Abstract
Aims
This systematic literature review (SLR) consolidated economic and healthcare resource utilization (HCRU) evidence for positron emission tomography (PET) and single-photon emission computed tomography (SPECT) myocardial perfusion imaging (MPI) to inform future economic evaluations.
Materials and methods
An electronic search was conducted in MEDLINE, Embase, and Cochrane databases from 2012–2022. Economic and HCRU studies in adults who underwent PET- or SPECT-MPI for coronary artery disease (CAD) diagnosis were eligible. A qualitative methodological assessment of existing economic evaluations, HCRU, and downstream cardiac outcomes was completed. Exploratory meta-analyses of clinical outcomes were performed.
Results
The search yielded 13,439 results, with 71 records included. Economic evaluations and comparative clinical trials were limited in number and outcome types (HCRU, downstream cardiac outcomes, and diagnostic performance) assessed. No studies included all outcome types and only one economic evaluation linked diagnostic performance to HCRU. The meta-analyses of comparative studies demonstrated significantly higher rates of early- and late-invasive coronary angiography and revascularization for PET- compared to SPECT-MPI; however, the rate of repeat testing was lower with PET-MPI. The rate of acute myocardial infarction was lower, albeit non-significant with PET- vs. SPECT-MPI.
Limitations and conclusions
This SLR identified economic and HCRU evaluations following PET- and SPECT-MPI for CAD diagnosis and determined that existing studies do not capture all pertinent outcome parameters or link diagnostic performance to downstream HCRU and cardiac outcomes, thus, resulting in simplified assessments of CAD burden. A limitation of this work relates to heterogeneity in study designs, patient populations, and follow-up times of existing studies. Resultingly, it was challenging to pool data in meta-analyses. Overall, this work provides a foundation for the development of comprehensive economic models for PET- and SPECT-MPI in CAD diagnosis, which should link diagnostic outcomes to HCRU and downstream cardiac events to capture the full CAD scope.
Introduction
Coronary artery disease (CAD) represents a significant burden on healthcare systems globally, leading to substantial morbidity and mortalityCitation1–3. CAD is associated with downstream cardiovascular events, extensive testing, and revascularization procedures, resulting in a substantial economic strain on healthcare systemsCitation1,Citation4,Citation5. The incidence of CAD is expected to rise further due to the increasing prevalence of risk factors, including diabetes and obesityCitation3.
The significant burden of CAD emphasizes the need for accurate and timely CAD diagnosis; however, there are several challenges in the diagnostic pathway such as suboptimal test accuracy and lack of access to novel technologiesCitation6. False negative (FN) and inconclusive results from cardiac diagnostic testing are common and result in missed diagnoses, subsequent cardiovascular events, hospitalization, and mortality. False positive (FP) results often require additional and sometimes unnecessary diagnostic follow-up testing and therapeutic invasive imaging, thereby escalating healthcare expensesCitation7–11.
An additional confounding challenge in diagnosing CAD is associated with the fact that current CAD screening and diagnosis professional guidelines are inconsistent, especially in difficult-to-image populations, including women and patients with obesity or diabetesCitation12–15. Accurately diagnosing CAD in these specific patient subgroups is often challenging due to patients’ anatomy and the mechanisms of these underlying diseasesCitation2,Citation16,Citation17. For example, coronary microvascular disease commonly occurs in female, diabetic, and obese patientsCitation18–23; however, traditional semi-quantitative perfusion diagnostic tests may not accurately capture the condition as a result of poor image qualityCitation24–26. More specifically, women with large breasts and obese individuals experience diminished diagnostic sensitivity due to anterior wall attenuation artifacts, consequently increasing the risk of a FP diagnosisCitation19. A large proportion (52%) of all diagnostic scans occur in the aforementioned subpopulations (women and patients with obesity or diabetes). Therefore, ensuring accurate diagnosis of these high-risk subgroups is imperative in facilitating timely and effective healthcareCitation27.
While various diagnostic testing options are available, nuclear-based myocardial perfusion imaging (MPI) modalities, such as single-photon emission computed tomography (SPECT) and positron emission tomography (PET), currently serve as gatekeepers for invasive coronary angiography (ICA), the standard of care for diagnosing CADCitation28,Citation29. SPECT is the most commonly used MPI modalityCitation30; however, it has limitationsCitation6,Citation30. PET-MPI has emerged as an alternative due to its superior image qualityCitation31,Citation32, improved attenuation correction (AC)Citation33, lower radiation exposureCitation34, better myocardial extractionCitation31, and the ability to measure myocardial blood flow and coronary reserve flowCitation31,Citation35,Citation36. Using PET-MPI to diagnose CAD leads to more accurate prediction of cardiac events, and better risk stratification in patients with suspected CADCitation21,Citation25,Citation37–39.
Several clinical trials and large-scale meta-analyses have demonstrated improved diagnostic (i.e. sensitivity and specificity) and prognostic performance of PET- compared to SPECT-MPI in the general populationCitation40–47. Improved sensitivity and specificity have also been observed in a head-to-head clinical trial of PET- compared to SPECT-MPI in subpopulations including women and patients with obesity and diabetesCitation47. Despite this evidence, PET-MPI use is currently limited due to low availability of PET machines, incongruent guidelines, reimbursement challenges, and a lack of evidence on the economic benefits compared to SPECT-MPICitation38,Citation48,Citation49.
To date, no published systematic literature reviews (SLR) or meta-analyses have collated evidence on healthcare resource utilization (HCRU) and downstream clinical outcomes following PET- and SPECT-MPI use or captured economic evaluations for PET- compared to SPECT-MPI for diagnosing CAD. This SLR aimed to consolidate and analyze existing economic evaluations and reports of HCRU and downstream cardiac outcomes following PET- and SPECT-MPI in CAD. Specific objectives included a qualitative assessment of parameters and limitations of existing economic evaluations, as well as a comprehensive appraisal of comparative and single-arm trials reporting on HCRU and downstream cardiac outcomes to inform the development of future health economic evaluations of MPI in CAD.
Methods
Search strategy
A multiphase search strategy was conducted on 2 November 2022, and included searches of both electronic databases and manual references. The database search was performed using the Ovid search interface and included: MEDLINE, Embase, Cochrane CENTRAL, and Cochrane Database of Systematic Reviews. The search strategy was designed by an experienced information specialist and independently peer-reviewed by a second information specialist using the Peer Review for Electronic Search Strategies toolCitation50. The search was restricted to studies conducted in adults, humans, and written in the English language. See Supplementary Appendix 1 for a full description of the search strategy.
To ensure that all relevant studies were included, a manual search of the bibliographies of relevant SLRs, meta-analyses, and network meta-analyses identified through the database search was performed. To further complement bibliography searches, an additional targeted search was conducted in PubMed in March 2023 to confirm all relevant articles had been captured. For economic evaluations, any additional relevant studies identified during the bibliographic search of on-topic SLRs were included irrespective of their publication date to ensure that all available PET-MPI economic studies were captured and appraised.
Selection criteria and data extraction process
Studies were eligible for inclusion based on criteria established a priori ()Citation51. Articles meeting the following criteria were included: (a) Studies conducted in adults with suspected and/or confirmed CAD; (b) Single-arm and/or comparative studies or economic evaluations involving the utilization of PET- and/or SPECT-MPI; (c) Studies comprising a minimum of 30 patients each; (d) Studies conducted in countries with comparable availability and utilization patterns of PET- and SPECT MPI technology; and (e) Articles published from 2012 to the present. Exclusion criteria included: (a) Narrative review articles, case study reports, opinion pieces, or conference abstracts; (b) Animal or phantom studies; and (c) Studies focusing on SPECT- or PET-MPI without addressing HCRU, downstream CAD events, or economics.
Table 1. Description of eligibility criteria.
The study selection process was conducted using DistillerSR (Ottawa, Canada) SLR software in two levelsCitation52. Level one included screening the titles and abstracts of records retrieved from the initial database search for relevance by two independent reviewers to combat individual inclusion bias. Level two consisted of reviewing the full texts of studies deemed potentially relevant in duplicate. Any discrepancies or conflicts between the reviewers regarding record inclusion were resolved by a third impartial reviewer.
