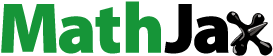
ABSTRACT
Debate persists regarding the nature of age-related deficits in inhibition, and whether inhibitory functioning depends on working memory systems. The current research aimed to measure age-related differences in inhibition and working memory, characterize the relationship between inhibitory functions and working memory performance, and determine how these relationships are affected by age. Toward these ends, we measured performance on a range of established paradigms in 60 young adults (18–30 years) and 60 older adults (60–88 years). Our findings support age-related increases in reflexive inhibition (based on the fixation offset effect and inhibition of return) and age-related decrements in volitional inhibition (based on several paradigms: antisaccade, Stroop, flanker, and Simon). This evidence of stronger reflexive inhibition combined with weaker volitional inhibition suggests that age-related deterioration of cortical structures may allow subcortical structures to operate less controlled. Regarding working memory, older adults had lower backward digit scores and lower forward and backward spatial scores. However, of the 32 analyses (16 in each age group) that tested for dependence of inhibitory functioning on working memory functioning, only one (in young adults) indicated that inhibition performance significantly depended on working memory performance. These results indicate that inhibition and working memory function largely independently in both age groups, and age-related working memory difficulties cannot account for age-related declines in inhibitory control.
PUBLIC SIGNIFICANCE STATEMENT
This study indicates that, compared to young adults, older adults have difficulties with both working memory and volitional inhibition but show stronger reflexive inhibition.
The pattern of age differences shown in this study suggests that the balance of power shifts from cortical to subcortical brain structures as we age, which may reflect cortical brain regions deteriorating more rapidly as we age, thus limiting their ability to control subcortical brain regions.
In contrast to current theory, in this study the difficulties that older adults showed with volitional inhibition could not be explained by failing working memory systems, and both young and older adults showed very limited evidence that inhibitory functioning depends on working memory.
Inhibition enables humans to prevent reflexive responses in favor of intentional behaviors and facilitates effective responses in the presence of distracting information (Easton, Citation1972). Less effective inhibitory mechanisms can compromise the ability to strategically control behavior (Friedman & Miyake, Citation2004; Houghton & Tipper, Citation1996) and can adversely affect the performance of daily activities (Anstey et al., Citation2005, Citation2009; Hoffman et al., Citation2005). A number of studies have indicated that inhibition tends to decline with adult aging, affecting a variety of functions (Zanto & Gazzaley, Citation2014). For example, compared to young adults, older adults tend to have more difficulty inhibiting distracting identity-based information during a flanker task (Colcombe et al., Citation2005; Machado et al., Citation2009), inhibiting distracting spatial information during a Simon task (Castel et al., Citation2007; van der Lubbe & Verleger, Citation2002), and inhibiting word reading during a Stroop task (Henik et al., Citation1993; Troyer et al., Citation2006). In addition, older adults tend to have more difficulty suppressing reflexive eye movements toward a visual stimulus appearing in the periphery (prosaccade) and take longer to initiate eye movements away from a visual stimulus appearing in the periphery (antisaccade; Brett & Machado, Citation2017; Chen & Machado, Citation2016, Citation2017).
Other studies, however, have reported a lack of age differences in inhibition. For example, Pratt et al. (Citation1997) found that the magnitude of the fixation offset effect (FOE) did not differ between young and older adults. Likewise, in Hartley and Kieley (Citation1995), inhibition of return (IOR) – an inhibitory mechanism for optimizing visual search – was similar in young and older adults (see also Faust & Balota, Citation1997; McCrae & Abrams, Citation2001). Moreover, a meta-analysis by Rey-Mermet and Gade (Citation2018) investigating age differences on 10 tasks commonly used to measure inhibition only found age-related impaired performance on two of them (go/no-go and stop-signal), which led the authors to question the theory of a general inhibition deficit in older adults (for more studies that observed no age differences in measures of inhibitory control, see Borella et al., Citation2009; Eenshuistra et al., Citation2004; Fernandez Duque & Black, Citation2006; Langley et al., Citation2005; Rey-Mermet et al., Citation2018; Verhaeghen, Citation2011; Waszak et al., Citation2010).
Bugg et al. (Citation2007) suggested that the observed age differences in some tasks (e.g., Stroop) could be attributed to a general slowing in processing speed, in addition to age-related changes in inhibitory control. It has also been suggested that aging only affects volitional inhibition, hence IOR and FOE may be unaffected because they are non-conscious in nature (Nieuwenhuis et al., Citation2000). In a similar vein, in relation to adult aging being associated with atrophy of the frontal lobes in particular (e.g., see Hedden & Gabrieli, Citation2004; Vinke et al., Citation2018), it has been suggested that performance on frontally mediated volitional inhibition tasks would be more adversely affected by adult aging than performance on more reflexive inhibition tasks (Andres et al., Citation2008; Kramer et al., Citation2001). Given that performance on tasks that tap volitional inhibition tends to rely more on cortical structures (e.g., Stroop, flanker; Chambers et al., Citation2009; Tsuchida & Fellows, Citation2013), whereas performance on tasks that tap reflexive inhibition tends to be more subcortically mediated (e.g., FOE and IOR paradigms; Machado & Rafal, Citation2004a; Munoz & Fecteau, Citation2002; Sapir et al., Citation1999, Citation2002), differences in age-related deterioration of cortical versus subcortical structures (Aanerud et al., Citation2012; Walhovd et al., Citation2011) could help explain why we do not see an across-the-board decline in inhibitory functioning. Thus, to fully characterize age-related changes in inhibitory functioning, assessment of a wide range of measures is needed, with attention to whether the relevant inhibitory process is considered to be predominantly cortically or subcortically mediated.
There is evidence to suggest that inhibitory processes depend on working memory (WM) functioning (e.g., Andrés et al., Citation2004; Borella et al., Citation2007; Conway et al., Citation1999; Gazzaley et al., Citation2007; Roberts & Pennington, Citation1996; Roberts et al., Citation1994; Verhaeghen & Basak, Citation2005). WM is a system for temporarily processing task-relevant information during complex cognitive tasks and involves the manipulation of information in short-term memory (STM) stores (Baddeley, Citation1992). According to Eenshuistra et al. (Citation2004) and McCabe et al. (Citation2005), inhibitory control uses a significant amount of WM capacity, and successful inhibition is dependent on the WM demands of the task at hand and the available WM resources needed to execute the appropriate response (see also Boucher et al., Citation2021; Spangler & Friedman, Citation2017). WM abilities could therefore determine inhibitory control performance. Indeed, there is evidence to suggest that individuals with better WM functioning are able to use more attentional resources and therefore outperform those with worse WM functioning on tasks that require inhibition (Kane & Engle, Citation2003; Kane et al., Citation2001; Rosen & Engle, Citation1998; Unsworth et al., Citation2004). For example, studies assessing young and middle-aged adults with high versus low WM capacity reported that, compared to participants with high WM capacity, participants with low WM capacity experienced more interference from distractors and were slower and less accurate on measures of both reflexive and volitional inhibition (Kane & Engle, Citation2003; Kane et al., Citation2001; Kiefer et al., Citation2005; Minamoto et al., Citation2010).
In relation to this, it has been suggested that age-related difficulties with inhibitory processes are due to age-related declines in the WM system, rather than impaired inhibitory control in its own right (Eenshuistra et al., Citation2004; McCabe et al., Citation2005), as WM functioning also tends to deteriorate with adult aging (Braver & West, Citation2008; Cansino et al., Citation2013; Hurley & Machado, Citation2018). For example, in Borella et al. (Citation2007) study on age differences in WM and inhibition, they reported that older adults showed worse WM performance than young adults, and furthermore that old-old (75–86 years) participants performed significantly worse than young-old participants (65–74 years), which suggests progressively worsening WM performance with advancing age. In addition, compared to young and young-old adults, old-old adults suffered from significantly more intrusion errors, indicating inhibition difficulties. Regarding potential interrelationships between WM and inhibition systems, age could be a relevant factor (e.g., see Eenshuistra et al., Citation2004). Given the evidence of age-related declines in WM and inhibition systems, it may be that their interrelationships change as we age, presumably with shifts toward stronger interrelationships in older adults due to more limited WM resources, assuming inhibitory functioning depends on the WM system.
Another factor that needs to be considered is that the relationship between WM and inhibition can be domain-dependent. WM can be broken down into subsystems distinguishable by the nature of the content being processed. The most common distinctions in the literature are between verbal and spatial material, with verbal WM linked to the left hemisphere and spatial WM to the right hemisphere (Hurley & Machado, Citation2018; Owen et al., Citation2005; Ramos et al., Citation2020; Ramos & Machado, Citation2021). Regarding associations with inhibitory functioning, different types of WM appear to be linked to different types of inhibition. For example, Castel et al. (Citation2003) discovered that IOR is partially mediated by spatial, but not verbal, WM. Similarly, using a dual-task paradigm, Chou and Yeh (Citation2008) found that IOR was affected by a spatial but not a non-spatial WM secondary task. Clouter et al. (Citation2015) discovered that the Simon effect is affected by both verbal and spatial WM secondary tasks, although the extent of the effect was much larger during the spatial dual-task conditions. In contrast, in McNab et al. (Citation2008) the flanker effect was strongly associated with spatial but not verbal WM. It is therefore important to assess different types of WM to better understand how each of them relates to different inhibitory functions, especially as age-related deficits in WM appear to be domain-specific (Jenkins et al., Citation2000), with performance on spatial WM tasks deteriorating at a faster rate than performance on verbal WM tasks (Hale et al., Citation2011).
Present study
The aims of the current study were to: 1) measure age-related differences in inhibition and WM function with consideration of inhibition type (reflexive/subcortical vs. volitional/cortical) and WM domain (verbal vs. spatial); and 2) explore the relationship between each domain of WM and each type of inhibition, and whether these relationships are influenced by adult aging. By comparing the performance of samples of young and older adults on a range of tasks that differentially tap verbal versus spatial WM and reflexive/subcortical versus volitional/cortical inhibition, the current study aimed to provide a detailed characterization of age-related changes in inhibitory functioning and to shed further light on possible interrelationships between WM and inhibition. To these ends, we tested groups of young and older adults using established paradigms to yield a comprehensive assessment of the effects of healthy aging on inhibitory and WM functioning.
To measure reflexive/subcortical inhibitory functioning, we used fixation offset and IOR tasks. Regarding the FOE, the presence of a stimulus at fixation suppresses the eye movement system, and this stimulus-induced suppression must be overcome in order to make a saccade, resulting in longer latencies to commence movement compared to when no stimulus is present at fixation (Machado & Rafal, Citation2000a, Citation2000b). Weaker inhibition is associated with difficulty initiating saccades during active fixation, resulting in a larger FOE. Regarding IOR, this mechanism makes visual search more efficient by biasing against the return of attention (and gaze) to previously attended locations (Lupianez et al., Citation2006; Machado & Rafal, Citation2004c). Weaker inhibition is associated with a smaller IOR effect, rendering individuals more likely to revisit previously attended locations, which results in a less efficient visual search (Hartley & Kieley, Citation1995).
