Abstract
Aims
To provide an update on the cost-effectiveness of the chimeric antigen receptor (CAR) T-cell therapies axicabtagene ciloleucel (axi-cel) and tisagenlecleucel (tisa-cel) for the treatment of relapsed/refractory (r/r) large B-cell lymphoma (LBCL) among patients who have previously received ≥2 lines of systemic therapy using more mature clinical trial data cuts (60 months for axi-cel overall survival [OS] and 45 months for tisa-cel OS and progression-free survival [PFS]).
Methods
A partitioned survival model consisting of three health states (pre-progression, post-progression and death) was used to estimate quality-adjusted life years (QALYs) and costs associated with axi-cel and tisa-cel over a lifetime horizon. PFS and OS inputs for axi-cel and tisa-cel were based on a previously published matching-adjusted indirect treatment comparison (MAIC). Long-term OS and PFS were extrapolated using parametric survival mixture cure models (PS-MCMs). Costs of CAR-T cell therapy drug acquisition and administration, conditioning chemotherapy, apheresis, CAR T-specific monitoring, stem cell transplant, hospitalization, adverse events, routine care, and terminal care were sourced from US cost databases. Health state utilities were derived from previous publications. Model inputs were varied using a range of sensitivity and scenario analyses.
Results
Compared with tisa-cel, axi-cel resulted in 2.51 additional QALYs and $50,185 additional costs (an incremental cost-effectiveness ratio [ICER] of $19,994 per QALY gained). In probabilistic sensitivity analysis (PSA), the ICER for axi-cel versus tisa-cel was ≤$50,000/QALY in 99.4% of simulations and ≤$33,500 in 99% of simulations. Axi-cel remained cost-effective versus tisa-cel (assuming a willingness-to-pay threshold of $150,000 per QALY) across a range of scenarios.
Conclusions
With longer-term survival data, axi-cel continues to represent a cost-effective option versus tisa-cel for treatment of r/r LBCL among patients who have previously received ≥2 lines of systemic therapy, from a US payer perspective.
Introduction
Large B-cell lymphomas, (LBCL) include diffuse LBCL, primary mediastinal B-cell lymphoma, and transformed follicular lymphomaCitation1–3 are the most common type of non-Hodgkin lymphomaCitation4. Up to 50% of LBCL patients may be refractory to or may subsequently experience a relapse following first-line chemo-immunotherapy treatment (rituximab, cyclophosphamide, doxorubicin hydrochloride, vincristine, prednisolone [R-CHOP])Citation5. Historically, second-line treatment for relapsed/refractory (r/r) LBCL consisted of salvage chemotherapy followed by autologous stem cell transplant (ASCT), however around 50% of patients experienced disease progression within 4 yearsCitation6. Patients who relapsed after salvage therapy have historically a poor prognosis (median 6 months)Citation7.
The introduction of CAR T-cell therapy has transformed the treatment of 3 L + r/r large B-cell lymphoma, increasing the cure rate from approximately 10% to 40%Citation8. Axicabtagene ciloleucel (axi-cel) and tisagenlecleucel (tisa-cel) were approved by the FDA in 2017 and 2018, respectively for the treatment of adult patients with r/r LBCL after ≥2 lines of systemic therapyCitation9,Citation10. The registration trials for axi-cel (ZUMA-1Citation11) and tisa-cel (JULIETCitation12) were both Phase II, international, multicenter single-arm studies. A comparison of axi-cel and tisa-cel conducted using a matching-adjusted indirect comparison (MAIC) showed that axi-cel was associated with a greater objective response rate (relative risk [RR] 1.61 [95% CI 1.29, 2.01]), complete response (RR 1.62 [95% CI 1.16, 2.27]) and overall survival (OS) (hazard ratio [HR] 0.51 [95% CI 0.31, 0.83] from the point of infusion) than tisa-cel (based on 39.1 month and 33 month median follow-up for axi-cel and tisa-cel, respectively)Citation13.
A cost-effectiveness partitioned survival model which incorporated the MAIC effectiveness estimates for axi-cel vs tisa-cel demonstrated that axi-cel was associated with greater quality-adjusted life years (QALYs) and lower costs than tisa-cel over a lifetime horizonCitation14. These findings were robust to various scenarios and sensitivity analyses performedCitation14.
In 2021, a longer-term follow up of the JULIET study was published, reporting survival data at a median follow up of 40.3 monthsCitation15. Additional long-term survival data for 63.1 months is also now available for axi-cel from ZUMA-1, with a median OS of 25.8 monthsCitation16. Given that robust relative clinical and cost-effectiveness are of great interest to decision makers to obtain the best outcomes for patients and the best value for money for healthcare providers, an updated MAIC and cost-effectiveness analyses, using this longer-term follow-up data, was deemed necessary to provide greater confidence in the longer-term costs and benefits associated with the treatment of r/r LBCL among patients who previously received ≥2 lines of systemic therapy using axi-cel and tisa-cel.
Methods
Model overview
A partitioned survival model consisting of three health states (pre-progression, post-progression and death) was developed in Microsoft Excel to determine the cost-effectiveness of axi-cel versus tisa-cel for the treatment of r/r LBCL in patients who had previously received ≥2 lines of systemic therapy. The economic model structure has been described previouslyCitation14. The model included OS and PFS adjusted through a MAICCitation13 to correct imbalances in baseline characteristics between the ZUMA-1 and JULIET trials for axi-cel and tisa-cel treatment. Adjusted OS and PFS curves for the therapies were then applied to determine the proportion of patients in each model health state over time. Patients in each health state accrued life years, QALYs and costs (treatments, administrations, end of life care, adverse events, and resource use). Patient characteristics were based on the JULIET trial. The model was conducted over a lifetime horizon from a US payer perspective (2022 costs), with costs and benefits discounted at 3% in line with current recommendations from the Institute for Clinical and Economic ReviewCitation17. The primary outcomes of the model were life years (LY) gained, QALY gained and the incremental cost per QALY (ICER).