Data extraction was performed using a standardized Microsoft Excel template by a single reviewer and validated by a second reviewer. General study information (e.g. reference identification, country/region of study), key patient baseline characteristics (e.g. age, sex, prevalence of patients with suspected or confirmed CAD, body mass index [BMI], obesity, hypertension, dyslipidemia, diabetes, current or history of smoking), study design, diagnostic modality, and study protocol details were extracted from the included studies. In addition, outcomes of interest: (a) Any economic outcomes (e.g. analysis type [cost-effectiveness analyses [CEA], budget impact models, observational utilization], duration/time horizon, costs, quality adjusted life years) from economic evaluations of PET-MPI; (b) HCRU (coronary angiography [CA], coronary revascularization, repeat testing, and hospitalization rates); and (c) Downstream cardiac outcomes (myocardial infarction [MI] events, major adverse cardiovascular events [MACE], and cardiac death [CD]) were also extracted. Early CA referrals and revascularization were deemed to occur within 3 months post-MPI, while late CA and revascularization occurred after 3 months and within the study follow-up period. Revascularization procedures included percutaneous coronary intervention (PCI) and coronary artery bypass graft (CABG). Acute MI referred to reported nonfatal heart attacks occurring post-MPI. MACE encompassed any reported composite endpoints of MI, and CD or all-cause mortality, stroke, unstable anginal or hospitalization due to heart failure. Repeat testing referred to additional non-invasive imaging procedures following an initial diagnostic test, often conducted during subsequent evaluations or follow-up visits.
Statistical analysis
Meta-analyses were conducted using R (Version 4.0.5, “meta” package). For comparative studies, meta-analyses were performed by pooling hazards ratios of PET- and SPECT-MPI across studies and calculating the 95% confidence interval with the inverse variance method to evaluate HCRU and downstream cardiac event outcomes. Considering the limited number of head-to-head studies, a fixed-effects model was utilized to reduce the uncertainty that could be introduced with a random-effects model by inaccurately estimating between-study variance under a small sample size.
Additionally, single-arm meta-analyses were conducted to estimate the pooled rates of early and late CA and revascularization rates, MI, and CD for overall and categorized suspected CAD (<25%, 25–75%, >75%). Univariable meta-regression with random effects was performed to assess the impact of suspected CAD status on study outcomes. To mitigate the high variability in follow-up time across studies, logistic regression was used, utilizing the complementary log-log link function. By applying logistic regression, hazard estimates were obtained and utilized in the meta-analysis and meta-regression analyses. This standardized approach enabled reliable comparisons and combined analysis of data from different studies, regardless of follow-up durations. Bubble plots were generated to show the association between suspected CAD status and HCRU and event rates after adjustment for follow-up time. Studies with data points lying far from the regression line were considered outliers. When considering MI, late revascularization and late ICA, outliers were determined based on visual inspection, whereas for early revascularization and ICA, outliers were considered if the value was above quartile 3 + 1.5 interquartile range (IQR) or below quartile 1–1.5 IQR.
Results
Search results
The search yielded 13,439 results. Of this, 791 full texts were assessed for eligibility, resulting in 727 excluded records and 64 included records. Additionally, seven records were included through citation searching and targeted searches for a total of 71 included records. The results of the systematic search and study selection process are summarized in .
Economic evaluations
Eight PET-MPI economic evaluations were identified. Parameters and limitations of these evaluations were qualitatively assessed with significant variability observed across studies. Six records included both PET- and SPECT-MPICitation53–58 and two records included PET-MPI onlyCitation59,Citation60. Four economic evaluations were published within the past decade, while the remaining four were published between 17 and 28 years ago. Five of the evaluations were CEACitation55–58,Citation60 and the remaining three were observational or registry evaluations encompassing HCRU, cardiac outcomes, and associated costsCitation53,Citation54,Citation59.
The economic evaluations mainly focus on a general CAD patient population, with only two publicationsCitation56,Citation57 providing women subgroup data. Time horizons ranged from 4-weeks to 30-years. There was also considerable variation in the types of parameters included in the evaluations. Few evaluations included both short-term (e.g. diagnostic characteristics, early HCRU) and long-term (e.g. MI events) outcomes. Two reports include HCRU onlyCitation59,Citation60, two reports include both HCRU and downstream cardiac outcomesCitation53,Citation54, and three records include both HCRU and diagnostic performanceCitation55,Citation57,Citation58. Only one record linked diagnostic performance to HCRU, but it did not establish a connection between diagnostics and downstream cardiac outcomesCitation56. This heterogeneity in model structure and included parameters may drive the differences in costing results, where some studies demonstrate lower costs with PET-MPI vs. SPECT-MPI useCitation54,Citation57,Citation58,Citation60 and others report higher costsCitation53,Citation55,Citation56,Citation59. Detailed characteristics and outcomes of the economic evaluations are presented in .
Table 2. Common characteristics and variability of included economic studies.
Comparative studies
Six comparative PET- and SPECT-MPI studies were included, with studies demonstrating variability in reported outcomes (). Two studies reported HCRU and cardiac outcomes in PET- compared to SPECT-MPICitation53,Citation61 and two studies evaluated downstream HCRU only between the two MPI modalitiesCitation62,Citation63. Only one study reported diagnostic performance and downstream HCRU for PET- vs. SPECT-MPICitation58. No comparative studies reported all three outcomes. Mimouni et al.Citation58 was the only comparative study reporting on the diagnostic performance of PET- and SPECT-MPI. The results demonstrated that PET-MPI had a significantly higher diagnostic accuracy compared to SPECT-MPI (.87 vs. .78; p = .048), which correlated with increased utilization of ICA procedures.
summarizes key characteristics in each comparative study, and the reported rates of clinical HCRU and cardiac outcomes. The total population sample across studies consisted of 88,371 patients who underwent PET-MPI and 1,945,625 patients who underwent SPECT-MPI. Patel et al.Citation61 was the only study that included an 100% confirmed CAD population. Conversely, two studies limited their inclusion criteria to patients with suspected CADCitation53,Citation58. The remaining studies had a mixed population of known or suspected CAD. Female patients comprised 37–69% of the total PET-MPI population and 33–69% of the total SPECT-MPI population. The mean age of patients ranged between 59–66 years old. Diabetes was prevalent in 14–77% of patients. Most patients also exhibited moderate to high mean BMI (28.8–31.8 kg/m2) or were classified with obesity.
Table 3. Characteristics of PET- vs. SPECT-MPI studies and outcomes.
The meta-analysis of comparative studies demonstrated that the rate of early and late ICA, as well as revascularization, was significantly higher for PET- compared to SPECT-MPI; however, the rate of repeat testing was significantly lower with PET-MPI. The rate of acute MI was lower, albeit non-significant, with PET- vs. SPECT-MPI ().
Table 4. Meta-analysis of PET- vs. SPECT-MPI studies.
Single-arm studies
A total of 61 single-arm studies were included. Of these, 62% were SPECT-MPICitation64–100 and 38% were PET-MPI studiesCitation21,Citation25,Citation59,Citation61,Citation101–119. Population baseline characteristics were highly heterogeneous across the included studies. Twenty studies specifically limited their inclusion criteria to suspected CAD patientsCitation25,Citation59,Citation67,Citation74–77,Citation83,Citation87,Citation92,Citation94–96,Citation100,Citation101,Citation107,Citation109–111,Citation117. Conversely, the remaining studies included a mixed patient population with confirmed or suspected CAD. The proportion of female patients ranged from 3% to 100%. The patients also exhibited various CAD comorbidities, including diabetes and obesity. Follow-up time varied significantly, ranging from 2–102 months. Supplementary Table S1 summarizes the key patient characteristics and outcomes of each single-arm study.
depicts rates of HCRU and CAD events in each study by the proportion of patients with suspected CAD, where ‘0′ indicates 0% suspected CAD and ‘1′ indicates 100% suspected CAD. Significant outliers have been excluded from the analysis. HCRU increases with increasing prevalence of confirmed CAD in the population in patients imaged with PET- or SPECT-MPI. See Supplementary Figure S1 for plots with included outlier studies.