To measure volitional/cortical inhibitory functioning, we used flanker (Eriksen & Eriksen, Citation1974), Simon (Hommel, Citation2011), and Stroop (Stroop, Citation1935) tasks. In these tasks, selectively ignoring distractors requires participants to inhibit processing of the distracting information (Machado & Devine, Citation2019; Machado et al., Citation2007, Citation2011, Citation2013; Wyatt & Machado, Citation2013a, Citation2013b). The additional time it takes to inhibit processing associated with the distracting information is reflected in the compatibility effect (Castel et al., Citation2007; Colcombe et al., Citation2005), with larger effects indicating weaker inhibitory control (evidenced by the absence of negative compatibility effects at longer distractor-target delays; Machado et al., Citation2009). In addition, we measured the rate of reflexive errors during an antisaccade task as another indicator of cortically mediated inhibitory functioning. This task requires participants to inhibit a reflexive response to a peripheral stimulus and initiate a voluntary saccade to the opposite side. Inhibition of this reflexive response is cortically mediated by the frontal eye fields (Machado & Rafal, Citation2004a; Neggers et al., Citation2012; Rafal et al., Citation2000; Vernet et al., Citation2014), and reflexive errors toward the target indicate a failure of volitional inhibition.
We used forward, backward, and addition digit span tasks (based on the subscale of the Wechsler Memory Scale III) to measure the functioning of the verbal WM system, and forward and backward spatial span tasks to measure the functioning of the spatial WM system (Forsyth et al., Citation2016; White et al., Citation2018, Citation2019, Citation2020). Although performance on forward span tasks is often considered a measure of STM and performance on backward and addition span tasks a measure of WM as these require manipulation of information in STM stores (Hester et al., Citation2004), it has been argued that all of these span tasks tap components of the WM system (albeit to different degrees; Hale et al., Citation2011). For this reason, and to fit with previous research comparing inhibitory functioning in participants with varying levels of WM ability, in the current study we used combined forward and backward scores as a measure of WM ability (Kiefer et al., Citation2005). Consistent with previous findings, we expected performance deficits in older compared to young adults to be greater for the spatial than verbal WM tasks (Hale et al., Citation2011).
Finally, regarding potential links between WM and inhibition, we anticipated that higher WM scores would be associated with better performance in inhibition tasks. In consideration of age-related declines in both WM and inhibitory functioning, we also postulated that functional declines in the relevant systems could impact interrelationships, as evidenced by WM-inhibition interactions that differ by age group. In addition, with respect to aging particularly affecting cortical structures, we postulated that possible interactions between WM and inhibition would be more pronounced on tasks that require volitional as opposed to reflexive inhibition, as both WM and volitional inhibition rely more on cortical structures (Chambers et al., Citation2009; Zaehle et al., Citation2011), whereas reflexive inhibition relies more on subcortical structures (Machado & Rafal, Citation2004a; Sapir et al., Citation1999, Citation2002). provides a visual representation of the proposed conceptual framework and illustrates the categorization of each cognitive measure in the current study.
Figure 1. Conceptual framework for working memory and inhibition system interactions.

Method
Transparency and openness
The University of Otago Human Ethics Committee (#03/171) approved this study, and participants provided written informed consent before taking part. We describe all methods in detail but did not pre-register the study. Code and data are available upon request, in accordance with the ethical approval.
Participants
Sixty neurologically healthy young adults (mean age in years = 21, SD = 3, range = 18–30; 22 males; 55 right-handed; mean years of education = 15) recruited at the University of Otago participated in association with a course. Sixty neurologically healthy older adults (mean age in years = 71, SD = 6, range = 60–88; 22 males; 54 right-handed; mean years of education = 14) recruited from the local community participated and received NZ$20 reimbursement. The young and older adult groups did not significantly differ in years of education (p > .3) or proportion of right-handed participants (p > .7). All older adults scored at least 25 out of 30 on the Mini-Mental State Examination (Folstein et al., Citation1975), suggesting none had dementia. Two additional young adults and four additional older adults participated but were excluded due to computer issues (n = 2), eye-tracking difficulties (n = 3), or a history of neurological issues (n = 1). These six participants will not be discussed further.
Materials and procedure overview
The session took approximately 1.5 h, during which participants performed 12 cognitive tasks that stemmed from seven paradigms: fixation offset (pro- and antisaccade tasks), IOR (central and peripheral saccade signal tasks), flanker, Simon, Stroop, digit span (forward, backward, and addition tasks), and spatial span (forward and backward tasks). The order of the tasks was counterbalanced across participants within each age group with the constraint that the following were yoked together due to methodological overlap: fixation offset and IOR (both involved eye tracking); flanker and Simon (both involved the same stimulus-response mapping); digit span forward, backward, and addition; and spatial span forward and backward. During testing, participants sat 57 cm from a computer monitor in a dimly lit room, with viewing distance maintained via a chinrest (Applied Science Laboratories, Bedford, MA). For all tasks, stimuli were presented against a black background via MATLAB (The MathWorks, Natick, MA) and the Psychophysics Toolbox (Brainard, Citation1997; Pelli, Citation1997). Except for the memory tasks, participants were instructed to respond as quickly as they could without compromising accuracy; all conditions were equally probable, counterbalanced, and randomized; and an error was recorded and a 1000 Hz tone sounded for 500 ms if the reaction time (RT) was less than 80 ms or more than 1500 ms, or an incorrect response was made.
Fixation offset and inhibition of return paradigms
Participants wore a lightweight head-mounted infrared scleral reflectance device (Model 310; Applied Science Laboratories, Bedford, MA), which measured eye position at an approximate rate of 1100 Hz using the Data Acquisition Toolbox (The MathWorks, Natick, MA). An eye movement response was defined as the first time since the onset of the saccade signal that eye velocity exceeded 50°/s for at least 2.5°. To determine the start of the eye movement response, the program searched backward from this point to the point at which eye velocity reached 10°/s. Reaction time (RT) was calculated as the timing delay between saccade signal onset and the start of the eye movement response. An error was recorded and a 1000 Hz tone sounded for 500 ms if the eye position was not within 3° of the fixation dot at the start of the eye movement, RT was less than 80 ms or more than 1500 ms, or the eyes moved in the incorrect direction. In addition to computer analysis of eye movement responses, the experimenter monitored the participant’s eye position overlaid on the task display on a separate screen. During the practice trials, the experimenter provided verbal error feedback, and during the test trials the experimenter marked trials in which the participant blinked or failed to maintain fixation until the target appeared or an external disruption occurred. All trials marked as erroneous by the automated program or rejected by the experimenter were excluded from RT analyses, which included correct responses only. A black screen displayed for 500 ms between trials.
Fixation offset tasks
Two light gray unfilled 1° squares were displayed 8° to the left and right of the center, throughout these tasks. Each trial began with a 0.3° white central fixation dot displayed at center for 500 ms, after which a white cue appeared at center, occluding the fixation dot. The cue was either a single arrowhead pointing toward the impending saccade signal or a double arrowhead pointing in both directions. After 200, 400, or 600 ms, a white filled 0.5° square (saccade signal) appeared in the center of one of the two light gray squares for 1500 ms. In the fixation offset condition, the central cue disappeared when the saccade signal appeared. In the fixation overlap condition, the central cue remained on screen until the end of the trial. illustrates two example trials. There were two tasks: pro- and antisaccade. In the prosaccade task, participants were instructed to move their eyes to the white square. In the antisaccade task, participants were instructed to move their eyes away from the white square to the opposite box. Eye position recording commenced when the cue appeared and continued until the trial ended. Participants completed 10 practice trials followed by 96 test trials of each task (pro- and antisaccade).
Figure 3. Example trial sequences from the fixation offset paradigm.

Inhibition of Return Tasks
Two light gray unfilled 1° squares were displayed 8° to the left and right of the center, throughout these tasks. Each trial began with a 0.3° white central fixation dot displayed for 500 ms, after which the lines of one of the light gray squares thickened and turned white for 200 ms. Following cue offset, the fixation dot remained on screen for a further 700, 900, or 1100 ms before one of the two possible saccade signals appeared, depending on the task: a central arrowhead pointing in the direction to saccade (central saccade signal task) or a peripheral white filled 0.5° square (centered in one of the two light gray squares) indicating the saccade goal (peripheral saccade signal task). illustrates an example trial from each task. For each task, on 50% of the trials the saccade goal was the cued square (cued), and on the other 50% of the trials the saccade goal was the other square (uncued). For each of the two tasks (central and peripheral saccade signals), participants completed 10 practice trials followed by 60 test trials.
Figure 4. Example trial sequences from the inhibition of return paradigm.

Flanker and Simon paradigms
For flanker and Simon, participants responded by pressing a button using a two-button DirectIN Response Box (Empirisoft, New York). To determine the stimulus-response mapping, for each participant, the computer program randomly selected three consonants and assigned two of them to the response buttons (e.g., “F” might be assigned to the left button and “P” to the right button), to be used for flanker and Simon; the stimulus-response mapping was depicted on the screen at the beginning of the practice and test trials. The third consonant, which was not assigned to a response button, could appear as a distractor during the flanker task.
Flanker Task
Each trial began with a 0.3° white central fixation dot displayed at center for 500 ms, after which two white uppercase consonants (Arial font, 1° in height) appeared simultaneously: a target consonant in the center of the screen (occluding the fixation dot) and a distractor consonant 1° above or below the target (to avoid effects of spatial compatibility with the response buttons; Machado & Franz, Citation2004). Participants were instructed to indicate the identity of the central letter by pressing the assigned button. The target could be either of the consonants assigned to a response button, and the distractor could be any one of the three consonants. Thus, the identity of the distractor could match the identity of the target (compatible), not match the identity of the target and not be associated with a response (neutral), or not match the identity of the target and be associated with the incorrect response (incompatible). shows examples displays. A black screen displayed for 1000 ms between trials. Participants completed 10 practice trials followed by 84 test trials.
Figure 5. Example displays illustrating the three conditions in the flanker paradigm.

Simon Task
Each trial began with a 0.3° white central fixation dot displayed at the center for 500 ms, after which a white uppercase consonant (Arial font, 1° in height) appeared either in the center of the screen, 2° to the left of center, or 2° to the right. Participants were instructed to indicate the identity of this letter by pressing the assigned button. The position of the consonant varied randomly in relation to the side of the correct response, thus making its position either compatible, neutral (central letter), or incompatible with the side of the correct response. shows examples displays. A black screen displayed for 1000 ms between trials. Participants completed 10 practice trials followed by 84 test trials.
Figure 6. Example displays illustrating the three conditions in the Simon paradigm.