Model inputs
Survival data (PFS and OS)
MAIC
PFS (centrally assessed; used in the model base case) data for axi-cel were based on 24-month dataCitation18 and PFS (investigator assessed; used in a model scenario) and OS data for axi-cel were based on 60-month patient-level data. PFS and OS of tisa-cel was based on 45-month JULIET trial dataCitation15. MAIC weights utilized were derived from the previously published MAICCitation13.
Parametric survival models
Within the clinical trial period, health state occupancy could be determined directly from the KM curves of OS and PFS from ZUMA-1 for axi-cel and JULIET for tisa-cel. However, to examine long-term survival and resource use over a lifetime horizon, it was necessary to extrapolate the OS and PFS curves beyond the trial period. For the base case analysis, a partitioned survival mixture cure modelling (PS-MCM) approach was used, with survival estimates fitted for OS and PFS independently, as previously describedCitation14. The mixture-cure approach assumes that a proportion of patients in each cohort achieve long-term remission while the remaining patients are at risk of disease-related progression and disease-related mortalityCitation19,Citation20, with both populations subject to age- and gender-specific background mortalityCitation21. OS and PFS distributions used in the model were based on both the visual model fit and statistical model fit (as measure by the Akaike information criterion [AIC] for axi-cel and tisa-cel, see Supplementary Information Tables 1 and 2) and clinical expectations and plausibility of survival extrapolations.
Adverse events
Grade 3/4 adverse events (AEs) were considered in the model as previously describedCitation14. Briefly, AEs such as cytokine release syndrome (CRS) and neurotoxicity were assumed to be treated while patients were hospitalized for CAR T-cell therapy treatment, with the length of stay taken from the ZUMA-1 trial. A cohort average of 3.7 intensive care unit (ICU) days (as a sub-set of hospitalization days) was included, based on the duration of CRS events This approach to AE inclusion is in line with previous publications which have modelled the cost-effectiveness of axi-celCitation14,Citation22,Citation23. B-cell aplasia was considered separately; the proportion of patients receiving treatment for B-cell aplasia was based on ZUMA-1 data for axi-cel, while the dose and frequency of administration was based on a previously published economic modelCitation22. The proportion of tisa-cel patients receiving treatment for B-cell aplasia was based on data from the JULIET trial, as implemented in a previous economic modelCitation24 ().
Table 1. Model inputs.
Utilities
Utility values were applied to model health states as previously describedCitation14 and are presented in . Pre-progression utilities were based on data from ZUMA-1Citation26. Utilities for progressed disease were taken from the published literatureCitation27. In the base case analysis, a time cut-off of 60 months was applied to patients in the pre-progression health state. Given the uncertainty of the criteria for determining when patients will be considered to have improved utility, scenario analyses were performed to fully explore the robustness of the time cut-off.
Costs
Costs were included as per the previously published modelCitation14. All costs are expressed in 2022 US dollars. Costs obtained from published literature or data sources not reported in 2022 were inflated to 2022 dollars using the medical care component of the Consumer Price IndexCitation32. All costs used in the base case along with distributions used for sensitivity analyses and data sources are presented in .
CAR T-cell therapy
As CAR T-cell therapy is administered as a single treatment, all associated costs were applied as one-off costs during the first model cycle. In addition to drug acquisition costs which were based on wholesale acquisition costs (WAC) from RED BOOKCitation28,Citation29, CAR T-cell therapy treatment requires an apheresis procedure where T-cells are harvested from the patient’s blood, re-engineered and re-administered to the patient. Apheresis and administration costs were based on the Medicare reimbursement rate ().
Conditioning chemotherapy
After manufacturing the T-cells, patients receive one-time conditioning chemotherapy before the infusion of CAR T-cell therapy. Chemotherapy type and doses were based on treatments received by patients in the ZUMA-1 trial for axi-celCitation11 and the JULIET trial for tisa-celCitation12. Drug acquisition costs were based on WACs obtained from RED BOOKCitation29 and administration costs were based on the Medicare reimbursement rate as detailed in . For all drugs for CAR T-cell conditioning chemotherapy, a dose intensity of 100% and no vial sharing was assumed. In line with previous cost-effectiveness analysis, it was assumed that all patients received one positron emission tomography (PET)/computed tomography (CT) scan and four follow-up office visits after CAR T-cell infusionCitation22.
AEs
Drug acquisition costs for intravenous immunoglobulin for the treatment of B-cell aplasia were based on 2019 WACs obtained from RED BOOKCitation33 and inflated to 2022 costsCitation29 and the administration cost was obtained from the CMS Physician Fee ScheduleCitation31.
Stem cell transplant (SCT)
SCT costs were included for patients who were refractory to or who relapsed after CAR T-cell therapy (). An SCT rate of 7.9% was applied for axi-cel based on 1-year ZUMA-1 follow up data (Kite Pharma, data on file). An SCT rate of 5.4% for tisa-cel (6/111 patients) was used from the JULIET trialCitation12. The cost of SCT was based on the cost of allogeneic SCT from Broder et al.Citation34 For simplicity, SCT costs were applied as one-off costs in the first model cycle.