Figure 3. Annualized rates of HCRU and CAD events adjusted for time in single-arm MPI studies (excluding outlier studies).
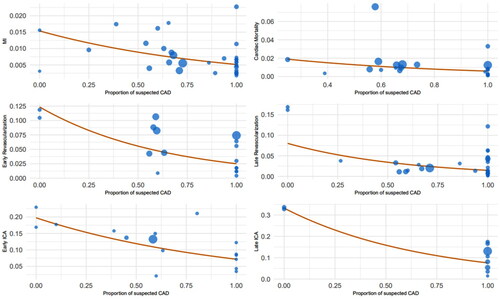
Pooled rates which controlled for variability in follow-up time and suspected CAD status (i.e. 0–100%) are presented in excluding outlier studies and Supplementary Table S2, including outlier studies. Findings demonstrated that studies comprising over a 75% suspected CAD population exhibited significantly lower annualized rates of HCRU and cardiac events compared to studies with a population less than 25% suspected CAD. These findings are independent of the exclusion of outliers. Annualized rates were 7.7% and 7.3% for early and late ICA, 2.4% and 1.6% for early and late revascularization, and 0.5% for cardiac death for the >75% suspected CAD subgroup compared to annualized rates of 17.3% and 15.2% for early and late ICA, and 10.6% and 15.2% for early and late revascularization in the <25% suspected CAD subgroup. In all patients, regardless of suspected CAD status, the pooled HCRU and CAD event annualized rates were 9.8% and 9.6% for early and late ICA, 3.7% and 2% for early and late revascularization, 0.6% for MI, and 0.8% for cardiac death.
Table 5. Pooled annualized rates (%) of HCRU and CAD events across MPI studies adjusted for time (excluding outlier studies).
Discussion
This SLR sought to identify and consolidate existing economic evaluations and investigations of HCRU and downstream cardiac outcomes following PET- and SPECT-MPI for CAD diagnosis, addressing a key evidence gap. The economic and clinical evaluations reviewed in this SLR do not capture all pertinent outcome parameters (i.e. diagnostic performance, HCRU, and cardiac events) or link diagnostic performance to downstream HCRU and cardiac outcomes, thus, resulting in simplified assessments of CAD burden. This work builds a framework to inform the development of future economic evaluations of PET- and SPECT-MPI and yields HCRU and cardiac event estimates that can be used in future economic models.
Economic evaluations
The existing economic evaluations for PET-MPI are limited in number, are heterogeneous, and possess several design limitations that hinder consensus for comparative cost effectiveness and affordability of PET- relative to SPECT-MPI. Firstly, the economic evaluation time horizons are highly variable, and often the follow-up time is short (up to 1-year). Several of the economic evaluations did not include downstream cardiac outcomes, which may be due to the short time horizons that are insufficient to capture the occurrence of downstream health outcomes. Additionally, PET-MPI has been shown to have a higher rate of early referrals to CA and revascularizations which could be attributed to the higher sensitivity and specificity of PET- compared to SPECT-MPICitation57. As a result, evaluations that are short and only capture early HCRU may yield higher incurred costs for PET- than SPECT-MPI; however, they are not representative of the entire resource utilization landscape.
Although a few of the economic evaluations used diagnostic performance parameters to inform costCitation55,Citation57,Citation58, only one evaluation published over two decades ago included assumptions directly linking diagnostic performance to HCRU and cardiac eventsCitation56. Garber and SolomonCitation56 linked true positive (TP) results to necessary CA, true negative (TN) results to the appropriate avoidance of CA, FN to conservative management, and FP to unnecessary CA. However, they did not consider the downstream cardiac events that may occur from a FN test result and associated costs. Garber and SolomonCitation56 also relied on sensitivity and specificity values derived from published literature, which may be influenced by publication bias. Similar challenges have been observed in other economic evaluations for diagnostic tests because of the indirect nature of the diagnostic testing impact on patient outcomesCitation120. Similarly, simplified methods to estimate diagnostic test accuracy are often used to inform economic models, which may present challenges for decision makersCitation121,Citation122. The variable nature of parameters included in existing analyses emphasizes the need for a comprehensive economic model that links diagnostic performance to downstream health outcomes.
Another crucial aspect to consider in economic evaluations is the selection of appropriate cost parameters. Economic analyses that only included modality costs tended to favor SPECT- over PET-MPI due to the omission of pivotal high-cost components (i.e. HCRU and cardiac events)Citation53,Citation55,Citation56. However, in the most recent economic model developed by Mimouni et al.Citation58, several cost parameters, including human resources, materials (supplies and drugs), intervention rooms, and technician and physician time were considered. The inclusion of personnel time and intervention room costing increases the cost of 2-day SPECT-MPI procedures, which is not considered in most economic evaluations. This variability poses a substantial challenge when attempting to conduct a comprehensive cost-effectiveness comparison between PET- and SPECT-MPI, comparing economic results of existing evaluations, and trying to apply results to other economic landscapes.
Of note, the existing economic evaluations focus only on a general CAD population and lack investigations into subpopulations with diagnostic imaging challenges and a high CAD burden such as female, obese, and diabetic patientsCitation19,Citation24,Citation25,Citation37. It is suspected that there will be differences in HCRU, clinical event occurrence, and costs among these subpopulations. Additionally, as the prevalence of diabetes and obesity continue to rise, consideration of these high-risk subpopulations will be imperative to accurately realize these diagnoses, otherwise an increase in adverse clinical outcomes will further contribute to an increase in HCRU.
Furthermore, the lack of recently published economic analyses for PET-MPI underscores the need for new comprehensive economic models. PET- and SPECT-MPI technology has changed overtime; therefore, economic models published between 17–28 years ago may not accurately reflect the costs of current technology. Utilizing publications that are over two decades old to inform current health care decisions is suboptimal and reinforces the need to critically evaluate data and create a holistic and up-to-date model.
Future economic models for imaging diagnostics should address the abovementioned limitations to enhance the validity and applicability of their results. Specifically, they should consider the link between diagnostic performance and downstream outcomes namely HCRU and cardiac events, both of which are costly. In addition, future economic models comparing PET- and SPECT-MPI should consider subpopulations where underlying disease mechanisms and anatomical imaging challenges limit the performance of current imaging diagnostics. The time horizon should also be of sufficient length to capture early and downstream medical costs including referrals to CA, revascularization, hospitalizations, intensive care, and follow-up visits due to MI or MACE events. Lastly, future models should include comparisons within imaging modality tracers and address novel PET-MPI radiotracers.
Comparative studies
There are limited comparative reports of HCRU and downstream cardiac outcomes following PET- and SPECT-MPI. The existing studies have several notable limitations in their design and sources of heterogeneity. Firstly, most studies were not designed to assess the long-term impact of diagnostic testing on clinical outcomes. Only one comparative studyCitation61 was designed to assess whether diagnostic accuracy impacts downstream management; however, the follow-up time was likely too short to fully capture the impact of clinical event occurrence. All patients in this study also had a history of CAD and were medically managed; therefore, the full benefit of PET as a diagnostic modality may not be shown. Follow-up time also varied among the included studies, ranging from 12 to 24 months. Differences in follow-up duration can impact the observed outcomes and limit the comparability across studies.
A significant source of heterogeneity is the lack of randomized study designs among the comparative studies. Patel et al.Citation61 was the only study with randomization, while others were retrospective observational designs or real word evidence claims studies. This lack of randomization introduces challenges when comparing and generalizing results. Additionally, it is often difficult to match study arms on important patient characteristics. Patel et al.Citation61 was randomized and the reported baseline characteristics were well-balanced between the PET- and SPECT-MPI arms; however, several of the non-randomized comparative studies noted differences in baseline characteristics. Knight et al.Citation123, Hlatky et al.Citation53, and Ananthasubramaniam et al.Citation62 showed that the PET-MPI population was older, and had a higher prevalence of prognostic CAD factors, including diabetes, higher BMI, chronic kidney disease, hypertension, dyslipidemia, gastroesophageal reflux disease, cardiac arrhythmias, family history of CAD, and medication history than the SPECT-MPI population. Knight et al.Citation123 also found that the PET-MPI population had a higher grade of obstructive CAD and multivessel disease, which reflected in the higher early referrals to CA and revascularization within the 60-day follow-up duration of the study. The differences in baseline characteristics among these studies demonstrate that the PET-MPI population is intrinsically sicker compared to the SPECT-MPI population, presenting challenges in the direct comparison of outcomes between PET- and SPECT-MPI.