Stroop paradigm
Each trial began with a 0.3° white central fixation dot displayed at the center for 500 ms, after which a word (Arial font, 1° in height) appeared in the center of the screen for 2000 ms. Participants were instructed to verbally report the color of the font. The possible font colors were red, orange, yellow, green, blue, and purple. The word could be a color word (“RED,” “ORANGE,” “YELLOW,” “GREEN,” “BLUE,” or “PURPLE”) or a neutral word (“ONE,” “ELEVEN,” “TWELVE,” “SEVEN,” “FOUR,” or “NINETY”), such that the word was compatible (e.g., the word “BLUE” in blue font), incompatible (e.g., the word “RED” in blue font), or neutral (e.g., the word “ONE” in blue font) with the color of the font. shows examples displays. When participants responded, a Cedrus SV-1 Smart Voice Key and I/O recorded RT; the experimenter entered the first letter of the participant’s response (r, o, y, g, b, or p) or “e” if an error occurred (such as a cough triggered the voice key). A black screen displayed for 500 ms between trials. Participants completed 5 practice trials followed by 72 test trials.
Figure 7. Example displays illustrating the three conditions in the stroop paradigm.

Digit span paradigm
Based on the digit span subscale of the Wechsler Memory Scale III, the three tasks (forward, backward, and addition) differed only in the response instructions and the specific digits in the sequences. Each sequence began with a 0.3° white central fixation dot displayed at the center for 500 ms, after which a sequence of white digits (Arial font, 1° in height) appeared one after the other in the center of the screen. Each digit in the sequence appeared for 1 s with no interstimulus interval. At the end of each sequence, a 400 Hz tone sounded for 500 ms and the white fixation dot reappeared, cueing participants to respond verbally. Participants were instructed for the forward task to report the digits in the same order in which they appeared, for the backward task to report the digits in the reverse order to which they appeared, and for the addition task to report the sum total of all digits in the sequence. After the experimenter keyed in the response, a black screen displayed for 1000 ms. Two sequences of each length were presented, starting with a length of two digits. If participants responded correctly to at least one of the sequences of a particular length, the sequence length increased by one digit. If participants responded incorrectly to both of the sequences of a particular length, the task terminated. The maximum sequence length was nine digits.
Spatial span paradigm
Based on the Corsi block-tapping test (Corsi, Citation1972; Kessels et al., Citation2000) the two tasks (forward and backward) differed only in the response instructions and the specific sequences. The display (see ) consisted of nine gray filled 4° squares positioned as per Kessels et al. (Citation2000). Prior to each sequence, a 400 Hz tone sounded for 500 ms, and then a subset of the squares turned white for 1 s, one at a time, with no interstimulus interval. At the end of each sequence, a 400 Hz tone sounded for 500 ms, cueing the participant to respond by touching the relevant squares using a KeyTech MagicTouch touch screen; squares turned white when touched. Participants were instructed for the forward task to touch the squares in the same order in which they lit up, and for the backward task to touch the squares in the reverse order to which they lit up. After the participant responded, a black screen was displayed for 1000 ms. Two sequences of each length were presented, with the first comprising two squares. If participants responded correctly to at least one of the two sequences of a particular length, the sequence length increased by one square, else the task terminated. The maximum sequence length was nine squares.
Figure 8. Organization of the squares in the spatial span paradigm.

Statistical analyses
The measured variables of interest in the inhibition tasks were median RT for correct responses and, for the antisaccade task only, percentage of reflexive errors. The measured variable of interest in the verbal and spatial WM tasks was score, which was calculated by multiplying span (the longest sequence length for which the participant responded correctly to at least one of the two sequences; maximum = 9) by the number of correctly recalled sequences (maximum = 16), resulting in a maximum score of 144 (Kessels et al., Citation2000).
All statistical analyses were performed using SPSS version 25.0. To investigate age-group differences in inhibition, for fixation offset, IOR, flanker, Simon, and Stroop median correct RTs, we conducted mixed analyses of variance (ANOVAs) with trial type (offset, overlap or uncued, cued or compatible, incompatible) as the within-subjects variable and age group (young, old) as the between-subjects variable, done separately for each task, and for antisaccade reflexive errors we conducted a univariate ANOVA with age group (young, old) as the between-subjects variable. We also subjected the correct RT data to log transformations to help confirm the observed aging effects (see Bush et al., Citation1993; Faust et al., Citation1999). To investigate age-group differences in WM function, for each WM task we conducted a one-way ANOVA with age group as the between-subjects variable. Analyses in G*Power (Faul et al., Citation2007, Citation2009) indicated that with a sample size of 120 we had 80% power to detect medium-sized (ηp2 ≥0.06) main effects of age group and trial type, as well as Trial Type × Age Group interaction effects, which could be expected based on past research utilizing similar tasks to investigate inhibition and WM, as well as age differences in these functions (Andres et al., Citation2008; Bos & Machado, Citation2013; Castel et al., Citation2007; Hester et al., Citation2004; Kemtes & Allen, Citation2008). The effect sizes were interpreted as small (ηp2 ≥ .01), medium (ηp2 ≥ .06), and large (ηp2 ≥ .14), as is typical in the literature based on Cohen (Citation1988) (see Richardson, Citation2011).
To explore WM as a variable of interest regarding performance on the inhibition tasks, we first generated for each age group composite scores for verbal and spatial WM by averaging across tasks (Song et al., Citation2013), resulting in a verbal WM composite score (forward + backward) and a spatial WM composite score (forward + backward). We did not include digit addition score in the verbal WM composite as the results of the initial age-group comparisons suggested that crystalized intelligence (e.g., knowledge, ability, and skills) was likely confounded with age group and had a strong influence on scores (see Discussion section for further information about this). Based on recommendations by Schneider et al. (Citation2015), we grand-mean centered each WM composite score by subtracting the group mean score from each participant’s individual score () before entering it as a covariate (Schneider et al., Citation2015). For correct RTs, we conducted repeated-measures ANCOVAs with trial type as a within-subjects variable, and for antisaccade reflexive errors we conducted univariate ANCOVAs. ANCOVAs were conducted separately for young and older adults, as WM scores differed between the young and older adults (i.e., the data did not meet the assumption that the expected value of the covariate at each level of the between-subjects factor is the same; Schneider et al., Citation2015). For repeated-measures ANCOVAs, interaction effects indicate that the slope of the line relating the covariate (e.g., verbal WM composite score) to the dependent measure (e.g., Stroop RT) depends on the level of the within-subjects factor (e.g., compatible vs. incompatible; Schneider et al., Citation2015). Analyses in G*Power (Faul et al., Citation2007, Citation2009) indicated that with sample sizes of 60, we had 80% power to detect medium-to-large effect sizes (ηp2 ≥0.11), which could be expected based on past research (Borella et al., Citation2007; Kiefer et al., Citation2005; Unsworth et al., Citation2004). Effects reaching statistical significance (p < .05) or showing at least medium effect sizes (ηp2 ≥0.06) were explored by visually inspecting scatter graphs with a regression line plotting the relationship between the relevant WM composite score and the relevant inhibition task measure (for RTs, each trial type was plotted separately), as recommended by Schneider et al. (Citation2015).
Results
Mean of the median correct RTs and error percentages for the inhibition measures (except antisaccade reflexive errors) are shown in . show a summary of the mixed and one-way ANOVAs, respectively. also shows descriptive statistics for antisaccade reflexive errors and WM scores.
Table 1. Reaction times (ms) and excluded trials (%), separated by inhibition task and trial type, in young and older adults.
Table 2. A summary of mixed ANOVAs examining the effect of age groups (young versus older adults), trial types, and their interactions on raw and log-transformed reaction times in the inhibition tasks.
Table 3. A summary of one-way ANOVAs examining the effect of age groups (young versus older adults) on antisaccade reflexive errors and working memory scores.
Inhibition
Mixed ANOVAs on the raw RT data indicated large age-group effects (p < .001, ηp2 ≥0.25),medium-to-large trial-type effects (p ≤ .003, ηp2 ≥ 0.07), and interaction effects (p ≤ .029, ηp2 ≥ 0.04). After log transformations, all of the main effects and interaction effects remained significant: age group main effects (p < .001, ηp2 ≥0.28) and trial type main effects (p ≤ .009, ηp2 ≥ 0.06), as well as the Age Group × Trial Type interaction effects (p ≤ .041, ηp2 ≥0.04), thus confirming the observed age-group effects (see Bush et al., Citation1993; Faust et al., Citation1999). The main effects of trial type reflect slower RTs on trials associated with inhibition (i.e., overlap for fixation offset, cued for IOR, and incompatible for flanker, Simon, and Stroop). The significant Age Group × Trial Type interaction effects for FOE and IOR reflect older adults exhibiting considerably smaller FOEs and larger IOR than young adults (see ), indicating stronger reflexive inhibition in older adults. In contrast, the significant Age Group × Trial Type interaction effects for flanker, Simon, and Stroop RT and the significant main effect of age group for antisaccade reflexive errors reflect older adults exhibiting considerably greater slowing on incompatible relative to compatible trials (i.e., larger compatibility effects) and a higher percentage of reflexive errors on the antisaccade task compared to young adults, collectively indicating significantly weaker volitional inhibition in the older adults. Taken together, these results indicate stronger reflexive inhibition and weaker volitional inhibition in older adults.
Working memory
One-way ANOVAs investigating the main effect of age group on WM measures produced mixed results. The verbal WM tasks showed no significant effect of age group on digit forward score (p = .100, ηp2 = 0.02), but there were significant effects of age group on digit backward score (p = .008, ηp2 = 0.06) and digit addition score (p = .002, ηp2 = 0.08). These, respectively, reflect lower backward, but higher addition, scores in older compared to young adults. Regarding spatial WM, the significant effects of age group on both forward (p < .001, ηp2 = 0.39) and backward (p < .001, ηp2 = 0.28) scores reflect the considerably lower scores in older compared to young adults.
Inhibition and working memory
The results of the repeated-measures ANCOVAs exploring WM as a variable of interest regarding correct RT performance on fixation offset, IOR, flanker, Simon, and Stroop are shown in . Note that verbal and spatial WM were considered separately, and that we analyzed raw RTs (rather than transformed) as ANCOVAs were conducted separately for young and older adults due to group differences in WM scores (Schneider et al., Citation2015). As can be seen in , there was very limited evidence of WM being a variable of interest in inhibitory functioning in either age group. There was a significant Trial Type × Verbal Working Memory interaction in older adults for fixation offset antisaccade RT (p = .017, ηp2 = 0.09). However, visual inspection of scatter graphs, with a regression line plotting the relationship between verbal WM scores and antisaccade RTs (done separately for offset and overlap trials) showed that, although higher verbal WM scores were weakly associated with faster RTs for both trial types, the contribution of verbal WM to antisaccade RTs was greater on offset trials (i.e., the slope was steeper, r2 = .053) compared to overlap trials (r2 = .008). Two other interactions showed medium effect sizes, both also in the older adults (p = .066, ηp2 = 0.06, in both cases), one reflecting a small tendency for higher verbal WM scores to be associated with faster RTs on incompatible (r2 = .024) but not compatible (r2 = .000) trials of the Stroop task, and the other reflecting a small tendency for higher spatial WM scores to be associated with faster RTs on cued (r2 = .027) but not uncued (r2 = .000) trials of the peripheral saccade signal IOR task. No other interactions have reached statistical significance or shown at least medium effect size in either age group (p ≥ .073, ηp2 ≤0.05).