Hospitalization
The model assumed that patients were hospitalized during CAR T-cell therapy treatment and costs were applied as a one-off during the first model cycle. The hospital length of stay and the proportion of patients requiring ICU admission for axi-cel and tisa-cel was based on ZUMA-1 and JULIET data, respectively as previously reported in the published literatureCitation22,Citation35. Hospitalization costs for ICU and non-ICU stays were obtained from previous publications and the Medicare DRG Reimbursements for the Hospitalizations assigned in the Healthcare Cost and Utilization ProjectCitation36,Citation37 ().
Routine disease management
All r/r LBCL patients who remained alive were assumed to have the same routine care costs per month, based on disease-related imaging procedure costs (total disease-related health care costs, excluding chemotherapy, radiation therapy, AE and SCT costs) for the relapsed LBCL populationCitation38 ().
End of life care
Terminal care costs were applied in the model upon death (regardless of the cause of death) to account for resources utilized at the end of life. Terminal care costs were taken from Kutikova et al. 2006Citation39 and applied for 3 months in line with Roth et al.Citation22 ().
Sensitivity analysis
Deterministic sensitivity analysis (DSA) and probabilistic sensitivity analysis (PSA) were conducted to test the robustness of the base case results. The uncertainty around the central estimate was set according to distributional information provided in the original source of the data as detailed in . Where distributional information was not available, the standard error was typically assumed to be 20%. For event rates and utility values, a beta distribution was used to restrict draws to the 0–1 range. For costs and resource use estimates, a gamma distribution was used to restrict draws to the 0–positive infinity range to prevent values of less than 0. For survival estimates, uncertainty was captured in the variance–covariance matrix of the parameter estimates and applied using the Cholesky decomposition approachCitation40. Baseline population characteristics (i.e. proportion of males and females and the mean age at baseline) were not assessed in sensitivity analysis, as the survival data estimations for the base case were adjusted for background mortality based on these baseline parameters. In the DSA, model parameters were varied individually with the parameters that had the greatest impact on the ICER presented in a tornado diagram. In the PSA, all selected parameters were varied at the same time over 1,000 simulations with results presented on a cost-effectiveness plane.
Scenario analysis
Scenario analyses were conducted to explore the impact of using alternative inputs on the model results. (1) An analysis which used standard PSM (i.e. without a mixture of cured and non-cured patients) to fit OS and PFS independently for both therapies; (2) An analysis using unadjusted ZUMA-1 data (naïve comparison) was conducted to assess the impact of the MAIC; (3) An analysis matching ZUMA-1 investigator assessed PFS data to tisa-cel JULIET PFS data (base case used centrally assessed PFS); (4) an analysis assuming equal acquisition costs for axi-cel and tisa-cel, with the acquisition costs for both treatments set to the list price of tisa-cel. A set of exploratory scenario analyses were also conducted to assess the impact of other key assumptions on model inputs including the discount rate, time horizon, time cut-off for assigning resource use and utility specific to long-term progression-free survivors and resource use of SCT and hospitalization for tisa-cel (see Supplementary Information Table 3 for full details of settings used).
Results
MAIC and survival curve selection
Following MAIC adjustment, median OS and PFS with axi-cel were almost reached: 50.009% patients were alive at the final cut-off (60-months), while 50.893% were progression free at the last data cut-off for PFS (24-months). A summary of the KM curves for axi-cel (ZUMA-1 unadjusted and adjusted) and tisa-cel (JULIET) for OS and PFS are presented in Supplementary Information Section 1, Figures 1 and 2, respectively).
In the base case analysis using the PS-MCM, the predicted long-term OS curve for axi-cel (with MAIC adjustment) was based on the exponential function, a Weibull model for axi-cel PFS, a log-normal model for tisa-cel OS, and a Generalized Gamma model for tisa-cel PFS. More details on visual and statistical model fit are provided in Supplementary Information Section 2.
Base case analysis
In the base case analysis compared with tisa-cel, axi-cel was associated with greater life years (LY; +3.89 undiscounted, +2.71 discounted), greater QALYs (+2.51 discounted) and higher costs ($50,185). This resulted in an ICER of $19,994 per QALY gained for axi-cel versus tisa-cel. With axi-cel treatment, costs were higher for drug acquisition, AE management, stem cell transplantation and pre-progression treatment. With tisa-cel treatment, costs were higher for hospitalization, post-progression treatment and terminal care costs ().
Table 2. Base case results.
Sensitivity analysis
presents a tornado plot of the top 10 model parameters that had the greatest impact on the cost-per QALY gained for axi-cel versus tisa-cel in DSA. The costs of axi-cel and tisa-cel had the greatest impacts on the ICER, followed by the proportion of axi-cel patients undergoing SCT and the cost of pre-progression care. In PSA axi-cel was cost-effective vs tisa-cel across 1,000 model simulations (see for the cost-effectiveness plane. The CEAC () showed that the cost per QALY gained for axi-cel versus tisa-cel was ≤$50,000 in 99.4% of simulations and ≤$33,500 in 99% of simulations.
Figure 1. Deterministic sensitivity analysis – tornado plot of parameter impact on cost per QALY gained. Negative values of cost per QALY gained from the tornado plot should be interpreted with caution as a negative cost per QALY gained may be caused by negative incremental costs (a good outcome) or negative incremental QALYs (a bad outcome). Abbreviations. AE, adverse event; ICU, intensive care unit; PFS, progression-free survival; QALY, quality-adjusted life year.