Another source of heterogeneity was the variability in MPI protocol among the included studies, including differences and uncertainties in the type of stress used (exercise or pharmacologic), perfusion tracers, type of imaging cameras (cadmium-zinc telluride [CZT] SPECT camera or conventional SPECT camera), and the incorporation of AC techniques. For instance, Knight et al.Citation123 and Patel et al.Citation61 also utilized AC in all SPECT-MPI scans. Conversely, it is not clear whether AC was implemented in the other included studies. Moreover, Patel et al.Citation61 and Mimouni et al.Citation58 utilized CZT cameras for imaging assessments, whereas other studies used conventional SPECT cameras. CZT cameras have been shown to improve image quality and diagnostic performance for SPECT-MPI, which may result in reduced downstream outcomes compared to studies utilizing traditional SPECT camerasCitation124. These technical disparities can affect the diagnostic performance of the imaging tests and thus impact the observed outcomes.
Single-arm studies
The single-arm analyses utilized a large dataset to inform pooled estimates of HCRU with various CAD populations. The results suggest that there are lower rates of HCRU and cardiac events in studies with 100% suspected CAD patients compared to studies with 100% confirmed CAD patients. The pooled estimates can be used to inform future economic modeling of PET- and SPECT-MPI for CAD diagnosis. The results presented herein highlight that economic models should differentiate between suspected and confirmed CAD patients as the rates of HCRU and cardiac events differ and consider both early- and late-HCRU.
The high heterogeneity among the included single-arm studies creates challenges when pooling data to determine if HCRU differs across studies in various subgroups. There are several sources of heterogeneity in the single-arm studies including variable population baseline characteristics, study designs, and follow-up times. There are also MPI protocol and technology differences among studies, including type of stress test used, camera type, perfusion tracer type, and the use of AC techniques. A future comparative study that assesses more closely how HCRU and cardiac event rates differ by higher risk subpopulations (e.g. diabetes or obesity) is required.
Strengths and limitations
This is the first SLR to comprehensively synthesize economic, HCRU, and cardiac outcomes associated with PET- and SPECT-MPI imaging for CAD diagnosis, which advances the scientific literature for this disease landscape. Additionally, a thorough examination of existing studies was conducted to understand the extent of heterogeneity and limitations in study design. This work will inform the development of future economic evaluations of PET- and SPECT-MPI and add to the value framework of appropriate and timely utilization of CAD diagnosis. A limitation to note is that PCI and CABG rates were treated as mutually exclusive events and were added together into an aggregate value for early- and late-revascularization when pooled. Additionally, given the heterogeneity in study designs, patient characteristics, and follow-up times, pooling the data in meta-analyses was challenging. Caution is warranted when interpreting the findings.
Conclusion
This SLR collated economic and HCRU evidence following PET- and SPECT-MPI for CAD diagnosis and highlights important limitations and challenges of pooling HCRU and clinical event data. The economic evaluations comparing PET-MPI and SPECT-MPI in populations with known and/or suspected CAD are also limited and highly variable in nature. These evaluations typically have short time horizons, resulting in an incomplete assessment of the costs associated with downstream HCRU outcomes. Despite its higher procedure cost, PET-MPI has the potential to be cost-effective based on its improved diagnostic performance compared to SPECT-MPI; however, this economic potential is underestimated in current economic model frameworks. PET-MPI can facilitate more targeted and effective interventions by accurately identifying and stratifying patients with CAD, improving patient outcomes, and potentially reducing long-term healthcare costs. Future economic models of PET- and SPECT-MPI should consider the inclusion of diagnostic performance and link to HCRU and downstream cardiac events to capture the full resource utilization landscape.
Transparency
Declaration of funding
This work was funded by GE HealthCare.
Declaration of financial/other relationships
This work received funding from GE HealthCare. GE HealthCare contracted EVERSANA to complete this work. NF, SP, LA, AWM, DW, and AOR are employees of EVERSANA. ES and AC are employees of GE HealthCare.
Author contributions
Concept and design: NF, SP, AC; Acquisition of data: LA, AWM, DW, AOR; Analysis and interpretation of data: NF, SP, LA, AWM, DW, AOR, ES; Drafting of the manuscript: NF, SP, LA, AWM, DW, AOR; Critical revision of the paper for important intellectual content: NF, SP, ES, AC; Obtaining funding: ES, AC; Administrative, technical, or logistic support: SP, ES, AC; Supervision: NF, AC.
Role of the funder/sponsor
ES and AC supported the development of this work and are employees of GE HealthCare (the Funder). The publication of study results was not contingent on the sponsor’s approval or censorship of the manuscript.
Reviewer disclosures
Peer reviewers on this manuscript have no relevant financial or other relationships to disclose.
Previous presentations
Ferko N, Priest S, Ramirez AO, et al. Challenges and Opportunities with Quantifying Comprehensive Health Economics of Diagnostic Imaging Modalities Using Radiopharmaceuticals: A Case Study in Coronary Artery Disease. Poster session presented at: The Professional Society for Health Economics and Outcomes Research (ISPOR); 2023 May 7–10; Boston, MA.
Supplemental Material
Download MS Word (398.8 KB)Acknowledgement
Data and statistical analysis support was provided by Tim Disher of EVERSANA (Burlington, ON, Canada). Scientific advice was provided by Francois Tranquart of GE HealthCare (Chalfont St Giles, UK).
References
- Toia P, La Grutta L, Smeraldi T, et al. Updated diagnostic & prognostic paradigm for CAD: a narrative review. Cardiovasc Diagn Ther. 2020;10(6):1979–1991. doi: 10.21037/cdt-20-526.
- Brown JC, Gerhardt TE, Kwon E. Risk factors for coronary artery disease. StatPearls. Treasure Island (FL): statPearls Publishing; 2023.
- Khan MA, Hashim MJ, Mustafa H, et al. Global epidemiology of ischemic heart disease: results from the global burden of disease study. Cureus. 2020;12(7):e9349. doi: 10.7759/cureus.9349.
- Bauersachs R, Zeymer U, Brière J-B, et al. Burden of coronary artery disease and peripheral artery disease: a literature review. Cardiovasc Ther. 2019;2019:8295054–8295059. doi: 10.1155/2019/8295054.
- Walker S, Asaria M, Manca A, et al. Long-term healthcare use and costs in patients with stable coronary artery disease: a population-based cohort using linked health records (CALIBER). Eur Heart J Qual Care Clin Outcomes. 2016;2(2):125–140. doi: 10.1093/ehjqcco/qcw003.
- Nelson AJ, Ardissino M, Psaltis PJ. Current approach to the diagnosis of atherosclerotic coronary artery disease: more questions than answers. Ther Adv Chronic Dis. 2019;10:2040622319884819. doi: 10.1177/2040622319884819.
- Goyal A, Pagidipati N, Hill CL, et al. Clinical and economic implications of inconclusive noninvasive test results in stable patients with suspected coronary artery disease: insights from the PROMISE trial. Circ Cardiovasc Imaging. 2020;13(4):e009986. doi: 10.1161/CIRCIMAGING.119.009986.
- Ladapo JA, Goldfeld KS, Douglas PS. Projected morbidity and mortality from missed diagnoses of coronary artery disease in the United States. Int J Cardiol. 2015;195:250–252. doi: 10.1016/j.ijcard.2015.05.033.
- Bradley SM, Spertus JA, Kennedy KF, et al. Patient selection for diagnostic coronary angiography and hospital-level percutaneous coronary intervention appropriateness: insights from the national cardiovascular data registry. JAMA Intern Med. 2014;174(10):1630–1639. doi: 10.1001/jamainternmed.2014.3904.
- Patel MR, Dai D, Hernandez AF, et al. Prevalence and predictors of nonobstructive coronary artery disease identified with coronary angiography in contemporary clinical practice. Am Heart J. 2014;167(6):846–852. e2. doi: 10.1016/j.ahj.2014.03.001.