Table 4. A summary of repeated-measures ANCOVAs exploring the contribution of working memory to performance on measures of inhibition in each age group.
Results of the univariate ANCOVAs exploring WM as a variable of interest regarding antisaccade reflexive errors showed in the young adults a significant effect of spatial WM score on antisaccade reflexive errors, F(1, 58) = 5.85, p = .019, ηp2 = 0.09, and visual inspection of a scatter graph indicated higher spatial WM scores were associated with fewer reflexive errors (r2 = .092). The young adults also showed a medium effect size for higher verbal WM scores to be associated with fewer reflexive errors (p = .065, ηp2 = 0.06, r2 = .057). In contrast, the results in the older adults showed no evidence linking verbal or spatial WM scores to antisaccade reflexive errors (p ≥ .478, ηp2 ≤0.01).
Discussion
The aims of the current study were to investigate age-related differences in inhibition and WM function with consideration of inhibition type (reflexive/subcortical vs. volitional/cortical) and WM domain (verbal vs. spatial) and to characterize relationships between each WM domain and inhibition type in both young and older adults. Compared to young adults, older adults showed significantly stronger reflexive inhibition and significantly weaker volitional inhibition. Regarding the WM tasks, aside from mental arithmetic performance, older adults tended to show worse performance, especially for the spatial tasks, which showed quite large effect sizes for age-group differences. Surprisingly, we found very limited evidence of relationships between WM and inhibition, with only one significant effect (in the young adults) supporting WM ability as a determinate of inhibition performance. These results will be discussed in more detail in subsequent sections.
Age-group differences in inhibition
Our results indicate age-related increases in reflexive inhibition, as evidenced by FOE and IOR, and age-related decrements in volitional inhibition demonstrated across several measures (antisaccade reflexive errors and flanker, Simon, and Stroop effects). Analyses of the transformed data confirmed the observed age–group interaction effects (see Bush et al., Citation1993; Faust et al., Citation1999). These patterns of results are consistent with previous studies reporting, in older compared to young adults, a smaller FOE (Bos & Machado, Citation2013), stronger IOR (McLaughlin et al., Citation2010; Poliakoff et al., Citation2007), more antisaccade reflexive errors (Brett & Machado, Citation2017), and larger flanker (Machado et al., Citation2009; Zeef & Kok, Citation1993; Zeef et al., Citation1996), Simon (Castel et al., Citation2007; van der Lubbe & Verleger, Citation2002), and Stroop effects (Spieler et al., Citation1996; Troyer et al., Citation2006). The observation of these age-group differences in the current study but not in some past studies in most cases may relate to the larger sample sizes in the current study (60 versus 20 or fewer per age group in past studies), although differences in the details of the tasks utilized to measure inhibitory functioning may be a factor as well and may help explain the lack of age-related inhibitory deficits in some meta-analyses (e.g., Rey-Mermet & Gade, Citation2018) as previously argued in relation to WM tasks (Hurley & Machado, Citation2018). It should be noted that in the current study the age-group differences in inhibition occurred in the absence of older adults exhibiting lower error rates, thus indicating that the older adults did not adopt a more cautious strategy (in contrast to Vallesi et al., Citation2021).
The observed combination of weaker volitional inhibition and stronger reflexive inhibition suggests that age-related deterioration of cortical structures may allow subcortical structures to operate under a reduced level of cortical control. For example, the superior colliculus is a subcortical structure that mediates reflexive oculomotor responses to both central visual signals, which stimulate the fixation reflex to help anchor the eyes, and peripheral visual signals, which inhibit the fixation reflex (Munoz & Istvan, Citation1998; Munoz & Wurtz, Citation1993). These subcortical mechanisms allow for efficient reflexive responding, but they require cortical areas to impose control over them to implement goal-directed behavior (Machado & Rafal, Citation2004a, Citation2004b; van Koningsbruggen et al., Citation2009). Age-related deterioration of cortical structures may allow the superior colliculus to operate under less control, thus resulting in stronger attentional and/or oculomotor capture by peripheral stimuli and weaker volitional inhibition of prepotent responses (Machado & Rafal, Citation2004b; Nieuwenhuis et al., Citation2000). Indeed, in addition to the present findings, there is evidence that older adults experience stronger attentional capture by peripheral cues (Juola et al., Citation2000), they are less successful at suppressing a reflexive eye movement toward a sudden stimulus in the periphery (Brett & Machado, Citation2017; Chen & Machado, Citation2016; Olincy et al., Citation1997), and have more difficulty ignoring spatial information triggered by a target located in the periphery (Castel et al., Citation2007). This suggests that older adults may experience hyper-responsiveness to peripheral visual stimulation.
Age-group differences in working memory
Regarding verbal WM, digit forward scores were not significantly worse in older compared to young adults, but older adults had significantly lower scores for digits backward. In contrast, older adults had significantly higher digit addition scores, which we suspect likely reflect factors unrelated to WM (namely, crystallized intelligence, which has been shown to remain stable or even improve with healthy aging; Salthouse, Citation2012). In relation to this, a cohort effect may have contributed to the age-group differences in digit addition performance (Harada et al., Citation2013), as the older adults were more likely to have more experience adding numbers to their heads due to more training in school followed by many years of practice. By comparison, the young adults may not have received as much mental arithmetic training or engaged in as much practice, due in part to greater availability and higher reliance on electronic calculators (e.g., on their mobile phones). These conjectures fit with reports in the United States of America (Geary & Lin, Citation1998; Geary & Wiley, Citation1991; Geary et al., Citation1993).
Regarding the spatial WM tasks, compared to young adults, older adults had substantially lower scores for both forward and backward recall. In addition, as predicted, the effect sizes for age differences in WM were much larger for the spatial tasks than for the equivalent verbal tasks (digit forward and backward), indicating greater age-related deficits in spatial WM performance. These findings support those of previous studies reporting a more pronounced age-related decline in spatial compared to verbal domains (e.g., Cansino et al., Citation2013; Hale et al., Citation2011), which interestingly has also been reported in association with Parkinson’s disease (Ramos & Machado, Citation2021).
Inhibition and working memory
Despite robust age-group differences in measures of both inhibition and WM, we found very limited evidence that WM abilities influenced performance on the tasks measuring inhibition. Of the eight inhibition measures (four reflexive/subcortical and four volitional/cortical) considered in each age group, very few showed the predicted relationships with WM measures (verbal or spatial). In the older adults, of the 16 analyses run, one yielded a statistically significant effect and two yielded medium effect sizes that did not reach significance (p = .066 in both cases). With regard to the latter, higher verbal WM scores tended to be associated with faster RTs on incompatible (but not compatible) Stroop trials, which fits with the conceptual framework regarding verbal WM influencing volitional inhibition (see ); however, the association was fairly weak (r2 = .024) and limited to this measure of volitional inhibition. In addition, higher spatial WM scores tended to be associated with faster RTs on cued (but not uncued) trials of the peripheral saccade signal IOR task, which fits with the conceptual framework regarding spatial WM influencing reflexive inhibition; however, again the association was fairly weak (r2 = .027) and limited to this measure of reflexive inhibition. The only statistically significant evidence in the older adults linking WM to inhibition task performance stems from higher verbal WM scores being associated with faster fixation offset antisaccade RTs, but this relationship was stronger on offset trials (r2 = .053) and bordered on negligible on overlap trials (r2 = .008), which does not fit with the model as it is the overlap trials that require inhibition to overcome the effects of the fixation stimulus in order to initiate the saccade. In the young adults, of the 16 analyses run, one yielded a statistically significant effect and one yielded a medium effect size that did not reach significance (p = .065). Respectively, these provided evidence for an association between higher spatial and verbal WM scores and fewer antisaccade reflexive errors, consistent with the model linking verbal and spatial WM to volitional inhibition; however, none of the other measures yielded evidence consistent with the conceptual framework regarding WM influencing inhibition in young adults.
Regarding the tendency in the older adults for spatial WM scores to be associated with faster cued peripheral saccade signal RTs (IOR paradigm), although consistent with the model depicted in , this result was somewhat surprising in the context of the lack of WM links with volitional inhibition measures, as IOR is a reflexive subcortically mediated mechanism (Sapir et al., Citation1999, Citation2002) and we expected to see effects of WM ability primarily on volitionally mediated inhibition in relation to both relying on cortical structures (Chambers et al., Citation2009; Zaehle et al., Citation2011). Nevertheless, this finding is consistent with the concept that inferior cortical functioning is associated with hyperactive subcortical functioning, and fits with previous reports that IOR is partially mediated by spatial WM (Castel et al., Citation2003; Chou & Yeh, Citation2008).
The only link in the older adults between WM and volitional inhibition that reached the threshold of an at least medium effect size stemmed from the Stroop task: higher verbal WM scores tended to be associated with faster correct RTs on incompatible trials. As reading becomes increasingly automatic over the years, which can in part account for larger Stroop effects in older than young adults (Lien et al., Citation2006), older adults with poorer verbal WM ability may have found it particularly difficult to inhibit reading the words due to having fewer resources available for conflict resolution, relative to older adults with superior verbal WM abilities (Aschenbrenner & Balota, Citation2015). Greater automaticity of word reading among older adults might help explain why evidence of a relationship with WM ability emerged for this volitional inhibition measure but not the others. The indication that verbal WM may be linked to Stroop performance more so in older than young adults fits with a previous study reporting on a lifespan sample (Aschenbrenner & Balota, Citation2015; see also McCabe et al., Citation2005). However, the lack of a relationship in young adults contrasts with past research linking superior verbal WM performance to less interference from incompatible Stroop words in university students (Hutchison, Citation2011; Kane & Engle, Citation2003; Long & Prat, Citation2002; Meier & Kane, Citation2013). This difference does not appear to be related to sample size as the current study involved a larger sample than some of these previous studies. The discrepancy may relate to differences in the WM tests and/or the Stroop tests. In contrast to the current study, in assessing WM these previous studies interjected a second task (reading or operation/sentence/symmetry judgments) between items in the memory set, and in assessing Stroop performance they manipulated the frequency of compatible versus incompatible trials (mostly compatible or mostly incompatible), which was a determinant of the WM-inhibition links and thus potentially the main factor underpinning the different outcomes.