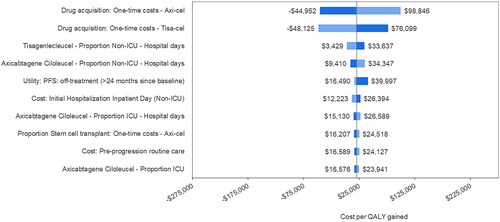
Scenarios
In all scenarios investigated, axi-cel remained cost-effective compared with tisa-cel (see Supplementary Information Tables 4 and 5 for a full breakdown of results), assuming a willingness-to -pay threshold of $150,000 per QALY gainedCitation41. When a standard PSM was used to generate survival curves, the ICER was $12,378. When the PS-MCM but without the MAIC adjusted OS and PFS curves, the ICER was $41,688. In a scenario using 60-month investigator assessed PFS data from ZUMA-1, the ICER was $42,635. When the acquisition costs of axi-cel and tisa-cel were assumed to be equal, axi-cel was the dominant treatment option. In additional scenarios conducted, axi-cel also remained cost-effective assuming a willingness-to-pay threshold of $100,000 per QALY gained. When the discount rate was varied, the ICER for axi-cel ranged from $13,380 to $28,235 per QALY gained. The ICER for axi-cel increased as the time horizon was shortened, ranging from $20,047 at 40 years to $78,609 at 5 years. Axi-cel remained cost-effective when it was conservatively assumed that long-term progression-free survivors start to experience a reduction in monthly routine care costs or improvements in utilities after 90 months (ICER $27,280/QALY). Axi-cel also remained cost-effective in scenarios where either SCT or hospitalization use was equivalent for axi-cel and tisa-cel.
Discussion
This analysis presents an update to a previously published model to assess the cost-effectiveness of axi-cel versus tisa-cel for treatment of r/r LBCL after ≥2 lines of systemic therapy, incorporating a more mature survival data cut for both axi-cel (60-month OS data) and tisa-cel (45-month OS and PFS data). The results of our model indicate that axi-cel is associated with 3.89 additional undiscounted LYs (2.71 discounted), and 2.51 additional discounted QALYs with increased costs of $19,994 compared with tisa-cel over a lifetime horizon. The model findings were robust to changes in key model assumptions as explored in scenario and sensitivity analyses. Scenarios analyses showed that axi-cel remained cost-effective when using the standard PSM, and when the PS-MCM was used without the MAIC adjustment. The base case analysis used centrally assessed axi-cel PFS data on the assumption that PFS was centrally assessed for tisa-cel in the JULIET trial (although this was not explicitly stated in the publication, the primary endpoint of best overall response rate was centrally assessedCitation12) However, the investigator assessed PFS data was available from ZUMA-1 up to 60 months and so these data were considered in a scenario. Axi-cel remained cost-effective versus tisa-cel with an ICER of $42,635 per QALY gained.
The previously published model, which was based on 39.1-month survival data for axi-cel and 33-month data for tisa-cel showed that axi-cel was dominant (i.e. associated with a greater QALY gain and lower costs) compared with tisa-celCitation14. In our analysis, axi-cel was associated with a more substantial incremental QALY gain than in the previous model (+2.51 vs +2.31 QALYs) but a higher incremental cost (+$19,994 vs −$1,407). The cost differences between the two models seem to be largely driven by the higher acquisition cost of axi-cel versus tisa-cel.
Our model benefits from the availability of more mature survival data for both axi-cel and tisa-cel than was available for the original model publication, which subsequently provides greater certainty in the cost-effectiveness conclusions.
The findings of our model are consistent with other published health economic analyses, which have demonstrated that axi-cel represents a cost-effective treatment option versus tisa-cel for the treatment patients with r/r LBCL who have previously received ≥2 lines of systemic therapy from the perspective of the Spanish NHS (ICER of €13,049/QALY gainedCitation42, the UK NHS (ICER dominant)Citation43 and the French health serviceCitation44. Furthermore, in addition to the clinical trial data which has been utilized in this model, there is a growing body of real-world clinical evidence which also demonstrates the significant clinical benefits of axi-cel versus tisa-cel for OS, PFS and response ratesCitation45,Citation46.
Our model has several strengths. The most up to date clinical data for both axi-cel and tisa-cel have been used to generate the most accurate results possible, and an effort was made to create a fair comparison between axi-cel and tisa-cel by adjusting the ZUMA-1 trial population via the MAIC. Furthermore, model assumptions were extensively tested in sensitivity analysis and scenarios. Additionally, a number of conservative assumptions were included. Several inputs for axi-cel such as hospitalization use, SCT rates, and AE rates were based on unadjusted ZUMA-1 data, assuming no differences before and after MAIC. As tisa-cel-treated patients from the JULIET trial had a better prognosis at baseline (compared with JULIET, ZUMA-1 had 25% more patients with refractory disease, 20% more patients with ≥4 prior therapies, 15% more patients with double/triple hit status, and 13% more patients with ECOG 1 at baseline)Citation13, using unadjusted ZUMA-1 data may have overestimated the resource use for axi-cel patients.
