detection by continuous electroencephalographic monitoring affects the prescribing of antiepileptic medications. Arch Neurol. 2009; 66(6):723–728. doi: 10.1001/archneurol.2009.100.
- Westover MB, Gururangan K, Markert MS, et al. Diagnostic value of electroencephalography with ten electrodes in critically ill patients. Neurocrit Care. 2020;33(2):479–490. doi: 10.1007/s12028-019-00911-4.
- Herman ST, Abend NS, Bleck TP, et al. Consensus statement on continuous EEG in critically ill adults and children, part I: indications. J Clin Neurophysiol. 2015; 32(2):87–95. doi: 10.1097/WNP.0000000000000166.
- Vespa PM, Olson DM, John S, et al. Evaluating the clinical impact of rapid response electroencephalography: the DECIDE multicenter prospective observational clinical study. Crit Care Med. 2020;48(9):1249–1257. doi: 10.1097/CCM.0000000000004428.
- Kamousi B, Grant AM, Bachelder B, et al. Comparing the quality of signals recorded with a rapid response EEG and conventional clinical EEG systems. Clin Neurophysiol Pract. 2019;4:69–75. doi: 10.1016/j.cnp.2019.02.002.
- Ney JP, Gururangan K, Parvizi J. Modeling the economic value of Ceribell Rapid Response EEG in the inpatient hospital setting. J Med Econ. 2021; 24(1):318–327. doi: 10.1080/13696998.2021.1887877.
- Cornell Law School. 5 U.S. Code § 6101 - Basic 40-hour workweek; work schedules; regulations [Internet]. Ithaca (NY):[cited 2023 May 2]. Available from: https://www.law.cornell.edu/uscode/text/5/6101.
- Gururangan K, Razavi B, Parvizi J. Utility of electroencephalography: experience from a U.S. tertiary care medical center. Clin Neurophysiol. 2016;127(10):3335–3340. doi: 10.1016/j.clinph.2016.08.013.
- Gaínza-Lein M, Fernández IS, Ulate-Campos A, et al. Timing in the treatment of status epilepticus: from basics to the clinic. Seizure. 2019; 68:22–30. doi: 10.1016/j.seizure.2018.05.021.
- Rossetti AO, Schindler K, Sutter R, et al. Continuous vs routine electroencephalogram in critically ill adults with altered consciousness and no recent seizure: a multicenter randomized clinical trial. JAMA Neurol. 2020;77(10):1225–1232. doi: 10.1001/jamaneurol.2020.2264.
- Hill CE, Blank LJ, Thibault D, et al. Continuous EEG is associated with favorable hospitalization outcomes for critically ill patients. Neurology. 2019; 92(1):e9–e18. doi: 10.1212/WNL.0000000000006689.
- Chamberlain JM, Kapur J, Shinnar S, et al. Efficacy of levetiracetam, fosphenytoin, and valproate for established status epilepticus by age group (ESETT): a double-blind, responsive-adaptive, randomised controlled trial. Lancet. 2020; 395(10231):1217–1224. doi: 10.1016/S0140-6736(20)30611-5.
- Spatola M, Alvarez V, Rossetti AO. Benzodiazepine overtreatment in status epilepticus is related to higher need of intubation and longer hospitalization. Epilepsia. 2013;54(8):e99–e102. doi: 10.1111/epi.12235.
- Silbergleit R, Durkalski V, Lowenstein D, et al. Intramuscular versus intravenous therapy for prehospital status epilepticus. N Engl J Med. 2012; 366(7):591–600. doi: 10.1056/NEJMoa1107494.
- Jungilligens J, Michaelis R, Popkirov S. Misdiagnosis of prolonged psychogenic non-epileptic seizures as status epilepticus: epidemiology and associated risks. J Neurol Neurosurg Psychiatry. 2021; 92(12):1341–1345. doi: 10.1136/jnnp-2021-326443.
- Cengiz O, Jungilligens J, Michaelis R, et al. Dissociative seizures in the emergency room: room for improvement. J Neurol Neurosurg Psychiatry. 2023;jnnp-2023-332063. doi: 10.1136/jnnp-2023-332063.
- Wasserman D, Herskovitz M. Epileptic vs psychogenic nonepileptic seizures: a video-based survey. Epilepsy Behav. 2017;73:42–45. doi: 10.1016/j.yebeh.2017.04.020.
- Shrank WH, Rogstad TL, Parekh N. Waste in the US health care system: estimated costs and potential for savings. JAMA. 2019;322(15):1501–1509. doi: 10.1001/jama.2019.13978.