The findings in the young adults linking higher WM scores to fewer reflexive errors during the antisaccade task fit with past reports in young adults (Kane et al., Citation2001; Unsworth et al., Citation2004). However, there was virtually no evidence of links between WM scores and reflexive errors during the antisaccade task in the older adults, which goes against what one would expect if inhibitory control depended on deteriorating WM systems (see Eenshuistra et al., Citation2004). That is, given that older adults showed both poorer WM performance and more reflexive errors during the antisaccade task, based on the model illustrated in one would expect the latter to be linked to the former in older adults. The absence of this link calls into question the concept that inhibitory functioning depends on WM systems.
Overall, our findings largely fail to support previous conjectures that WM mediates performance on tasks that require inhibitory control (e.g., Castel et al., Citation2003; Kramer et al., Citation2001; Roberts et al., Citation1994) and that participants with low WM capacity experience more interference from distractors and are slower and less accurate on inhibition tasks than participants with high WM capacity (e.g., Kane & Engle, Citation2003; Kane et al., Citation2001; Long & Prat, Citation2002; Minamoto et al., Citation2010). The discrepant findings likely reflect differences in the methodological details of the tasks used to assess WM and inhibition. Further to key methodological differences already discussed in relation to past Stroop studies in young adults observing links with verbal WM not observed here, many of the previous studies reporting WM-inhibition links used a combined task that tapped both WM and inhibition (e.g., Blair et al., Citation2011; Borella et al., Citation2007; Castel et al., Citation2003; Chou & Yeh, Citation2008; Clouter et al., Citation2015; Roberts et al., Citation1994) else intermixed the WM and inhibition tasks and measures (Borella et al., Citation2008). Consequently, in contrast to the current study, the results of these studies cannot speak to whether inhibition and WM systems function interdependently when the two systems are not expressly co-activated.
Limitations and suggestions for future research
One limitation of the current study relates to the assumptions of the categorization scheme. For example, tasks categorized as indexing reflexive/subcortical inhibition are not purely subcortically mediated and performance relies on many factors beyond inhibition. As an example of the latter, the magnitude of a flanker effect reflects not only the extent to which distractor processing was inhibited but also the extent to which target processing was facilitated (Wyatt & Machado, Citation2013a). Thus, differences in effects may reflect differences in other task-relevant functions, which would have added extraneous variation that could have led to erroneous conclusions regarding the profile of age-related decline and may have compromised our ability to detect WM-inhibition relationships. Covariance matrices for inhibition measures supported the grouping of the variables categorized as volitional/cortical inhibition, but only partially supported the grouping of the variables categorized as reflexive/subcortical inhibition (see Supplementary Material A). In a related note, the age-related deterioration of cortical regions is not uniform, and many subcortical regions are also adversely affected (Sele et al., Citation2020; Vinke et al., Citation2018). Although we used the broad terms cortical and subcortical, the former was primarily in reference to the prefrontal cortex, which is known to atrophy with adult aging (e.g., see Hedden & Gabrieli, Citation2004) and the latter in reference to the superior colliculi, which we have presumed to undergo less age-related atrophy, however this is based on limited data (Aanerud et al., Citation2012; Bellot et al., Citation2016; Luft et al., Citation1999; Walhovd et al., Citation2011). Moreover, although in the current study all of the inhibition measures categorized as volitional/cortical indicated reduced inhibition in the older adults, it should be noted that different cortical inhibitory control processes rely on distinct neural networks (e.g., Zhang et al., Citation2017) and, in relation to this, the different volitional/cortical inhibition measures in the current study are not assumed to be tapping a unitary inhibition construct, consistent with past findings (Rey-Mermet & Gade, Citation2018; Rey-Mermet et al., Citation2018).
Another limitation of the current study relates to all of the older participants being grouped together despite their ages spanning a fairly wide range (60–88 years). According to Persad et al. (Citation2002), such designs fail to account for the continued age-related decline among older adults (see also Borella et al., Citation2007). Previous studies classifying older participants as “young-old” (60–74 years) and “old-old” (75 years and older) found that old-old participants performed significantly worse on a range of neuropsychological tasks compared to their younger counterparts (Christensen et al., Citation1994; Palladino & De Beni, Citation1999; Persad et al., Citation2002). Unfortunately, in the current study, we were unable to separate the older adults into subgroups as 48 of the 60 older adults were aged 74 or under. Future research seeking to characterize age-related differences in WM and inhibitory functioning should consider utilizing a study design that allows for the comparison of young, young-old, and old-old adults. A final limitation of the current study worthy of note relates to the sample size. Although we had relatively large sample sizes and power analyses indicated that we had 80% power to detect medium-to-large effects (which could be expected based on previous studies), larger samples would nevertheless have been preferable to improve the power of the current study. While it seems unlikely that adding more participants would change the conclusions, given that in some instances the direction of effects opposed the prediction that inhibitory functioning depends on WM ability, inclusion of larger sample sizes would allow for the use of more sophisticated statistical models, such as structural equation modeling (Westland, Citation2010). This could enable more direct testing of the conceptual framework proposed here, resulting in much stronger conclusions.
Conclusions
In summary, the results reported here support age-related increases in reflexive inhibition (based on the fixation offset effect and inhibition of return) and age-related decrements in both volitional inhibition (based on performance on the antisaccade, Stroop, flanker, and Simon paradigms) and WM performance (verbal and spatial). This pattern of results provides novel evidence suggestive of an age-related shift in the balance of power from cortical to subcortical structures, as indicated by weaker volitional inhibition and poorer WM performance but stronger reflexive inhibition in older than young adults. This behavioral evidence of hyperactive subcortical functioning presumably reflects age-related deterioration of cortical structures, leaving subcortical structures to operate under less cortically orchestrated control. Functionally, this would result in older adults behaving in a relatively more reflexive and less volitional manner, effectively losing some of the earlier life gains in strategic control (e.g., Fischer et al., Citation1997; Munoz et al., Citation1998); however, it should be noted that the trajectory of these shifts varies among individuals and much can be done via lifestyle choices to promote a more favorable trajectory (Machado, Citation2021). Regarding potential links between WM ability and inhibition, in contrast to current theory, our findings suggest that inhibition and WM are largely independent and that WM limitations cannot account for age-related declines in inhibitory functioning.
Acknowledgments
The authors wish to thank Andrew Webb for assisting with preparing the software used for cognitive testing and Natalie Wyatt for assisting with data collection.
Disclosure statement
No potential conflict of interest was reported by the authors.
Data availability statement
Data and code will be made available upon request.
Additional information
Funding
References
- Aanerud, J., Borghammer, P., Chakravarty, M. M., Vang, K., Rodell, A. B., Jonsdottir, K. Y., Gjedde, A., Gjedde, A. & Gjedde, A. (2012). Brain energy metabolism and blood flow differences in healthy aging. Journal of Cerebral Blood Flow and Metabolism, 32(7), 1177–1187. https://doi.org/10.1038/jcbfm.2012.18
- Andres, P., Guerrini, C., Phillips, L. H., & Perfect, T. J. (2008). Differential effects of aging on executive and automatic inhibition. Developmental Neuropsychology, 33(2), 101–123. https://doi.org/10.1080/87565640701884212
- Andrés, P., Van der Linden, M., & Parmentier, F. B. (2004). Directed forgetting in working memory: Age-related differences. Memory, 12(2), 248–256. https://doi.org/10.1080/09658210244000612
- Anstey, K. J., Wood, J., Kerr, G., Caldwell, H., & Lord, S. R. (2009). Different cognitive profiles for single compared with recurrent fallers without dementia. Neuropsychology, 23(4), 500–508. https://doi.org/10.1037/a0015389
- Anstey, K. J., Wood, J., Lord, S., & Walker, J. G. (2005). Cognitive, sensory and physical factors enabling driving safety in older adults. Clinical Psychology Review, 25(1), 45–65. https://doi.org/10.1016/j.cpr.2004.07.008
- Aschenbrenner, A. J., & Balota, D. A. (2015). Interactive effects of working memory and trial history on stroop interference in cognitively healthy aging. Psychology and Aging, 30(1), 1–8. https://doi.org/10.1037/pag0000012
- Baddeley, A. (1992). Working memory. Science, 255(5044), 556–560. https://doi.org/10.1126/science.1736359
- Bellot, E., Coizet, V., Warnking, J., Knoblauch, K., Moro, E., & Dojat, M. (2016). Effects of aging on low luminance contrast processing in humans. Neuroimage, 139, 415–426. https://doi.org/10.1016/j.neuroimage.2016.06.051
- Blair, M., Vadaga, K. K., Shuchat, J., & Li, K. Z. H. (2011). The role of age and inhibitory efficiency in working memory processing and storage components. The Quarterly Journal of Experimental Psychology, 64(6), 1157–1172. https://doi.org/10.1080/17470218.2010.540670
- Borella, E., Carretti, B., Cornoldi, C., & De Beni, R. (2007). Working memory, control of interference and everyday experience of thought interference: When age makes the difference. Aging Clinical and Experimental Research, 19(3), 200–206. https://doi.org/10.1007/BF03324690
- Borella, E., Carretti, B., & De Beni, R. (2008). Working memory and inhibition across the adult lifespan. Acta Psychologica, 128(1), 33–44. https://doi.org/10.1016/j.actpsy.2007.09.008
- Borella, E., Delaloye, C., Lecerf, T., Renaud, O., & de Ribaupierre, A. (2009). Do age differences between young and older adults in inhibitory tasks depend on the degree of activation of information? The European Journal of Cognitive Psychology, 21(2–3), 445–472. https://doi.org/10.1080/09541440802613997
- Bos, H., & Machado, L. (2013). Aging delays strategic modulation of the fixation reflex. Psychology and Aging, 28(3), 796–801. https://doi.org/10.1037/a0032015
- Boucher, L., Viparina, B., & Collins, W. M. (2021). Working memory load selectively influences response inhibition in a stop signal task. Psychological Reports, 124(3), 1268–1281. https://doi.org/10.1177/0033294120928271
- Brainard, D. H. (1997). The psychophysics toolbox. Spatial Vision, 10(4), 433–436. https://doi.org/10.1163/156856897X00357
- Braver, T. S., & West, R. (2008). Working memory, executive control, and aging. In F. I. M. Craik & T. A. Salthouse (Eds.), The handbook of aging and cognition (pp. 311–372). Psychology Press.