The model is associated with a number of limitations. A lack of head-to-head clinical data meant that this analysis relied on MAIC-adjusted OS and PFS for axi-cel (ZUMA-1 population reweighted to match JULIET trial) to compare long-term outcomes and costs of axi-cel vs tisa-cel. An MAIC is generally accepted by health technology assessment (HTA) agencies as a method of performing unanchored indirect treatment comparisons when an anchored comparison is not possibleCitation47. One general limitation of an MAIC is that only observed differences in patient characteristics can be adjusted. When an unanchored comparison is undertaken, it is desirable to adjust for differences in all prognostic factors and effect-modifiers, but there is rarely a complete set of such variables available for use in the analysis. To adjust for between-trial differences in patient characteristics likely to bias the relative treatment effect estimates of axi-cel versus tisa-cel, an MAIC was performed using individual patient data from ZUMA-1Citation13. It was feasible to adjust for the following differences in patient characteristics between trials: International Prognostic Index score, Eastern Cooperative Oncology Group score, relapsed versus refractory status, double/triple hit, stage of disease, number of prior therapies, and cell of origin. The MAIC weighting resulted in an effective sample size that was a 60% reduction from the actual sample size, which is a reflection of the magnitude of the overlap and differences in the matching covariatesCitation13. Individual patient data were not available from JULIET for this analysis and therefore patient-level propensity score matching could not be performed, representing a limitation of this analysis. Therefore, the MAIC results may be biased in the case that there are unobserved differences across trials. Partitioned survival models have been commonly used in other economic models of CAR T-cell therapies and have been accepted as appropriate for decision making by HTA bodies given their intuitiveness, and ease of constructionCitation14,Citation23,Citation48–50. Nevertheless, this approach is associated with limitations including its fundamental structural assumption that the survival endpoints are independent. This lack of a link between clinical endpoints also limits the degree to which the biological and clinical plausibility of extrapolations can be subject to scrutiny. In both PS-MCM and PSM approaches, the OS and PFS curves can only be fitted separately which could produce clinically implausible scenarios, such as PFS exceeding OS in the long term, or PFS being associated with a higher cure fraction than OS, as occurring in the base case analysis. To deal with this limitation, the PFS was set to be equal to the OS in circumstances where the PFS was predicted to be higher than the OS. Alternative model structures, such as state transition models, also require strong assumptions to be made, particularly in their use with CAR T-cell therapies where more complex structures (e.g. semi-Markov or patient-level models) may be required in order to appropriately reflect time dependencies (e.g. cure-points) within the modelling of event rates. A future modeling approach could consider a multistate Markov model with a mixture cure to model OS and PFS simultaneously as well as account for the potential cure effect of CAR T-cell therapies. Our model also did not consider disutilities associated with treatment-related AEs in line with the approach taken in previous publications of axi-cel modelsCitation14,Citation23. However, in an exploratory analysis where patients experiencing Grade 3 or 4 AEs were assigned a utility of 0 for the first week of treatment, the impact on the ICER was negligible (data not shown). Our model also did not consider post-progression salvage therapy costs due to a lack of publicly available data for tisa-cel. However, given that both treatments considered in this analysis are CAR T-cell therapies, there is not expected to be a substantial difference in the use of post-progression treatments, and therefore minimal impact on model findings. Another limitation is that the proportional hazards assumption appears to have been violated based on tests on the survival analysis and visual inspection of the Kaplan Meier curves. This limitation may introduce challenges regarding the validity of the treatment effect evaluations and potentially distorts the interpretation of the treatment effect over time. Future models may explore the use of flexible parametric survival models in order to overcome the violation of the proportional hazards assumption. A further limitation of the model is that it does not fully reflect the current treatment landscape for r/r LBCL. In 2022, the CAR T-cell therapy lisocabtagene maraleucel (liso-cel), was approved by the FDA for use in adult patients with r/r LBCL after ≥2 previous lines of systemic therapyCitation51. Two publications have reported the cost-effectiveness of axi-cel versus liso-cel from the perspective of a US payer, with one reporting axi-cel to be the most cost-effectiveCitation23, and one reporting liso-cel to be the most cost-effectiveCitation49. The contrasting results were due to differences in MAIC findings; notably, Parker et al. was based on one post hoc sensitivity analysis set of MAIC reported in Maloney et al. and omitted the number of prior treatment lines as a variableCitation52 whereas Oluwole et al. was based on the pre-specified MAIC base-case analysis set of Oluwole et al.; in this MAIC number of prior treatment lines was determined to be a key prognostic factorCitation53. Future economic model updates should also consider this additional therapy.
Robust relative clinical effectiveness and cost-effectiveness are essential to decision makers to obtain the best outcomes for patients and the best value for money for healthcare providers. This manuscript represents an important advancement in our understanding of the potential costs and benefits associated with axi-cel and tisa-cel for the treatment of LBCL due to the availability of more mature survival data than was available at the time of the original model publication, and as such provides greater certainty for decision makers in the cost-effectiveness estimates provided. This analysis also demonstrates that models using earlier data cuts can accurately predict future outcomes. As the inputs for this study were based on clinical trial data, the results should be interpreted in this context. However, there is evidence to suggest that our findings are applicable to the real-world setting. A systematic review and meta-analysis of observational CAR T-cell therapy studies has shown that axi-cel is associated with improved PFS and OS compared with tisa-cel, with a similar rate of Grade ≥3 AEsCitation45. Utilization of these data within a cost-effectiveness model showed that axi-cel continued to be cost-effective versus tisa-cel from a US payer perspective, with an ICER pf $50,947 per QALY gainedCitation54, thus supporting the findings of our clinical trial-based analysis. Certain event rates (for example, use of SCT, ICU admissions) observed in clinical trials may not accurately reflect those seen in real world clinical practice, and this is acknowledged as a limitation of this study. Further work is therefore needed to determine whether the use of and outcomes associated with axi-cel and tisa-cel in the real-world setting reflects that observed clinical trials and observational studies.