- Rachwan RJ, Mshelbwala FS, Dardari Z, et al. False-positive stress echocardiograms: predictors and prognostic relevance. Int J Cardiol. 2019;296:157–163. doi: 10.1016/j.ijcard.2019.08.037.
- Gulati M, Levy PD, Mukherjee D, et al. AHA/ACC/ASE/CHEST/SAEM/SCCT/SCMR guideline for the evaluation and diagnosis of chest pain: a report of the American college of cardiology/American heart association joint committee on clinical practice guidelines (vol 144, pg e368, 2021). CIRCULATION. 2021;144(22):E455–E455. 2021
- Madhavan MV, Gersh BJ, Alexander KP, et al. Coronary artery disease in patients ≥80 years of age. J Am Coll Cardiol. 2018;71(18):2015–2040. doi: 10.1016/j.jacc.2017.12.068.
- Raso I, Passarelli I, Valenti G, et al. The diagnostic process of stable angina: still many doubts since heberden’s first description 250 years ago. J Cardiovasc Med (Hagerstown). 2018;19(2):45–50. doi: 10.2459/JCM.0000000000000610.
- Knuuti J, Wijns W, Saraste A, et al. 2019 ESC guidelines for the diagnosis and management of chronic coronary syndromes: the task force for the diagnosis and management of chronic coronary syndromes of the european society of cardiology (ESC). Eur Heart J. 2020;41(3):407–477. doi: 10.1093/eurheartj/ehz425.
- Garcia M, Mulvagh SL, Merz CN, et al. Cardiovascular disease in women: clinical perspectives. Circ Res. 2016;118(8):1273–1293. doi: 10.1161/CIRCRESAHA.116.307547.
- Nguyen PK, Nag D, Wu JC. Sex differences in the diagnostic evaluation of coronary artery disease. J Nucl Cardiol. 2011;18(1):144–152. doi: 10.1007/s12350-010-9315-2.
- Taqueti VR. Coronary microvascular dysfunction in vasospastic angina: provocative role for the microcirculation in macrovessel disease prognosis. J Am Coll Cardiol. 2019;74(19):2361–2364. doi: 10.1016/j.jacc.2019.09.042.
- Taqueti VR, Dorbala S, Wolinsky D, et al. Myocardial perfusion imaging in women for the evaluation of stable ischemic heart disease-state-of-the-evidence and clinical recommendations. J Nucl Cardiol. 2017;24(4):1402–1426. doi: 10.1007/s12350-017-0926-8.
- Crea F, Camici PG, Bairey Merz CN. Coronary microvascular dysfunction: an update. Eur Heart J. 2014;35(17):1101–1111. doi: 10.1093/eurheartj/eht513.
- Murthy VL, Naya M, Foster CR, et al. Association between coronary vascular dysfunction and cardiac mortality in patients with and without diabetes mellitus. Circulation. 2012;126(15):1858–1868. doi: 10.1161/CIRCULATIONAHA.112.120402.
- Park S, Kang HJ, Jeon JH, et al. Recent advances in the pathogenesis of microvascular complications in diabetes. Arch Pharm Res. 2019;42(3):252–262. doi: 10.1007/s12272-019-01130-3.
- Wu KY, Timmerman NP, McPhedran R, et al. Differential association of diabetes mellitus and female sex with impaired myocardial flow reserve across the spectrum of epicardial coronary disease. Eur Heart J Cardiovasc Imaging. 2020;21(5):576–584. doi: 10.1093/ehjci/jez163.
- Bajaj NS, Osborne MT, Gupta A, et al. Coronary microvascular dysfunction and cardiovascular risk in obese patients. J Am Coll Cardiol. 2018;72(7):707–717. doi: 10.1016/j.jacc.2018.05.049.
- Taqueti VR, Everett BM, Murthy VL, et al. Interaction of impaired coronary flow reserve and cardiomyocyte injury on adverse cardiovascular outcomes in patients without overt coronary artery disease. Circulation. 2015;131(6):528–535. doi: 10.1161/CIRCULATIONAHA.114.009716.
- Pepine CJ, Anderson RD, Sharaf BL, et al. Coronary microvascular reactivity to adenosine predicts adverse outcome in women evaluated for suspected ischemia results from the national heart, lung and blood institute WISE (women’s ischemia syndrome evaluation) study. J Am Coll Cardiol. 2010;55(25):2825–2832. doi: 10.1016/j.jacc.2010.01.054.
- IMV. 2023 PET imaging market summary report 2023.
- Rahman H, Corcoran D, Aetesam-Ur-Rahman M, et al. Diagnosis of patients with angina and non-obstructive coronary disease in the catheter laboratory. Heart. 2019;105(20):1536–1542. doi: 10.1136/heartjnl-2019-315042.
- Takx RA, Blomberg BA, El Aidi H, et al. Diagnostic accuracy of stress myocardial perfusion imaging compared to invasive coronary angiography with fractional flow reserve meta-analysis. Circ Cardiovasc Imag. 2015;8(1):e002666. doi: 10.1161/CIRCIMAGING.114.0026666.
- Mettler FA, Guiberteau MJ. Essentials of nuclear medicine and molecular imaging E-Book. Philadelphia (PA): Elsevier Health Sciences; 2018.
- Driessen RS, Raijmakers PG, Stuijfzand WJ, et al. Myocardial perfusion imaging with PET. Int J Cardiovasc Imaging. 2017;33(7):1021–1031. doi: 10.1007/s10554-017-1084-4.
- Stendahl JC, Kwan JM, Pucar D, et al. Radiotracers to address unmet clinical needs in cardiovascular imaging, part 1: technical considerations and perfusion and neuronal imaging. J Nucl Med. 2022;63(7):986–994. doi: 10.2967/jnumed.121.263507.
- Sciagrà R, Lubberink M, Hyafil F, et al. EANM procedural guidelines for PET/CT quantitative myocardial perfusion imaging. Eur J Nucl Med Mol Imaging. 2021;48(4):1040–1069. doi: 10.1007/s00259-020-05046-9.
- Carpeggiani C, Picano E, Brambilla M, et al. Variability of radiation doses of cardiac diagnostic imaging tests: the RADIO-EVINCI study (RADIationdOse subproject of the EVINCI study). BMC Cardiovasc Disord. 2017;17(1):63. doi: 10.1186/s12872-017-0474-9.
- El Fakhri G, Kardan A, Sitek A, et al. Reproducibility and accuracy of quantitative myocardial blood flow assessment with (82)Rb PET: comparison with (13)N-ammonia PET. J Nucl Med. 2009;50(7):1062–1071. doi: 10.2967/jnumed.104.007831.
- Schindler TH, Dilsizian V. Coronary microvascular dysfunction: clinical considerations and noninvasive diagnosis. JACC Cardiovasc Imaging. 2020;13(1 Pt 1):140–155. doi: 10.1016/j.jcmg.2018.11.036.
- Taqueti VR, Di Carli MF. Coronary microvascular disease pathogenic mechanisms and therapeutic options: JACC state-of-the-Art review. J Am Coll Cardiol. 2018;72(21):2625–2641. doi: 10.1016/j.jacc.2018.09.042.
- Bateman TM. Current status of myocardial perfusion PET in the United States. Annals of Nuclear Cardiology. 2017;3(1):157–162. doi: 10.17996/anc.17-00047.
- Ziadi MC, Dekemp RA, Williams KA, et al. Impaired myocardial flow reserve on rubidium-82 positron emission tomography imaging predicts adverse outcomes in patients assessed for myocardial ischemia. J Am Coll Cardiol. 2011;58(7):740–748. doi: 10.1016/j.jacc.2011.01.065.
- Mc Ardle BA, Dowsley TF, deKemp RA, et al. Does rubidium-82 PET have superior accuracy to SPECT perfusion imaging for the diagnosis of obstructive coronary disease?: a systematic review and meta-analysis. J Am Coll Cardiol. 2012;60(18):1828–1837. doi: 10.1016/j.jacc.2012.07.038.