- Brett, C. H. R., & Machado, L. (2017). Manual versus saccadic assessment of cognitive inhibition and switching in young and older adults. Psychological Assessment, 29(11), 1420–1425. https://doi.org/10.1037/pas0000453
- Bugg, J. M., DeLosh, E. L., Davalos, D. B., & Davis, H. P. (2007). Age differences in Stroop interference: Contributions of general slowing and task-specific deficits. Neuropsychology, Development, and Cognition Section B, Aging, Neuropsychology and Cognition, 14(2), 155–167. https://doi.org/10.1080/138255891007065
- Bush, L. K., Hess, U., & Wolford, G. (1993). Transformations for within-subject designs: A monte carlo investigation. Psychological Bulletin, 113(3), 566–579. https://doi.org/10.1037/0033-2909.113.3.566
- Cansino, S., Hernandez-Ramos, E., Estrada-Manilla, C., Torres-Trejo, F., Martinez-Galindo, J. G., Ayala-Hernandez, M., Rodriguez-Ortiz, M. D., Rodriguez-Ortiz, M. D. & Rodriguez-Ortiz, M. D. (2013). The decline of verbal and visuospatial working memory across the adult life span. AGE, 35(6), 2283–2302. https://doi.org/10.1007/s11357-013-9531-1
- Castel, A. D., Balota, D. A., Hutchison, K. A., Logan, J. M., & Yap, M. J. (2007). Spatial attention and response control in healthy younger and older adults and individuals with Alzheimer’s disease: Evidence for disproportionate selection impairments in the Simon task. Neuropsychology, 21(2), 170–182. https://doi.org/10.1037/0894-4105.21.2.170
- Castel, A. D., Pratt, J., & Craik, F. I. (2003). The role of spatial working memory in inhibition of return: Evidence from divided attention tasks. Perception & Psychophysics, 65(6), 970–981. https://doi.org/10.3758/BF03194827
- Chambers, C. D., Garavan, H., & Bellgrove, M. A. (2009). Insights into the neural basis of response inhibition from cognitive and clinical neuroscience. Neuroscience & Biobehavioral Reviews, 33(5), 631–646. https://doi.org/10.1016/j.neubiorev.2008.08.016
- Chen, P. L., & Machado, L. (2016). Age-related deficits in voluntary control over saccadic eye movements: Consideration of electrical brain stimulation as a therapeutic strategy. Neurobiology of Aging, 41, 53–63. https://doi.org/10.1016/j.neurobiolaging.2016.02.010
- Chen, P. L., & Machado, L. (2017). Developing clinically practical transcranial direct current stimulation protocols to improve saccadic eye movement control. Journal of Eye Movement Research, 10(3). https://doi.org/10.16910/jemr.10.3.5
- Chou, W. -L., & Yeh, S. -L. (2008). Location-and object-based inhibition of return are affected by different kinds of working memory. The Quarterly Journal of Experimental Psychology, 61(12), 1761–1768. https://doi.org/10.1080/17470210802194308
- Christensen, H., Mackinnon, A., Jorm, A. F., Henderson, A. S., Scott, L. R., & Korten, A. E. (1994). Age differences and interindividual variation in cognition in community-dwelling elderly. Psychology and Aging, 9(3), 381–390. https://doi.org/10.1037/0882-7974.9.3.381
- Clouter, A., Wilson, R., Allen, S., Klein, R. M., & Eskes, G. A. (2015). The influence of verbal and spatial working memory load on the time course of the Simon effect. Journal of Experimental Psychology Human Perception and Performance, 41(2), 342. https://doi.org/10.1037/a0038715
- Cohen, J. (1988). Statistical power analysis for the behavioral sciences (2nd ed.). Lawrence Earlbaum Associates.
- Colcombe, S. J., Kramer, A. F., Erickson, K. I., & Scalf, P. (2005). The implications of cortical recruitment and brain morphology for individual differences in inhibitory function in aging humans. Psychology and Aging, 20(3), 363–375. https://doi.org/10.1037/0882-7974.20.3.363
- Conway, A. R., Tuholski, S. W., Shisler, R. J., & Engle, R. W. (1999). The effect of memory load on negative priming: An individual differences investigation. Memory & Cognition, 27(6), 1042–1050. https://doi.org/10.3758/BF03201233
- Corsi, P. M. (1972). Human memory and the medial temporal region of the brain [ Doctoral thesis, McGill University]. https://escholarship.mcgill.ca/downloads/4m90dw30g.
- Easton, T. A. (1972). On the normal use of reflexes. American Scientist, 60(5), 591–599.
- Eenshuistra, R. M., Ridderinkhof, K. R., & van der Molen, M. W. (2004). Age-related changes in antisaccade task performance: Inhibitory control or working-memory engagement? Brain & Cognition, 56(2), 177–188. https://doi.org/10.1016/j.bandc.2004.02.077
- Eriksen, B. A., & Eriksen, C. W. (1974). Effects of noise letters upon the identification of a target letter in a nonsearch task. Perception & Psychophysics, 16(1), 143–149. https://doi.org/10.3758/BF03203267
- Faul, F., Erdfelder, E., Buchner, A., & Lang, A. G. (2009). Statistical power analyses using G*Power 3.1: Tests for correlation and regression analyses. Behavior Research Methods, 41(4), 1149–1160. https://doi.org/10.3758/BRM.41.4.1149
- Faul, F., Erdfelder, E., Lang, A. G., & Buchner, A. (2007). G*power 3: A flexible statistical power analysis program for the social, behavioral, and biomedical sciences. Behavior Research Methods, 39(2), 175–191. https://doi.org/10.3758/bf03193146
- Faust, M. E., & Balota, D. A. (1997). Inhibition of return and visuospatial attention in healthy older adults and individuals with dementia of the Alzheimer type. Neuropsychology, 11(1), 13–29. https://doi.org/10.1037/0894-4105.11.1.13
- Faust, M. E., Balota, D. A., Spieler, D. H., & Ferraro, F. R. (1999). Individual differences in information-processing rate and amount: Implications for group differences in response latency. Psychological Bulletin, 125(6), 777–799. https://doi.org/10.1037/0033-2909.125.6.777
- Fernandez Duque, D., & Black, S. E. (2006). Attentional networks in normal aging and Alzheimer’s disease. Neuropsychology, 20(2), 133–143. https://doi.org/10.1037/0894-4105.20.2.133
- Fischer, B., Biscaldi, M., & Gezeck, S. (1997). On the development of voluntary and reflexive components in human saccade generation. Brain Research, 754(1–2), 285–297. https://doi.org/10.1016/S0006-8993(97)00094-2
- Folstein, M. F., Folstein, S. E., & McHugh, P. R. (1975). “Mini-mental state”. A practical method for grading the cognitive state of patients for the clinician. Journal of Psychiatric Research, 12(3), 189–198. https://doi.org/10.1016/0022-3956(75)90026-6
- Forsyth, B., Machado, L., Jowett, T., Jakobi, H., Garbe, K., Winter, H., & Glue, P. (2016). Effects of low dose ibogaine on subjective mood state and psychological performance. Journal of Ethnopharmacology, 189, 10–13. https://doi.org/10.1016/j.jep.2016.05.022
- Friedman, N. P., & Miyake, A. (2004). The relations among inhibition and interference control functions: A latent-variable analysis. Journal of Experimental Psychology: General, 133(1), 101–135. https://doi.org/10.1037/0096-3445.133.1.101
- Gazzaley, A., Sheridan, M. A., Cooney, J. W., & D’Esposito, M. (2007). Age-related deficits in component processes of working memory. Neuropsychology, 21(5), 532–539. https://doi.org/10.1037/0894-4105.21.5.532
- Geary, D. C., Frensch, P. A., & Wiley, J. G. (1993). Simple and complex mental subtraction: Strategy choice and speed-of-processing differences in younger and older adults. Psychology and Aging, 8(2), 242–256. https://doi.org/10.1037/0882-7974.8.2.242
- Geary, D. C., & Lin, J. (1998). Numerical cognition: Age-related differences in the speed of executing biologically primary and biologically secondary processes. Experimental Aging Research, 24(2), 101–137. https://doi.org/10.1080/036107398244274
- Geary, D. C., & Wiley, J. G. (1991). Cognitive addition: Strategy choice and speed-of-processing differences in young and elderly adults. Psychology and Aging, 6(3), 474–483. https://doi.org/10.1037/0882-7974.6.3.474
- Hale, S., Rose, N. S., Myerson, J., Strube, M. J., Sommers, M., Tye-Murray, N., & Spehar, B. (2011). The structure of working memory abilities across the adult life span. Psychology and Aging, 26(1), 92. https://doi.org/10.1037/a0021483
- Harada, C. N., Natelson Love, M. C., & Triebel, K. L. (2013). Normal cognitive aging. Clinics in Geriatric Medicine, 29(4), 737–752. https://doi.org/10.1016/j.cger.2013.07.002
- Hartley, A. A., & Kieley, J. M. (1995). Adult age differences in the inhibition of return of visual attention. Psychology and Aging, 10(4), 670–683. https://doi.org/10.1037/0882-7974.10.4.670
- Hedden, T., & Gabrieli, J. D. (2004). Insights into the ageing mind: A view from cognitive neuroscience. Nature Reviews Neuroscience, 5(2), 87–96. https://doi.org/10.1038/nrn1323
- Henik, A., Singh, J., Beckley, D. J., & Rafal, R. D. (1993). Disinhibition of automatic word reading in Parkinson’s disease. Cortex, 29(4), 589–599. https://doi.org/10.1016/S0010-9452(13)80283-3
- Hester, R. L., Kinsella, G. J., & Ong, B. (2004). Effect of age on forward and backward span tasks. Journal of the International Neuropsychological Society, 10(4), 475–481. https://doi.org/10.1017/S1355617704104037
- Hoffman, L., McDowd, J. M., Atchley, P., & Dubinsky, R. (2005). The role of visual attention in predicting driving impairment in older adults. Psychology and Aging, 20(4), 610–622. https://doi.org/10.1037/0882-7974.20.4.610
- Hommel, B. (2011). The Simon effect as tool and heuristic. Acta Psychologica, 136(2), 189–202. https://doi.org/10.1016/j.actpsy.2010.04.011
- Houghton, G., & Tipper, S. P. (1996). Inhibitory mechanisms of neural and cognitive control: Applications to selective attention and sequential action. Brain & Cognition, 30(1), 20–43. https://doi.org/10.1006/brcg.1996.0003
- Hurley, R., & Machado, L. (2018). Using transcranial direct current stimulation to improve verbal working memory: A detailed review of the methodology. Journal of Clinical and Experimental Neuropsychology, 40(8), 790–804. https://doi.org/10.1080/13803395.2018.1434133
- Hutchison, K. A. (2011). The interactive effects of listwide control, item-based control, and working memory capacity on stroop performance. Journal of Experimental Psychology: Learning, Memory & Cognition, 37(4), 851–860. https://doi.org/10.1037/a0023437
- Jenkins, L., Myerson, J., Joerding, J. A., & Hale, S. (2000). Converging evidence that visuospatial cognition is more age-sensitive than verbal cognition. Psychology and Aging, 15(1), 157. https://doi.org/10.1037/0882-7974.15.1.157
- Juola, J. F., Koshino, H., Warner, C. B., McMickell, M., & Peterson, M. (2000). Automatic and voluntary control of attention in young and older adults. The American Journal of Psychology, 113(2), 159–178. https://doi.org/10.2307/1423726
- Kane, M. J., Bleckley, M. K., Conway, A. R., & Engle, R. W. (2001). A controlled-attention view of working-memory capacity. Journal of Experimental Psychology: General, 130(2), 169–183. https://doi.org/10.1037/0096-3445.130.2.169
- Kane, M. J., & Engle, R. W. (2003). Working-memory capacity and the control of attention: The contributions of goal neglect, response competition, and task set to stroop interference. Journal of Experimental Psychology: General, 132(1), 47–70. https://doi.org/10.1037/0096-3445.132.1.47
- Kemtes, K. A., & Allen, D. N. (2008). Presentation modality influences WAIS digit span performance in younger and older adults. Journal of Clinical and Experimental Neuropsychology, 30(6), 661–665. https://doi.org/10.1080/13803390701641414
- Kessels, R. P., van Zandvoort, M. J., Postma, A., Kappelle, L. J., & de Haan, E. H. (2000). The corsi block-tapping task: Standardization and normative data. Applied Neuropsychology, 7(4), 252–258. https://doi.org/10.1207/S15324826AN0704_8
- Kiefer, M., Ahlegian, M., & Spitzer, M. (2005). Working memory capacity, indirect semantic priming, and stroop interference: Pattern of interindividual prefrontal performance differences in healthy volunteers. Neuropsychology, 19(3), 332–344. https://doi.org/10.1037/0894-4105.19.3.332
- Kramer, A. F., Scialfa, C. T., Peterson, M. S., & Irwin, D. E. (2001). Attentional capture, attentional control and aging. In C. L. Folk & B. S. Gibson (Eds.), Attraction, distraction, and action: Multiple perspectives on attentional capture (pp. 293–322). Elsevier Science.