Conclusions
Over a lifetime horizon of 45 years, treatment of r/r LBCL with axi-cel was estimated to generate 2.71 additional life years and 2.51 additional QALYs, combined with an additional cost of $50,185 compared with tisa-cel. As such, axi-cel continues to be considered a cost-effective treatment option for patients with r/r LBCL who have previously received ≥2 lines of systemic therapy in the USA.
Transparency
Declaration of funding
Funding for this study was provided by Kite Pharma, a Gilead Company.
Reviewer disclosures
Peer reviewers on this manuscript have received an honorarium from JME for their review work but have no other relevant financial relationships to disclose.
Previous presentations
Results of an earlier version of the model were presented at the European Hematology Association (EHA) 2023 congress.
Results of a previous version of this model using an earlier clinical data cut have previously been published in Journal of Medical Economics (Rongzhe Liu, et al. (2021) Journal of Medical Economics, 24:1, 458-468, DOI: 10.1080/13696998.2021.1901721).
Supplemental Material
Download MS Word (521.4 KB)Acknowledgements
We thank Rongzhe Liu, Ibrahim Diakite and Marc F. Botteman (Pharmerit) who developed the original cost-effectiveness model, Julia Snider, Madhu Palivela and Jeff Shafiroff (Kite Pharma) who provided input on the analysis design and Helen Smethurst (Mtech Access) for medical writing services in preparation of the manuscript, all funded by consultancy payments from Kite Pharma.
Declaration of financial/other relationships
OOO has undertaken consultancy and advisory boards for: Pfizer, Kite, Gilead, AbbVie, Janssen, TGR therapeutics, ADC, Novartis, Epizyme, Curio science, Nektar, Cargo, Caribou and has received institution funding from: Kite, Pfizer, Daichi Sankyo, Allogene and honoraria from: Pfizer, Gilead. MDR is an employee of Kite Pharma, who funded the study. ND, RB and CJ are employees of Mtech Access, who have received consultancy fees for the development of the economic model and manuscript. FLL performed a consulting/advisory role for Allogene, Amgen, Bluebird Bio, Bristol-Myers Squibb, Calibr, Cellular Biomedicine Group, Cowen, ecoR1, Emerging Therapy Solutions Gerson Lehman Group, GammaDelta Therapeutics, Iovance, Janssen, Kite, a Gilead Company, Legend Biotech, Novartis, Umoja, and Wugen; received research funding from Allogene, Kite, and Novartis; and holds patents, royalties, other intellectual property from several patents held by the institution in author’s name (unlicensed) in the field of cellular immunotherapy.
References
- Sehn LH, Gascoyne RD. Diffuse large B-cell lymphoma: optimizing outcome in the context of clinical and biologic heterogeneity. Blood. 2015;125(1):22–32. doi: 10.1182/blood-2014-05-577189.
- Dunleavy K, Wilson WH. Primary mediastinal B-cell lymphoma and mediastinal gray zone lymphoma: do they require a unique therapeutic approach? Blood. 2015;125(1):33–39. doi: 10.1182/blood-2014-05-575092.
- Casulo C, Burack WR, Friedberg JW. Transformed follicular non-Hodgkin lymphoma. Blood. 2015;125(1):40–47. doi: 10.1182/blood-2014-04-516815.
- American Cancer Society. About non-Hodgkin lymphoma. 2022.
- Coiffier B, Sarkozy C. Diffuse large B-cell lymphoma: r -CHOP failure-what to do? Hematology Am Soc Hematol Educ Program. 2016;2016(1):366–378. doi: 10.1182/asheducation-2016.1.366.
- Gisselbrecht C, Schmitz N, Mounier N, et al. Rituximab maintenance therapy after autologous stem-cell transplantation in patients with relapsed CD20(+) diffuse large B-cell lymphoma: final analysis of the collaborative trial in relapsed aggressive lymphoma. J Clin Oncol. 2012;30(36):4462–4469. doi: 10.1200/JCO.2012.41.9416.
- Crump M, Neelapu SS, Farooq U, et al. Outcomes in refractory diffuse large B-cell lymphoma: results from the international SCHOLAR-1 study. Blood. 2017;130(16):1800–1808. doi: 10.1182/blood-2017-03-769620.
- Vitale C, Strati P. CAR T-cell therapy for B-cell non-Hodgkin lymphoma and chronic lymphocytic leukemia: clinical trials and real-world experiences. Front Oncol. 2020;10:849. doi: 10.3389/fonc.2020.00849.
- Food and Drug Administration. YESCARTA® (axicabtagene ciloleucel) suspension for intravenous infusion. 2017.
- Food and Drug Administration. KYMRIAH® (tisagenlecleucel) suspension for intravenous infusion. 2017.
- Neelapu SS, Locke FL, Bartlett NL, et al. Axicabtagene ciloleucel CAR T-cell therapy in refractory large B-cell lymphoma. N Engl J Med. 2017;377(26):2531–2544. doi: 10.1056/NEJMoa1707447.
- Schuster SJ, Bishop MR, Tam CS, et al. Tisagenlecleucel in adult relapsed or refractory diffuse large B-cell lymphoma. N Engl J Med. 2019;380(1):45–56. doi: 10.1056/NEJMoa1804980.
- Oluwole OO, Jansen JP, Lin VW, et al. Comparing efficacy, safety, and preinfusion period of axicabtagene ciloleucel versus tisagenlecleucel in relapsed/refractory large B cell lymphoma. Biol Blood Marrow Transplant. 2020;6(9):1581–1588. doi: 10.1016/j.bbmt.2020.06.008.