- Parker MW, Iskandar A, Limone B, et al. Diagnostic accuracy of cardiac positron emission tomography versus single photon emission computed tomography for coronary artery disease: a bivariate meta-analysis. Circ Cardiovasc Imaging. 2012;5(6):700–707. doi: 10.1161/CIRCIMAGING.112.978270.
- Xu J, Cai F, Geng C, et al. Diagnostic performance of CMR, SPECT, and PET imaging for the identification of coronary artery disease: a meta-analysis. Front Cardiovasc Med. 2021;8:621389. doi: 10.3389/fcvm.2021.621389.
- Jaarsma C, Leiner T, Bekkers SC, et al. Diagnostic performance of noninvasive myocardial perfusion imaging using single-photon emission computed tomography, cardiac magnetic resonance, and positron emission tomography imaging for the detection of obstructive coronary artery disease: a meta-analysis. J Am Coll Cardiol. 2012;59(19):1719–1728. doi: 10.1016/j.jacc.2011.12.040.
- Juárez-Orozco LE, Tio RA, Alexanderson E, et al. Quantitative myocardial perfusion evaluation with positron emission tomography and the risk of cardiovascular events in patients with coronary artery disease: a systematic review of prognostic studies. Eur Heart J Cardiovasc Imaging. 2018;19(10):1179–1187. doi: 10.1093/ehjci/jex331.
- Green R, Cantoni V, Acampa W, et al. Prognostic value of coronary flow reserve in patients with suspected or known coronary artery disease referred to PET myocardial perfusion imaging: a meta-analysis. J Nucl Cardiol. 2021;28(3):904–918. doi: 10.1007/s12350-019-02000-7.
- Smulders MW, Jaarsma C, Nelemans PJ, et al. Comparison of the prognostic value of negative non-invasive cardiac investigations in patients with suspected or known coronary artery disease-a meta-analysis. Eur Heart J Cardiovasc Imaging. 2017;18(9):980–987. doi: 10.1093/ehjci/jex014.
- Maddahi J, Agostini D, Bateman TM, et al. Flurpiridaz F-18 PET myocardial perfusion imaging in patients with suspected coronary artery disease. J Am Coll Cardiol. 2023;82(16):1598–1610. doi: 10.1016/j.jacc.2023.08.016.
- Nayfeh M, Ahmed AI, Saad JM, et al. The role of cardiac PET in diagnosis and prognosis of ischemic heart disease: optimal modality across different patient populations. Curr Atheroscler Rep. 2023;25(7):351–357. doi: 10.1007/s11883-023-01107-0.
- Patterson JC, 2nd, Mosley ML. How available is positron emission tomography in the United States? Mol Imaging Biol. 2005;7(3):197–200. doi: 10.1007/s11307-005-4116-8.
- McGowan J, Sampson M, Salzwedel DM, et al. PRESS peer review of electronic search strategies: 2015 guideline statement. J Clin Epidemiol. 2016;75:40–46. doi: 10.1016/j.jclinepi.2016.01.021.
- Amir-Behghadami M, Janati A. Population, intervention, comparison, outcomes and study (PICOS) design as a framework to formulate eligibility criteria in systematic reviews. Emerg Med J. 2020;37(6):387–387. doi: 10.1136/emermed-2020-209567.
- DistillerSR. Version 2.35. DistillerSR Inc.: 2021. Accessed June 12, 2023. https://distillersr.com.
- Hlatky MA, Shilane D, Hachamovitch R, et al. Economic outcomes in the study of myocardial perfusion and coronary anatomy imaging roles in coronary artery disease registry: the SPARC study. J Am Coll Cardiol. 2014;63(10):1002–1008. doi: 10.1016/j.jacc.2013.11.038.
- Merhige ME, Breen WJ, Shelton V, et al. Impact of myocardial perfusion imaging with PET and (82)Rb on downstream invasive procedure utilization, costs, and outcomes in coronary disease management. J Nucl Med. 2007;48(7):1069–1076. doi: 10.2967/jnumed.106.038323.
- Lorenzoni V, Bellelli S, Caselli C, et al. Cost-effectiveness analysis of stand-alone or combined non-invasive imaging tests for the diagnosis of stable coronary artery disease: results from the EVINCI study. Eur J Health Econ. 2019;20(9):1451–1449. doi: 10.1007/s10198-019-01096-5.
- Garber AM, Solomon NA. Cost-effectiveness of alternative test strategies for the diagnosis of coronary artery disease. Ann Intern Med. 1999;130(9):719–728. doi: 10.7326/0003-4819-130-9-199905040-00003.
- Patterson RE, Eisner RL, Horowitz SF. Comparison of cost-effectiveness and utility of exercise ECG, single photon emission computed tomography, positron emission tomography, and coronary angiography for diagnosis of coronary artery disease. Circulation. 1995;91(1):54–65. doi: 10.1161/01.cir.91.1.54.
- Mimouni M, Bulsei J, Darlington M, et al. Cost-effectiveness of 82-Rubidium PET myocardial perfusion imaging for the diagnosis of myocardial ischemia depending on the prevalence of coronary artery disease. EJNMMI Res. 2023;13(1):9. doi: 10.1186/s13550-023-00954-x.
- Ma Q, Sridhar G, Power T, et al. Assessing the downstream value of first-line cardiac positron emission tomography (PET) imaging using real world medicare fee-for-service claims data. J Nucl Cardiol. 2021;28(5):2126–2137. doi: 10.1007/s12350-019-01974-8.
- Siegrist PT, Husmann L, Knabenhans M, et al. (13)N-ammonia myocardial perfusion imaging with a PET/CT scanner: impact on clinical decision making and cost-effectiveness. Eur J Nucl Med Mol Imaging. 2008;35(5):889–895. doi: 10.1007/s00259-007-0647-3.
- Patel KK, Al Badarin F, Chan PS, et al. Randomized comparison of clinical effectiveness of pharmacologic SPECT and PET MPI in symptomatic CAD patients. JACC Cardiovasc Imaging. 2019;12(9):1821–1831. doi: 10.1016/j.jcmg.2019.04.020.
- Ananthasubramaniam K, Kitt TM, Saxena A, et al. Healthcare resource utilization among patients receiving non-invasive testing for coronary artery disease in an outpatient setting: a cohort study reflecting daily practice trends. J Nucl Cardiol. 2022;29(4):1776–1787. doi: 10.1007/s12350-021-02549-2.
- Pelletier-Galarneau M, Vandenbroucke E, Lu M, et al. Characteristics and key differences between patient populations receiving imaging modalities for coronary artery disease diagnosis in the US. BMC Cardiovasc Disord. 2023;23(1):251. doi: 10.1186/s12872-023-03218-7.
- Klein E, Miller RJH, Sharir T, et al. Automated quantitative analysis of CZT SPECT stratifies cardiovascular risk in the obese population: analysis of the REFINE SPECT registry. J Nucl Cardiol. 2022;29(2):727–736. doi: 10.1007/s12350-020-02334-7.
- Tamarappoo BK, Otaki Y, Sharir T, et al. Differences in prognostic value of myocardial perfusion single-Photon emission computed tomography using high-Efficiency Solid-State detector between men and women in a large international multicenter study. Circ Cardiovasc Imaging. 2022;15(6):e012741.
- Acampa W, Petretta M, Daniele S, et al. Incremental prognostic value of stress myocardial perfusion imaging in asymptomatic diabetic patients. Atherosclerosis. 2013;227(2):307–312. doi: 10.1016/j.atherosclerosis.2013.01.011.
- Alnabelsi T, Ahmed AI, Han Y, et al. Added prognostic value of plaque burden to computed tomography angiography and myocardial perfusion imaging in patients with diabetes. Am J Med. 2022;135(6):761–768.e7. doi: 10.1016/j.amjmed.2021.12.010.
- Boiten HJ, van Domburg RT, Valkema R, et al. Dobutamine stress myocardial perfusion imaging: 8-year outcomes in patients with diabetes mellitus. Eur Heart J Cardiovasc Imaging. 2016;17(8):871–876. doi: 10.1093/ehjci/jev351.