- Langley, L. K., Vivas, A. B., Fuentes, L. J., & Bagne, A. G. (2005). Differential age effects on attention-based inhibition: Inhibitory tagging and inhibition of return. Psychology and Aging, 20(2), 356–360. https://doi.org/10.1037/0882-7974.20.2.356
- Lien, M. -C., Allen, P. A., Ruthruff, E., Grabbe, J., McCann, R. S., & Remington, R. W. (2006). Visual word recognition without central attention: Evidence for greater automaticity with advancing age. Psychology and Aging, 21(3), 431–447. https://doi.org/10.1037/0882-7974.21.3.431
- Long, D. L., & Prat, C. S. (2002). Working memory and stroop interference: An individual differences investigation. Memory & Cognition, 30(2), 294–301. https://doi.org/10.3758/bf03195290
- Luft, A. R., Skalej, M., Schulz, J. B., Welte, D., Kolb, R., Burk, K., Voight, K., Voight, K. & Voight, K. (1999). Patterns of age-related shrinkage in cerebellum and brainstem observed in vivo using three-dimensional MRI volumetry. Cerebral Cortex, 9(7), 712–721. https://doi.org/10.1093/cercor/9.7.712
- Lupianez, J., Klein, R. M., & Bartolomeo, P. (2006). Inhibition of return: Twenty years after. Cognitive Neuropsychology, 23(7), 1003–1014. https://doi.org/10.1080/02643290600588095
- Machado, L. (2021). Understanding cognition and how it changes with aging, brain disease, and lifestyle choices. Journal of the Royal Society of New Zealand, 15(1), 128–142. https://doi.org/10.1080/03036758.2020.1796102
- Machado, L., & Devine, A. (2019). Endogenous modulation of compatibility effects: An investigation into the temporal dynamics. Visual Cognition, 27(2), 120–126. https://doi.org/10.1080/13506285.2019.1611684
- Machado, L., Devine, A., & Wyatt, N. (2009). Distractibility with advancing age and Parkinson’s disease. Neuropsychologia, 47(7), 1756–1764. https://doi.org/10.1016/j.neuropsychologia.2009.02.018
- Machado, L., & Franz, E. (2004). Effects of unilateral distractors: A comparison of eye movement and key press responses. The Quarterly Journal of Experimental Psychology A, Human Experimental Psychology, 57(7), 1173–1190. https://doi.org/10.1080/02724980343000710
- Machado, L., Guiney, H., & Mitchell, A. (2011). Famous faces demand attention due to reduced inhibitory processing. PLos One, 6(5), e20544. https://doi.org/10.1371/journal.pone.0020544
- Machado, L., Guiney, H., & Struthers, P. (2013). Identity-based inhibitory processing during focused attention. The Quarterly Journal of Experimental Psychology, 66(1), 138–159. https://doi.org/10.1080/17470218.2012.701651
- Machado, L., & Rafal, R. (2000a). Control of eye movement reflexes. Experimental Brain Research, 135(1), 73–80. https://doi.org/10.1007/s002210000494
- Machado, L., & Rafal, R. (2000b). Strategic control over saccadic eye movements: Studies of the fixation offset effect. Perception & Psychophysics, 62(6), 1236–1242. https://doi.org/10.3758/BF03212125
- Machado, L., & Rafal, R. D. (2004a). Control of fixation and saccades during an anti-saccade task: An investigation in humans with chronic lesions of oculomotor cortex. Experimental Brain Research, 156(1), 55–63. https://doi.org/10.1007/s00221-003-1765-1
- Machado, L., & Rafal, R. D. (2004b). Control of fixation and saccades in humans with chronic lesions of oculomotor cortex. Neuropsychology, 18(1), 115–123. https://doi.org/10.1037/0894-4105.18.1.115
- Machado, L., & Rafal, R. D. (2004c). Inhibition of return generated by voluntary saccades is independent of attentional momentum. The Quarterly Journal of Experimental Psychology A, Human Experimental Psychology, 57(5), 789–796. https://doi.org/10.1080/02724980343000486
- Machado, L., Wyatt, N., Devine, A., & Knight, B. (2007). Action planning in the presence of distracting stimuli: An investigation into the time course of distractor effects. Journal of Experimental Psychology Human Perception and Performance, 33(5), 1045–1061. https://doi.org/10.1037/0096-1523.33.5.1045
- McCabe, D. P., Robertson, C. L., & Smith, A. D. (2005). Age differences in stroop interference in working memory. Journal of Clinical and Experimental Neuropsychology, 27(5), 633–644. https://doi.org/10.1080/13803390490919218
- McCrae, C. S., & Abrams, R. A. (2001). Age-related differences in object- and location-based inhibition of return of attention. Psychology and Aging, 16(3), 437–449. https://doi.org/10.1037/0882-7974.16.3.437
- McLaughlin, P. M., Szostak, C., Binns, M. A., Craik, F. I., Tipper, S. P., & Stuss, D. T. (2010). The effects of age and task demands on visual selective attention. Canadian Journal of Experimental Psychology, 64(3), 197–207. https://doi.org/10.1037/a0020650
- McNab, F., Leroux, G., Strand, F., Thorell, L., Bergman, S., & Klingberg, T. (2008). Common and unique components of inhibition and working memory: An fMRI, within-subjects investigation. Neuropsychologia, 46(11), 2668–2682. https://doi.org/10.1016/j.neuropsychologia.2008.04.023
- Meier, M. E., & Kane, M. J. (2013). Working memory capacity and stroop interference: Global versus local indices of executive control. Journal of Experimental Psychology: Learning, Memory & Cognition, 39(3), 748–759. https://doi.org/10.1037/a0029200
- Minamoto, T., Osaka, M., & Osaka, N. (2010). Individual differences in working memory capacity and distractor processing: Possible contribution of top–down inhibitory control. Brain Research, 1335, 63–73. https://doi.org/10.1016/j.brainres.2010.03.088
- Munoz, D. P., Broughton, J. R., Goldring, J. E., & Armstrong, I. T. (1998). Age-related performance of human subjects on saccadic eye movement tasks. Experimental Brain Research, 121(4), 391–400. https://doi.org/10.1007/s002210050473
- Munoz, D. P., & Fecteau, J. H. (2002). Vying for dominance: Dynamic interactions control visual fixation and saccadic initiation in the superior colliculus. Progress in Brain Research, 140, 3–19.
- Munoz, D. P., & Istvan, P. J. (1998). Lateral inhibitory interactions in the intermediate layers of the monkey superior colliculus. Journal of Neurophysiology, 79(3), 1193–1209. https://doi.org/10.1152/jn.1998.79.3.1193
- Munoz, D. P., & Wurtz, R. H. (1993). Fixation cells in monkey superior colliculus. I. Characteristics of cell discharge. Journal of Neurophysiology, 70(2), 559–575. https://doi.org/10.1152/jn.1993.70.2.559
- Neggers, S. F., Diepen, R. M., Zandbelt, B. B., Vink, M., Mandl, R. C., & Gutteling, T. P. (2012). A functional and structural investigation of the human fronto-basal volitional saccade network. PLos One, 7(1), e29517. https://doi.org/10.1371/journal.pone.0029517
- Nieuwenhuis, S., Ridderinkhof, K. R., de Jong, R., Kok, A., & van der Molen, M. W. (2000). Inhibitory inefficiency and failures of intention activation: Age-related decline in the control of saccadic eye movements. Psychology and Aging, 15(4), 635–647. https://doi.org/10.1037/0882-7974.15.4.635
- Olincy, A., Ross, R. G., Youngd, D. A., & Freedman, R. (1997). Age diminishes performance on an antisaccade eye movement task. Neurobiology of Aging, 18(5), 483–489. https://doi.org/10.1016/s0197-4580(97)00109-7
- Owen, A. M., McMillan, K. M., Laird, A. R., & Bullmore, E. (2005). N-back working memory paradigm: A meta-analysis of normative functional neuroimaging studies. Human Brain Mapping, 25(1), 46–59. https://doi.org/10.1002/hbm.20131
- Palladino, P., & De Beni, R. (1999). Working memory in aging: Maintenance and suppression. Aging Clinical and Experimental Research, 11(5), 301–306. https://doi.org/10.1007/BF03339804
- Pelli, D. G. (1997). The VideoToolbox software for visual psychophysics: Transforming numbers into movies. Spatial Vision, 10(4), 437–442. https://doi.org/10.1163/156856897X00366
- Persad, C. C., Abeles, N., Zacks, R. T., & Denburg, N. L. (2002). Inhibitory changes after age 60 and their relationship to measures of attention and memory. The Journals of Gerontology: Series B, 57(3), 223–P232. https://doi.org/10.1093/geronb/57.3.P223
- Poliakoff, E., Coward, R. S., Lowe, C., & O’Boyle, D. J. (2007). The effect of age on inhibition of return is independent of non-ocular response inhibition. Neuropsychologia, 45(2), 387–396. https://doi.org/10.1016/j.neuropsychologia.2006.06.012
- Pratt, J., Abrams, R. A., & Chasteen, A. L. (1997). Initiation and inhibition of saccadic eye movements in younger and older adults: An analysis of the gap effect. Journals of Gerontology, Series B: Psychological Sciences & Social Sciences, 52B(2), 103. https://doi.org/10.1093/geronb/52B.2.P103
- Rafal, R., Machado, L., Ro, T., & Ingle, H. (2000). Looking forward to looking: Saccade preparation and control of the visual grasp reflex. In S. Monsell & J. Driver (Eds.), Control of cognitive processes: attention and performance XVIII (pp. 155–174). MIT Press.