- Liu R, Oluwole OO, Diakite I, et al. Cost effectiveness of axicabtagene ciloleucel versus tisagenlecleucel for adult patients with relapsed or refractory large B-cell lymphoma after two or more lines of systemic therapy in the United States. J Med Econ. 2021;24(1):458–468. doi: 10.1080/13696998.2021.1901721.
- Schuster SJ, Tam CS, Borchmann P, et al. Long-term clinical outcomes of tisagenlecleucel in patients with relapsed or refractory aggressive B-cell lymphomas (JULIET): a multicentre, open-label, single-arm, phase 2 study. Lancet Oncol. 2021;22(10):1403–1415. doi: 10.1016/s1470-2045(21)00375-2.
- Neelapu SS, Jacobson CA, Ghobadi A, et al. 5-Year follow-up supports curative potential of axicabtagene ciloleucel in refractory large B-cell lymphoma (ZUMA-1). Blood. 2023;141(19):2307–2315. doi: 10.1182/blood.2022018893.
- Institute for Clinical and Economic Review. ICER's reference case for economic evaluations: principles and rationale. 2020.
- Locke FL, Ghobadi A, Jacobson CA, et al. Long-term safety and activity of axicabtagene ciloleucel in refractory large B-cell lymphoma (ZUMA-1): a single-arm, multicentre, phase 1-2 trial. Lancet Oncol. 2019;20(1):31–42. doi: 10.1016/S1470-2045(18)30864-7.
- Lambert PC, Thompson JR, Weston CL, et al. Estimating and modeling the cure fraction in population-based cancer survival analysis. Biostatistics. 2007;8(3):576–594. doi: 10.1093/biostatistics/kxl030.
- Lambert PC. Modeling of the cure fraction in survival studies. Stata J. 2007;7(3):351–375. doi: 10.1177/1536867X0700700304.
- Centers for Disease Control and Prevention. NCHS – National vital statistics system. mortality: life tables US 2020. 2020 [cited 2020 Nov]. https://ftpcdcgov/pub/Health_Statistics/NCHS/Publications/NVSR/67_07/(Table02 males, Table 03 females)
- Roth JA, Sullivan SD, Lin VW, et al. Cost-effectiveness of axicabtagene ciloleucel for adult patients with relapsed or refractory large B-cell lymphoma in the United States. J Med Econ. 2018;21(12):1238–1245. doi: 10.1080/13696998.2018.1529674.
- Oluwole OO, Liu R, Diakite I, et al. Cost-effectiveness of axicabtagene ciloleucel versus lisocabtagene maraleucel for adult patients with relapsed or refractory large B-cell lymphoma after two or more lines of systemic therapy in the US. J Med Econ. 2022;25(1):541–551. doi: 10.1080/13696998.2022.2065787.
- Yang H, Hao Y, Chai X, et al. Estimation of total costs in patients with relapsed or refractory diffuse large B-cell lymphoma receiving tisagenlecleucel from a US hospital’s perspective. J Med Econ. 2020;23(9):1016–1024. doi: 10.1080/13696998.2020.1769109.
- Canadian Agency for Drugs and Technologies in Health. Tisagenlecleucel for diffuse large B-cell lymphoma: economic review report. Optimal Use Report. 2019 [cited 2019 Oct 25]. https://www.cadth.ca/sites/default/files/pdf/car-t/op0538-tisagenlecleucel-economic-report-DLBCL-jan2019.pdf.
- Lin VW, Jiang Y, Chuang LH, et al. Health utilities for patients with relapsed or refractory large B-cell lymphoma (R/R-LBCL): ad hoc analysis from an axicabtagene ciloleucel (axi-cel) safety management study. 44th Annual Meeting of the European Society for Blood and Marrow Transplantation; Lisbon, Portugal. 2018.
- Chen Q, Staton AD, Ayer T, et al. Exploring the potential cost-effectiveness of precision medicine treatment strategies for diffuse large B-cell lymphoma. Leuk Lymphoma. 2018;59(7):1700–1709. doi: 10.1080/10428194.2017.1390230.
- IBM Micromedex(a). RED BOOK ® online. 2023 [cited 2023 Nov 3]. www.ibm.com/products/micromedex-red-book.
- IBM Micromedex(b). RED BOOK® online. 2022 [cited 2022 Oct 12]. www.ibm.com/products/micromedex-red-book.
- Centers for Medicare & Medicaid Services. Physician fee schedule. 2022 [cited 2022 Nov 28]. https://www.cms.gov/apps/physician-fee-schedule/search/search-criteria.aspx.
- CMS – Centers for Medicare & Medicaid Services. CMS medicare fee schedule. 2022. https://www.cms.gov/Medicare/Medicare-Fee-for-Service-Payment/FeeScheduleGenInfo/index.html.
- Bureau of Labor Statistics. Consumer price index. Medical care component. 2022 [cited 2022 Nov 28]. https://data.bls.gov/timeseries/CUUR0000SAM?output_view=data.
- IBM Micromedex(c). RED BOOK® online. 2019 [cited 2019 Dec 3]. www.ibm.com/products/micromedex-red-book.
- Broder MS, Quock TP, Chang E, et al. The cost of hematopoietic stem-cell transplantation in the United States. Am Health Drug Benefits. 2017;10(7):366–374.
- Wakase S, Teshima T, Zhang J, et al. Cost effectiveness analysis of tisagenlecleucel for the treatment of adult patients with relapsed or refractory diffuse large B cell lymphoma in Japan. Transplant Cell Ther. 2021;27(6):506 e1-506–e10. doi: 10.1016/j.jtct.2021.03.005.