- Sathe AG, Underwood E, Coles LD, et al. Patterns of benzodiazepine underdosing in the Established Status Epilepticus Treatment Trial. Epilepsia. 2021;62(3):795–806. doi: 10.1111/epi.16825.
- Uppal P, Cardamone M, Lawson JA. Outcomes of deviation from treatment guidelines in status epilepticus: a systematic review. Seizure. 2018;58:147–153. doi: 10.1016/j.seizure.2018.04.005.
- Kamousi B, Karunakaran S, Gururangan K, et al. Monitoring the burden of seizures and highly epileptiform patterns in critical care with a novel machine learning method. Neurocrit Care. 2021; 34(3):908–917. doi: 10.1007/s12028-020-01120-0.
- Rittenberger JC, Weissman A, Baldwin M, et al. Preliminary experience with point-of-care EEG in post-cardiac arrest patients. Resuscitation. 2019; 135:98–102. doi: 10.1016/j.resuscitation.2018.12.022.
- Yazbeck M, Sra P, Parvizi J. Rapid response electroencephalography for urgent evaluation of patients in community hospital intensive care practice. J Neurosci Nurs. 2019; 51(6):308–312. doi: 10.1097/JNN.0000000000000476.
- Hobbs K, Krishnamohan P, Legault C, et al. Rapid bedside evaluation of seizures in the ICU by listening to the sound of brainwaves: a prospective observational clinical trial of Ceribell’s brain stethoscope function. Neurocrit Care. 2018; 29(2):302–312. doi: 10.1007/s12028-018-0543-7.
- Kozak R, Gururangan K, Dorriz PJ, et al. Point-of-care electroencephalography enables rapid evaluation and management of non-convulsive seizures and status epilepticus in the emergency department. J Am Coll Emerg Physicians Open. 2023;4(4):e13004. doi: 10.1002/emp2.13004.
- Benbadis SR, Beniczky S, Bertram E, et al. The role of EEG in patients with suspected epilepsy. Epileptic Disord. 2020; 22(2):143–155. doi: 10.1684/epd.2020.1151.
- Ward J, Green A, Cole R, et al. Implementation and impact of a point of care electroencephalography platform in a community hospital: a cohort study. Front Digit Health. 2023;5:1035442. doi: 10.3389/fdgth.2023.1035442.
- Eberhard E, Beckerman SR. Rapid-response electroencephalography in seizure diagnosis and patient care: lessons from a community hospital. J Neurosci Nurs. 2023;55(5):157–163. doi: 10.1097/JNN.0000000000000715.
- Davey Z, Gupta PB, Li DR, et al. Rapid response EEG: current state and future directions. Curr Neurol Neurosci Rep. 2022; 22(12):839–846. doi: 10.1007/s11910-022-01243-1.
- Gururangan K, Parvizi J. Midline and parasagittal seizures are rare in adult patients. Neurocrit Care. 2020; 32(1):193–197. doi: 10.1007/s12028-019-00804-6.
- LaMonte MP. Ceribell EEG shortens seizure diagnosis and workforce time and is useful for COVID isolation. Epilepsia Open. 2021; 6(2):331–338. doi: 10.1002/epi4.12474.
- Biondi A, Santoro V, Viana PF, et al. Noninvasive mobile EEG as a tool for seizure monitoring and management: a systematic review. Epilepsia. 2022;63(5):1041–1063. doi: 10.1111/epi.17220.
- Sharma S, Nunes M, Alkhachroum A. Adult critical care electroencephalography monitoring for seizures: a narrative review. Front Neurol. 2022;13:951286. doi: 10.3389/fneur.2022.951286.
- Simma L, Bauder F, Schmitt-Mechelke T. Feasibility and usefulness of rapid 2-channel-EEG-monitoring (point-of-care EEG) for acute CNS disorders in the paediatric emergency department: an observational study. Emerg Med J. 2021; 38(12):919–922. doi: 10.1136/emermed-2020-209891.
- Wright NMK, Madill ES, Isenberg D, et al. Evaluating the utility of Rapid Response EEG in emergency care. Emerg Med J. 2021;38(12):923–926. doi: 10.1136/emermed-2020-210903.
- Kurup D, Davey Z, Hoang P, et al. Effect of rapid EEG on anti-seizure medication usage. Epileptic Disord. 2022;24(5):831–837. doi: 10.1684/epd.2022.1463.