- Ramos, A. A., Hamdan, A. C., & Machado, L. (2020). A meta-analysis on verbal working memory in children and adolescents with ADHD. The Clinical Neuropsychologist, 34(5), 873–898. https://doi.org/10.1080/13854046.2019.1604998
- Ramos, A. A., & Machado, L. (2021). A comprehensive meta-analysis on short-term and working memory dysfunction in parkinson’s disease. Neuropsychology Review, 31(2), 288–311. https://doi.org/10.1007/s11065-021-09480-w
- Rey-Mermet, A., & Gade, M. (2018). Inhibition in aging: What is preserved? What declines? A meta-analysis. Psychonomic Bulletin & Review, 25(5), 1695–1716. https://doi.org/10.3758/s13423-017-1384-7
- Rey-Mermet, A., Gade, M., & Oberauer, K. (2018). Should we stop thinking about inhibition? Searching for individual and age differences in inhibition ability. Journal of Experimental Psychology: Learning, Memory & Cognition, 44(4), 501–526. https://doi.org/10.1037/xlm0000450
- Richardson, J. T. E. (2011). Eta squared and partial eta squared as measures of effect size in educational research. Educational Research Review, 6(2), 135–147. https://doi.org/10.1016/j.edurev.2010.12.001
- Roberts, R. J., Hager, L. D., & Heron, C. (1994). Prefrontal cognitive processes: Working memory and inhibition in the antisaccade task. Journal of Experimental Psychology: General, 123(4), 374–393. https://doi.org/10.1037/0096-3445.123.4.374
- Roberts, R. J., & Pennington, B. F. (1996). An interactive framework for examining prefrontal cognitive processes. Developmental Neuropsychology, 12(1), 105–126. https://doi.org/10.1080/87565649609540642
- Rosen, V. M., & Engle, R. W. (1998). Working memory capacity and suppression. Journal of Memory and Language, 39(3), 418–436. https://doi.org/10.1006/jmla.1998.2590
- Salthouse, T. (2012). Consequences of age-related cognitive declines. Annual Review of Psychology, 63(1), 201–226. https://doi.org/10.1146/annurev-psych-120710-100328
- Sapir, A., Rafal, R., & Henik, A. (2002). Attending to the thalamus: Inhibition of return and nasal-temporal asymmetry in the pulvinar. Neuroreport, 13(5), 693–697. https://doi.org/10.1097/00001756-200204160-00031
- Sapir, A., Soroker, N., Berger, A., & Henik, A. (1999). Inhibition of return in spatial attention: Direct evidence for collicular generation. Nature Neuroscience, 2(12), 1053–1054. https://doi.org/10.1038/15977
- Schneider, B. A., Avivi-Reich, M., & Mozuraitis, M. (2015). A cautionary note on the use of the Analysis of Covariance (ANCOVA) in classification designs with and without within-subject factors. Frontiers in Psychology, 6(474), 474. https://doi.org/10.3389/fpsyg.2015.00474
- Sele, S., Liem, F., Merillat, S., & Jancke, L. (2020). Decline variability of cortical and subcortical regions in aging: A longitudinal study. Frontiers in Human Neuroscience, 14, 363. https://doi.org/10.3389/fnhum.2020.00363
- Song, M. K., Lin, F. C., Ward, S. E., & Fine, J. P. (2013). Composite variables: When and how. Nursing Research, 62(1), 45–49. https://doi.org/10.1097/NNR.0b013e3182741948
- Spangler, D. P., & Friedman, B. H. (2017). A little goes a long way: low working memory load is associated with optimal distractor inhibition and increased vagal control under anxiety. Frontiers in Human Neuroscience, 11, 43. https://doi.org/10.3389/fnhum.2017.00043
- Spieler, D. H., Balota, D. A., & Faust, M. E. (1996). Stroop performance in healthy younger and older adults and in individuals with dementia of the Alzheimer’s type. Journal of Experimental Psychology Human Perception and Performance, 22(2), 461–479. https://doi.org/10.1037/0096-1523.22.2.461
- Stroop, J. R. (1935). Studies of interference in serial verbal reactions. Journal of Experimental Psychology, 18(6), 643–662. https://doi.org/10.1037/h0054651
- Troyer, A. K., Leach, L., & Strauss, E. (2006). Aging and response inhibition: normative data for the Victoria Stroop test. Section B: Aging, Neuropsychology and Cognition, 13(1), 20–35. https://doi.org/10.1080/138255890968187
- Tsuchida, A., & Fellows, L. K. (2013). Are core component processes of executive function dissociable within the frontal lobes? Evidence from humans with focal prefrontal damage. Cortex, 49(7), 1790–1800. https://doi.org/10.1016/j.cortex.2012.10.014
- Unsworth, N., Schrock, J. C., & Engle, R. W. (2004). Working memory capacity and the antisaccade task: Individual differences in voluntary saccade control. Journal of Experimental Psychology: Learning, Memory & Cognition, 30(6), 1302–1321. https://doi.org/10.1037/0278-7393.30.6.1302
- Vallesi, A., Tronelli, V., Lomi, F., & Pezzetta, R. (2021). Age differences in sustained attention tasks: A meta-analysis. Psychonomic Bulletin & Review, 28(6), 1755–1775. https://doi.org/10.3758/s13423-021-01908-x
- van der Lubbe, R. H., & Verleger, R. (2002). Aging and the Simon task. Psychophysiology, 39(1), 100–110. https://doi.org/10.1111/1469-8986.3910100
- van Koningsbruggen, M. G., Pender, T., Machado, L., & Rafal, R. D. (2009). Impaired control of the oculomotor reflexes in Parkinson’s disease. Neuropsychologia, 47(13), 2909–2915. https://doi.org/10.1016/j.neuropsychologia.2009.06.018
- Verhaeghen, P. (2011). Aging and executive control: reports of a demise greatly exaggerated. Current Directions in Psychological Science, 20(3), 174–180. https://doi.org/10.1177/0963721411408772
- Verhaeghen, P., & Basak, C. (2005). Ageing and switching of the focus of attention in working memory: Results from a modified N-back task. The Quarterly Journal of Experimental Psychology A, Human Experimental Psychology, 58(1), 134–154. https://doi.org/10.1080/02724980443000241
- Vernet, M., Quentin, R., Chanes, L., Mitsumasu, A., & Valero-Cabré, A. (2014). Frontal eye field, where art thou? Anatomy, function, and non-invasive manipulation of frontal regions involved in eye movements and associated cognitive operations. Frontiers in Integrative Neuroscience, 8(66). https://doi.org/10.3389/fnint.2014.00066
- Vinke, E. J., de Groot, M., Venkatraghavan, V., Klein, S., Niessen, W. J., Ikram, M. A., & Vernooij, M. W. (2018). Trajectories of imaging markers in brain aging: The Rotterdam study. Neurobiology of Aging, 71, 32–40. https://doi.org/10.1016/j.neurobiolaging.2018.07.001
- Walhovd, K. B., Westlye, L. T., Amlien, I., Espeseth, T., Reinvang, I., Raz, N., Fjell, A. M., Fjell, A. M. & Fjell, A. M. (2011). Consistent neuroanatomical age-related volume differences across multiple samples. Neurobiology of Aging, 32(5), 916–932. https://doi.org/10.1016/j.neurobiolaging.2009.05.013
- Waszak, F., Li, S. C., & Hommel, B. (2010). The development of attentional networks: Cross-sectional findings from a life span sample. Developmental Psychology, 46(2), 337–349. https://doi.org/10.1037/a0018541
- Westland, J. C. (2010). Lower bounds on sample size in structural equation modeling. Electronic Commerce Research and Applications, 9(6), 476–487. https://doi.org/10.1016/j.elerap.2010.07.003
- White, N., Flannery, L., McClintock, A., & Machado, L. (2019). Repeated computerized cognitive testing: Performance shifts and test-retest reliability in healthy older adults. Journal of Clinical and Experimental Neuropsychology, 41(2), 179–191. https://doi.org/10.1080/13803395.2018.1526888
- White, N., Forsyth, B., Lee, A., & Machado, L. (2018). Repeated computerized cognitive testing: Performance shifts and test-retest reliability in healthy young adults. Psychological Assessment, 30(4), 539–549. https://doi.org/10.1037/pas0000503
- White, N., Naldoza-Drake, P., Black, K., Scullion, L., & Machado, L. (2020). Can improving the nutritional content of bread enhance cognition? Cognitive outcomes from a randomized controlled trial. Journal of Cognitive Enhancement, 4(2), 167–178. https://doi.org/10.1007/s41465-019-00149-0
- Wyatt, N., & Machado, L. (2013a). Distractor inhibition: Principles of operation during selective attention. Journal of Experimental Psychology Human Perception and Performance, 39(1), 245–256. https://doi.org/10.1037/a0027922
- Wyatt, N., & Machado, L. (2013b). Evidence inhibition responds reactively to the salience of distracting information during focused attention. PLos One, 8(4), e62809. https://doi.org/10.1371/journal.pone.0062809
- Zaehle, T., Sandmann, P., Thorne, J. D., Jäncke, L., & Herrmann, C. S. (2011). Transcranial direct current stimulation of the prefrontal cortex modulates working memory performance: Combined behavioural and electrophysiological evidence. BMC Neuroscience, 12(1), 2. https://doi.org/10.1186/1471-2202-12-2
- Zanto, T. P., & Gazzaley, A. (2014). Attention and Ageing. In A. C. Nobre & S. Kastner (Eds.), The oxford handbook of attention (pp. 927–971). Oxford University Press.
- Zeef, E. J., & Kok, A. (1993). Age-related differences in the timing of stimulus and response processes during visual selective attention: Performance and psychophysiological analyses. Psychophysiology, 30(2), 138–151. https://doi.org/10.1111/j.1469-8986.1993.tb01727.x
- Zeef, E. J., Sonke, C. J., Kok, A., Buiten, M. M., & Kenemans, J. L. (1996). Perceptual factors affecting age-related differences in focused attention: Performance and psychophysiological analyses. Psychophysiology, 33(5), 555–565. https://doi.org/10.1111/j.1469-8986.1996.tb02432.x
- Zhang, R., Geng, X., & Lee, T. M. C. (2017). Large-scale functional neural network correlates of response inhibition: An fMRI meta-analysis. Brain Structure & Function, 222(9), 3973–3990. https://doi.org/10.1007/s00429-017-1443-x