- Agency for Healthcare Research and Quality. Healthcare cost and utilization project (HCUP) 2018 [cited 2022 Nov 28]. https://hcupnet.ahrq.gov.
- Gershengorn HB, Garland A, Gong MN. Patterns of daily costs differ for medical and surgical intensive care unit patients. Ann Am Thorac Soc. 2015;12(12):1831–1836. doi: 10.1513/AnnalsATS.201506-366BC.
- Huntington SF, Svoboda J, Doshi JA. Cost-effectiveness analysis of routine surveillance imaging of patients with diffuse large B-cell lymphoma in first remission. J Clin Oncol. 2015;33(13):1467–1474. doi: 10.1200/JCO.2014.58.5729.
- Kutikova L, Bowman L, Chang S, et al. Medical costs associated with non-Hodgkin’s lymphoma in the United States during the first two years of treatment. Leuk Lymphoma. 2006;47(8):1535–1544. doi: 10.1080/10428190600573325.
- Briggs A, Sculpher M, Claxton K. Decision modelling for health economic evaluation. Oxford: Press OU; 2006.
- Institute for Clinical and Economic Review. 2020–2023 value assessment framework. 2020.
- Bastos-Oreiro M, de Las Heras A, Presa M, et al. Cost-Effectiveness analysis of axicabtagene ciloleucel vs. Tisagenlecleucel for the management of relapsed/refractory diffuse large B-cell lymphoma in Spain. Cancers. 2022;14(3):538. doi: 10.3390/cancers14030538.
- Brighton N, Lovato E, Castaigne JG. Assessing the cost-effectiveness of axicabtagene ciloleucel compared with other CAR T-cell therapies in the treatment of diffuse large B-cell lymphoma in the UK. Value Health. 2022;25(12): S141. doi: 10.1016/j.jval.2022.09.683.
- Brighton N, Zang A, Castaigne JG. Cost-effectiveness of axicabtagene ciloleucel versus other CAR T-cell therapies in the treatment of diffuse large B-cell lymphoma in France. Value Health. 2022;25(12):S81. doi: 10.1016/j.jval.2022.09.398.
- Jacobson CA, Munoz J, Sun F, et al. Effectiveness and safety of axicabtagene-ciloleucel and tisagenlecleucel for large B-cell lymphoma in the real-world setting: a systematic review and meta-analysis. Poster presented at EBMT-EHA 5th European CAR T-cell meeting February 2023. 2023.
- Bachy E, Le Gouill S, Di Blasi R, et al. A real-world comparison of tisagenlecleucel and axicabtagene ciloleucel CAR T cells in relapsed or refractory diffuse large B cell lymphoma. Nat Med. 2022;28(10):2145–2154. doi: 10.1038/s41591-022-01969-y.
- Phillippo DM, Ades AE, Dias S, et al. NICE DSU technical support document 18: methods for population-adjusted indirect comparisons in submission to NICE. 2016 [cited 2023 Dec]. http://wwwnicedsuorguk.
- National Institute for Health and Care Excellence. Axicabtagene ciloleucel for treating diffuse large B-cell lymphoma and primary mediastinal large B-cell lymphoma after 2 or more systemic therapies. NICE TA872. 2023 [cited 2023 Dec]. https://wwwniceorguk/guidance/ta872/resources/axicabtagene-ciloleucel-for-treating-diffuse-large-bcell-lymphoma-and-primary-mediastinal-large-bcell-lymphoma-after-2-or-more-systemic-therapies-pdf-82613669792965
- Parker C, Liu FF, Deger KA, et al. Cost-Effectiveness of lisocabtagene maraleucel versus axicabtagene ciloleucel and tisagenlecleucel in the third-line or later treatment setting for relapsed or refractory large B-cell lymphoma in the United States. Adv Ther. 2023;40(5):2355–2374. doi: 10.1007/s12325-023-02444-x.
- Canadian Agency for Drugs and Technologies in Health. Axicabtagene ciloleucel for large B-cell lymphoma: economic review report. CADTH optimal use report: vol 9, no 1d. 2019 [cited 2023 Dec]. https://www.cadth.ca/sites/default/files/pdf/car-t/ct0002-axicabtagene-ciloleucel-economic-report-redacted.pdf
- Food and Drug Administration. BREYANZI®(lisocabtagene maraleucel) suspension for intravenous infusion. 2022.
- Maloney DG, Kuruvilla J, Liu FF, et al. Matching-adjusted indirect treatment comparison of liso-cel versus axi-cel in relapsed or refractory large B cell lymphoma. J Hematol Oncol. 2021;14(1):140. doi: 10.1186/s13045-021-01144-9.
- Oluwole OO, Chen JMH, Chan K, et al. ABCL-289: matching-adjusted indirect comparison (MAIC) of axicabtagene ciloleucel (axi-cel) and lisocabtagene maraleucel (liso-cel) in relapsed or refractory (R/R) large B-cell lymphoma (LBCL) after two or more prior lines of therapy. Clin Lymphoma Myeloma Leuk. 2021;21:S388. doi: 10.1016/S2152-2650(21)01882-6.
- Locke FL, Ray MD, Bradford R, et al. Cost effectiveness of axicabtagene ciloleucel versus tisagenlecleucel in 3L + relapsed/refractory large b-cell lymphoma in the United States: utilizing real-world evidence of CAR T-cell therapies. Presented at ISPOR Europe 2023. Copenhagen, Denmark. 2023.