- Boiten HJ, van den Berge JC, Valkema R, et al. Ischemia burden on stress SPECT MPI predicts long-term outcomes after revascularization in stable coronary artery disease. J Nucl Cardiol. 2018;25(3):958–966. doi: 10.1007/s12350-016-0735-5.
- Boiten HJ, van der Sijde JN, Ruitinga PR, et al. Long-term prognostic value of exercise technetium-99m tetrofosmin myocardial perfusion single-photon emission computed tomography. J Nucl Cardiol. 2012;19(5):907–913. doi: 10.1007/s12350-012-9585-y.
- Korbee RS, Boiten HJ, Ottenhof M, et al. What is the value of stress (99m)Tc-tetrofosmin myocardial perfusion imaging for the assessment of very long-term outcome in obese patients? J Nucl Cardiol. 2013;20(2):227–233. doi: 10.1007/s12350-012-9657-z.
- Bourque JM, Patel CA, Ali MM, et al. Prevalence and predictors of ischemia and outcomes in outpatients with diabetes mellitus referred for single-photon emission computed tomography myocardial perfusion imaging. Circ Cardiovasc Imaging. 2013;6(3):466–477. doi: 10.1161/CIRCIMAGING.112.000259.
- Candell-Riera J, Ferreira-González I, Marsal JR, et al. Usefulness of exercise test and myocardial perfusion-gated single photon emission computed tomography to improve the prediction of major events. Circ Cardiovasc Imaging. 2013;6(4):531–541. doi: 10.1161/CIRCIMAGING.112.000158.
- Caobelli F, Haaf P, Haenny G, et al. Prognostic value of myocardial perfusion scintigraphy in asymptomatic patients with diabetes mellitus at high cardiovascular risk: 5-year follow-up of the prospective multicenter BARDOT trial. Eur J Nucl Med Mol Imaging. 2021;48(11):3512–3521. doi: 10.1007/s00259-021-05349-5.
- Engbers EM, Timmer JR, Mouden M, et al. Prognostic value of myocardial perfusion imaging with a Cadmium-Zinc-Telluride SPECT camera in patients suspected of having coronary artery disease. J Nucl Med. 2017;58(9):1459–1463. doi: 10.2967/jnumed.116.188516.
- Gaibazzi N, Barbieri A, Boriani G, et al. Imaging functional stress test for stable chest pain symptoms in patients at low pretest probability of coronary artery disease: current practice and long-term outcome. Echocardiography. 2019;36(6):1095–1102. doi: 10.1111/echo.14352.
- Gaibazzi N, Siniscalchi C, Porter TR, et al. Vasodilator stress single-Photon emission computed tomography or contrast stress echocardiography association with hard cardiac events in suspected coronary artery disease. J Am Soc Echocardiogr. 2018;31(6):683–691. doi: 10.1016/j.echo.2018.01.024.
- Ghatak A, Padala S, Katten DM, et al. Risk stratification among diabetic patients undergoing stress myocardial perfusion imaging. J Nucl Cardiol. 2013;20(4):529–538. doi: 10.1007/s12350-013-9731-1.
- Han D, Rozanski A, Gransar H, et al. Comparison of diabetes to other prognostic predictors among patients referred for cardiac stress testing: a contemporary analysis from the REFINE SPECT registry. J Nucl Cardiol. 2022;29(6):3003–3014. doi: 10.1007/s12350-021-02810-8.
- Levsky JM, Spevack DM, Travin MI, et al. Coronary computed tomography angiography versus radionuclide myocardial perfusion imaging in patients with chest pain admitted to telemetry: a randomized trial. Ann Intern Med. 2015;163(3):174–183. doi: 10.7326/M14-2948.
- López Rodríguez E, Jauregui Garrido B, Ruiz Franco-Baux J, et al. Prognostic value of myocardial perfusion scintigraphy in diabetic patients and without coronary lesion. Rev Esp Med Nucl Imagen Mol (Engl Ed). 2021;40(2):100–106. doi: 10.1016/j.remnie.2021.01.008.
- Meyer M, Nance JW, Jr., Schoepf UJ, et al. Cost-effectiveness of substituting dual-energy CT for SPECT in the assessment of myocardial perfusion for the workup of coronary artery disease. Eur J Radiol. 2012;81(12):3719–3725. doi: 10.1016/j.ejrad.2010.12.055.
- Min JK, Koduru S, Dunning AM, et al. Coronary CT angiography versus myocardial perfusion imaging for near-term quality of life, cost and radiation exposure: a prospective multicenter randomized pilot trial. J Cardiovasc Comput Tomogr. 2012;6(4):274–283. doi: 10.1016/j.jcct.2012.06.002.
- Morales DCV, Bhavnani SP, Ahlberg AW, et al. Coronary risk equivalence of diabetes assessed by SPECT-MPI. J Nucl Cardiol. 2019;26(4):1093–1102. doi: 10.1007/s12350-017-1114-6.
- Nanasato M, Matsumoto N, Nakajima K, et al. Prognostic impact of reducing myocardial ischemia identified using ECG-gated myocardial perfusion SPECT in japanese patients with coronary artery disease: J-ACCESS 4 study. Int J Cardiol. 2018;267:202–207. doi: 10.1016/j.ijcard.2018.05.090.
- Poulin MF, Alexander S, Doukky R. Prognostic implications of stress modality on mortality risk and cause of death in patients undergoing office-based SPECT myocardial perfusion imaging. J Nucl Cardiol. 2016;23(2):202–211. doi: 10.1007/s12350-014-0064-5.
- Ramsaran E, Dai Q, Sundaresan D, et al. Mortality in stable coronary disease in patients with intermediate- or high-risk myocardial perfusion imaging. Am J Cardiol. 2022;168:1–10. doi: 10.1016/j.amjcard.2021.12.018.
- Romero-Farina G, Aguadé-Bruix S, Cuellar-Calabria H, et al. Gender differences in outcome in patients with diabetes mellitus. J Nucl Cardiol. 2022;29(1):72–82. doi: 10.1007/s12350-020-02195-0.
- Romero-Farina G, Candell-Riera J, Aguadé-Bruix S, et al. A novel clinical risk prediction model for myocardial infarction, coronary revascularization, and cardiac death according to clinical, exercise, and gated SPECT variables (VH-RS). Eur Heart J Cardiovasc Imaging. 2020;21(2):210–221.
- Santos MT, Parker MW, Heller GV. Evaluating gender differences in prognosis following SPECT myocardial perfusion imaging among patients with diabetes and known or suspected coronary disease in the modern era. J Nucl Cardiol. 2013;20(6):1021–1029. doi: 10.1007/s12350-013-9771-6.
- Simonsen JA, Gerke O, Rask CK, et al. Prognosis in patients with suspected or known ischemic heart disease and normal myocardial perfusion: long-term outcome and temporal risk variations. J Nucl Cardiol. 2013;20(3):347–357. doi: 10.1007/s12350-013-9696-0.
- Songy B, Guernou M, Hivoux D, et al. Prognostic value of one millisievert exercise myocardial perfusion imaging in patients without known coronary artery disease. J Nucl Cardiol. 2018;25(1):120–130. doi: 10.1007/s12350-016-0601-5.
- Stillman AE, Gatsonis C, Lima JAC, et al. Coronary computed tomography angiography compared with single photon emission computed tomography myocardial perfusion imaging as a guide to optimal medical therapy in patients presenting with stable angina: the RESCUE trial. J Am Heart Assoc. 2020;9(24):e017993.
- Takura T, Yokoi H, Tanaka N, et al. Health economics-based verification of functional myocardial ischemia evaluation of stable coronary artery disease in Japan: a long-term longitudinal study using propensity score matching. J Nucl Cardiol. 2022;29(3):1356–1369. doi: 10.1007/s12350-020-02502-9.
- Tandon S, Wackers FJ, Inzucchi SE, et al. Gender-based divergence of cardiovascular outcomes in asymptomatic patients with type 2 diabetes: results from the DIAD study. Diab Vasc Dis Res. 2012;9(2):124–130. doi: 10.1177/1479164111431470.
- Tandon V, Hall D, Yam Y, et al. Rates of downstream invasive coronary angiography and revascularization: computed tomographic coronary angiography vs. Tc-99m single photon emission computed tomography. Eur Heart J. 2012;33(6):776–782. doi: 10.1093/eurheartj/ehr346.