- Agency for Healthcare Research and Quality. National Inpatient Sample: Healthcare Cost and Utilization Project (HCUP) [Internet]. Rockville (MD):2017 [cited 2020 May 1]. Available from: https://hcup-us.ahrq.gov/nisoverview.jsp.
- Madill ES, Gururangan K, Krishnamohan P. Improved access to rapid electroencephalography at a community hospital reduces inter-hospital transfers for suspected non-convulsive seizures. Epileptic Disord. 2022;24(3):507–516. doi: 10.1684/epd.2021.1410.
- Natafgi N, Shane DM, Ullrich F, et al. Using tele-emergency to avoid patient transfers in rural emergency departments: an assessment of costs and benefits. J Telemed Telecare. 2018; 24(3):193–201. doi: 10.1177/1357633X17696585.
- Centers for Medicare and Medicaid Services. Review of hospital compliance with Medicare’s transfer policy with the resumption of home health services & other information on patient discharge status codes [Internet]. Baltimore (MD):2021 [cited 2023 May 2]. Available from: https://www.cms.gov/files/document/se21001.pdf.
- Friebe I, Isaacs J, Mallu S, et al. Evaluation of appropriateness of patient transfers for hand and microsurgery to a level I trauma center. Hand. 2013;8(4):417–421. doi: 10.1007/s11552-013-9538-1.
- Acton EK, Blank LJ, Willis AW, et al. Interfacility transfers for seizure-related emergencies in the United States. Neurology. 2022; 99(24):e2718–e2727. doi: 10.1212/WNL.0000000000201319.
- Centers for Medicare and Medicaid Services. FY 2023 final rule and correcting amendment data files: Supplemental weights file [Internet]. 2023 [cited March 2023]. Available from: https://www.cms.gov/medicare/payment/prospective-payment-systems/acute-inpatient-pps/fy-2023-ipps-final-rule-home-page#DataFiles.
- Reith TP. Burnout in United States healthcare professionals: a narrative review. Cureus. 2018;10(12):e3681. doi: 10.7759/cureus.3681.
- Pega F, Náfrádi B, Momen NC, et al. Global, regional, and national burdens of ischemic heart disease and stroke attributable to exposure to long working hours for 194 countries, 2000-2016: a systematic analysis from the WHO/ILO joint estimates of the work-related burden of disease and injury. Environ Int. 2021;154:106595. doi: 10.1016/j.envint.2021.106595.
- Imes CC, Barthel NJ, Chasens ER, et al. Shift work organization on nurse injuries: a scoping review. Int J Nurs Stud. 2023;138:104395. doi: 10.1016/j.ijnurstu.2022.104395.
- Kolls BJ, Mace BE, Dombrowski KE. Implementation of continuous video-electroencephalography at a community hospital enhances care and reduces costs. Neurocrit Care. 2018; 28(2):229–238. doi: 10.1007/s12028-017-0468-6.
- Pandis N. Bias in observational studies. Am J Orthod Dentofacial Orthop. 2014; 145(4):542–543. doi: 10.1016/j.ajodo.2014.01.008.
- Begg CB, Mazumdar M. Operating characteristics of a rank correlation test for publication bias. Biometrics. 1994; 50(4):1088–1101. doi: 10.2307/2533446.
- Egger M, Davey Smith G, Schneider M, et al. Bias in meta-analysis detected by a simple, graphical test. BMJ. 1997; 315(7109):629–634. doi: 10.1136/bmj.315.7109.629.
- Wells GA, Shea B, O'Connell D, et al. The Newcastle-Ottawa Scale (NOS) for assessing the quality of nonrandomised studies in meta-analyses [Internet]. The Ottawa Hospital Research Institute; 2021 [cited 2023 November 14]. Available from: https://www.ohri.ca/programs/clinical_epidemiology/oxford.asp.
- Duncker D, Ding WY, Etheridge S, et al. Smart wearables for cardiac monitoring—real-world use beyond atrial fibrillation. Sensors. 2021;21(7):2539. doi: 10.3390/s21072539.
- Andersen CA, Holden S, Vela J, et al. Point-of-care ultrasound in general practice: a systematic review. Ann Fam Med. 2019; 17(1):61–69. doi: 10.1370/afm.2330.
- Zafar SF, Suarez JI. Automated pupillometer for monitoring the critically ill patient: a critical appraisal. J Crit Care. 2014; 29(4):599–603. doi: 10.1016/j.jcrc.2014.01.012.
- Vashist SK. Point-of-care diagnostics: recent advances and trends. Biosensors. 2017;7(4):62. doi: 10.3390/bios7040062.