- Thom H, West NE, Hughes V, et al. Cost-effectiveness of initial stress cardiovascular MR, stress SPECT or stress echocardiography as a gate-keeper test, compared with upfront invasive coronary angiography in the investigation and management of patients with stable chest pain: mid-term outcomes from the CECaT randomised controlled trial. BMJ Open. 2014;4(2):e003419. doi: 10.1136/bmjopen-2013-003419.
- Yoda S, Nakanishi K, Tano A, et al. Major cardiac event risk scores estimated with gated myocardial perfusion imaging in japanese patients with coronary artery disease. J Cardiol. 2016;67(1):64–70. doi: 10.1016/j.jjcc.2015.04.008.
- Zeltser R, Tortez LM, Druz RS, et al. Downstream resource utilization following SPECT: impact of age and gender. J Nucl Cardiol. 2017;24(5):1657–1661. doi: 10.1007/s12350-016-0464-9.
- Zellweger MJ, Fahrni G, Ritter M, et al. Prognostic value of "routine" cardiac stress imaging 5 years after percutaneous coronary intervention: the prospective long-term observational BASKET (basel stent kosteneffektivitäts trial) LATE IMAGING study. JACC Cardiovasc Interv. 2014;7(6):615–621. doi: 10.1016/j.jcin.2014.01.161.
- Murthy VL, Naya M, Taqueti VR, et al. Effects of sex on coronary microvascular dysfunction and cardiac outcomes. Circulation. 2014;129(24):2518–2527. doi: 10.1161/CIRCULATIONAHA.113.008507.
- Gupta A, Taqueti VR, van de Hoef TP, et al. Integrated noninvasive physiological assessment of coronary circulatory function and impact on cardiovascular mortality in patients with stable coronary artery disease. Circulation. 2017;136(24):2325–2336. doi: 10.1161/CIRCULATIONAHA.117.029992.
- Majmudar MD, Murthy VL, Shah RV, et al. Quantification of coronary flow reserve in patients with ischaemic and non-ischaemic cardiomyopathy and its association with clinical outcomes. Eur Heart J Cardiovasc Imaging. 2015;16(8):900–909. doi: 10.1093/ehjci/jev012.
- Ahmed AI, Al Rifai M, Alahdab F, et al. Incremental prognostic value of digital positron emission tomography derived myocardial flow reserve: a prospective cohort study. Int J Cardiol. 2022;371:465–471. doi: 10.1016/j.ijcard.2022.09.015.
- Brophey MD, Farukhi IM, Castanon R, et al. Accuracy of (82)Rb PET/CT myocardial perfusion imaging with regadenoson stress, including 3-Year clinical outcomes. J Nucl Med Technol. 2017;45(2):75–81. doi: 10.2967/jnmt.116.188938.
- Farhad H, Dunet V, Bachelard K, et al. Added prognostic value of myocardial blood flow quantitation in rubidium-82 positron emission tomography imaging. Eur Heart J Cardiovasc Imaging. 2013;14(12):1203–1210. doi: 10.1093/ehjci/jet068.
- von Felten E, Benz DC, Benetos G, et al. Prognostic value of regional myocardial flow reserve derived from (13)N-ammonia positron emission tomography in patients with suspected coronary artery disease. Eur J Nucl Med Mol Imaging. 2021;49(1):311–320. doi: 10.1007/s00259-021-05459-0.
- Gebhard C, Fiechter M, Herzog BA, et al. Sex differences in the long-term prognostic value of (13)N-ammonia myocardial perfusion positron emission tomography. Eur J Nucl Med Mol Imaging. 2018;45(11):1964–1974. doi: 10.1007/s00259-018-4046-8.
- Miller RJH, Han D, Singh A, et al. Relationship between ischaemia, coronary artery calcium scores, and major adverse cardiovascular events. Eur Heart J Cardiovasc Imaging. 2022;23(11):1423–1433. doi: 10.1093/ehjci/jeac082.
- Miura S, Naya M, Kumamaru H, et al. Prognostic value of modified coronary flow capacity by (13)N-ammonia myocardial perfusion positron emission tomography in patients without obstructive coronary arteries. J Cardiol. 2022;79(2):247–256. doi: 10.1016/j.jjcc.2021.09.001.
- Taqueti VR, Solomon SD, Shah AM, et al. Coronary microvascular dysfunction and future risk of heart failure with preserved ejection fraction. Eur Heart J. 2018;39(10):840–849. doi: 10.1093/eurheartj/ehx721.
- van Diemen PA, Wijmenga JT, Driessen RS, et al. Defining the prognostic value of [15O]H2O positron emission tomography-derived myocardial ischaemic burden. Eur Heart J Cardiovasc Imaging. 2021;22(6):638–646. doi: 10.1093/ehjci/jeaa305.
- Bom MJ, van Diemen PA, Driessen RS, et al. Prognostic value of [15O]H2O positron emission tomography-derived global and regional myocardial perfusion. Eur Heart J Cardiovasc Imaging. 2020;21(7):777–786. doi: 10.1093/ehjci/jez258.
- Aggarwal NR, Drozdova A, Askew JW, et al. Feasibility and diagnostic accuracy of exercise treadmill nitrogen-13 ammonia PET myocardial perfusion imaging of obese patients. J Nucl Cardiol. 2015;22(6):1273–1280. doi: 10.1007/s12350-015-0073-z.
- Chow BJ, Dorbala S, Di Carli MF, et al. Prognostic value of PET myocardial perfusion imaging in obese patients. JACC Cardiovasc Imaging. 2014;7(3):278–287. doi: 10.1016/j.jcmg.2013.12.008.
- Kay J, Dorbala S, Goyal A, et al. Influence of sex on risk stratification with stress myocardial perfusion Rb-82 positron emission tomography: results from the PET (positron emission tomography) prognosis multicenter registry. J Am Coll Cardiol. 2013;62(20):1866–1876. doi: 10.1016/j.jacc.2013.06.017.
- Le VT, Knight S, Min DB, et al. Absence of coronary artery calcium during positron emission tomography stress testing in patients without known coronary artery disease identifies individuals with very low risk of cardiac events. Circ Cardiovasc Imaging. 2020;13(1):e009907.
- Skali H, Di Carli MF, Blankstein R, et al. Stress myocardial perfusion PET provides incremental risk prediction in patients with and patients without diabetes. Radiol Cardiothorac Imaging. 2019;1(2):e180018. doi: 10.1148/ryct.2019180018.
- van der Zant FM, Maurits W, Lazarenko SV, et al. Relation between pharmacological stress ECG, 13NH3-PET/CT outcome and the occurrence of cardiac events during follow-up in women with chest pain. Nucl Med Commun. 2020;41(8):783–789. doi: 10.1097/MNM.0000000000001212.
- Breheny K, Sutton AJ, Deeks JJ. Model-based economic evaluations of diagnostic point of care tests were rarely fit for purpose. J Clin Epidemiol. 2019;109:1–11. doi: 10.1016/j.jclinepi.2018.11.003.
- Novielli N, Cooper NJ, Abrams KR, et al. How is evidence on test performance synthesized for economic decision models of diagnostic tests? A systematic appraisal of health technology assessments in the UK since 1997. Value Health. 2010;13(8):952–957. doi: 10.1111/j.1524-4733.2010.00762.x.
- Xie X, Tiggelaar S, Guo J, et al. Developing economic models for assessing the cost-effectiveness of multiple diagnostic tests: methods and applications. Med Decis Making. 2022;42(7):861–871. doi: 10.1177/0272989X221089268.
- Knight S, Min DB, Le VT, et al. Implementation of a cardiac PET stress program: comparison of outcomes to the preceding SPECT era. JCI Insight. 2018;3(9)doi: 10.1172/jci.insight.120949.
- Yokota S, Mouden M, Ottervanger JP, et al. Prognostic value of normal stress-only myocardial perfusion imaging: a comparison between conventional and CZT-based SPECT. Eur J Nucl Med Mol Imaging. 2016;43(2):296–301. doi: 10.1007/s00259-015-3192-5.